- 1Neurorehabilitation Research Group, Department of Rehabilitation Sciences, KU Leuven, Leuven, Belgium
- 2Department of Neurology, University Hospital Schleswig-Holstein, Christian-Albrechts-University (CAU) Kiel, Kiel, Germany
Background: Dual-tasking is challenging for people with Parkinson's disease and freezing of gait (PD+FOG) and can exacerbate freezing episodes and falls. Split-belt treadmill training (SBT) is a novel tool to train complex gait and may improve dual-task (DT) walking and turning.
Objective: To investigate the single-session effects of SBT on DT walking and DT turning performance in PD+FOG and older adults (OA), compared to regular treadmill training.
Methods: Forty-five PD+FOG and 36 OA participated in a single training session (30 min). They were randomized into one of four training groups: (A) SB75—steady belt speed ratio 0.75:1; (B) SB50—steady belt speed ratio 0.5:1; (C) SBCR—changing belt speed ratios between 0.75:1 and 0.5:1; and (D) Tied-Belt (TBT). Over-ground straight-line gait and an alternating turning in place task combined with a cognitive dual-task (DT) (auditory Stroop) were assessed pre- and post-training, and the following day (retention). Constrained longitudinal data analysis was used to investigate the training effects for all participants and for PD+FOG alone.
Results: DT gait speed improved at post-training for all groups (p < 0.001). However, SBT (SB50 and SBCR) led to larger post-training improvements compared to TBT, which were still visible at retention (SB50). For mean DT turning speed and Stroop response time while walking, only SBT groups showed significant improvements at post-training or retention. DT stride length, peak DT turning speed, and Stroop performance index while walking also showed larger gains in SBT compared to TBT. Results for PD+FOG alone showed similar effects although with smaller effect sizes.
Conclusions: A single session of SBT in PD+FOG and OA showed larger short-term effects on DT walking and turning compared to TBT. Cognitive DT performance was also improved in SBT, likely due to reduced cortical control of gait. These results illustrate the potential for SBT to improve DT during complex gait and possibly reduce fall risk in clinical and healthy populations.
Introduction
Dual-tasking is defined as the concurrent performance of two or more (motor or cognitive) goal-directed tasks (1). Dual-tasking occurs frequently in daily life activities. However, performance of these tasks deteriorates with aging and cognitive decline (2). In Parkinson's disease (PD), due to motor automaticity deficits, increased cognitive control of gait is required (3), reducing available capacity to perform secondary tasks. Evidently, dual-tasking is more affected in PD in comparison to their healthy peers (4), and even more so in persons who show freezing of gait (FOG) (5) where cognitive impairment is greater (6). FOG is an episodic phenomenon that occurs in PD and can be characterized by the patients' “feeling of the feet being glued to the floor,” and are “unable to generate effective stepping despite the intention to progress forward and reach a destination” (7). Complex gait tasks—those that require locomotor adaptation—such as turning, are known to trigger FOG, and addition of a cognitive task worsens FOG (5, 8).
For both persons with PD and healthy older adults (OA), dual-tasking during walking impacts gait, as gait speed reduces (9, 10). Although the relationship between dual-task gait speed and fall risk is not yet established, reduced usual-gait speed has been associated with higher fall risk in OA (11) and together with FOG and previous fall status predicts fall risk in PD (12). Independent of gait speed, an experimental study using a reactive balance paradigm in PD showed that dual-tasking during protective stepping led to an increased incidence of falls, with little benefit of dopaminergic medication (13). Therefore, aiming to improve dual-task gait abilities is an important therapeutic goal in these populations, particularly during complex gait situations where fall risk is higher (14, 15). Although studies showing the effects of only motor training on dual-tasking in PD are scarce (16), improving motor and cognitive task performance separately or together through consecutive or integrated task training may be effective to improve dual-tasking (16, 17).
A recent systematic review (18) on the cognitive effects of acute aerobic exercise showed that short bouts of aerobic exercise improve interference control—a subcomponent of inhibition and closely linked to dual-task performance (19). Moderator analyses further revealed that effects were larger in age groups with poorer interference control such as preadolescent children and older adults. Based on the attentional capacity model, these effects of aerobic exercise modalities such as treadmill walking would boost attentional capacity and thereby dual-tasking performance. On the other hand, improving automaticity of a motor task may reduce its attentional demands, thereby freeing up resources for secondary task performance. Treadmill training may therefore improve dual-task gait through its cognitive as well as motor effects. However, given the task-specificity of motor learning, it is unclear whether these dual-tasking gains would transfer to complex gait situations and whether these gains would be similar in PD, thus necessitating this work.
A split-belt treadmill—where the belt of each foot can be driven independently of the other—offers the possibility to train motor adaptation during gait with varying amounts of complexity. In addition to the aerobic effects on cognition, the added variation and complexity of the motor task may lead to better gait automaticity and transfer to dual-tasking during complex gait situations. Although these effects have not yet been studied, the limited research in PD (20) has shown that patients are able to adapt to split-belt perturbations similarly to OA, albeit to a lesser extent (21, 22). Evidence of treadmill-driven gait adaptation effects on dual-tasking was shown in a study in OA and PD fallers which compared 6-weeks of regular treadmill walking to treadmill walking combined with a virtual reality (VR) based locomotor adaptation training (23). The VR offered some extra motor challenges and also increased cognitive task load. The authors found that while both groups similarly improved single task walking speed, walking under dual-task conditions improved more in the group that received the locomotor plus VR training. Although VR-based treadmill training was taken up well by people with FOG (24), it may be preferable to train tasks separately in this severely affected group, both in terms of motor and cognitive abilities, to avoid overloading available resources and optimize learning (25).
In this single-session proof-of-concept study, we used split-belt training (SBT) in comparison to tied-belt training (TBT) to investigate whether SBT had greater effects on dual-task performance in PD+FOG and OA. Our aim was to compare the effects of three levels of SBT complexity to tied-belt training (TBT) on dual-task performance during two over-ground conditions, namely straight-ahead gait and alternating turning in place. Based on the arguments presented above, we hypothesized that both TBT and SBT would improve dual-task gait speed, while only SBT would improve dual-task turning speed. Further, we expected similar effects on the cognitive task outcomes in both TBT and SBT during straight-line walking, while only SBT would improve cognitive performance while turning. As a secondary aim, we compared the various SBT groups based on retention of training effects, with a view toward making recommendations for longer-term repeated-training studies. We expected that the SBT condition with the largest difference in speeds would require greater adaptation and thus lead to the largest effect sizes. Overall, we anticipated that the results of this study would elucidate the effects of SBT on dual-tasking during complex gait as a stepping stone toward reducing fall risk in vulnerable populations.
Methods
Recruitment and training of participants was conducted in two centers—namely the Department of Rehabilitation Sciences, KU Leuven, Belgium, and the Department of Neurology of the University Hospital Schleswig-Holstein, Christian-Albrechts-University of Kiel, Germany. The trial was pre-registered at ClinicalTrials.gov (NCT03725215), this study being a secondary analysis of the dual-task outcomes.
Participants
Forty-five persons with idiopathic PD as defined by the UK PD Brain Bank Criteria and FOG (as defined by the New Freezing of Gait Questionnaire—positive response to the question “did you experience Freezing episodes over the past month?”) (26) as well as 36 healthy OA were enrolled in the study between November 2017 and June 2019. Recruitment channels and numbers at each follow-up are given in Figure 1. Inclusion criteria were the ability to walk unassisted for at least 5 min (PD+FOG and OA) and to have stable antiparkinsonian medication and deep brain stimulator settings for at least 1 month to be included in the study (PD+FOG only). Exclusion criteria were neurological diseases apart from PD, treadmill exposure more than once a week, recent lower limb or back surgery (<6 months), cardiovascular risks for exercise assessed with the revised Physical Activity Readiness Questionnaire (27) (blood pressure regulator and/or antiplatelet usage permitted if no other risk), and cognitive impairment based on a Mini Mental State Examination (MMSE) score ≤ 24. The study protocol obtained ethical approval from the Ethics Committee for Research University Hospital/KU Leuven (Approval number: B322201734218) and the Ethics Committee of the University Hospital Schleswig-Holstein, Kiel (Approval number: D 454/13). Written informed consent was obtained prior to commencing screening in accordance with the declaration of Helsinki.
