- 1Department of Biomedical and Dental Sciences and Morphofunctional Imaging, University of Messina, Messina, Italy
- 2National Institutes of Health, National Institute of Nursing Research, Bethesda, MD, United States
- 3Cohen Veterans Biosciences, Cambridge, MA, United States
- 4University of Rochester School of Medicine and Dentistry, Rochester, NY, United States
Sex differences in molecular biomarkers after sports-related concussion (SRC) could steadily advance our understanding of injury heterogeneity and complexity, and help capture phenotypic characteristics, by unveiling sex-dependent pathobiological processes and disease mechanisms. Such knowledge will help improve diagnosis, clinical management, and prognosis. Total-tau (t-tau) has recently emerged as a promising blood marker showing sex-associated differences in neurodegenerative diseases. Nonetheless, to date, little is known about the potential influence of sex on its injury-related concentration and dynamics after SRC. We hypothesized that measurements of circulating levels of t-tau over time would reflect a differential vulnerability signature, providing insights into the sex-related phenotypes and their relationship with clinical outcomes. To test this hypothesis, plasma levels of t-tau were measured using an ultrasensitive immunoassay up to 7 days after injury, in 46 concussed athletes (20 males, 26 females). We used trajectory analysis to generate two distinct temporal profiles of t-tau, which were then compared with gender and return to play (RTP). The majority of subjects (~63%) started with low t-tau concentrations that further declined within the first 48 h; while the remaining (“maximal decliners”) started with concentrations comparable to the baseline levels that also fell over time, but persisting markedly higher compared with the first profile. The maximal decliner group was primarily composed of female subjects (p = 0.007) and was significantly associated with poor outcome (RTP ≥ 10 days after concussion) (p = 0.011). Taken together, our data provide evidence for the existence of sex-related biosignatures following sports-related concussions, possibly indicating a differential effect as a result of distinct brain vulnerability and inherent injury response. Future studies will be required to further elucidate underlying sex-based biological and pathophysiological mechanisms, and determine the value of t-tau signatures for management and therapeutic decision-making in sports-related concussions.
Introduction
Research on sport-related concussion (SRC) has increased dramatically in recent years, owing to its daunting burden—estimates suggest that up to 3.8 million cases occur annually, in the US alone—and potential for severe long-term consequences (1, 2). Contextually, sex-based differences have been examined suggesting distinct sequalae and outcomes in male and female athletes, possibly requiring a tailored approach to clinical management (3). Hence, sensitive and objective tools capable of providing new insights into the unique aspects of sex-dependent biological mechanisms and identifying associated phenotypes are vital to inform future research and therapeutic advances.
Several candidate biomarkers have emerged as potential blood tests to aid concussion management. Aside from objectively assessing SRC presence, such markers have been suggested to predict and monitor recovery, while advancing our understanding of the underlying pathophysiological mechanisms of concussion (4–7). Nonetheless, there has been a preponderant focus on male athletes, and very few studies have explored sex-related variability of brain injury biomarkers and their relationships with the underlying neuropathological and clinical characteristics of SRC (8, 9). This lack of biological data and the ensuing knowledge gap of the molecular drivers and processes associated with sex disparities following sports-concussion is a major obstacle to biomarker clinical translation.
Clinical studies corroborated by epidemiological evidence in neurodegenerative diseases have suggested a role that sex may play in modulating the release of tau, a neuroaxonal injury marker, into biofluids while interacting with disease development and progression (10–12). Moreover, the use of an ultra-sensitive assay—single molecule array (SIMOA) technology—has consistently demonstrated the feasibility and reliability of detecting substantially low concentrations of total-tau (t-tau) in blood, making possible its accurate longitudinal measurement in athletes (5, 13–15). Thus, the current work investigated the potential influence of sex in the expression and release of t-tau (16), following sports-related concussion. More specifically, using SIMOA technology, we evaluated temporal profiles for t-tau in a population of concussed and non-concussed athletes in relation to sex and outcome. We hypothesized that the identification of sex-related biomarker signatures could improve phenotype characterization and help elucidate the biological and pathophysiological basis of SRC.
Methods
Participants Selection and Assessment
This research is part of a prospective study on concussion conducted among collegiate Athletes from the National Collegiate Athletic Association (NCAA) division I and III (5). Here, we report on a group of athletes (male 41 and female 42) from soccer (n = 38), American football (n = 26), basketball (n = 8), hockey (n = 4), and lacrosse (n = 4), selected from a cohort of 632 NCAA participants accrued between 2009 and 2014 (Table 1).
