- 1Neurology Unit, OCSAE Hospital, AOU Modena, Modena, Italy
- 2Department of Clinical and Experimental Epilepsy, UCL Queen Square Institute of Neurology, London, United Kingdom
- 3Chalfont Centre for Epilepsy, Chalfont, United Kingdom
- 4Department of Biomedical, Metabolic and Neural Science, University of Modena and Reggio Emilia, Modena, Italy
Valproate is a drug widely used to treat epilepsy, bipolar disorder, and occasionally to prevent migraine headache. Despite its clinical efficacy, prenatal exposure to valproate is associated with neurodevelopmental impairments and its use in children and adults was associated with rare cases of reversible brain atrophy and ventricular enlargement. To determine whether valproate use is related with structural brain changes we examined through a cross-sectional study cortical and subcortical structures in a group of 152 people with epilepsy and a normal clinical brain MRI. Patients were grouped into those currently using valproate (n = 54), those taking drugs other than valproate (n = 47), and drug-naïve patients (n = 51) at the time of MRI, irrespectively of their epilepsy syndrome. Cortical thickness and subcortical volumes were analyzed using Freesurfer, version 5.0. Subjects exposed to valproate (either in mono- or polytherapy) showed reduced cortical thickness in the occipital lobe, more precisely in the cuneus bilaterally, in the left lingual gyrus, and in left and right pericalcarine gyri when compared to patients who used other antiepileptic drugs, to drug-naïve epilepsy patients, and to healthy controls. Considering the subgroup of patients using valproate monotherapy (n = 25), both comparisons with healthy controls and drug-naïve groups confirmed occipital lobe cortical thickness reduction. Moreover, patients using valproate showed increased left and right lateral ventricle volume compared to all other groups. Notably, subjects who were non-valproate users at the time of MRI, but who had valproate exposure in the past (n = 27) did not show these cortical or subcortical brain changes. Cortical changes in the posterior cortex, particularly in the visual cortex, and ventricular enlargement, are present in people with epilepsy using valproate, independently from clinical and demographical variables. These findings are relevant both for the efficacy and adverse events profile of valproate use in people with epilepsy.
Introduction
Valproate (VPA) is a drug widely used to treat epilepsy, bipolar disorder, and occasionally to prevent migraine headache. VPA is a first-line antiepileptic drug (AED) in the treatment of Genetic Generalized Epilepsies (GGE) with established efficacy for absence, tonic-clonic and myoclonic seizures (1–3). VPA is also particularly effective in reducing the photoparoxysmal response in various epilepsy syndromes (4–6), but the mechanism whereby photosensitivity is abolished is still unknown. Possible effects of VPA on occipital cortical hyperexcitability have been demonstrated in migraine (7–9), though its mechanisms of action are still debated. Despite its clinical efficacy, its use in females must be carefully evaluated since recent evidence shows that prenatal exposure to valproate is associated with neurodevelopmental impairments, impaired language and intellectual abilities and also with structural brain cortical changes, suggesting an effect of this drug on the prenatal brain (10, 11). In children and adults, valproate use was associated with rare cases of reversible brain atrophy and ventricular enlargement with accompanying cognitive impairment or parkinsonism (12–14). In Alzheimer's Disease, valproate use was also related to accelerated brain volume loss, in particular with ventricular enlargement and hippocampal atrophy, and greater cognitive impairment (15, 16). Recently, Pardoe and colleagues evaluated brain structural measures in seven patients with epilepsy taking valproate compared to normal controls and to people with epilepsy not taking valproate (all males): they found that total brain volume, white matter volume, and parietal cortical thickness were reduced in the valproate group relative to controls and non-valproate users; in addition, when comparing past-valproate users with patients who had never taken valproate, no differences in brain structures emerged, suggesting that valproate-related alterations were transient and reversible (17). The largest published neuroimaging study of epilepsy identified patterns of shared gray matter reduction across epilepsy syndromes, informing our understanding of epilepsy as a network disorder (18). However, this study had not investigated the possible effects of antiepileptic drugs on brain structures.
In this study, we analyzed structural MRI brain scans from a large cohort of people with epilepsy in order to determine whether VPA use is associated with cortical and subcortical brain changes.