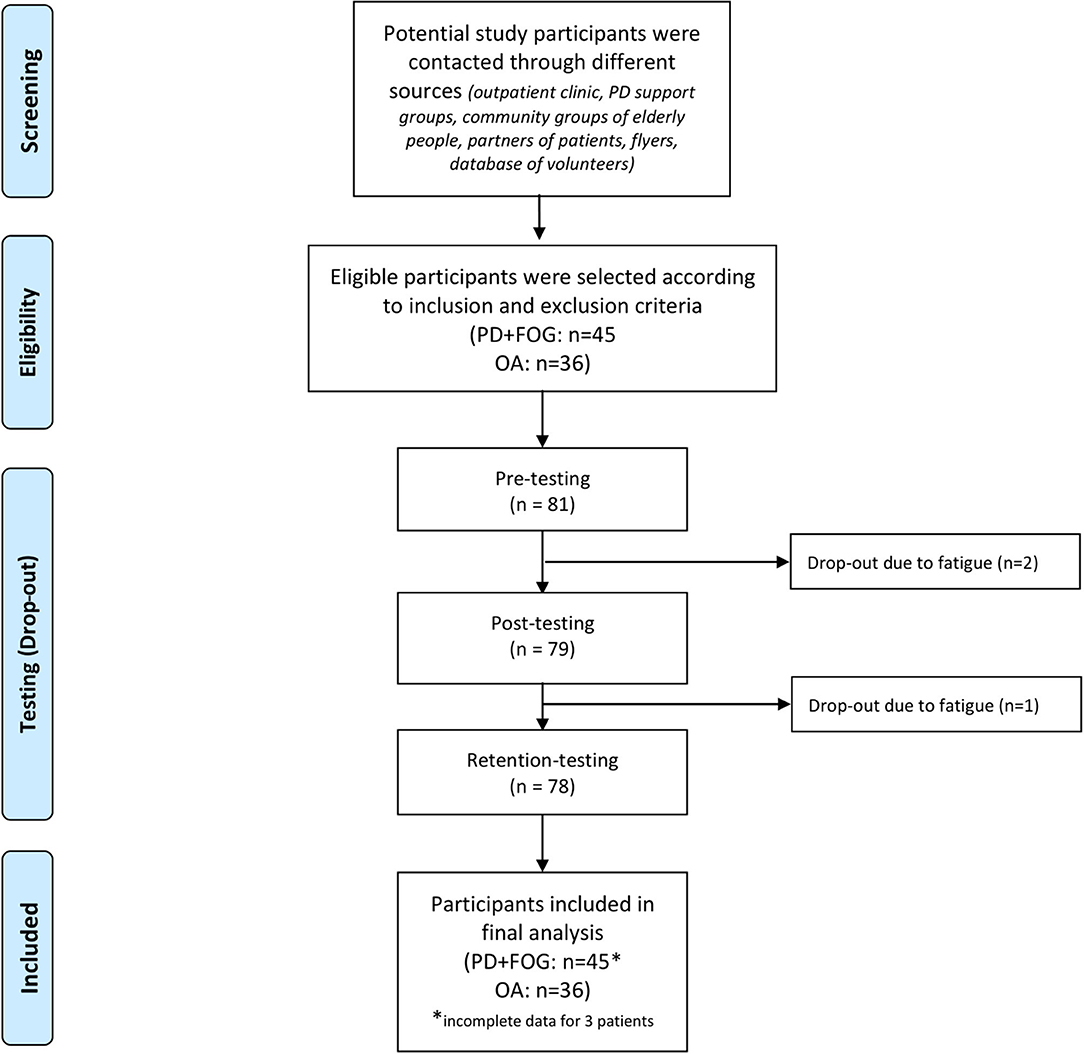
Figure 1. Flow diagram of recruitment process and study conduction; n, number of participants; PD+FOG, Parkinson's Disease with Freezing of Gait; OA, healthy older adults.
Design and Intervention
This study utilized a non-blinded randomized parallel design. On inclusion, participants were block-randomized (block size: 4) within strata based on their Hoehn and Yahr disease stage (PD+FOG—four strata, stages I–IV) or their age group (OA—four strata from 45 to 85 years, each spanning 10 years) to receive one of four interventions. For the tied-belt training (TBT), both belts were set to the training speed. For the SBT conditions, one of the belts remained at the training speed while the other was slowed down. For one split-belt training paradigm, the slow belt was set to 75% of the training speed (SB75), based on previous work (28). Another split-belt training condition utilized a larger contrast, where the slow belt was set to half the training speed (SB50). The other condition was a switching-directed split-belt training where the speed of one belt alternated between 75 and 50% of the training speed (SBCR). The length of these switches was selected to have equal numbers of blocks at each speed with at least 20 strides before each switch (average of 37.5 s per switch). Blocked practice was used as it was previously shown to improve transfer to novel tasks compared with serial practice or random practice (29).
For all SBTs, the side to be reduced was the side with the longer step length during single-task over-ground walking, based on the best-side reduction principle that was previously shown to improve worse side step length in PD+FOG (28). Training speed was personalized to 85–100% self-paced single task over-ground walking speed, based on self-reported ability to train at that speed. This was assessed during a pre-training 2-min tied-belt treadmill familiarization trial. Participants were first exposed to the over-ground walking speed, and then speed was reduced in 5% steps, based on participant feedback. Over-ground walking speed was determined based on motion capture of at least five trials of straight line walking at a steady self-selected pace. The training was delivered in six blocks of 5 min, with a 1-min break in-between. For each training block in the split-belt condition, belts accelerated simultaneously (tied) until the target speed for the slower belt was achieved, while the faster belt continued accelerating until its target speed. Similarly, at the end of the block, the faster belt decreased speed until both belts were tied, after which both sides decelerated and came to a stop. Acceleration and deceleration was set to 1 m/s2 for participant safety.
If a participant indicated difficulty in completing the training, blocks were shortened in duration rather than in number and breaks were lengthened. Participants were not made aware of their particular training condition and were asked not to look down at the belts. Handrail use while training was discouraged to maximize motor learning (30), and participants wore a harness for safety without body weight support. Feedback was restricted to mentioning if foot placement was likely to cross the midline, and regarding the amount of time remaining in the training block.
Assessments
Assessments were performed over two days at the same time of day and in the same order to standardize test timing in relationship to medication intake and circadian rhythm. Outcome measures were assessed thrice: pre-training (PRE) and post-training (POST) on day 1 and once on day 2 (retention—RET). To minimize the impact of split-belt after-effects on motor outcomes, post-training gait and turning assessments were separated from the training bout by at least 5 min of walking (~250 strides) on the treadmill as well as over-ground. PD+FOG were assessed and trained in the optimally medicated state. Demographics, cognitive, balance and clinical measures were assessed once as descriptors: Global cognition was measured with the Montreal Cognitive Assessment (MoCA) (31) and executive function with the Frontal Assessment Battery (32). Fall-related self-efficacy was assessed with the international version of the Falls Efficacy Scale (33) and balance performance with the Mini-Balance Evaluation Systems Test (Mini-BESTest) (34). Additionally, retrospective self-reported number of falls in the past 6 months was recorded. Clinical measures for PD+FOG included Hoehn and Yahr staging for randomization, the Movement Disorders Society sponsored revision of the Unified Parkinson's Disease Rating Scale motor examination part to assess disease motor burden (35), medication information from which the daily levodopa equivalent dose was calculated (36, 37) and the validated New Freezing of Gait Questionnaire (NFOGQ) for assessing FOG severity and impact (26).
Outcome Measures
In this study, we only report on dual-task outcomes, which may more closely reflect daily-living gait (38). Based on previous work that investigated the reliability of dual-task outcomes in PD (39), primary outcome measures for the motor tasks were dual-task (DT) gait speed and mean DT turning speed and for the cognitive task was DT reaction time on the auditory Stroop test. Secondary outcomes for DT gait include outcomes previously shown to decrement on dual-tasking in PD (40) such as stride length, gait speed variability, stride length variability, step width and step width variability. Secondary outcomes for DT turning included mean speed variability, peak speed, peak speed variability and mediolateral jerkiness. Secondary outcomes for the cognitive dual task were response time variability, accuracy, and performance index (measure accounting for speed-accuracy tradeoff). For all variability measures, the coefficient of variation was used [CV = (SD/mean) * 100].
Motor Tasks
Gait
Straight segments of over-ground self-paced gait over a 4-m walkway were captured using 3D motion analysis (Vicon Motion Systems, UK and Qualisys AB, Sweden), sampled at 100 Hz. Retroflective markers were placed on the heel and second toe and lateral malleolus bilaterally. Usual footwear was permitted for safety and comfort. No assistive devices were used. Ten walking trials were performed to capture at least 40 steps within the middle segment of the walkway at steady speed (41). Initial and terminal contact were determined based on the vertical velocity of the heel and toe markers (42). Spatiotemporal parameters of gait were calculated using custom scripts implemented in Matlab (version 2016b, The Mathworks, Natick, MA, USA).