Enrolled subjects underwent blood sampling and cognitive testing prior to the sports season, and were followed prospectively for a diagnosis of SRC. To avoid a missed diagnosis of concussion, an on-field certified athletic trainer was present at every game and defined SRC according to diagnostic guidelines on sports-related concussion (17). The severity of SRC was graded based on the resolution of concussion symptoms (i.e., number of days it takes for a player to return to play [RTP]) into short (<10 days) and long (≥10 days) RTP (14). The return-to-play decision was determined by athletic trainers or team physicians at their respective universities and was based on the NCAA best practices (http://www.ncaa.org/sport-science-institute/concussion-diagnosis-and-management-best-practices). In players who sustained SRC, consecutive blood samples were collected within 6 h of injury (median 1.6 h), and at 2, 3, and 7 days post-injury. For the follow-up time points, blood samples were collected between 9 and 10 AM under non-fasting conditions. Plasma sampling at the same time points as SRC athletes was also performed in non-concussed teammate athletes, who did not significantly differ in sport played, history of SRC, or any other demographic feature and served as controls.
The study was approved by the Institutional Review Board at the University of Rochester and Rochester Institute of Technology (approval protocol numbers: 24457 and 22971). Written informed consent was obtained from all participants before enrollment.
Blood Collection and Biomarker Analysis
Venous blood was collected in a non-fasting state by venipuncture into EDTA tubes and placed on ice until processed. All blood was centrifuged within 60 min from the time of blood draw, at 4°C at 3,000 rpm for 10 min. Then plasma was separated, aliquoted, and stored at−80°C pending analysis.
T-Tau concentrations in plasma samples were measured by an ultrasentive immunoassay using SIMOA technology (Quanterix Corporation, Lexington, MA), a digital form of ELISA (13, 18). The Simoa human t- tau assay is based on a sandwich antibody complex that reacts with an epitope in the midregion of the molecule and recognizes all tau isoforms. Two quality control (QC) samples at low and high concentration of the respective analyte were used for assay quality assurance and to assess the overall precision. The limit of detection for the assay is 0.012 pg/mL. The average intra-assay duplicate coefficient of variation was 8.25% (SD <10%). Results were reported in picograms/milliliter (pg/mL). Samples were analyzed at the same time in duplicates and using the same batch of reagents by trained laboratory technicians who were blind to demographic and clinical information.
Statistical Analysis
Statistical analyses were conducted using Stata Data Analysis and Statistical Software (v.13, College Station, Texas). Baseline characteristics were summarized using standard descriptive statistics, and an exploratory analysis was carried out to determine the distribution of the demographic and clinical variables. Continuous variables are presented as mean (SD) or median (interquartile range [IQR]), and categorical variables are summarized as absolute frequencies and percentages. To identify differences between groups in biomarker concentrations, Mann-Whitney U and Wilcoxon signed-rank tests were applied, as appropriate. The non-parametric Friedman test, followed by post-hoc pairwise multiple comparisons (Dunn's test) was performed to evaluate biomarker changes over time. Group-based trajectory analysis (TRAJ) was used to explore biomarker levels in blood using the Stata program and to identify clusters of individuals following trends over time. The TRAJ procedure determines patterns in longitudinal biomarker data by assuming that the population is composed of distinct subgroups containing their own unique biomarker profiles and can handle data that is missing completely at random (19, 20). The trajectories are identified on a likelihood basis using methods previously described (21–23). A censored normal model was used given the minimal detectable limit for each biomarker and the skewed distribution. The number of distinct trajectories for each biomarker was determined by using a combination of the Bayesian information criterion (BIC), Akaike information criterion (AIC), and clinical judgment. Specifically, while the clinical knowledge guided the decision on the maximum number of plausible groups, BIC and AIC were used as the criteria for model selection, which was also moderated by the rule of parsimony (i.e., selecting the simplest model that best describes the data). The final model captured the essential features of the data in the most comprehensible, parsimonious, and analytically tractable manner. Bivariate analyses were performed to explore the TRAJ group associations with sex and outcome. A contingency table was constructed to determine sensitivity and specificity. All tests were two-sided, and significance was determined at p < 0.05.
