- 1Department of Neurology, State University of New York Health Sciences University, Brooklyn, NY, United States
- 2Department of Physiology & Pharmacology, State University of New York Health Sciences University, Brooklyn, NY, United States
- 3Department of Otolaryngology, North Shore Long Island Jewish Medical Center, New Hyde Park, NY, United States
- 4Department of Otolaryngology, State University of New York Health Sciences University, Brooklyn, NY, United States
- 5Department of Cell Biology, State University of New York Health Sciences University, Brooklyn, NY, United States
Sudden unexpected death in epilepsy (SUDEP) claims the lives of one in every thousand epileptic patients each year. Autonomic, cardiac, and respiratory pieces to a mechanistic puzzle have not yet been completely assembled. We propose a single sequence of causes and effects that unifies disparate and competitive concepts into a single algorithm centered on ictal obstructive apnea. Based on detailed animal studies that are sometimes impossible in humans, and striking parallels with a growing body of clinical examples, this framework (1) accounts for the autonomic, cardiac, and respiratory data to date by showing the causal relationships between specific elements, and (2) highlights specific kinds of data that can be used to precisely classify various patient outcomes. The framework also justifies a “near miss” designation to be applied to any cases with evidence of obstructive apnea even, and perhaps especially, in individuals that do not require resuscitation. Lastly, the rationale for preventative oxygen therapy is demonstrated. With better mechanistic understanding of SUDEP, we suggest changes for detection and classification to increase survival rates and improve risk stratification.
Introduction: Physiological Background and Key Concepts
Reviews of preclinical and clinical data on autonomic, cardiovascular, and respiratory contributions to sudden unexpected death in epilepsy (SUDEP) have captured the progress made toward understanding this important aspect of epilepsy (1–7). Whereas, the mechanisms for SUDEP remain unknown, the main categories of potential mechanism are (1) autonomic derangements, as these are the critical link between seizure activity and the rest of the body (2) lethal cardiac events, which can link epileptogenesis and cardiac risk among the channelopathies, and (3) apnea, which may result from seizure spread to brainstem or a catastrophic failure of brainstem circuits. The key challenge has been to demonstrate which of these is/are responsible for SUDEP given the “unexpected” nature of cases and the limitations on physiological monitoring during events.
Humans and animals have extensive pathways involving insular cortex, subiculum, and amygdala that permit seizure spread to reach hypothalamus and brainstem autonomic preganglionic and premotor neurons and thus impact all body systems with autonomic innervation (8). Changes in cardiac, respiratory, gastrointestinal, and genitourinary function before, during and after a seizure are well-known from clinical and preclinical data (2, 9–15). Significant autonomic effects of seizures occur more commonly in association with generalized tonic-clonic seizures or partial seizures originating in the temporal lobe (16–18).
In contrast to the direct ictal activation of the autonomic nervous system (ANS), there can be autonomic activity that is secondary to other ictal phenomena (e.g., hypoxemia from obstructive apnea) (13, 19, 20). Such autonomic activity is a “normal” response to protect core blood flow during a survival threat.
Repeated organ stress caused by recurring seizures or parallel pathophysiological processes as in the heritable channelopathies can lead to sustained autonomic abnormalities that impact the direct or indirect responses to seizure activity. Some have argued that an abnormal autonomic baseline is essential for the extreme physiological events leading to sudden death (21–26).
Pathways exist for seizure activity to impact respiratory rhythm generation and motor output (27–30). Reports of ictal tachypnea, bradypnea, and apnea all point to an impact of seizure activity on respiratory physiology (3, 31–35) and thereby a role in ictal oxygen desaturation (36–39).
Ictal airway obstruction has been reported in humans (40–44), and our group demonstrated laryngospasm as the basis for ictal obstructive apnea (defined as periods of no airflow with evidence of inspiratory effort) using continuous laryngoscopy, recurrent laryngeal nerve recordings, plethysmography, ECG, and EEG in a rat model (34). Obstructive apnea (OA) was accompanied by pronounced hypoxemia, followed by bradycardia, respiratory arrest, and eventually death (34, 45). Further evidence of airway obstruction as part of the SUDEP mechanism is the fact that pulmonary edema is often found at autopsy in SUDEP cases (46–50).
Ictal central apnea (defined as periods of no airflow and no evidence of respiratory effort) has been demonstrated with recordings that can distinguish central apnea from OA or respiratory arrest (34, 51–53). During ictal central apnea, the central respiratory rhythm generation continues and the respiratory motor output is inhibited in the same manner as during the apnea that occurs with voluntary breath holding or the diving response, a complex reflex that includes apnea and co-activation of the divisions of the ANS (51, 54–56). A remarkable example is the “central” apnea associated with amygdala stimulation (57, 58). The absence of “stress” during amygdala-evoked apnea and the minimal oxygen desaturation is consistent with spontaneous ictal central apnea events having resemblance to the diving response. Mouse deaths from audiogenic seizures have been suspected to involve central apnea or respiratory arrest due to brainstem disruption, particularly brainstem circuits involving serotonergic neurons (27, 29, 32, 59–64), but we showed deaths to include obstructive apnea leading to respiratory arrest (65).
Lethal arrhythmias appear to be less common. Cases of ventricular fibrillation (VF) arising from seizure activity (66, 67) or seizure-induced takotsubo cardiomyopathy (68) have been reported. Whereas, the most common cause of VF in humans is regional cardiac ischemia in the setting of myocardial infarction, global hypoxemia, such as may occur during asystole or apnea, has also been implicated in severe tachyarrhythmias (69, 70). We have shown in rats that entry into ventricular tachycardia and ventricular fibrillation could occur spontaneously under narrow conditions of moderate, but not severe hypoxia, sympathetic overdrive, and minimal vagal activity (71, 72). Whereas, VF is certainly one path to SUDEP, the existing literature indicates that it is uncommon.