Methods
Participants and Study Population
We retrospectively reviewed the entire cohort of people with epilepsy who underwent an MRI study for various research projects between May 2007 and April 2017 at the Department of Neuroscience (total of 354 patients). We included only subjects with normal structural brain MRI on conventional diagnostic protocol at 3 Tesla and absence of intellectual disability (full-scale IQ > 80) and psychiatric comorbidities. We excluded participants with a progressive disease (e.g., Rasmussen's encephalitis, progressive myoclonus epilepsy), malformations of cortical development, hippocampal sclerosis, tumors or previous neurosurgery, as well as patients with developmental and epileptic encephalopathies. We therefore focused on a final pool of 152 people with epilepsy. Sixty-five patients had a diagnosis of Genetic Generalized Epilepsy (GGE) and 87 patients had a diagnosis of focal epilepsy, according to the definitions of the Commission on Classification and Terminology of the International League Against Epilepsy (19, 20). Patients were categorized with a focal epilepsy according to interictal or ictal EEG abnormalities and concordant clinical seizure semiology. Participants were included in the GGE group if they presented with tonic-clonic, absence, or myoclonic seizures with generalized spike-wave discharges on EEG. All patients included in the study had a video-EEG recording/monitoring confirming the clinical diagnosis.
Patients were grouped into those currently taking valproate (VPA+), those who were not valproate users (VPA-), and drug-naïve patients at the time of MRI, irrespectively of their epilepsy syndrome.
Figure 1 outlines the study flow-chart and the final study population.
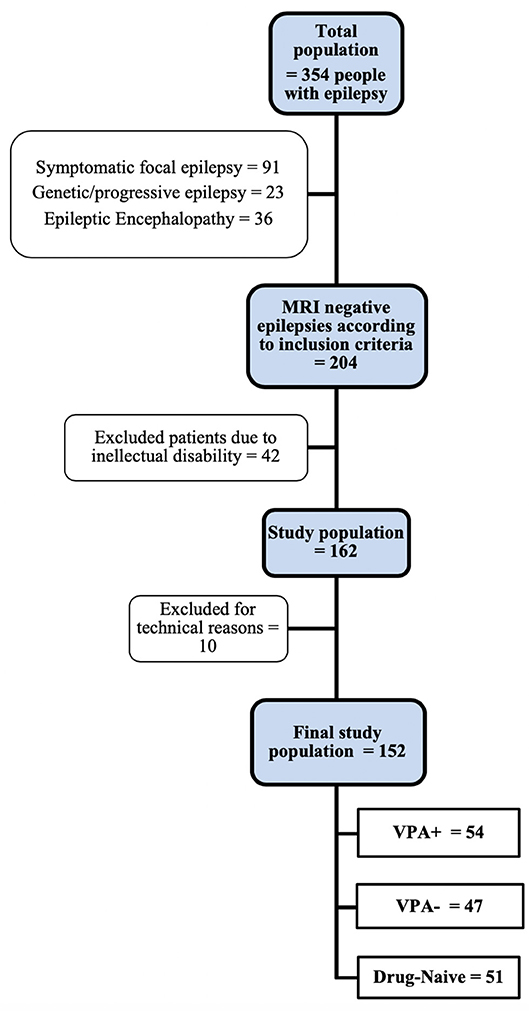
Figure 1. Study flow-chart. See text for inclusion/exclusion criteria. VPA+, patients using valproate at the time of MRI study. VPA-, patients using antiepileptic drugs other than valproate.
For secondary analyses and in order to evaluate the effect of VPA use in mono- or polytherapy regimens, VPA+ patients were classified into subjects using VPA monotherapy and those taking VPA in polytherapy. No subject used drugs other than AEDs at the time of MRI.
To evaluate possible correlations between VPA exposure and brain morphometry measures, we recorded for each patient the VPA total daily dose, the plasma concentration of VPA, and the length of VPA exposure (months). Demographic data and clinical information such as duration of epilepsy, age of epilepsy onset, past antiepileptic drug prescriptions, type and number of AEDs at MRI, and treatment response were also collected for each patient.
For group comparisons, 40 volunteers were recruited as healthy controls (HC). HC had no history of neurological diseases or past valproate use, or family history of epilepsy, and had normal structural neuroimaging. Moreover, all controls had a normal EEG since they were recruited for previous EEG-fMRI co-registration study protocols by our group.
MRI Acquisition
All subjects were studied with the same MRI scanner, sequences, and protocol across the study period. Three-dimensional (3D) T1-weighted MRI images were acquired using a 3 Tesla Philips Intera MRI scanner (Best, The Netherlands). A SPGR pulse sequence [echo time (TE) = 4.6, repetition time (TR) = 9.9 ms] was used. One hundred seventy contiguous sagittal slices were acquired (voxel size = 1 × 1 × 1 mm) and field of view was 240 mm with a matrix size of 256 × 256 × 170. A T2-weighted axial scan was also acquired to allow visual determination of vascular burden or tissue abnormalities.
Statistical Analysis of Patients' Variables
Demographic and clinical characteristics of the subjects were analyzed using SPSS software 26.0 (SPSS Statistics, IBM, Chicago, IL). Independent samples t-tests were used to compare continuous variables and chi-square was used to compare categorical variables between groups.