Results
Description of Population
Eighty-three (41 male and 42 female) athletes were included in the study. The average age was 18.9 years (SD, 0.97 years; range 18–23 years), and over two-thirds (69.9%) of participants were white. Baseline demographic characteristics did not differ between female and male athletes (Table 1). Female athletes were more likely than their male counterpart to play soccer (30 [71.42%] vs. 8 [19.51%]) and basketball (6 [14.19%] vs. 2 [4.88%]), while the vast majority of male participants were football players (29 [70.73%]). Forty-six subjects (55.4%) suffered a concussion, and twelve (28%) of them had a prior history of concussion. No significant differences were seen between males and females in regard to the occurrence of concussion and previous exposure, though, symptoms in female athletes lasted longer (median 7 vs. 13 days, p = 0.01).
Blood Levels and Longitudinal Changes of T-Tau in Male and Female Athletes
T-tau results in men and women were compared both at baseline and longitudinally. We found no significant difference between men and women with respect to the blood levels of t-tau at baseline (p = 0.4). Among non-concussed athletes, there were no substantial changes in t-tau over time in either men or women, and we found no differences between sexes (Figure 1A, Supplementary Table 1). Conversely, among concussed athletes, we found an altered temporal profile of plasma t-tau following injury in both sexes. In men, plasma t-tau concentration substantially decreased at day 2 (p < 0.01 vs. baseline), with the lowest concentrations being measured in samples collected 3 days post-SRP (2.2-fold decreased compared to baseline, p < 0.001). In women, after an initial, but not significant, increase at the 6 h time point, plasma t-tau concentrations dropped on day 2 and remained stably low throughout the study period. Nonetheless, plasma t-tau after SRC was consistently higher in female than male athletes up to 3 days after injury, while the highest differences were observed at 6 h and 3 days after SRC (10.78 vs. 5.42 pg/ml, p = 0.017, and 6.78 vs. 3.49 pg/ml, p = 0.0006, respectively), and returned to comparable levels only on day 7 (Figure 1B, Supplementary Table 1).
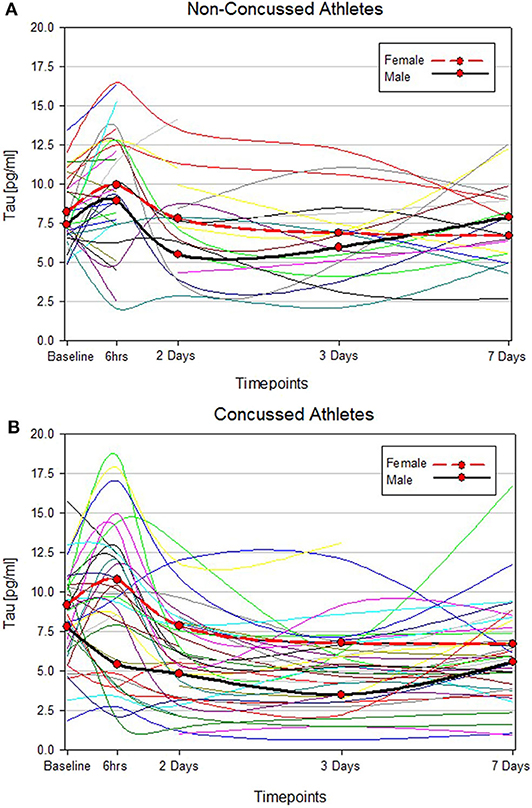
Figure 1. Individual t-tau time course profiles in control and concussed athletes. The spline curves represent the time course of t-tau in non-concussed (A) and concussed (B) study participants. The 2 bold lines represent median values of t-tau in male (black) and female (red).
Trajectory Profiles of Plasma T-Tau in Concussed Players
Two statistically distinct temporal profiles were identified for tau as the best model by trajectory analysis (TRAJ) (Figure 2). T-tau concentrations in both groups decreased over time after concussion. However, while one group (“low transient decliners”), which includes the majority of subjects (~63%), started with low concentrations of t-tau that more substantially declined between day 2 and 3 to slightly rose again on day 7; the other group (“maximal decliners”) started with concentrations comparable to the baseline levels that decreased over time, albeit, remained markedly higher compared with the other group (Figure 2).
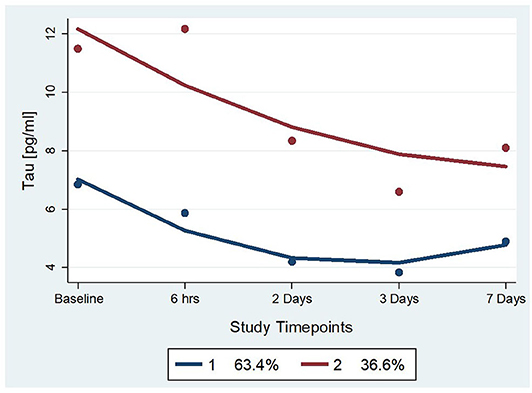
Figure 2. Trajectory groups for profiles over time and percent membership for each trajectory group for serum t-tau. The group-based trajectory analysis (TRAJ) procedure identified 2 groups. The “Low Transient Decliners” group (blue line) included 63% of the subjects. These were subjects with initially low concentrations of plasma t-tau, which further decreased over time. The “Maximal Decliners” group (red line) included the remaining 37% of the subjects, who showed a similar temporal pattern but with consistently higher levels of t-tau.