Proposed Sudep Mechanism Accounts For Causes and Effects
Two recent lines of research enable us to propose a comprehensive mechanistic sequence for the majority of SUDEP cases (Figure 1). The first was the report of results from the MORTEMUS study (1), which summarized the range of autonomic, cardiac, and respiratory data between seizure onset and death from the rare human SUDEP cases that could be clearly identified as such and at the same time were accompanied by recordings of vital signs. This critical consensus established the sequence of clinical “landmarks” in SUDEP cases. The second was extensive work with invasive and non-invasive monitoring in rodents that showed how OA occurs during seizures, how OA serves as the link between a seizure and respiratory arrest (RA), and how non-invasive measures can be used to interpret human data. Demonstrations that ictal OA can be due to laryngospasm (34), that inspiratory effort can be detected with EMG (45) or inductance plethysmography (73), and even that a surrogate airway protects against death in a widely-studied mouse model of SUDEP (65) collectively argue that OA is part of a common mechanism for SUDEP. These data permit events associated with a seizure to be defined as causes or effects.
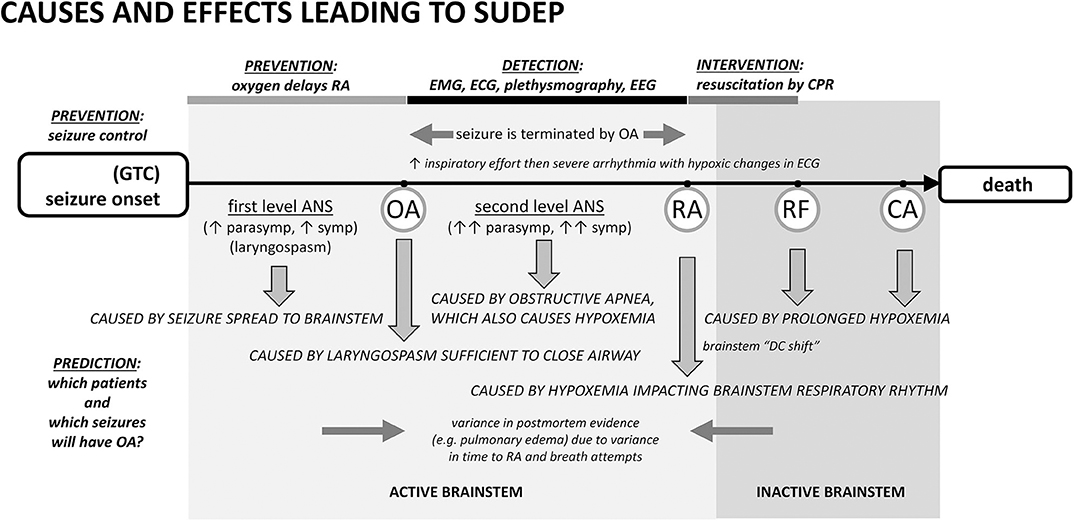
Figure 1. Proposed cascade of autonomic, cardiac, and respiratory causes and effects leading to sudden death following a seizure with points of prevention, detection, and intervention. Seizure spread to brainstem laryngomotor neurons causes laryngospasm, which can be sufficient in a minority of cases for obstructive apnea (OA). OA drives additional autonomic co-activation and is associated with a rapid oxygen desaturation. Desaturation leads to respiratory arrest (RA), respiratory failure (RF), and ultimately cardiac arrest (CA). The best-established form of prevention is seizure control. An alternative prevention is to provide oxygen at the beginning of the seizure or earlier. Oxygen can delay the time to RA long enough to permit the seizure to end spontaneously even if the airway is transiently occluded by ictal laryngospasm. In the detection period, EMG or inductance plethysmography can provide evidence of inspiratory exertion during obstructive apnea. EEG can show that the seizure was aborted. Pre- and post-mortem evidence of airway occlusion is variable because consequences such as pulmonary edema will depend upon the amount of time the airway was obstructed and the frequency and amplitude of inspiratory attempts. ANS, autonomic nervous system.
In our opinion, the sequence begins with a generalized seizure. Seizure generalization to brainstem autonomic and respiratory areas is the cause of “first level” autonomic co-activation, irregular ventilation, and laryngospasm producing partial airway occlusion. Autonomic co-activation is a source of physiological variance. Heart rate, for example, will be altered and the observed increase or decrease in ictal rate depends upon the relative levels of the autonomic components [as well as the baseline heart rate (74)]. Laryngomotor neurons are driven by seizure spread (34) and the resulting “convulsive” movement of the vocal folds (laryngospasm) occurs throughout the seizure, but an adequate airway is usually maintained. As the seizure ends spontaneously, the drive to alter autonomic and respiratory activity is eliminated (12, 74).
Occasionally laryngospasm is sufficient to cause OA (34, 52). OA is associated with intense effort to inspire, rapid desaturation, and a significant “second level” autonomic co-activation to protect core blood flow. EMG evidence of the unproductive inspiratory effort can appear in ECG and EEG records (1, 45). This measure has been used in studies of obstructive sleep apnea (75–77) and can been seen in recordings from elite apneists (e.g., breath-holding divers) (78), where stertorous breathing is not a confound. Inductance plethysmography in epilepsy patients also shows the inspiratory effort (73).
Seizures can end in two different ways after a period of OA has started. In the first, most common way, seizures end spontaneously, i.e., on their own. The stimulus for laryngospasm ends as the seizure ends. Alternatively, the hypoxemia and decreased cardiac output associated with the autonomic changes can abort the ongoing seizure activity (34, 45, 79, 80) by starving it of blood flow and oxygen. Seizure termination by asystole has been specifically noted in the clinical literature (81–83). Once aborted, recovery of baseline autonomic, cardiac, and respiratory function occurs because the seizure stimulus was removed. The full set of outcomes is illustrated in Figure 2. Based on our experience with verified OA (34), controlled airway occlusion (45), or asystole (80), the EEG can differentiate between seizures that end spontaneously and those that are aborted [see (84) for a mechanistic example in a different context]. Aborted seizure activity ends with a decrease in EEG amplitude and modest increase in EEG frequency, not the typical increase in amplitude and associated decrease in frequency.