Turning in Place
For the turning in place task, participants were asked to turn in place (360°) as quickly as possible for 1 min (43), alternating direction to avoid developing dizziness. Turning performance was captured with an inertial sensor placed on the lower back (OPAL™, APDM Inc., Portland, OR, USA). Angular velocity around the vertical axis was calculated from the gyroscope signal and changes in velocity direction were used to determine the start and end of each turn. Average and max angular velocity within each turn were calculated and averaged over turns to give the mean turning speed and peak turning speed. Integral of the squared time derivative of the accelerometer signal in the mediolateral direction was used to calculate turning jerkiness (44), a measure of turning fluidity. FOG episodes that occurred while turning were not excluded from the analyses, as this represents an ecological assessment of turning quality.
Cognitive Task
The auditory Stroop (45) was used for the cognitive task as it is easy to deliver and record, with low possibility of learning effects over repeated testing. Further, quantification of response time as well as accuracy is possible, allowing more sensitive measures of task performance. For this task, the words “high” and “low” were presented in either a high pitch or a low pitch. The participant was asked to name the pitch that was heard, as quickly as possible. Random sequences of stimuli at varying intervals (spaced between 0.8 and 1.2 seconds apart to minimize cueing effects) were generated separately for the gait and turning task and for pre- and post-training. For the retention assessment, the pre-training sequences were re-used. Participant responses were recorded through a wireless headset and synced with the stimuli in an audio recording software (Audacity®, version 2.2.2, audacityteam.org). Response time and accuracy of responses were manually scored on replaying the recorded audio files. Performance index was calculated to take into account the speed-accuracy tradeoff as the percentage of correct responses per second. Instructions were neutral with regards to task prioritization (46)—participants were asked “to walk/turn and respond to the cognitive task at the same time.”
Statistical Analysis
Demographic, cognitive, balance and clinical data were analyzed between training groups with one-way analysis of variance (Kruskal-Wallis for non-normal data) or chi-square likelihood tests for scalar or categorical variables, respectively. Constrained longitudinal data analysis (47) was used for the outcome analysis. This method is similar to an analysis of covariance, constraining groups to be similar at baseline as differences at baseline are due to chance and not of particular study interest. The Group*Time effect was dummy encoded to model changes from PRE compared to TBT (6 levels: 3 groups—SB75/SB50/SBCR * 2 time points—POST/RET). A linear mixed model was fit for each outcome measure as dependent variable and Age, Sex (2 levels—male and female), MoCA score, Center (2 levels—Leuven and Kiel), PD status (2 levels—PD and OA), Time (3 levels—PRE, POST, and RET) and the Group*Time effect (6 levels) as independent variables. An unstructured covariance matrix allowing for heterogeneity within each subgroup (PD/OA) of each training group (6 × 2 × 4 = 48 parameters) was used for the repeated effect of Time, after comparing the model fit of various covariance specifications (variance components, autoregressive, toeplitz) with the Akaike's Information Criteria. Satterthwaite approximation of the denominator degrees of freedom was used as the sample size was relatively small. Normality of residuals was visually assessed with histograms and Q-Q plots. Between and within-group effects over time were estimated from the model and effects sizes calculated using the t-statistic to d conversion.
(48)
Effect sizes were interpreted as small (0.2–0.49), medium (0.5–0.79), or large (>0.8), in line with recommendations (49). A contrast statement with an F-test was used to test whether any group showed a change over time (within-group effect-−8 degrees of freedom) and whether there were differences between TBT and any SBT group (Group*Time effect - 6 degrees of freedom), before undertaking post-hoc testing to determine which group differed at which time point. Multiple comparisons were corrected with the false discovery rate (FDR) procedure (50). Sensitivity analyses were performed within the PD sub-group alone using the same methodology. Analyses were performed using SAS software, version 9.4 of the SAS System for Windows (SAS Institute Inc., Cary, NC, USA) and IBM SPSS Statistics for Windows, Version 26.0 (Armonk, NY: IBM Corp).
Results
Forty-five PD+FOG (mean age: 68.5 years, SD: 10.2; 27% female) and 36 OA (mean age: 69.6 years, SD: 6.5; 44% female) were randomized into the four training groups. None of the participants had prior split-belt experience. Two PD+FOG randomized to SBT could not complete the training due to fatigue and dropped out after pre-training assessment while another PD+FOG dropped out after the post-assessment, also due to fatigue (Figure 1). The training groups were well-matched on demographic, cognitive, balance and clinical measures (p > 0.251) (Table 1). Further, training speed, training duration, handrail use and perceived intensity was also similar between-groups (p > 0.242) (Table 1). No adverse events related to the training were reported.
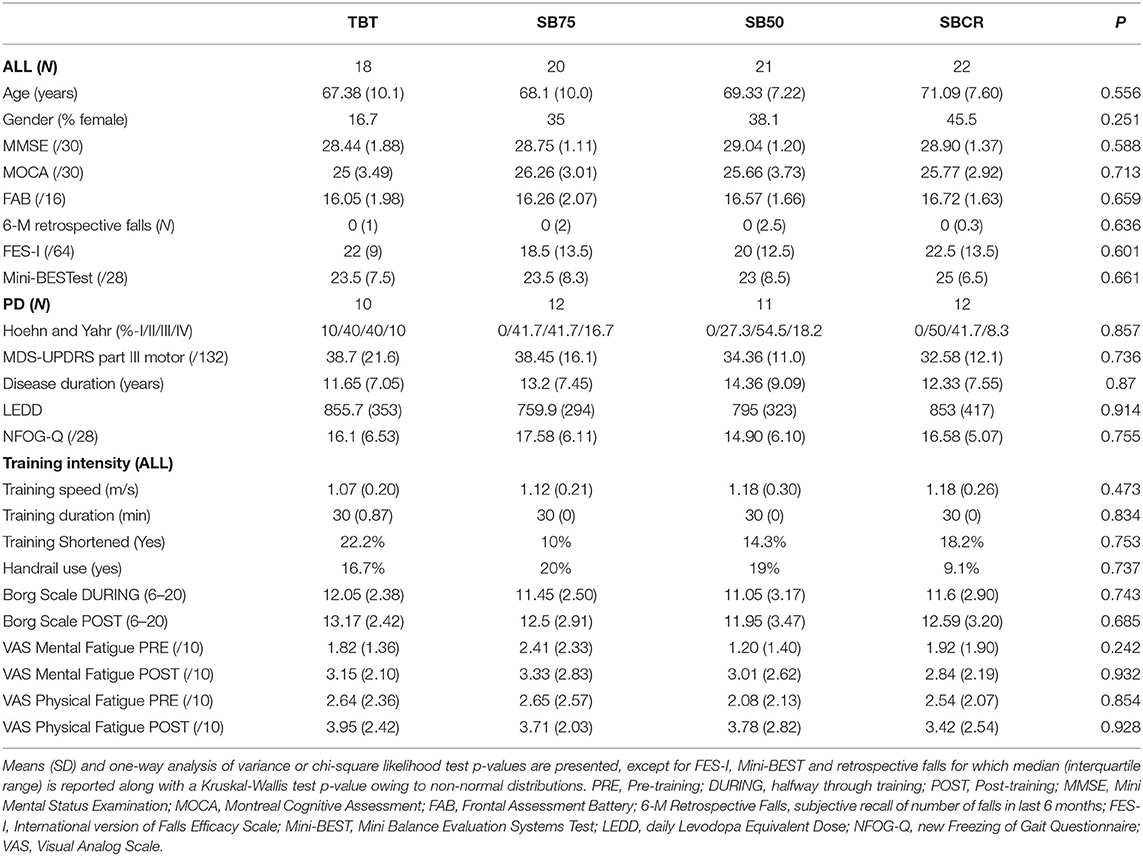
Table 1. Demographics, clinical measures (PD only) and training intensity for the four training groups.
Combined Analysis Including All Participants
Motor Tasks
Significant Group*Time effects between TBT and SBT were seen on multiple aspects of DT gait including gait speed (p = 0.002), stride length (p < 0.001), step width (p = 0.025), gait speed variability (p = 0.017), and step width variability (p < 0.001) (Table 2). Although all groups showed within-group increases in gait speed and stride length, post-hoc tests revealed that increments were larger for SB50 (gait speed: p−FDR = 0.001, stride length: p−FDR = 0.001) and SBCR (gait speed: p−FDR = 0.001, stride length: p−FDR < 0.001) at POST; and SB50 (gait speed: p−FDR = 0.02, stride length: p−FDR = 0.014) and SB75 at RET (stride length only: p−FDR = 0.05). Step width variability was significantly lower in SB50 at POST (p−FDR = 0.001), but differences were lost at RET. Post-hoc tests were not significant for step width and gait speed variability.