Tau TRAJ group comparisons were made with concussed athlete features. There were differences by tau TRAJ group membership with regard to gender and RTP, but not concerning the other demographic and clinical variables. The maximal decliner group was associated with female gender and RTP equal to or more than 10 days after concussion (Table 2). The sensitivity and specificity for the prediction of high RTP with the 2-group model were 57 and 78%, respectively (Table 3).
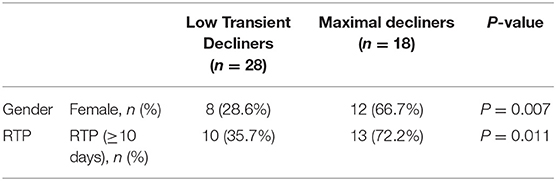
Table 2. Bivariate trajectory group associations with demographic and clinical variables after concussions.
Discussion
In this longitudinal study, we explored the effect of biologic sex on circulating t-tau in healthy collegiate athletes and following sports-related concussions. While there were no between-sex differences in blood t-tau levels at baseline and after normal activity, indicating that no adjusted thresholds are needed to be adopted for clinical use in young adult athletes, we show altered concentrations and dynamics in both sexes after concussion; with a distinct temporal profile and substantially higher levels of t-tau in concussed female athletes compared to their male counterparts. Taken together, these findings suggest a sex-specific biologic response of the brain to SRC.
Why circulating t-tau substantially decreases following sports-concussion is unexplained. In vitro and animal studies have shown that neuronal and synaptic activity dynamically regulate the active secretion of tau into the extracellular space (24). Thus, a potential interpretation could be that normal secretion of tau from neurons into brain interstitial fluid is compromised following sports-concussion, especially in men. Mechanical injury may possibly trigger intraneuronal accumulation and missorting of tau, leading to abnormal phosphorylation and tau truncation (25), which, in turn, results in tau-dependent neuronal malfunction and atrophy and reduced circulating levels (26). On the other hand, several mechanisms could underlie the observed sex difference in t-tau. These include the hormonal asset that may directly affect tau hyperphosphorylation (27). A second possibility is that sex interacts with genetic risk factors (e.g., the apolipoprotein E [APOE] genotype) to drive a distinct downstream response to SRC, including different mechanisms of intra- and trans-cellular mechanisms of tau sorting and exosome biogenesis and spreading (11, 12, 28). Moreover, additional reasons for the disparity observed in t-tau levels could lie in the marked sexual dimorphism in the brain organizational and connectivity patterns, cerebrovascular function, and the post-injury inflammatory responses, which may play a primary or contributing role in characterizing and determining sex-specific SRC pathophysiology and phenotypes (29–31).
Interestingly, no studies to date have identified female athletes with chronic traumatic encephalopathy (CTE) (32). It is, therefore, tempting to speculate that the more marked release of t-tau into the blood of female athletes following sports-concussion could in some extent exert a protective role by preventing brain interstitial accumulation (i.e., neurofibrillary tangles) (33), thereby entailing that the risk for neurodegeneration is modulated in a sex-specific manner. While suggestive, these speculations need to be investigated in future studies specifically targeting the clearance pathways, including the exosome profile, of endogenously produced injury markers after SRC. Nevertheless, our work suggests that sex differences in t-tau may present a powerful key to understanding the biological basis and mechanistic links between traumatic brain injury and pathogenesis of neurodegenerative processes and diseases.
Based on our characterization of the plasma kinetics of t-tau following sports-concussion, substantial differences in t-tau between male and female athletes were measured between 6 and 3 days following injury, but not after (7 days), pointing out a specific time window for investigating sex-based mechanisms underlying SRC. From a pathophysiological perspective, the female early monophasic rise compared to the male delayed monophasic decrease may reflect distinct immediate responses to initial insult as well as secondary injury mechanisms—axonal injury, induced tau hyperphosphorylation—or a combination of both. Future studies are required to explore whether the observed divergence in t-tau levels match distinct anatomical patterns, and if that is the case, their correlation with the extent of the injuries (34, 35).