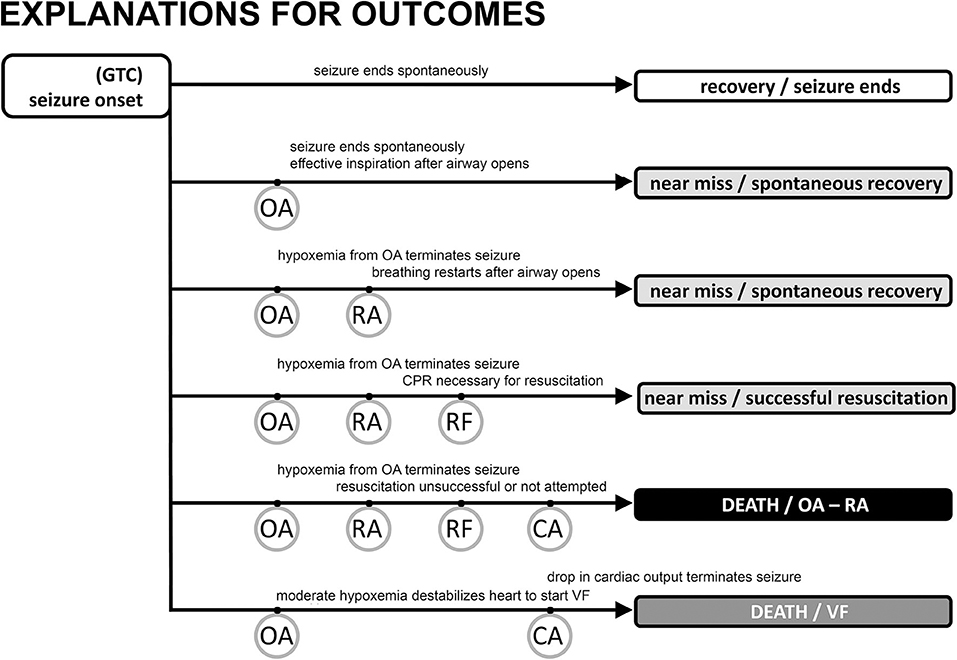
Figure 2. Possible outcomes following generalized tonic clonic seizure activity with major “landmarks.” The vast majority of seizures are associated with a spontaneous end of the seizure, no evidence of obstructive apnea, and a rapid return of autonomic, cardiac, and respiratory function to baseline levels (track 1). Obstructive apnea (OA) can occur, resulting in three types of near miss outcome (tracks 2, 3, 4) or sudden death (track 5). If the seizure ends spontaneously during OA (track 2) there may be evidence of inspiratory effort, but the EEG will show a normal pattern of decreasing frequency/increasing amplitude associated with the seizure ending on its own. Preventative oxygen treatment will successfully move any case to track 2. Seizure activity will be aborted in tracks 3 and 4 evidenced by EEG frequency increase/amplitude decrease due to a lack of brain blood flow and oxygen. The difference between tracks 3 and 4 is whether resuscitation is necessary (track 4) because of the inability to spontaneously recover breathing, i.e., respiratory failure (RF). SUDEP results from the events in tracks 5 and 6 where cardiac arrest (CA) is the endpoint. Track 5 is the sequence described in the text and shown in greater detail in Figure 1. CA is hypoxic cardiac failure in track 5. Track 6 is the outcome of seizure-induced ventricular fibrillation and a global hypoxemia trigger due to OA or asystole with or without OA.
In the second, rarer, but more dangerous way, seizure activity can persist and thus OA can last to the point of respiratory arrest (RA), defined as the point at which attempts to inspire cease (45, 53). This is a critical concept because it is the point at which spontaneous recovery is in jeopardy, and intervention by personnel other than the person experiencing the seizure may be necessary. Based on our work, the point of RA corresponds to the onset of “terminal apnea” as described in the MORTEMUS data (1). RA is distinct from respiratory failure (RF), which is defined as the point at which attempts to inspire are no longer possible. If the airway opens after the point of RA, but before RF, spontaneous recovery of respiration can sometimes occur (53). Apneic oxygenation is possible once the airway re-opens due to glottic relaxation (85), and this may account for the spontaneous recovery of respiration. Postural or positional factors can contribute to a compromised airway and block spontaneous recovery (86, 87). Based on this conceptual framework, we suggest that any case involving OA should be identified as a near-miss case, irrespective of whether the seizure ended spontaneously or was aborted, or whether the individual required resuscitation for recovery (Figure 2).
Cardiopulmonary resuscitation is known to be effective within a short time after the onset of terminal apnea/respiratory arrest (1). In our experience, a majority of cases that reach the point of RA, without resuscitation attempts, progress to respiratory failure and death (34). Cases progressing to respiratory failure will show hypoxic cardiac failure as the final sign of life.
Postictal EEG suppression (PGES) or brain shutdown, suggested as a cause of brainstem dysfunction and death (26, 88, 89) is not a cessation of brain activity (90, 91). Rather, it reflects the termination of seizure activity by hypoxemia and decreased cardiac output (12, 34, 80). The work on brainstem depolarization (92) demonstrates that this form of spreading depression likely accounts for the development of respiratory failure after reaching the point of respiratory arrest. The time to develop, the time of occurrence, and the time that would be necessary for resolution all point to brainstem depolarization occurring as a result of hypoxemia and after the point of respiratory arrest (52, 93).
Lastly, any seizure-driven cascade of events is further complicated by the possibility that (and predictive opportunity that arises when) seizures impact an abnormal background physiology due to repeated seizures, genetic variation (30, 94–97), pharmacotherapy, or other causes. None of our proposed sequence of events from seizure onset to death depends upon an abnormal background physiology.
Discussion: Application In Prevention, Intervention, and Classification
Two forms of prevention have been discussed in the literature. The most straightforward prevention is seizure control, which avoids the sequence of life-threatening events (98). A second strategy is to expose the individual to oxygen as early as possible. Oxygen, even for a short time prior to the onset of OA, delays the time to RA (53, 99, 100) and thus permits spontaneous seizure termination even if OA is present. Based on our data and proposed sequence of events, a critical preventative intervention is to enrich the inspired oxygen as near the onset of a seizure as possible, without waiting for evidence of obstructive apnea (oxygen will NOT prevent obstructive apnea, but will help the individual to survive an episode of OA). This should delay RA and prevent SUDEP (Figure 2). Oxygen would also minimize the potential for VF. We propose that any case with evidence of OA should be counted as a near miss so that in these higher risk patients, oxygen will be applied earlier, potentially preventing SUDEP.