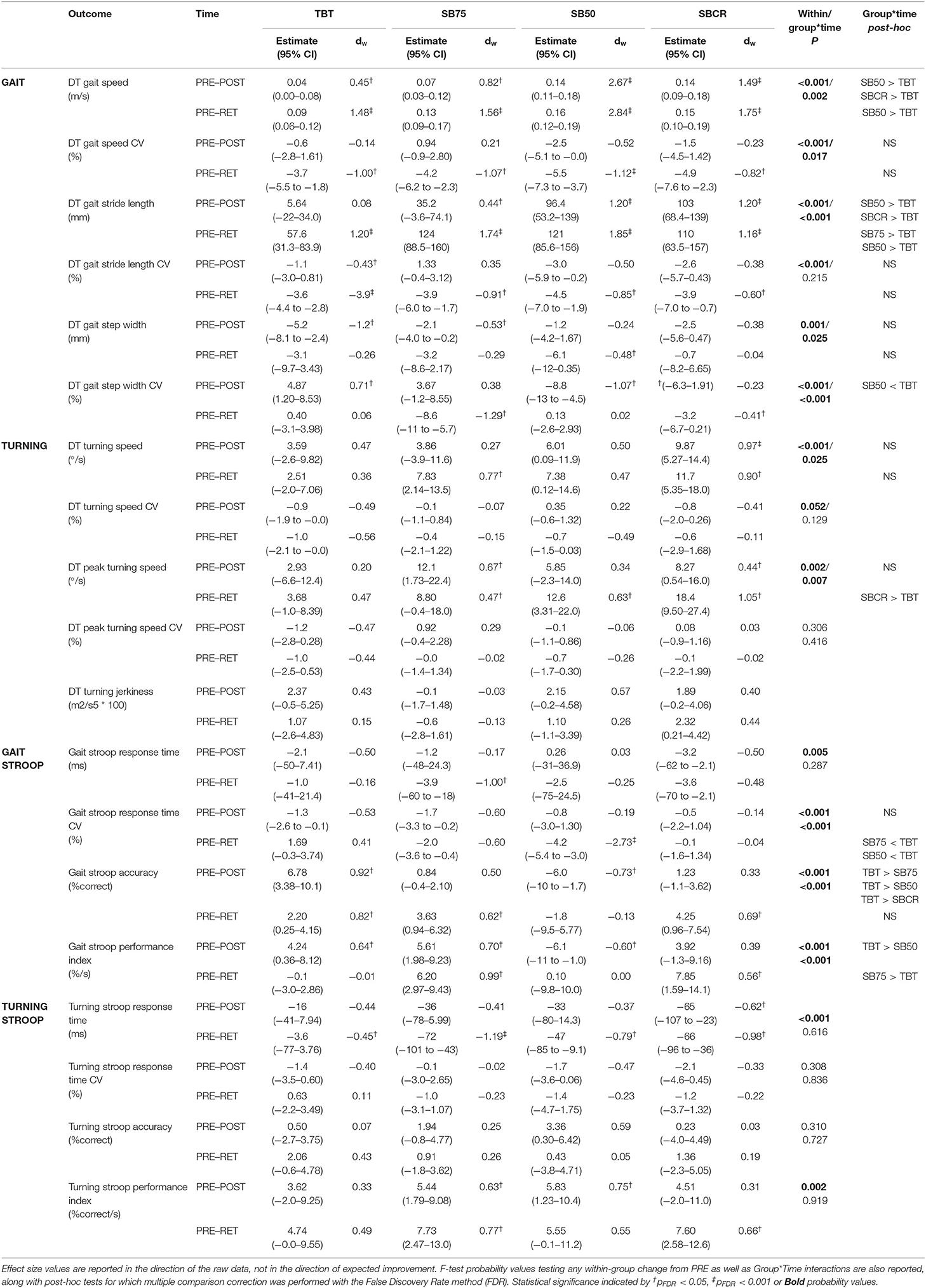
Table 2. Within-group effects for each training group (PD+FOG and OA) from PRE to POST and PRE to RET, along with 95% Confidence Intervals (CI) and effect sizes (dw from t statistic-paired).
For DT turning outcomes, significant interaction effects between TBT and SBT were found for mean (p = 0.025) and peak (p = 0.007) turning speed. Although post-hoc tests were not significant for mean turning speed, peak turning speed was significantly faster in SBCR at RET (p−FDR = 0.019). Importantly, no within-group increases in either mean or peak turning speed were found in TBT.
Cognitive Tasks
No significant interactions were found for Stroop response time while walking. However, secondary outcomes of Stroop performance while walking did show significant interactions between SBT and TBT, particularly Stroop response time variability (p < 0.001), accuracy (p < 0.001) and performance index (p < 0.001). SB75 (p−FDR = 0.016) and SB50 (p−FDR < 0.001) showed greater reductions in response time variability at RET compared to TBT. Although TBT showed higher accuracy at POST compared to SB75, SB50, and SBCR (all p−FDR < 0.01), differences were lost at RET. A similar pattern was seen for performance index with significant higher values in TBT at POST compared to SB50 (p−FDR = 0.004), while at RET, SB75 showed higher performance index compared to TBT (p−FDR = 0.031).
No significant interactions between TBT and SBT were found for cognitive task performance while turning, as all groups showed similar reductions on Stroop response time and increase in performance index at RET. Progression within OA and PD+FOG on primary outcomes is shown in Figure 2.
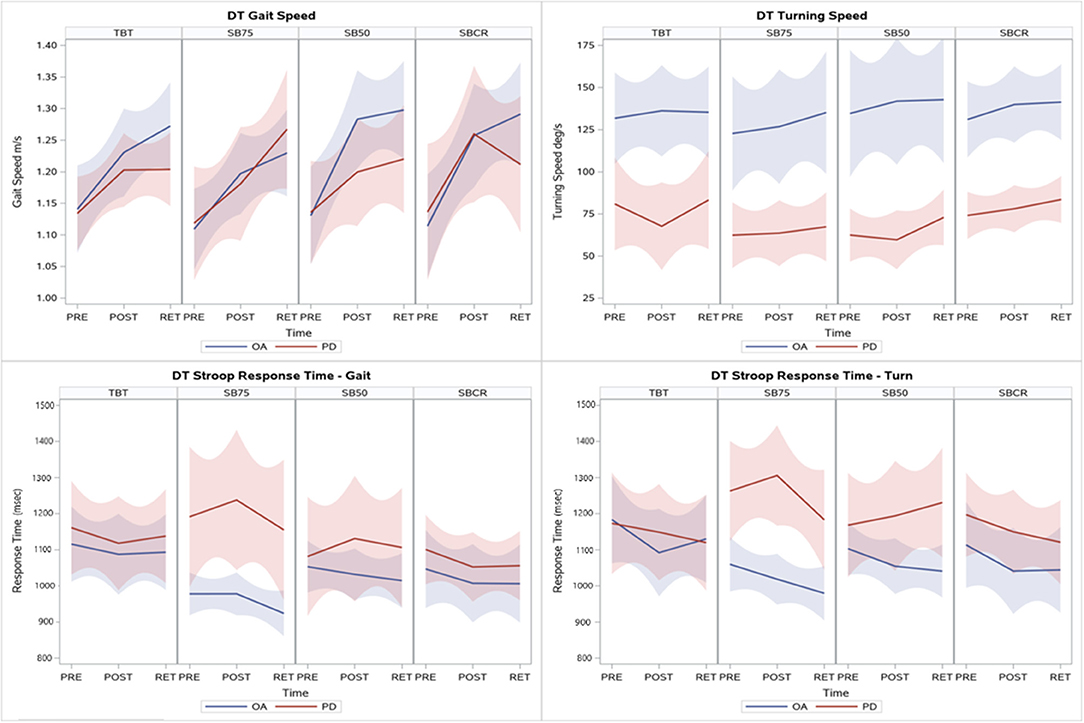
Figure 2. Locally estimated scatterplot smoothing (LOESS) regression lines and 95% confidence intervals from PRE to POST and RET for the primary outcomes fitted separately for OA and PD+FOG within each training group. In the full sample analysis combining data from OA and PD+FOG, increase in dual-task gait speed and decrease in stroop response time while turning were seen in all groups, while only SBT groups increased their dual-task turning speed and decreased stroop response time while walking. Similar results were seen in the PD+FOG sub-group, although with smaller effect sizes.