The trajectory results show that the maximal decliner group is associated with both being female and worse outcomes, which may seem counterintuitive. These findings may perhaps be a function of the presence of APOE ε4 allele, given its interaction with tau pathogenesis and association with outcome after SRC (11, 36, 37). Future work should evaluate the genetic drivers of biomarker profile and cognitive impairment after SRC in a sex-specific manner to identify novel pathways of risk and promote safer sports play. It is also possible that the observed association reflects women's inherent neuroanatomical differences (38), leading to higher susceptibility to traumatic axonal injury and neuronal vulnerability. Such argument fits well with the hypothesis that axonal injury is the main determinant of long-term impairments following SRC, and is in line with a recent study reporting more widespread evidence—a 5-fold difference—of microstructural white matter alterations in female athletes following subconcussive repetitive trauma (35).
In regards to the clinical implications of the trajectory patterns, the maximal decliner group shows fairly high specificity (78%) for the identification of prolonged RTP and may be suitable for the recruitment of subjects into interventional trials. In contrast, the relatively modest sensitivity (57%), which may be partly explained by the fact that t-tau alone is unable to capture the complex pathobiology and ensuing sequelae of SRC, underpins the need for a multimarker strategy.
Our findings, while corroborating and complementing previous studies (39–43), link SRC with a sex biologic divergence of a circulating neuroaxonal injury marker, namely t-tau, demonstrating their association with adverse clinical outcomes-longer RTP. We interpret these results as emphasizing the need for increased awareness of sex-related SRC variability and clinical relevance, and for more extensive research to unearth and elucidate the neurobiological underpinnings of sex-differences in biomedicine, particularly following sports-concussions (44–46).
Limitations of this study include the overall modest sample size. Caution, therefore, is needed in interpreting our results until they can be confirmed in subsequent larger cohorts. Another limitation is that our examination was restricted to young adult athletes (age range 18–22 yrs). Future studies are required to carefully explore the effect of biological sex on circulating t-tau after SRC across the age spectrum. Such information could be particularly valuable in pediatric SRC to interpret the impact of insults during the various phases of brain development (47). Finally, we did not have advanced imaging data. Future work integrating neuroimaging parameters and a panel of novel pathobiologically diverse blood biomarkers (28, 48, 49) is a critical avenue of investigation as it is likely to enhance our understanding of the complex relationships between sex and brain injury following SRC, improve our ability to characterize sex-related phenotype, and deliver targeted and tailored interventions.
The impact of sex, a primary aspect of biological and pathological variability, has been underestimated in sports research and, particularly, related biomarker studies. To move the field forward, it is vital to identify drivers of sexual disparities through the identification of blood biomarkers of specific underlying pathobiological mechanisms. Ultimately, this knowledge will have a significant role and transformative potential in informing clinical trial design and guidelines, and for developing precision-based management and therapies of concussed athletes.
Data Availability Statement
The datasets generated for this study are available on request to the corresponding author.
Ethics Statement
The studies involving human participants were reviewed and approved by The institutional review board at the University of Rochester and Rochester Institute of Technology. The patients/participants provided their written informed consent to participate in this study.
Author Contributions
SM was responsible for performing the statistical analysis and the interpretation of the data and wrote the first draft of the paper. VG and CL carried out the laboratory work, participated in data analysis and data interpretation, and contributed to final review and amendment of the manuscript. AJ participated in data analysis, contributed to data interpretation, and to the final review and amendment of the manuscript. JB was responsible for designing the project, participated in data collection and patient enrollment, contributed to the interpretation of data, and revised the manuscript for content. JG contributed to conceptualizing the study, supervised laboratory work, contributed to data interpretation, and revised the manuscript for intellectual content. All authors read and approved the article for publication.
Funding
This work was supported by funds from the National Institutes of Health, National Institutes of Nursing Research, Intramural Research Program - NIH/NICHD (award no. K24HD064754). The funders had no role in the study design, collection, analysis, and interpretation of data, nor in the writing of the report or in publication decisions.
Conflict of Interest
The authors declare that the research was conducted in the absence of any commercial or financial relationships that could be construed as a potential conflict of interest.
Acknowledgments
We are grateful to all participants for their invaluable contributions in this study.