CPR has been shown to be an effective for resuscitation within an adequate time window (1). During OA, artificial ventilation will be possible only after the laryngospasm relaxes to permit airflow. Chest compressions are important because cardiac contractility is minimal after the point of respiratory arrest (34). There is a vital race to start CPR before irreversible respiratory failure.
Risk stratification and prediction of life-threatening events remain a challenge. Post convulsive central apnea (PCCA) (70, 101) has been suggested as a predictive biomarker to stratify risk of SUDEP among epilepsy patients (102). PCCA in our framework would describe the period of time from respiratory arrest to spontaneous or assisted recovery of respiration. Prior to RA, OA accounts for the absence of airflow. PCCA is clear evidence of near miss status and this explains its predictive value.
Still missing is an answer to the question of why only some seizures have laryngospasm sufficient to cause OA. Fortunately, indicators of OA exist (e.g., our biomarker or inductance plethysmography) and can be used for risk stratification even if oxygen is delivered at the start of every seizure to prevent RA. Further complicating the classification of cases and prediction, but not complicating our mechanistic sequence, is whether the individual experiencing the seizure that causes sudden death is considered epileptic (103), i.e., what if the first seizure you have is the one that causes sudden death?
In summary, (1) we propose that obstructive apnea is the critical mechanistic link between seizure activity and respiratory and cardiac failure in the majority of SUDEP cases, (2) we recommend modifying the “near SUDEP” definition (104) to include any individuals with a near miss event because these indicate that the patient is prone to seizure-induced obstructive apnea and thus at increased risk for SUDEP, and (3) we argue that early oxygen exposure is a rational preventative step that can significantly reduce SUDEP rates. Based on our improved mechanistic understanding, we suggest changes for detection and classification of SUDEP patients to increase their survival and enhance their risk stratification.
Author Contributions
All authors listed have made a substantial, direct and intellectual contribution to the work, and approved it for publication.
Funding
This work was supported by NIH/NINDS (NS104796) and philanthropic contributions.
Conflict of Interest
The authors declare that the research was conducted in the absence of any commercial or financial relationships that could be construed as a potential conflict of interest.
References
1. Ryvlin P, Nashef L, Lhatoo SD, Bateman LM, Bird J, Bleasel A, et al. Incidence and mechanisms of cardiorespiratory arrests in epilepsy monitoring units (MORTEMUS): a retrospective study. Lancet Neurol. (2013) 12:966–77. doi: 10.1016/S1474-4422(13)70214-X
2. Bermeo-Ovalle AC, Kennedy JD, Schuele SU. Cardiac and autonomic mechanisms contributing to SUDEP. J Clin Neurophysiol. (2015) 32:21–9. doi: 10.1097/WNP.0000000000000155
3. Devinsky O, Hesdorffer DC, Thurman DJ, Lhatoo S, Richerson G. Sudden unexpected death in epilepsy: epidemiology, mechanisms, and prevention. Lancet Neurol. (2016) 15:1075–88. doi: 10.1016/S1474-4422(16)30158-2
4. Dlouhy BJ, Gehlbach BK, Richerson GB. Sudden unexpected death in epilepsy: basic mechanisms and clinical implications for prevention. J Neurol Neurosurg Psychiatry. (2016) 87:402–13. doi: 10.1136/jnnp-2013-307442
5. Barot N, Nei M. Autonomic aspects of sudden unexpected death in epilepsy (SUDEP). Clin Auton Res. (2019) 29:151–60. doi: 10.1007/s10286-018-0576-1
6. Buchanan GF. Impaired CO2-induced arousal in SIDS and SUDEP. Trends Neurosci. (2019) 42:242–50. doi: 10.1016/j.tins.2019.02.002
7. Manolis TA, Manolis AA, Melita H, Manolis AS. Sudden unexpected death in epilepsy: the neuro-cardio-respiratory connection. Seizure. (2019) 64:65–73. doi: 10.1016/j.seizure.2018.12.007
8. Jänig W. The integrative action of the autonomic nervous system: neurobiology of homeostasis. Cambridge, NY: Cambridge University Press. (2006) doi: 10.1017/CBO9780511541667
9. Goodman JH, Homan RW, Crawford IL. Kindled seizures activate both branches of the autonomic nervous system. Epilepsy Res. (1999) 34:169–76. doi: 10.1016/S0920-1211(98)00120-X
10. Baumgartner C, Lurger S, Leutmezer F. Autonomic symptoms during epileptic seizures. Epileptic Disord. (2001) 3:103−16.
11. Goodman JH, Stewart M, and Drislane FW. Autonomic Disturbances. In: editors Engel J, Pedley TA, Epilepsy: A Comprehensive Textbook. Second edition. Philadelphia, PA: Lippincott Williams and Wilkins (2008). p. 1999–2005.