Sensitivity Analysis in PD+FOG
Motor Tasks
As with the full sample analyses, significant Group*Time interactions were found between SBT and TBT on DT stride length (p = 0.006), with post-hoc tests revealing that SBCR had larger increase in stride length at POST (p-FDR = 0.011). Differences between SBT and TBT were not significant at RET as all groups showed similar within-group increments.
Similarly, significant Group*Time interactions were found for DT mean (p = 0.039) and peak turning speed (p = 0.046). Post-hoc tests at RET showed a trend toward significantly faster turning in all SBT groups compared to TBT (all p-FDR ≤ 0.1). Additionally a significant interaction effect was found for mean turning speed variability (p = 0.037), with TBT showing a larger decrease in mean turning speed variability compared to SB50 at POST (p-FDR = 0.046). These differences were not maintained at RET.
Cognitive Tasks
Stroop accuracy (p = 0.016) and performance index (p = 0.054) showed significant interactions between TBT and SBT, however post-hoc tests were not significant.
Comparison of SBT Within-Group Effect Sizes at RET
In the full sample analysis, effect sizes at RET were largest for SB50 (dw = 2.84) for DT gait speed, while SBCR had largest effect size on mean DT turning speed (dw = 0.9), and SB75 showed largest effect sizes on Stroop response time while walking (dw = −1) and turning (dw = −1.19). When looking only at the sub-group of PD+FOG, effect sizes at RET were largest for SB75 for DT gait speed (dw = 1.37) and Stroop response time while walking (dw = −1), while SBCR showed the largest effect size for mean DT turning speed (dw = 0.77) and Stroop response time while turning (dw = −0.97). Exploration of the relative improvement (median group % change) on primary outcomes from PRE to RET showed that trade-off between improvements on motor and cognitive performance were only seen for DT turning in PD+FOG in TBT and SB50 (Figure 3).
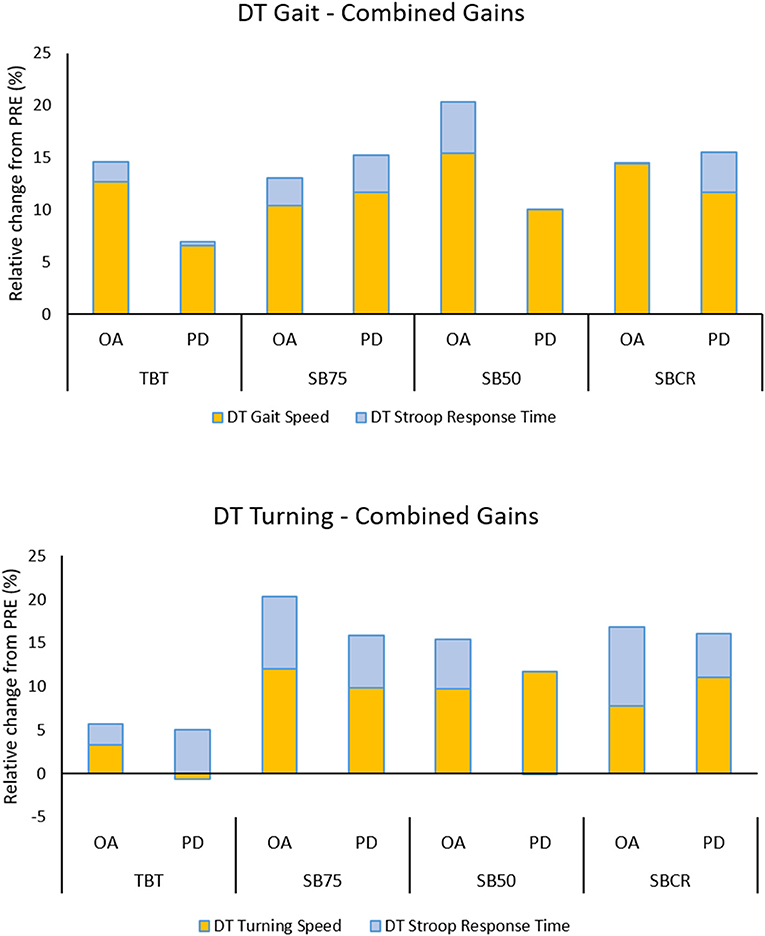
Figure 3. Investigation of trade-off between motor and cognitive gains. Relative change from PRE for motor and cognitive performance while walking (upper panel) and turning (lower panel), shown separately for training and OA and PD+FOG sub-groups. Raw change scores from PRE to RET were calculated and normalized to % PRE value. Median values for each training group and sub-group is shown on a stacked bar chart to show the additive or competitive effects of gains on the motor vs. the cognitive task. Stroop change scores were inverted, so that positive scores are better. Combined effects were consistently larger for SBT groups for DT turning, in OA as well as PD+FOG. Some tradeoff between motor and cognitive gains is seen while turning in persons with PD in TBT and SB50 groups.
Discussion
In this single-session training study, we investigated the effects of SBT and TBT on DT walking and DT turning in PD+FOG and healthy OA. In line with our hypotheses, both SBT and TBT led to improvements on DT gait speed, while only SBT improved DT turning speed. Improvements on the Stroop task while walking and turning were seen in all groups, in contrast to our expectations. However, in PD+FOG, improvements in stoop response time while turning may have come at the expense of motor improvements in the TBT group. Further, improvements in DT gait speed and Stroop performance while walking were larger in SBT compared to TBT. This transfer of SBT effects to DT walking and turning, with moderate to large effect sizes, highlights the promising role of SBT to improve DT performance in PD+FOG as well as in OA.
In their review of the effect of treadmill training in PD, Mehrholz and colleagues found it to be a robust tool to improve single-task gait, particularly gait speed and stride length (51). We were able to replicate these results under dual-task conditions (improvement in DT gait speed from PRE to RET in TBT: 0.09 m/s, 95% CI: 0.06–0.12, Mehrholz: 0.09 m/s, 95% CI: 0.03–0.14), and additionally showed that SBT led to even larger effects on these outcomes (improvement from PRE to RET in SB50: 0.16 m/s, 95% CI: 0.12–0.19), in the range of clinically meaningful improvements (52). The fact that these effects were clearly visible when attention was diverted, suggests that gait automaticity was improved by the training. The mechanism for SBT to induce stronger automatization of gait compared to TBT is unknown. Treadmill walking is externally guided as movement is driven by the belts. Externally guided movement is thought to rely strongly on cerebellar input within cerebellar-thalamo-ventral premotor networks (53). Indeed, after a 6-month treadmill training program, stroke patients showed increased activation in the cerebellum and midbrain during movement of their paretic leg, which was associated with gains in walking velocity (54). During our training, both TBT and SBT would benefit from recruitment of this network, freeing up frontal executive resources (55) for learning related processes (56). In this boosted learning environment, split-belt demands may lead to additional gains, through other mechanisms that were at play. One of these mechanisms may be imposing sensory prediction error repeatedly through these abnormal split-belt gait patterns that required motor adaptation, in which the cerebellum also plays an important role (57). Evidence supporting this mechanism comes from two studies that showed that cerebellar damage impairs SBT adaptation (58), and that cerebellar modulation with non-invasive stimulation influences spatial adaptation during SBT (59). However, the exact neurophysiological mechanism on how the relationship between varying sensory manipulations and motor responses strengthens the learning circuits within the cerebellum are still largely illusive (57). Taken together, we surmise that adaptations driven by sensory prediction error based training with SBT recruits the cerebellum and cerebellar locomotor region additionally compared to TBT, leading to larger spatial gait effects seen here.
In this study, these adaptations led to a greater increase in gait speed, stride length, and turning speed, possibly by increasing step length on the side that was slowed down. These measures are highly related, recently being shown to load on the same movement domain and to be strongly associated with attention and executive function (60). Based on the attentional capacity theory of dual-task interference, we expected that improved automaticity on the motor task would be accompanied by improved cognitive task performance, as cognitive resources would be freed from gait control. In this cohort, all groups apart from SB75 had average MoCA scores below the cutoff for mild cognitive impairment. This may suggest a diminished cognitive reserve and limited capacity for improvement. Despite this, besides response time itself, improvements on cognitive measures such as the performance index and response time variability were found. Response time variability measures the construct of fluctuating attention, which deteriorates early in PD and may be a marker of PD dementia (61). These results indicate that SBT would be beneficial even for persons with cognitive impairment, and may slow down cognitive decline. Importantly, these improvements on the cognitive task did not come at the expense of, but rather in addition to the improvements on the motor tasks (recall Figure 3). Overlap in the neural resources responsible for cognitive set-shifting and motor adaptation, may have resulted in cognitive facilitation (62), particularly while turning. One contradictory cognitive result was that TBT showed larger improvements compared to SBT on accuracy on the Stroop while walking post-training. The lack of improvement in Stroop accuracy for SBT may be explained by a difference in intensity of the training, as deterioration in accuracy of cognitive tasks has been reported after acute intermediate intensity exercise (63). Although we did not find differences on self-report measures of perceived intensity, the limping gait during SBT likely had higher energy costs than regular gait on the tied-belts. Further, these seemingly negative effects were transient as they were not detectable at retention. Moreover, performance index which takes into account the speed-accuracy tradeoff, was significantly more improved in SBT at retention. Overall, the SBT effects seen were very positive, both for the motor as well as the cognitive effects.