Supplementary Material
The Supplementary Material for this article can be found online at: https://www.frontiersin.org/articles/10.3389/fneur.2020.00651/full#supplementary-material
References
1. Jordan BD. The clinical spectrum of sport-related traumatic brain injury. Nat Rev Neurol. (2013) 9:222–30. doi: 10.1038/nrneurol.2013.33
2. Theadom A, Mahon S, Hume P, Starkey N, Barker-Collo S, Jones K, et al. Incidence of sports-related traumatic brain injury of all severities: a systematic review. Neuroepidemiology. (2020) 54:192–99. doi: 10.1159/000505424
3. Ono KE, Burns TG, Bearden DJ, McManus SM, King H, Reisner A. Sex-based differences as a predictor of recovery trajectories in young athletes after a sports-related concussion. Am J Sports Med. (2016) 44:748–52. doi: 10.1177/0363546515617746
4. McCrea M, Broglio SP, McAllister TW, Gill J, Giza CC, Huber DL, et al. Association of blood biomarkers with acute sport-related concussion in collegiate athletes: findings from the ncaa and Department of Defzense Care consortium. JAMA Netw Open. (2020) 3:e1919771. doi: 10.1001/jamanetworkopen.2019.19771
5. Gill J, Merchant-Borna K, Jeromin A, Livingston W, Bazarian J. Acute plasma tau relates to prolonged return to play after concussion. Neurology. (2017) 88:595–602. doi: 10.1212/WNL.0000000000003587
6. Bazarian JJ, Korley F, Mannix R. Biomarkers may provide unique insights into neurological effects associated with sport-related concussions. JAMA Netw Open. (2020) 3:e1919799. doi: 10.1001/jamanetworkopen.2019.19799
7. Asken BM, Bauer RM, DeKosky ST, Svingos AM, Hromas G, Boone JK, et al. Concussion BASICS III: serum biomarker changes following sport-related concussion. Neurology. (2018) 91:e2133–43. doi: 10.1212/WNL.0000000000006617
8. Asken BM, Bauer RM, DeKosky ST, Houck ZM, Moreno CC, Jaffee MS, et al. Concussion biomarkers assessed in collegiate student-athletes (BASICS) I: normative study. Neurology. (2018) 91:e2109–22. doi: 10.1212/WNL.0000000000006613
9. Di Battista AP, Rhind SG, Richards D, Churchill N, Baker AJ, Hutchison MG. Altered blood biomarker profiles in athletes with a history of repetitive head impacts. PLoS ONE. (2016) 11:e0159929. doi: 10.1371/journal.pone.0159929
10. GBD. Dementia Collaborators. Global, regional, and national burden of Alzheimer's disease and other dementias, 1990-2016: a systematic analysis for the global burden of disease study 2016. Lancet Neurol. (2019) 18:88–106. doi: 10.1016/S1474-4422(18)30403-4
11. Hohman TJ, Dumitrescu L, Barnes LL, Thambisetty M, Beecham G, Kunkle B. Alzheimer's disease genetics, and I. the Alzheimer's disease neuroimaging, sex-specific association of apolipoprotein E with cerebrospinal fluid levels of tau. JAMA Neurol. (2018) 75:989–98. doi: 10.1001/jamaneurol.2018.0821
12. Altmann A, Tian L, Henderson VW, Greicius MD for the ADNI Investigators. Alzheimer's disease neuroimaging initiative, sex modifies the APOE-related risk of developing alzheimer disease. Ann Neurol. (2014) 75:563–73. doi: 10.1002/ana.24135
13. Gill J, Latour L, Diaz-Arrastia R, Motamedi V, Turtzo C, Shahim P, et al. Glial fibrillary acidic protein elevations relate to neuroimaging abnormalities after mild TBI. Neurology. (2018) 91:e1385–9. doi: 10.1212/WNL.0000000000006321
14. Shahim P, Tegner Y, Marklund N, Blennow K, Zetterberg H. Neurofilament light and tau as blood biomarkers for sports-related concussion. Neurology. (2018) 90:e1780–88. doi: 10.1212/WNL.0000000000005518
15. Rosen A, Oscarsson N, Kvarnstrom A, Gennser M, Sandstrom G, Blennow K, et al. Serum tau concentration after diving - an observational pilot study. Diving Hyperb Med. (2019) 49:88–95. doi: 10.28920/dhm49.2.88-95
16. Zetterberg H, Smith DH, Blennow K. Biomarkers of mild traumatic brain injury in cerebrospinal fluid and blood. Nat Rev Neurol. (2013) 9:201–10. doi: 10.1038/nrneurol.2013.9