12. Sakamoto K, Saito T, Orman R, Koizumi K, Lazar J, Salciccioli L, et al. Autonomic consequences of kainic acid-induced limbic cortical seizures in rats: peripheral autonomic nerve activity, acute cardiovascular changes, and death. Epilepsia. (2008) 49:982–96. doi: 10.1111/j.1528-1167.2008.01545.x
13. Hotta H, Koizumi K, Stewart M. Cardiac sympathetic nerve activity during kainic acid-induced limbic cortical seizures in rats. Epilepsia. (2009) 50:923–7. doi: 10.1111/j.1528-1167.2008.01860.x
14. Poh MZ, Loddenkemper T, Reinsberger C, Swenson NC, Goyal S, Madsen JR, et al. Autonomic changes with seizures correlate with postictal EEG suppression. Neurology. (2012) 78:1868–76. doi: 10.1212/WNL.0b013e318258f7f1
15. Surges R, Sander JW. Sudden unexpected death in epilepsy: mechanisms, prevalence, and prevention. Curr Opin Neurol. (2012) 25:201–7. doi: 10.1097/WCO.0b013e3283506714
16. Opherk C, Coromilas J, Hirsch LJ. Heart rate and EKG changes in 102 seizures: analysis of influencing factors. Epilepsy Res. (2002) 52:117–27. doi: 10.1016/S0920-1211(02)00215-2
17. Leutmezer F, Schernthaner C, Lurger S, Potzelberger K, Baumgartner C. Electrocardiographic changes at the onset of epileptic seizures. Epilepsia. (2003) 44:348–54. doi: 10.1046/j.1528-1157.2003.34702.x
18. Devinsky O. Effects of seizures on autonomic and cardiovascular function. Epilepsy Curr. (2004) 4:43–6. doi: 10.1111/j.1535-7597.2004.42001.x
19. Brostrom A, Johansson P, Stromberg A, Albers J, Martensson J, Svanborg E. Obstructive sleep apnoea syndrome–patients' perceptions of their sleep and its effects on their life situation. J Adv Nurs. (2007) 57:318–27. doi: 10.1111/j.1365-2648.2006.04110.x
20. Weiss JW, Tamisier R, Liu Y. Sympathoexcitation and Arterial Hypertension Associated with Obstructive Sleep Apnea and Cyclic Intermittent Hypoxia. J Appl Physiol. (2015) 119:1449–54. doi: 10.1152/japplphysiol.00315.2015
21. Surges R, Henneberger C, Adjei P, Scott CA, Sander JW, Walker MC. Do alterations in inter-ictal heart rate variability predict sudden unexpected death in epilepsy? Epilepsy Res. (2009) 87:277–80. doi: 10.1016/j.eplepsyres.2009.08.008
22. DeGiorgio CM, Miller P, Meymandi S, Chin A, Epps J, Gordon S, et al. RMSSD, a measure of vagus-mediated heart rate variability, is associated with risk factors for SUDEP: the SUDEP-7 Inventory. Epilepsy Behav. (2010) 19:78–81. doi: 10.1016/j.yebeh.2010.06.011
23. Toth V, Hejjel L, Fogarasi A, Gyimesi C, Orsi G, Szucs A, et al. Periictal heart rate variability analysis suggests long-term postictal autonomic disturbance in epilepsy. Eur J Neurol. (2010) 17:780–7. doi: 10.1111/j.1468-1331.2009.02939.x
24. Lotufo PA, Valiengo L, Bensenor IM, Brunoni AR. A systematic review and meta-analysis of heart rate variability in epilepsy and antiepileptic drugs. Epilepsia. (2012) 53:272–82. doi: 10.1111/j.1528-1167.2011.03361.x
25. Myers KA, Bello-Espinosa LE, Symonds JD, Zuberi SM, Clegg R, Sadleir LG, et al. Heart rate variability in epilepsy: a potential biomarker of sudden unexpected death in epilepsy risk. Epilepsia. (2018) 59:1372–80. doi: 10.1111/epi.14438
26. Okanari K, Maruyama S, Suzuki H, Shibata T, Pulcine E, Donner EJ, et al. Autonomic dysregulation in children with epilepsy with postictal generalized EEG suppression following generalized convulsive seizures. Epilepsy Behav. (2019) 102:106688. doi: 10.1016/j.yebeh.2019.106688
27. Faingold CL, Randall M, Tupal S. DBA/1 mice exhibit chronic susceptibility to audiogenic seizures followed by sudden death associated with respiratory arrest. Epilepsy Behav. (2010) 17:436–40. doi: 10.1016/j.yebeh.2010.02.007
28. Sowers LP, Massey CA, Gehlbach BK, Granner MA, Richerson GB. Sudden unexpected death in epilepsy: fatal post-ictal respiratory and arousal mechanisms. Respir Physiol Neurobiol. (2013) 189:315–23. doi: 10.1016/j.resp.2013.05.010
29. Faingold CL, Kommajosyula SP, Long X, Plath K, Randall M. Serotonin and sudden death: Differential effects of serotonergic drugs on seizure-induced respiratory arrest in DBA/1 mice. Epilepsy Behav. (2014) 37C:198–203. doi: 10.1016/j.yebeh.2014.06.028
30. Massey CA, Sowers LP, Dlouhy BJ, Richerson GB. Mechanisms of sudden unexpected death in epilepsy: the pathway to prevention. Nat Rev Neurol. (2014) 10:271–82. doi: 10.1038/nrneurol.2014.64
31. Nashef L, Walker F, Allen P, Sander JW, Shorvon SD, Fish DR. Apnoea and bradycardia during epileptic seizures: relation to sudden death in epilepsy. J Neurol Neurosurg Psychiatry. (1996) 60:297–300. doi: 10.1136/jnnp.60.3.297
32. Blum AS. Respiratory physiology of seizures. J Clin Neurophysiol. (2009) 26:309–15. doi: 10.1097/WNP.0b013e3181b7f14d
33. Seyal M, Bateman LM. Ictal apnea linked to contralateral spread of temporal lobe seizures: intracranial EEG recordings in refractory temporal lobe epilepsy. Epilepsia. (2009) 50:2557–62. doi: 10.1111/j.1528-1167.2009.02245.x
34. Nakase K, Kollmar R, Lazar J, Arjomandi H, Sundaram K, Silverman J, et al. Laryngospasm, central and obstructive apnea during seizures: defining pathophysiology for sudden death in a rat model. Epilepsy Res. (2016) 128:126–39. doi: 10.1016/j.eplepsyres.2016.08.004
35. Lacuey N, Zonjy B, Hampson JP, Rani MRS, Zaremba A, Sainju RK, et al. The incidence and significance of periictal apnea in epileptic seizures. Epilepsia. (2018) 59:573–82. doi: 10.1111/epi.14006
36. Johnston SC, Horn JK, Valente J, Simon RP. The role of hypoventilation in a sheep model of epileptic sudden death. Ann Neurol. (1995) 37:531–7. doi: 10.1002/ana.410370416
37. Johnston SC, Siedenberg R, Min JK, Jerome EH, Laxer KD. Central apnea and acute cardiac ischemia in a sheep model of epileptic sudden death. Ann Neurol. (1997) 42:588–94. doi: 10.1002/ana.410420409
38. Bateman LM, Li CS, Seyal M. Ictal hypoxemia in localization-related epilepsy: analysis of incidence, severity and risk factors. Brain. (2008) 131(Pt 12):3239–45. doi: 10.1093/brain/awn277
39. Seyal M, Bateman LM, Albertson TE, Lin TC, Li CS. Respiratory changes with seizures in localization-related epilepsy: analysis of periictal hypercapnia and airflow patterns. Epilepsia. (2010) 51:1359–64. doi: 10.1111/j.1528-1167.2009.02518.x
40. Fogel EJ, Hinderer KH. Laryngospasm: its incidence and management in electro-convulsive treatment. Dis Nerv Syst. (1958) 19:61–5.