The effects seen in the PD sub-group alone were promising, although they seemed to suffer from the motor learning deficits known to affect PD+FOG in particular (64). In spite of this, differences between SBT and TBT were found on DT stride length and peak DT turning speed. A key difference noticed in the sensitivity analyses, was that SB50 was less effective in PD than in OA (Supplementary Table 1 and Figures 2, 3). Analysis of adaptations during the training session may provide insights into whether PD+FOG were able to adapt to the large belt-speed difference, and the large asymmetry induced. Further, interpreting the large error without any explicit feedback about the direction in which, or degree to which to adapt, can be particularly challenging for PD+FOG (65). These failings of SB50 were contrary to our expectations on the secondary aim. Effects of SB75 and SBCR seemed consistent, in both the full sample and PD+FOG sub-group. Of note, gains from the training were often larger at retention than at post-training (Table 2 and Figure 2), indicating that the training triggered offline consolidation on these tasks. Aerobic exercise has been shown to improve offline consolidation of a novel task in PD (66), and we expect that similar mechanisms were at work here for relearning previously trained tasks. These effects were particularly evident for SB75, which in contrast to the more explicit SB50 and SBCR conditions, may have relied on implicit learning processes due to the almost imperceptible belt speed differences (67). On the other hand, the effects of fatigue on post-training measurements cannot completely be ruled out, after the training and the extensive testing pre-training.
Limitations and Recommendations
This study, to our knowledge, is the first clinical study to investigate the effectiveness of treadmill training on dual-task outcomes specifically, besides presenting proof of the potential of SBT for improving dual-tasking in PD and OA. The study was slightly underpowered to detect training differences within the sub-group of PD+FOG alone. Therefore, we pooled OA and PD for the main analysis, and performed a sensitivity analysis in the PD+FOG. Apart from SB50, results for the remaining groups were very similar in both analyses. These results allayed concerns of a differential response in OA and PD, in line with previous work (21). Future work could utilize a repeated training design to improve effect sizes and power to detect differences in the PD+FOG. Another limitation of the study is that it did not include a broader sample of people with PD. While this limits the generalizability of these results, given the motor learning difficulties in PD+FOG (64), effects would likely extend to people without FOG. While interpreting the effects of SBT on turning outcomes, it is of some import to note that test-retest reliability of these turning metrics with or without dual-tasking, and their clinical relevance have not yet been established. Longer term follow-up in free-living conditions would be required to investigate transfer to daily life, as laboratory based turning may not reflect turning at home (68). Further, this study used preferred dual-task gait speed as a primary outcome. Inter-individual interpretation of the “as usual” instruction may have led to some participants measuring closer to capacity than others. On the other hand, “as fast as possible” was the instruction given for the turning in place task, which led to a similar pattern of improvements from PRE, downplaying concerns over the influence of this instruction. Future studies using gait speed as an outcome should ensure that they standardize instructions across tasks to capture true increases in dual-task capacity rather than just performance.
Although this study presents some aspects of the effects of SBT on dual-tasking with moderate to large effect sizes, these are only the immediate and short-term effects. How these would translate to dual-tasking in the daily life environment and the impact on falls and FOG should be the subject of future longer term training studies. Based on the effect sizes, SBCR would be preferred to improve dual-task turning, while SB75 might be preferred for its low challenge and stable effects, particularly in participants with cognitive decline such as PD+FOG. Alternatively, providing relevant feedback while training may assist persons with PD to adapt to SB50 similarly to OA, which may also improve outcomes. These questions are yet to be answered before personalized prescription of SBT for dual-tasking will be made possible.
Conclusion
This study investigated the effects of single-session split-belt treadmill training, compared to regular treadmill training, on dual-task walking and turning in people with PD with FOG and healthy OA. We found that split-belt training led to significantly larger effects on dual-task gait compared to tied-belt training and its effects also transferred to a dual-task turning task. Further, most of these training effects were stronger when tested the following day, suggesting offline consolidation. These results provide initial evidence of the potential for SBT to improve dual tasking during complex gait in PD as well as OA. Longer training and follow-up studies are required to further explore the impact of this training on daily function and disease burden.
Data Availability Statement
The datasets presented in this article are not readily available because of ethical and privacy reasons. Requests to access the datasets should be directed to Nicholas D'Cruz, nicholas.dcruz@kuleuven.be.
Ethics Statement
The studies involving human participants were reviewed and approved by Ethics Committee for Research UZ/KU Leuven and Ethics Committee of the University Hospital Schleswig-Holstein Kiel. The patients/participants provided their written informed consent to participate in this study.
Author Contributions
ND'C: research project execution, data acquisition, processing, statistical analysis design, execution, and manuscript writing of the first draft. JS: research project execution, data acquisition, processing, and manuscript review and critique. PG and FH: research project execution, data acquisition, and manuscript review and critique. CS and AN: research project conception, organization, research project execution, statistical analysis review and critique, and manuscript review and critique. All authors: contributed to the article and approved the submitted version.
Funding
The Jacques and Gloria Gossweiler Foundation funded this study. The funders had no role in study design, data collection and analysis, decision to publish, or preparation of the manuscript.
Conflict of Interest
The authors declare that the research was conducted in the absence of any commercial or financial relationships that could be construed as a potential conflict of interest.
Acknowledgments
The authors acknowledge Dr. Steffen Fieuws (L-BioSTAT) for his excellent statistical input.
Supplementary Material
The Supplementary Material for this article can be found online at: https://www.frontiersin.org/articles/10.3389/fneur.2020.560084/full#supplementary-material
References
1. McIsaac TL, Lamberg EM, Muratori LM. Building a framework for a dual task taxonomy. Biomed Res Int. (2015) 2015:591475. doi: 10.1155/2015/591475
2. Al-Yahya E, Dawes H, Smith L, Dennis A, Howells K, Cockburn J. Cognitive motor interference while walking: a systematic review and meta-analysis. Neurosci Biobehav Rev. (2011) 35:715–28. doi: 10.1016/j.neubiorev.2010.08.008
3. Iansek R, Danoudis M, Bradfield N. Gait and cognition in Parkinson's disease: implications for rehabilitation. Rev Neurosci. (2013) 24:293–300. doi: 10.1515/revneuro-2013-0006
4. Rochester L, Hetherington V, Jones D, Nieuwboer A, Willems AM, Kwakkel G, et al. Attending to the task: interference effects of functional tasks on walking in Parkinson's disease and the roles of cognition, depression, fatigue, and balance. Arch Phys Med Rehabil. (2004) 85:1578–85. doi: 10.1016/j.apmr.2004.01.025
5. Spildooren J, Vercruysse S, Desloovere K, Vandenberghe W, Kerckhofs E, Nieuwboer A. Freezing of gait in Parkinson's disease: the impact of dual-tasking and turning. Mov Disord. (2010) 25:2563–70. doi: 10.1002/mds.23327
6. Heremans E, Nieuwboer A, Spildooren J, Vandenbossche J, Deroost N, Soetens E, et al. Cognitive aspects of freezing of gait in Parkinson's disease: a challenge for rehabilitation. J Neural Transm. (2013) 120:543–57. doi: 10.1007/s00702-012-0964-y
7. Nutt JG, Bloem BR, Giladi N, Hallett M, Horak FB, Nieuwboer A. Freezing of gait: moving forward on a mysterious clinical phenomenon. Lancet Neurol. (2011) 10:734–44. doi: 10.1016/S1474-4422(11)70143-0
8. Snijders AH, Haaxma CA, Hagen YJ, Munneke M, Bloem BR. Freezer or non-freezer: clinical assessment of freezing of gait. Park Relat Disord. (2012) 18:149–54. doi: 10.1016/j.parkreldis.2011.09.006
9. Raffegeau TE, Krehbiel LM, Kang N, Thijs FJ, Altmann LJP, Cauraugh JH, et al. A meta-analysis: Parkinson's disease and dual-task walking. Park Relat Disord. (2019) 62:28–35. doi: 10.1016/j.parkreldis.2018.12.012