17. McCrory P, Meeuwisse W, Johnston K, Dvorak J, Aubry M, Molloy M, et al. Consensus statement on concussion in sport 3rd) international conference on concussion in sport held in zurich, November 2008. Clin J Sport Med. (2009) 19:185–200. doi: 10.4085/1062-6050-44.4.434
18. Rissin DM, Fournier DR, Piech T, Kan CW, Campbell TG, Song L, et al. Simultaneous detection of single molecules and singulated ensembles of molecules enables immunoassays with broad dynamic range. Anal Chem. (2011) 83:2279–85. doi: 10.1021/ac103161b
19. Jones B, Nagin D, Roeder K. A SAS procedure based on mixture models for estimating developmental trajectories. Sociol Methods Res. (2001) 29:19. doi: 10.1177/0049124101029003005
20. Nagin DS, Odgers CL. Group-based trajectory modeling in clinical research. Annu Rev Clin Psychol. (2010) 6:109–38. doi: 10.1146/annurev.clinpsy.121208.131413
21. Mondello S, Buki A, Italiano D, Jeromin A. α-Synuclein in CSF of patients with severe traumatic brain injury. Neurology. (2013) 80:1662–8. doi: 10.1212/WNL.0b013e3182904d43
22. Wagner AK, McCullough EH, Niyonkuru C, Ozawa H, Loucks TL, Dobos JA, et al. Acute serum hormone levels: characterization and prognosis after severe traumatic brain injury. J Neurotrauma. (2011) 28:871–88. doi: 10.1089/neu.2010.1586
23. Mondello S, Guedes VA, Lai C, Czeiter E, Amrein K, Kobeissy F, et al. Circulating brain injury exosomal proteins following moderate-to-severe traumatic brain injury: temporal profile, outcome prediction and therapy implications. Cells. (2020) 9:977. doi: 10.3390/cells9040977
24. Pooler AM, Phillips EC, Lau DH, Noble W, Hanger DP. Physiological release of endogenous tau is stimulated by neuronal activity. EMBO Rep. (2013) 14:389–94. doi: 10.1038/embor.2013.15
25. Zetterberg H. Tauomics and kinetics in human neurons and biological fluids. Neuron. (2018) 97:1202–5. doi: 10.1016/j.neuron.2018.02.030
26. McKee AC, Stein TD, Kiernan PT, Alvarez VE. The neuropathology of chronic traumatic encephalopathy. Brain Pathol. (2015) 25:350–64. doi: 10.1111/bpa.12248
27. Alvarez-de-la-Rosa M, Silva I, Nilsen J, Perez MM, Garcia-Segura LM, Avila J, et al. Estradiol prevents neural tau hyperphosphorylation characteristic of alzheimer's disease. Ann N Y Acad Sci. (2005) 1052:210–24. doi: 10.1196/annals.1347.016
28. Mondello S, Thelin EP, Shaw G, Salzet M, Visalli C, Cizkova D, et al. Extracellular vesicles: pathogenetic, diagnostic and therapeutic value in traumatic brain injury. Expert Rev Proteomics. (2018) 15:451–61. doi: 10.1080/14789450.2018.1464914
29. Gong G, He Y, Evans AC. Brain connectivity: gender makes a difference. Neuroscientist. (2011) 17:575–91. doi: 10.1177/1073858410386492
30. Rahimian R, Cordeau P Jr, Kriz J. Brain response to injuries: when microglia go sexist. Neuroscience. (2019) 405:14–23. doi: 10.1016/j.neuroscience.2018.02.048
31. Hamer J, Churchill NW, Hutchison MG, Graham SJ, Schweizer TA. Sex differences in cerebral blood flow associated with a history of concussion. J Neurotrauma. (2019) 37:1197–1203. doi: 10.1089/neu.2019.6800
32. Esopenko C, Simonds AH, Anderson EZ. The synergistic effect of concussions and aging in women? Disparities and perspectives on moving forward. Concussion. (2018) 3:CNC55. doi: 10.2217/cnc-2018-0004
33. Iverson GL, Gardner AJ, Shultz SR, Solomon GS, McCrory P, Zafonte R, et al. Chronic traumatic encephalopathy neuropathology might not be inexorably progressive or unique to repetitive neurotrauma. Brain. (2019) 142:3672–93. doi: 10.1093/brain/awz286
34. Tran HT, Sanchez L, Esparza TJ, Brody DL. Distinct temporal and anatomical distributions of amyloid-beta and tau abnormalities following controlled cortical impact in transgenic mice. PLoS ONE. (2011) 6:e25475. doi: 10.1371/journal.pone.0025475
35. Rubin TG, Catenaccio E, Fleysher R, Hunter LE, Lubin N, Stewart WF, et al. MRI-defined white matter microstructural alteration associated with soccer heading is more extensive in women than men. Radiology. (2018) 289:478–86. doi: 10.1148/radiol.2018180217