41. Ravindran M. Temporal lobe seizure presenting as “laryngospasm”. Clin Electroencephalogr. (1981) 12:139–40. doi: 10.1177/155005948101200307
42. Amir J, Ashkenazi S, Schonfeld T, Weitz R, Nitzan M. Laryngospasm as a single manifestation of epilepsy. Arch Dis Child. (1983) 58:151–3. doi: 10.1136/adc.58.2.151
43. Murray RC, Powell D, Curry JM, Sperling MR, Evans JJ, Spiegel JR. Epileptic laryngospasm presenting as a primary sleep disturbance. Arch Otolaryngol Head Neck Surg. (2010) 136:1025–7. doi: 10.1001/archoto.2010.174
44. Tavee J, Morris H, III. Severe postictal laryngospasm as a potential mechanism for sudden unexpected death in epilepsy: a near-miss in an EMU. Epilepsia. (2008) 49:2113–17. doi: 10.1111/j.1528-1167.2008.01781.x
45. Stewart M, Kollmar R, Nakase K, Silverman J, Sundaram K, Orman R, et al. Obstructive apnea due to laryngospasm links ictal to postictal events in SUDEP cases and offers practical biomarkers for review of past cases and prevention of new ones. Epilepsia. (2017) 58:e87–e90. doi: 10.1111/epi.13765
46. Antoniuk SA, Oliva LV, Bruck I, Malucelli M, Yabumoto S, Castellano JL. Sudden unexpected, unexplained death in epilepsy autopsied patients. Arq Neuropsiquiatr. (2001) 59:40–5. doi: 10.1590/S0004-282X2001000100009
47. Salmo EN, Connolly CE. Mortality in epilepsy in the west of Ireland: a 10-year review. Ir J Med Sci. (2002) 171:199–201. doi: 10.1007/BF03170280
48. Swallow RA, Hillier CE, Smith PE. Sudden unexplained death in epilepsy (SUDEP) following previous seizure-related pulmonary oedema: case report and review of possible preventative treatment. Seizure. (2002) 11:446–8. doi: 10.1053/seiz.2002.0683
49. Pezzella M, Striano P, Ciampa C, Errichiello L, Penza P, Striano S. Severe pulmonary congestion in a near miss at the first seizure: further evidence for respiratory dysfunction in sudden unexpected death in epilepsy. Epilepsy Behav. (2009) 14:701–2. doi: 10.1016/j.yebeh.2009.02.012
50. Kennedy JD, Hardin KA, Parikh P, Li CS, Seyal M. Pulmonary edema following generalized tonic clonic seizures is directly associated with seizure duration. Seizure. (2015) 27:19–24. doi: 10.1016/j.seizure.2015.02.023
51. Villiere SM, Nakase K, Kollmar R, Silverman J, Sundaram K, Stewart M. Seizure-associated central apnea in a rat model: evidence for resetting the respiratory rhythm and activation of the diving reflex. Neurobiol Dis. (2017) 101:8–15. doi: 10.1016/j.nbd.2017.01.008
52. Jefferys JGR, Arafat MA, Irazoqui PP, Lovick TA. Brainstem activity, apnea, and death during seizures induced by intrahippocampal kainic acid in anaesthetized rats. Epilepsia. (2019) 60:2346–58. doi: 10.1111/epi.16374
53. Mooney S, Kollmar R, Gurevich R, Tromblee J, Banerjee A, Sundaram K, et al. An oxygen-rich atmosphere or systemic fluoxetine extend the time to respiratory arrest in a rat model of obstructive apnea. Neurobiol Dis. (2019) 134:104682. doi: 10.1016/j.nbd.2019.104682
54. Gooden BA. Mechanism of the human diving response. Integr Physiol Behav Sci. (1994) 29:6–16. doi: 10.1007/BF02691277
55. Panneton WM. The mammalian diving response: an enigmatic reflex to preserve life? Physiology (Bethesda). (2013) 28:284–97. doi: 10.1152/physiol.00020.2013
56. Mooney S, Chin B, Villiere S, Nakase K, Kollmar R, Kim S, et al. Diving responses elicited by nasopharyngeal irrigation mimic seizure-associated central apneic episodes in a rat model. Neurobiol Dis. (2019) 124:408–15. doi: 10.1016/j.nbd.2018.12.019
57. Dlouhy BJ, Gehlbach BK, Kreple CJ, Kawasaki H, Oya H, Buzza C, et al. Breathing inhibited when seizures spread to the amygdala and upon amygdala stimulation. J Neurosci. (2015) 35:10281–9. doi: 10.1523/JNEUROSCI.0888-15.2015
58. Lacuey N, Zonjy B, Londono L, Lhatoo SD. Amygdala and hippocampus are symptomatogenic zones for central apneic seizures. Neurology. (2017) 88:701–5. doi: 10.1212/WNL.0000000000003613
59. Tupal S, Faingold CL. Evidence supporting a role of serotonin in modulation of sudden death induced by seizures in DBA/2 mice. Epilepsia. (2006) 47:21–6. doi: 10.1111/j.1528-1167.2006.00365.x
60. Faingold CL, Tupal S, Randall M. Prevention of seizure-induced sudden death in a chronic SUDEP model by semichronic administration of a selective serotonin reuptake inhibitor. Epilepsy Behav. (2011) 22:186–90. doi: 10.1016/j.yebeh.2011.06.015
61. Richerson GB, Buchanan GF. The serotonin axis: shared mechanisms in seizures, depression, and SUDEP. Epilepsia. (2011) 52 (Suppl. 1):28–38. doi: 10.1111/j.1528-1167.2010.02908.x
62. Feng HJ, Faingold CL. Abnormalities of serotonergic neurotransmission in animal models of SUDEP. Epilepsy Behav. (2017) 71(Pt B):174–80. doi: 10.1016/j.yebeh.2015.06.008
63. Murugesan A, Rani MRS, Hampson J, Zonjy B, Lacuey N, Faingold CL, et al. Serum serotonin levels in patients with epileptic seizures. Epilepsia. (2018) 59:e91–e97. doi: 10.1111/epi.14198