10. Smith E, Cusack T, Blake C. The effect of a dual task on gait speed in community dwelling older adults: a systematic review and meta-analysis. Gait Posture. (2016) 999:2548–60. doi: 10.1016/j.gaitpost.2015.12.017
11. Callisaya ML, Blizzard L, Schmidt MD, Martin KL, Mcginley JL, Sanders LM, et al. Gait, gait variability and the risk of multiple incident falls in older people: a population-based study. Age Ageing. (2011) 40:481–7. doi: 10.1093/ageing/afr055
12. Paul SS, Canning CG, Sherrington C, Lord SR, Close JCT, Fung VSC. Three simple clinical tests to accurately predict falls in people with Parkinson's disease. Mov Disord. (2013) 28:655–62. doi: 10.1002/mds.25404
13. Jacobs JV, Nutt JG, Carlson-Kuhta P, Allen R, Horak FB. Dual tasking during postural stepping responses increases falls but not freezing in people with Parkinson's disease. Park Relat Disord. (2014) 20:779–81. doi: 10.1016/j.parkreldis.2014.04.001
14. Pluijm SMF, Smit JH, Tromp EAM, Stel VS, Deeg DJH, Bouter LM, et al. A risk profile for identifying community-dwelling elderly with a high risk of recurrent falling: results of a 3-year prospective study. Osteoporos Int. (2006) 17:417–25. doi: 10.1007/s00198-005-0002-0
15. Berg WP, Alessio HM, Mills EM, Tong C. Circumstances and consequences of falls in independent community-dwelling older adults. Age Ageing. (1997) 26:261–8. doi: 10.1093/ageing/26.4.261
16. Intzandt B, Beck EN, Silveira CRA. The effects of exercise on cognition and gait in Parkinson's disease: a scoping review. Neurosci Biobehav Rev. (2018) 95:136–69. doi: 10.1016/j.neubiorev.2018.09.018
17. Strouwen C, Molenaar EALM, Münks L, Keus SHJ, Zijlmans JCM, Vandenberghe W, et al. Training dual tasks together or apart in Parkinson's disease: results from the DUALITY trial. Mov Disord. (2017) 32:1201–10. doi: 10.1002/mds.27014
18. Oberste M, Javelle F, Sharma S, Joisten N, Walzik D, Bloch W, et al. Effects and moderators of acute aerobic exercise on subsequent interference control: a systematic review and meta-analysis. Front Psychol. (2019) 10:2616. doi: 10.3389/fpsyg.2019.02616
19. Olszanowski M, Bajo MT, Szmalec A. A conflict monitoring account of the control mechanisms involved in dual-tasking. J Cogn Psychol. (2015) 27:704–14. doi: 10.1080/20445911.2015.1022553
20. Seuthe J, D‘Cruz N, Ginis P, Weisser B, Berg D, Deuschl G, et al. Split-belt treadmill walking in patients with Parkinson's disease: a systematic review. Gait Posture. (2019) 69:187–94. doi: 10.1016/j.gaitpost.2019.01.032
21. Roemmich RT, Nocera JR, Stegemöller EL, Hassan A, Okun MS, Hass CJ. Locomotor adaptation and locomotor adaptive learning in Parkinson's disease and normal aging. Clin Neurophysiol. (2014) 125:313–9. doi: 10.1016/j.clinph.2013.07.003
22. Mohammadi F, Bruijn SM, Vervoort G, Van Wegen EE, Kwakkel G, Verschueren S, et al. Motor switching and motor adaptation deficits contribute to freezing of gait in Parkinson's disease. Neurorehabil Neural Repair. (2015) 29:132–42. doi: 10.1177/1545968314545175
23. Mirelman A, Rochester L, Maidan I, Del Din S, Alcock L, Nieuwhof F, et al. Addition of a non-immersive virtual reality component to treadmill training to reduce fall risk in older adults (V-TIME): a randomised controlled trial. Lancet. (2016) 388:1170–82. doi: 10.1016/S0140-6736(16)31325-3
24. Bekkers EMJ, Mirelman A, Alcock L, Rochester L, Nieuwhof F, Bloem BR, et al. Do patients with parkinson's disease with freezing of gait respond differently than those without to treadmill training augmented by virtual reality? Neurorehabil Neural Repair. (2020) 34:440–9. doi: 10.1177/1545968320912756
25. Wulf G, Shea CH. Principles derived from the study of simple skills do not generalize to complex skill learning. Psychon Bull Rev. (2002) 9:185–211. doi: 10.3758/BF03196276
26. Nieuwboer A, Rochester L, Herman T, Vandenberghe W, Emil GE, Thomaes T, et al. Reliability of the new freezing of gait questionnaire: agreement between patients with Parkinson's disease and their carers. Gait Posture. (2009) 30:459–63. doi: 10.1016/j.gaitpost.2009.07.108
27. Cardinal BJ, Esters J, Cardinal MK. Evaluation of the revised physical activity readiness questionnaire in older adults. Med Sci Sports Exerc. (1996) 28:46–72. doi: 10.1249/00005768-199505001-00102
28. Fasano A, Schlenstedt C, Herzog J, Plotnik M, Rose FEM, Volkmann J, et al. Split-belt locomotion in Parkinson's disease links asymmetry, dyscoordination and sequence effect. Gait Posture. (2016) 48:6–12. doi: 10.1016/j.gaitpost.2016.04.020
29. Hinkel-Lipsker JW, Hahn ME. The effects of variable practice on locomotor adaptation to a novel asymmetric gait. Exp Brain Res. (2017) 235:2829–41. doi: 10.1007/s00221-017-5015-3
30. Buurke TJW, Lamoth CJC, van der Woude LHV, den Otter R. Handrail holding during treadmill walking reduces locomotor learning in able-bodied persons. IEEE Trans Neural Syst Rehabil Eng. (2019) 27:1753–9. doi: 10.1109/TNSRE.2019.2935242
31. Nasreddine ZS, Phillips NA, Bédirian V, Charbonneau S, Whitehead V, Collin I, et al. The montreal cognitive assessment, MoCA: a brief screening tool for mild cognitive impairment. J Am Geriatr Soc. (2005) 53:695–9. doi: 10.1111/j.1532-5415.2005.53221.x
32. Dubois B, Slachevsky A, Litvan I, Pillon B. The FAB: a frontal assessment battery at bedside. Neurology. (2000) 55:1621–6. doi: 10.1212/WNL.55.11.1621
33. Yardley L, Beyer N, Hauer K, Kempen G, Piot-Ziegler C, Todd C. Development and initial validation of the Falls Efficacy Scale-International (FES-I). Age Ageing. (2005) 34:614–9. doi: 10.1093/ageing/afi196
34. Löfgren N, Lenholm E, Conradsson D, Ståhle A, Franzén E. The mini-BESTest - a clinically reproducible tool for balance evaluations in mild to moderate Parkinson's disease? BMC Neurol. (2014) 14:235. doi: 10.1186/s12883-014-0235-7
35. Goetz CG, Tilley BC, Shaftman SR, Stebbins GT, Fahn S, Martinez-Martin P, et al. Movement disorder society-sponsored revision of the Unified Parkinson's Disease Rating Scale (MDS-UPDRS): scale presentation and clinimetric testing results. Mov Disord. (2008) 23:2129–70. doi: 10.1002/mds.22340
36. Tomlinson CL, Stowe R, Patel S, Rick C, Gray R, Clarke CE. Systematic review of levodopa dose equivalency reporting in Parkinson's disease. Mov Disord. (2010) 25:2649–53. doi: 10.1002/mds.23429
37. Schade S, Mollenhauer B, Trenkwalder C. Levodopa equivalent dose conversion factors: an updated proposal including opicapone and safinamide. Mov Disord Clin Pract. (2020) 7:343–5. doi: 10.1002/mdc3.12921
38. Hillel I, Gazit E, Nieuwboer A, Avanzino L, Rochester L, Cereatti A, et al. Is every-day walking in older adults more analogous to dual-task walking or to usual walking? Elucidating the gaps between gait performance in the lab and during 24/7 monitoring. Eur Rev Aging Phys Act. (2019) 16:6. doi: 10.1186/s11556-019-0214-5
39. Strouwen C, Molenaar EALM, Keus SHJ, Münks L, Bloem BR, Nieuwboer A. Test-retest reliability of dual-task outcome measures in people with parkinson disease. Phys Ther. (2016) 96:1276–86. doi: 10.2522/ptj.20150244
40. Kelly VE, Eusterbrock AJ, Shumway-Cook A. A review of dual-task walking deficits in people with Parkinson's disease: motor and cognitive contributions, mechanisms, and clinical implications. Parkinsons Dis. (2012) 2012:918719. doi: 10.1155/2012/918719
41. Galna B, Lord S, Rochester L. Is gait variability reliable in older adults and Parkinson's disease? Towards an optimal testing protocol. Gait Posture. (2013) 37:580–5. doi: 10.1016/j.gaitpost.2012.09.025
42. Pijnappels M, Bobbert MF, Van Dieën JH. Changes in walking pattern caused by the possibility of a tripping reaction. Gait Posture. (2001) 14:11–8. doi: 10.1016/S0966-6362(01)00110-2
43. Mancini M, Smulders K, Cohen RG, Horak FB, Giladi N, Nutt JG. The clinical significance of freezing while turning in Parkinson's disease. Neuroscience. (2017) 343:222–8. doi: 10.1016/j.neuroscience.2016.11.045
44. Bertoli M, Croce UD, Cereatti A, Mancini M. Objective measures to investigate turning impairments and freezing of gait in people with Parkinson's disease. Gait Posture. (2019) 74:187–93. doi: 10.1016/j.gaitpost.2019.09.001
45. Hegeman J, Weerdesteyn V, Van den Bemt B, Nienhuis B, Van Limbeek J, Duysens J. Dual-tasking interferes with obstacle avoidance reactions in healthy seniors. Gait Posture. (2012) 36:236–40. doi: 10.1016/j.gaitpost.2012.02.024
46. Kelly VE, Eusterbrock AJ, Shumway-Cook A. The effects of instructions on dual-task walking and cognitive task performance in people with parkinson's disease. Parkinsons Dis. (2012) 2012:671261. doi: 10.1155/2012/671261
47. Van Breukelen GJP. ANCOVA versus change from baseline had more power in randomized studies and more bias in nonrandomized studies. J Clin Epidemiol. (2006) 59:920–5. doi: 10.1016/j.jclinepi.2006.02.007
48. Lakens D. Calculating and reporting effect sizes to facilitate cumulative science: a practical primer for t-tests and ANOVAs. Front Psychol. (2013) 4:863. doi: 10.3389/fpsyg.2013.00863