36. Hunter LE, Freudenberg-Hua Y, Davies P, Kim M, Lipton RB, Stewart WF, et al. Associations of apolipoprotein e epsilon4 genotype and ball heading with verbal memory in amateur soccer players. JAMA Neurol. (2020) 77:419–26. doi: 10.1001/jamaneurol.2019.4828
37. Kutner KC, Erlanger DM, Tsai J, Jordan B, Relkin NR. Lower cognitive performance of older football players possessing apolipoprotein E epsilon4. Neurosurgery. (2000) 47:651–7. doi: 10.1227/00006123-200009000-00026
38. Schmithorst VJ, Holland SK, Dardzinski BJ. Developmental differences in white matter architecture between boys and girls. Hum Brain Mapp. (2008) 29:696–710. doi: 10.1002/hbm.20431
39. Covassin T, Elbin R, Kontos A, Larson E. Investigating baseline neurocognitive performance between male and female athletes with a history of multiple concussion. J Neurol Neurosurg Psychiatry. (2010) 81:597–601. doi: 10.1136/jnnp.2009.193797
40. Covassin T, Schatz P, Swanik CB. Sex differences in neuropsychological function and post-concussion symptoms of concussed collegiate athletes. Neurosurgery. (2007) 61:345–50. doi: 10.1227/01.NEU.0000279972.95060.CB
41. Sandel NK, Schatz P, Goldberg KB, Lazar M. Sex-based differences in cognitive deficits and symptom reporting among acutely concussed adolescent lacrosse and soccer players. Am J Sports Med. (2017) 45:937–44. doi: 10.1177/0363546516677246
42. Zuckerman SL, Apple RP, Odom MJ, Lee YM, Solomon GS, Sills AK. Effect of sex on symptoms and return to baseline in sport-related concussion. J Neurosurg Pediatr. (2014) 13:72–81. doi: 10.3171/2013.9.PEDS13257
43. Gallagher V, Kramer N, Abbott K, Alexander J, Breiter H, Herrold A, et al. The effects of sex differences and hormonal contraception on outcomes after collegiate sports-related concussion. J Neurotrauma. (2018) 35:1242–7. doi: 10.1089/neu.2017.5453
44. Clayton JA. Sex influences in neurological disorders: case studies and perspectives. Dialogues Clin Neurosci. (2016) 18:357–60.
45. Brooks CE, Clayton JA. Sex/gender influences on the nervous system: basic steps toward clinical progress. J Neurosci Res. (2017) 95:14–16. doi: 10.1002/jnr.23902
46. Clayton JA. Studying both sexes: a guiding principle for biomedicine. FASEB J. (2016) 30:519–24. doi: 10.1096/fj.15-279554
47. Arambula SE, Reinl EL, El Demerdash N, McCarthy MM, Robertson CL. Sex differences in pediatric traumatic brain injury. Exp Neurol. (2019) 317:168–79. doi: 10.1016/j.expneurol.2019.02.016
48. Mondello S, Hayes RL. Biomarkers. Handb Clin Neurol. (2015) 127:245–65. doi: 10.1016/B978-0-444-52892-6.00016-7
Keywords: sports-related concussion (SRC), t-tau, sex differences, biomarker, brain injury- traumatic, outcome, return to play
Citation: Mondello S, Guedes VA, Lai C, Jeromin A, Bazarian JJ and Gill JM (2020) Sex Differences in Circulating T-Tau Trajectories After Sports-Concussion and Correlation With Outcome. Front. Neurol. 11:651. doi: 10.3389/fneur.2020.00651
Received: 12 March 2020; Accepted: 02 June 2020;
Published: 07 July 2020.
Edited by:
T. John Wu, Uniformed Services University of the Health Sciences, United StatesReviewed by:
Janna Harris, University of Kansas Medical Center, United StatesAndrew Ethan Lincoln, MedStar Health, United States
Copyright © 2020 Mondello, Guedes, Lai, Jeromin, Bazarian and Gill. This is an open-access article distributed under the terms of the Creative Commons Attribution License (CC BY). The use, distribution or reproduction in other forums is permitted, provided the original author(s) and the copyright owner(s) are credited and that the original publication in this journal is cited, in accordance with accepted academic practice. No use, distribution or reproduction is permitted which does not comply with these terms.
*Correspondence: Stefania Mondello, stm_mondello@hotmail.com; smondello@unime.it