64. Murugesan A, Rani MRS, Vilella L, Lacuey N, Hampson JP, Faingold CL, et al. Postictal serotonin levels are associated with peri-ictal apnea. Neurology. (2019) 93:e1485–94. doi: 10.1212/WNL.0000000000008244
65. Irizarry R, Sukato D, Kollmar R, Schild S, Silverman JB, Sundaram K, et al. Seizures induce obstructive apnea in DBA/2J audiogenic seizure-prone mice: lifesaving impact of tracheal implants. Epilepsia. (2020) 61:e13–e16. doi: 10.1111/epi.16431
66. Espinosa PS, Lee JW, Tedrow UB, Bromfield EB, Dworetzky BA. Sudden unexpected near death in epilepsy: malignant arrhythmia from a partial seizure. Neurology. (2009) 72:1702–3. doi: 10.1212/WNL.0b013e3181a55f90
67. Ferlisi M, Tomei R, Carletti M, Moretto G, Zanoni T. Seizure induced ventricular fibrillation: a case of near-SUDEP. Seizure. (2013) 22:249–51. doi: 10.1016/j.seizure.2012.12.008
68. Cunnington C, Garg S, Balachandran KP. Seizure-associated takotsubo cardiomyopathy presenting with unheralded ventricular fibrillation. Int J Cardiol. (2012) 162:e21–23. doi: 10.1016/j.ijcard.2012.05.118
69. Fava C, Montagnana M, Favaloro EJ, Guidi GC, Lippi G. Obstructive sleep apnea syndrome and cardiovascular diseases. Semin Thromb Hemost. (2011) 37:280–97. doi: 10.1055/s-0031-1273092
70. Jin L, Zhang Y, Wang XL, Zhang WJ, Liu YH, Jiang Z. Postictal apnea as an important mechanism for SUDEP: a near-SUDEP with continuous EEG-ECG-EMG recording. J Clin Neurosci. (2017) 43:130–2. doi: 10.1016/j.jocn.2017.04.035
71. Naggar I, Uchida S, Kamran H, Lazar J, Stewart M. Autonomic boundary conditions for ventricular fibrillation and their implications for a novel defibrillation technique. J Physiol Sci. (2012) 62:479–92. doi: 10.1007/s12576-012-0225-8
72. Naggar I, Stewart M. A rat model for exploring the contributions of ventricular arrhythmias to sudden death in epilepsy. In: Lathers CM, Schraeder PL, Leestma JE, Wannamaker BB, Verrier RL, Schachter SC, editors. Sudden Unexpected Death in Epilepsy: Mechanisms and New Methods for Analyzing Risks. Boca Raton, FL: Taylor & Francis (2015). p. 241–50. doi: 10.1201/b18304-29
73. Lacuey N, Vilella L, Hampson JP, Sahadevan J, Lhatoo SD. Ictal laryngospasm monitored by video-EEG and polygraphy: a potential SUDEP mechanism. Epileptic Disord. (2018) 20:146–50. doi: 10.1684/epd.2018.0964
74. Stewart M. An explanation for sudden death in epilepsy (SUDEP). J Physiol Sci. (2018) 68:307–20. doi: 10.1007/s12576-018-0602-z
75. Berry RB, Ryals S, Girdhar A, Wagner MH. Use of chest wall electromyography to detect respiratory effort during polysomnography. J Clin Sleep Med. (2016) 12:1239–44. doi: 10.5664/jcsm.6122
76. Berry RB, Ryals S, Wagner MH. Use of chest wall EMG to classify hypopneas as obstructive or central. J Clin Sleep Med. (2018) 14:725–33. doi: 10.5664/jcsm.7092
77. Berry RB, Ryals S, Dibra M, Wagner MH. Use of a transformed ECG signal to detect respiratory effort during apnea. J Clin Sleep Med. (2019) 15:9910998. doi: 10.5664/jcsm.7880
78. Bain AR, Drvis I, Dujic Z, MacLeod DB, Ainslie PN. Physiology of static breath holding in elite apneists. Exp Physiol. (2018) 103:635–51. doi: 10.1113/EP086269
79. Hotta H, Lazar J, Orman R, Koizumi K, Shiba K, Kamran H, et al. Vagus nerve stimulation-induced bradyarrhythmias in rats. Auton Neurosci. (2009) 151:98–105. doi: 10.1016/j.autneu.2009.07.008
80. Hotta H, Watanabe N, Orman R, Stewart M. Efferent and afferent vagal actions on cortical blood flow and kainic acid-induced seizure activity in urethane anesthetized rats. Auton Neurosci. (2010) 156:144–8. doi: 10.1016/j.autneu.2010.04.010
81. Schuele SU, Bermeo AC, Alexopoulos AV, Burgess RC. Anoxia-ischemia: a mechanism of seizure termination in ictal asystole. Epilepsia. (2010) 51:170–3. doi: 10.1111/j.1528-1167.2009.02168.x
82. Moseley BD, Ghearing GR, Benarroch EE, Britton JW. Early seizure termination in ictal asystole. Epilepsy Res. (2011) 97:220–4. doi: 10.1016/j.eplepsyres.2011.08.008
83. van der Lende M, Surges R, Sander JW, Thijs RD. Cardiac arrhythmias during or after epileptic seizures. J Neurol Neurosurg Psychiatry. (2016) 87:69–74. doi: 10.1016/j.autneu.2015.07.066
84. Naggar I, Stewart M, Orman R. High frequency oscillations in rat hippocampal slices: origin, frequency characteristics, and spread. Front Neurol. (2020) 11:326. doi: 10.3389/fneur.2020.00326
85. Lyons C, Callaghan M. Uses and mechanisms of apnoeic oxygenation: a narrative review. Anaesthesia. (2019) 74:497–507. doi: 10.1111/anae.14565
86. Liebenthal JA, Wu S, Rose S, Ebersole JS, Tao JX. Association of prone position with sudden unexpected death in epilepsy. Neurology. (2015) 84:703–9. doi: 10.1212/WNL.0000000000001260