49. Cohen J. Statistical Power for the Social Sciences. Hillsdale, NJ: Laurence Erlbaum Association (1988).
50. Benjamini Y, Hochberg Y. Controlling the false discovery rate: a practical and powerful approach to multiple testing. J R Stat Soc Ser B. (1995) 57:289–300. doi: 10.1111/j.2517-6161.1995.tb02031.x
51. Mehrholz J, Kugler J, Storch A, Pohl M, Hirsch K, Elsner B. Treadmill training for patients with Parkinson' s disease (review) summary of findings for the main comparison. Cochrane Collab Cochrane database Syst Rev. (2015) 2015:CD007830. doi: 10.1002/14651858.CD007830.pub3
52. Hass CJ, Bishop M, Moscovich M, Stegemöller EL, Skinner J, Malaty IA, et al. Defining the clinically meaningful difference in gait speed in persons with Parkinson disease. J Neurol Phys Ther. (2014) 38:233–8. doi: 10.1097/NPT.0000000000000055
53. Hackney ME, Lee HL, Battisto J, Crosson B, McGregor KM. Context-dependent neural activation: internally and externally guided rhythmic lower limb movement in individuals with and without neurodegenerative disease. Front Neurol. (2015) 6:251. doi: 10.3389/fneur.2015.00251
54. Luft AR, MacKo RF, Forrester LW, Villagra F, Ivey F, Sorkin JD, et al. Treadmill exercise activates subcortical neural networks and improves walking after stroke: a randomized controlled trial. Stroke. (2008) 39:3341–50. doi: 10.1161/STROKEAHA.108.527531
55. Thumm PC, Maidan I, Brozgol M, Shustak S, Gazit E, Shema Shiratzki S, et al. Treadmill walking reduces pre-frontal activation in patients with Parkinson's disease. Gait Posture. (2018) 62:384–7. doi: 10.1016/j.gaitpost.2018.03.041
56. Ridderinkhof KR, Van Den Wildenberg WPM, Segalowitz SJ, Carter CS. Neurocognitive mechanisms of cognitive control: the role of prefrontal cortex in action selection, response inhibition, performance monitoring, and reward-based learning. Brain Cogn. (2004) 56:129–40. doi: 10.1016/j.bandc.2004.09.016
57. Krakauer JW, Hadjiosif AM, Xu J, Wong AL, Haith AM. Motor learning. Compr Physiol. (2019) 9:613–63. doi: 10.1002/cphy.c170043
58. Morton SM, Bastian AJ. Cerebellar contributions to locomotor adaptations during splitbelt treadmill walking. J Neurosci. (2006) 26:9107–16. doi: 10.1523/JNEUROSCI.2622-06.2006
59. Jayaram G, Tang B, Pallegadda R, Vasudevan EVL, Celnik P, Bastian A. Modulating locomotor adaptation with cerebellar stimulation. J Neurophysiol. (2012) 107:2950–7. doi: 10.1152/jn.00645.2011
60. Morris R, Martini DN, Smulders K, Kelly VE, Zabetian CP, Poston K, et al. Cognitive associations with comprehensive gait and static balance measures in Parkinson's disease. Park Relat Disord. (2019) 69:104–10. doi: 10.1016/j.parkreldis.2019.06.014
61. Morris R, Lord S, Lawson RA, Coleman S, Galna B, Duncan GW, et al. Gait rather than cognition predicts decline in specific cognitive domains in early parkinson's disease. J Gerontol Ser A Biol Sci Med Sci. (2017) 72:1656–62. doi: 10.1093/gerona/glx071
62. Hausdorff JM, Maidan I, Bherer L, Li KZH, Mirelman A. cognitive involvement in balance, gait and dual-tasking in aging: a focused review from a neuroscience of aging perspective. Front Neurol. (2018) 9:1–13. doi: 10.3389/fneur.2018.00913
63. McMorris T, Sproule J, Turner A, Hale BJ. Acute, intermediate intensity exercise, and speed and accuracy in working memory tasks: a meta-analytical comparison of effects. Physiol Behav. (2011) 102:421–8. doi: 10.1016/j.physbeh.2010.12.007
64. Olson M, Lockhart TE, Lieberman A. Motor learning deficits in Parkinson's Disease (PD) and their effect on training response in gait and balance: a narrative review. Front Neurol. (2019) 10:62. doi: 10.3389/fneur.2019.00062
65. Ginis P, Heremans E, Ferrari A, Bekkers EMJ, Canning CG, Nieuwboer A. External input for gait in people with Parkinson's disease with and without freezing of gait: one size does not fit all. J Neurol. (2017) 264:1488–96. doi: 10.1007/s00415-017-8552-6
66. Steib S, Wanner P, Adler W, Winkler J, Klucken J, Pfeifer K. A single bout of aerobic exercise improves motor skill consolidation in parkinson's disease. Front Aging Neurosci. (2018) 10:328. doi: 10.3389/fnagi.2018.00328
67. Bekkers EMJ, Hoogkamer W, Bengevoord A, Heremans E, Verschueren SMP, Nieuwboer A. Freezing-related perception deficits of asymmetrical walking in Parkinson's disease. Neuroscience. (2017) 364:122–9. doi: 10.1016/j.neuroscience.2017.09.017
Keywords: dual-tasking, gait training, rehabilitation, freezing of gait, turning in place, constrained longitudinal data analysis
Citation: D'Cruz N, Seuthe J, Ginis P, Hulzinga F, Schlenstedt C and Nieuwboer A (2020) Short-Term Effects of Single-Session Split-Belt Treadmill Training on Dual-Task Performance in Parkinson's Disease and Healthy Elderly. Front. Neurol. 11:560084. doi: 10.3389/fneur.2020.560084
Received: 08 May 2020; Accepted: 19 August 2020;
Published: 30 September 2020.
Edited by:
Mark A. Hirsch, Carolinas Medical Center, United StatesReviewed by:
Giovanni Abbruzzese, University of Genoa, ItalyCaroline Paquette, McGill University, Canada
Copyright © 2020 D'Cruz, Seuthe, Ginis, Hulzinga, Schlenstedt and Nieuwboer. This is an open-access article distributed under the terms of the Creative Commons Attribution License (CC BY). The use, distribution or reproduction in other forums is permitted, provided the original author(s) and the copyright owner(s) are credited and that the original publication in this journal is cited, in accordance with accepted academic practice. No use, distribution or reproduction is permitted which does not comply with these terms.
*Correspondence: Nicholas D'Cruz, nicholas.dcruz@kuleuven.be