MRI Cortical Thickness and Subcortical Volume Analyses
Scans were analyzed using a standardized image toolbox (Freesurfer, version 5.0) (21), quality assurance (outlier detection based on inter quartile of 1.5 standard deviations along with visual inspection of segmentations), and statistical methods. Visual inspections of subcortical and cortical segmentations were conducted following standardized ENIGMA protocols (http://enigma.usc.edu), used in prior genetic studies of brain structure (22, 23), and large-scale case-control studies of epilepsy (18) and neuropsychiatric illnesses (24, 25). Analysts (MT; AEV) were blind to participant diagnoses. Briefly, as previously detailed the pipeline involves removal of non-brain tissue, automated Talaraich transformation, segmentation of white matter, and gray matter, tessellation of gray/white matter boundary, automated correction of topology defects, surface deformation to form the gray/white matter boundary and gray/cerebrospinal fluid boundary, and parcellation of cerebral cortex (26). Cortical thickness estimates were then calculated as the distance between the gray/white matter border and the pial surface at each vertex. Cortical thickness values were averaged over frontal, temporal, parietal, occipital, and insular lobes. In addition, single value labels extracted based on an automatic algorithm (27, 28) were calculated for more precise analyses (34 regions for each hemisphere). Subcortical volumes were calculated with FreeSurfer's automated procedure for volumetric measures. Each voxel in the normalized brain volume was assigned to one label using a probabilistic atlas obtained from a manually labeled training set (29). The labels adopted for the analysis included the putamen, caudate nucleus, globus pallidus, nucleus accumbens, thalamus, amygdala, hippocampus, and the ventricular system.
Statistical analyses were performed using SPSS software 26.0 (IBM, Chicago, IL). To compare cortical measures between groups, we conducted a univariate ANCOVA with each neuroanatomical value as the dependent variable, group diagnosis as fixed factor, and age, gender, intracranial volume (ICV), and disease duration (if applicable) as covariates. False discovery rate (FDR) was used to correct for multiple comparisons and a threshold of p < 0.05, estimated using SPSS, according to Benjamini and Hochberg methods (30), was considered statically significant.
Results
One hundred fifty-two patients were finally evaluated for cortical and subcortical measures. Mean age of the patients at MRI considering the whole epilepsy group was 24 ± 5.2 years; mean disease duration was 8.2 years (± 3.4; range 0.5–10); 77 were females.
Table 1 reports the different study groups according to VPA use. Patients using VPA at the time of MRI (VPA+) were 54 (mean VPA dose = 900 mg/day). The median exposure time was 40 months (range: 1–250 months). The mean plasma concentration of VPA was 57.8 ug/ml (median 63 ug/ml; range 30–98 ug/ml). The VPA+ group consisted of 25 patients on monotherapy and 29 patients using VPA in polytherapy regimens (median number of AEDs = 2). There were 47 VPA- patients taking different AEDs in mono or polytherapy regimens (median number of AEDs = 2). Fifty-one patients were drug-naïve at the time of the MRI study. The number of AEDs and treatment response were comparable between VPA+ and VPA- groups. Antiepileptic drugs used by non-VPA users (n = 47) and by VPA+ patients in polytherapy are reported in Supplementary Table 1 (numbers of patients with specific drugs were too small to allow sub-analysis for other single specific AEDs).
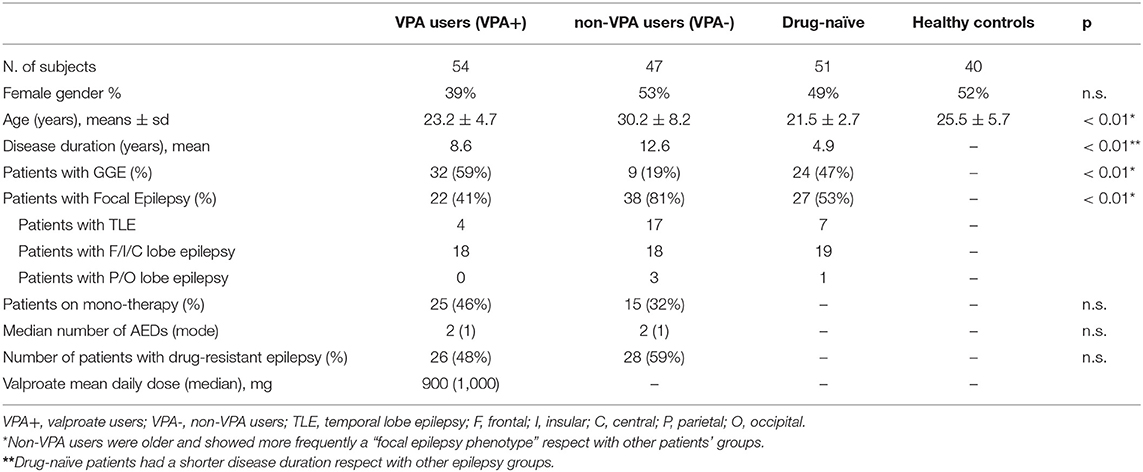
Table 1. Demographic and clinical variables of the different groups according to valproate (VPA) treatment.