87. Mahr K, Bergmann MP, Kay L, Moller L, Reif PS, Willems LM, et al. Prone, lateral, or supine positioning at seizure onset determines the postictal body position: a multicenter video-EEG monitoring cohort study. Seizure. (2020) 76:173–8. doi: 10.1016/j.seizure.2020.02.008
88. McLean BN, Wimalaratna S. Sudden death in epilepsy recorded in ambulatory EEG. J Neurol Neurosurg Psychiatry. (2007) 78:1395–7. doi: 10.1136/jnnp.2006.088492
89. Seyal M, Hardin KA, Bateman LM. Postictal generalized EEG suppression is linked to seizure-associated respiratory dysfunction but not postictal apnea. Epilepsia. (2012) 53:825–31. doi: 10.1111/j.1528-1167.2012.03443.x
90. Altenmuller DM, Schulze-Bonhage A, Elger CE, Surges R. Local brain activity persists during apparently generalized postictal EEG suppression. Epilepsy Behav. (2016) 62:218–24. doi: 10.1016/j.yebeh.2016.07.008
91. Kang JY, Rabiei AH, Myint L, Nei M. Equivocal significance of post-ictal generalized EEG suppression as a marker of SUDEP risk. Seizure. (2017) 48:28–32. doi: 10.1016/j.seizure.2017.03.017
92. Aiba I, Noebels JL. Spreading depolarization in the brainstem mediates sudden cardiorespiratory arrest in mouse SUDEP models. Sci Transl Med. (2015) 7:282ra246. doi: 10.1126/scitranslmed.aaa4050
93. Loonen ICM, Jansen NA, Cain SM, Schenke M, Voskuyl RA, Yung AC, et al. Brainstem spreading depolarization and cortical dynamics during fatal seizures in Cacna1a S218L mice. Brain. (2019) 142:412–25. doi: 10.1093/brain/awy325
94. Faingold C, Tupal S, N'Gouemo P. Genetic models of reflex epilepsy and SUDEP in rats and mice. In: Pitkänen A, Buckmaster PS, Galanopoulou AS, Moshé SL. Models of Seizures and Epilepsy. London: Elsevier/Academic Press (2017). p. 441–453. doi: 10.1016/B978-0-12-804066-9.00032-8
95. Ruthirago D, Julayanont P, Karukote A, Shehabeldin M, Nugent K. Sudden unexpected death in epilepsy: ongoing challenges in finding mechanisms and prevention. Int J Neurosci. (2018) 128:1052–60. doi: 10.1080/00207454.2018.1466780
96. Coll M, Oliva A, Grassi S, Brugada R, Campuzano O. Update on the genetic basis of sudden unexpected death in epilepsy. Int J Mol Sci. (2019) 20:1979. doi: 10.3390/ijms20081979
97. Chahal CAA, Salloum MN, Alahdab F, Gottwald JA, Tester DJ, Anwer LA, et al. Systematic review of the genetics of sudden unexpected death in epilepsy: potential overlap with sudden cardiac death and arrhythmia-related genes. J Am Heart Assoc. (2020) 9:e012264. doi: 10.1161/JAHA.119.012264
98. DeGiorgio CM, Curtis A, Hertling D, Moseley BD. Sudden unexpected death in epilepsy: risk factors, biomarkers, and prevention. Acta Neurol Scand. (2019) 139:220–30. doi: 10.1111/ane.13049
99. Nimmagadda U, Salem MR, Crystal GJ. Preoxygenation: physiologic basis, benefits, and potential risks. Anesth Analg. (2017) 124:507–17. doi: 10.1213/ANE.0000000000001589
100. Rheims S, Alvarez BM, Alexandre V, Curot J, Maillard L, Bartolomei F, et al. Hypoxemia following generalized convulsive seizures: risk factors and effect of oxygen therapy. Neurology. (2019) 92:e183–e193. doi: 10.1212/WNL.0000000000006777
101. So EL, Sam MC, Lagerlund TL. Postictal central apnea as a cause of SUDEP: evidence from near-SUDEP incident. Epilepsia. (2000) 41:1494–7. doi: 10.1111/j.1528-1157.2000.tb00128.x
102. Vilella L, Lacuey N, Hampson JP, Rani MRS, Sainju RK, Friedman D, et al. Postconvulsive central apnea as a biomarker for sudden unexpected death in epilepsy (SUDEP). Neurology. (2019) 92:e171–82. doi: 10.1212/WNL.0000000000008588
103. Hebel JM, Surges R, Stodieck SRG, Lanz M. SUDEP following the second seizure in new-onset epilepsy due to limbic encephalitis. Seizure. (2018) 62:124–6. doi: 10.1016/j.seizure.2018.10.007
Keywords: apnea, laryngospasm, SUDEP, airway obstruction, respiratory arrest
Citation: Stewart M, Silverman JB, Sundaram K and Kollmar R (2020) Causes and Effects Contributing to Sudden Death in Epilepsy and the Rationale for Prevention and Intervention. Front. Neurol. 11:765. doi: 10.3389/fneur.2020.00765
Received: 13 January 2020; Accepted: 22 June 2020;
Published: 31 July 2020.
Edited by:
Udaya Seneviratne, Monash Medical Centre, AustraliaReviewed by:
Lécio Figueira Pinto, University of São Paulo, BrazilSamden Lhatoo, University of Texas Health Science Center at Houston, United States
Copyright © 2020 Stewart, Silverman, Sundaram and Kollmar. This is an open-access article distributed under the terms of the Creative Commons Attribution License (CC BY). The use, distribution or reproduction in other forums is permitted, provided the original author(s) and the copyright owner(s) are credited and that the original publication in this journal is cited, in accordance with accepted academic practice. No use, distribution or reproduction is permitted which does not comply with these terms.
*Correspondence: Mark Stewart, bWFyay5zdGV3YXJ0QGRvd25zdGF0ZS5lZHU=