Cortical Thickness Analyses According to Valproate Exposure
For all analyses, disease duration (apart from age, gender and ICV) was included as covariate of no interest to rule out a possible effect of this variable on brain structural results.
Direct comparison between VPA- (n =47) and HC groups, and between VPA- and “drug-naïve” patients (n = 51) did not reach any significant differences, either at the lobar level, or for any cortical parcellation.
Table 2 show the main results and the between-groups significant comparisons. The VPA+ group (n = 54) showed reduced cortical thickness compared to the VPA- group within the occipital lobe (F = 6, p = 0.016). More precisely, cortical thickness reduction was found in left (F = 5.6, p = 0.020) and right (F = 5.8, p = 0.019) cuneus, in left (F = 6.7, p = 0.014) and right (F = 5.5, p = 0.020) lingual gyrus, and in right (F = 5.5, p = 0.020) pericalcarine gyrus (Figure 2). We repeated the analysis adding also the “epilepsy type” (focal epilepsy/GGE) as covariate confirming these results (Supplementary Table 2).
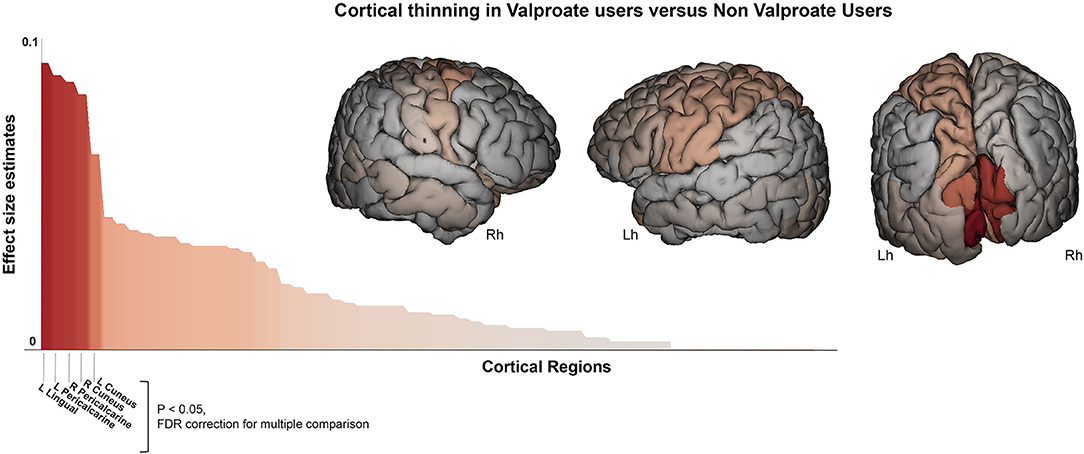
Figure 2. Cortical thickness difference between valproate users and non-valproate users. Surface brain template showing regions of cortical thinning in valproate users compared to non-valproate users. Brain images are generated using EnigmaViewer (https://www.nitrc.org/projects/enigmaviewer_20/); strength (color) of heat map is determined by the size of the regional effect size estimate. Effect size estimates (partial eta squared, y-axis) for cortical thickness differences across all brain regions is showed on the bar plots. Brain regions with significant differences between groups (p < 0.05 FDR; ANCOVA analysis adjusted for age, sex, disease duration, and intracranial volume) are reported on the x-axis. Lh, left hemisphere; Rh, right hemisphere.
A similar pattern of cortical thickness differences emerged when comparing VPA+ vs. drug-naïve epilepsy patients (n = 51): this analysis revealed thickness reduction in occipital lobe (F = 20, p < 0.001). In particular in left (F = 10.7, p = 0.001) and right cuneus (F = 15.9, p < 0.001), in left lingual gyrus (F = 15, p < 0.001), in left (F = 20, p < 0.001) and right (F = 16, p < 0.001) pericalcarine cortex (Figure 3). Notably these two groups were matched for epilepsy type, without significant differences by syndrome (GGE or focal epilepsies). Occipital lobe cortical thickness reduction was also revealed in VPA+ compared to HC (F = 11.5, p = 0.001).
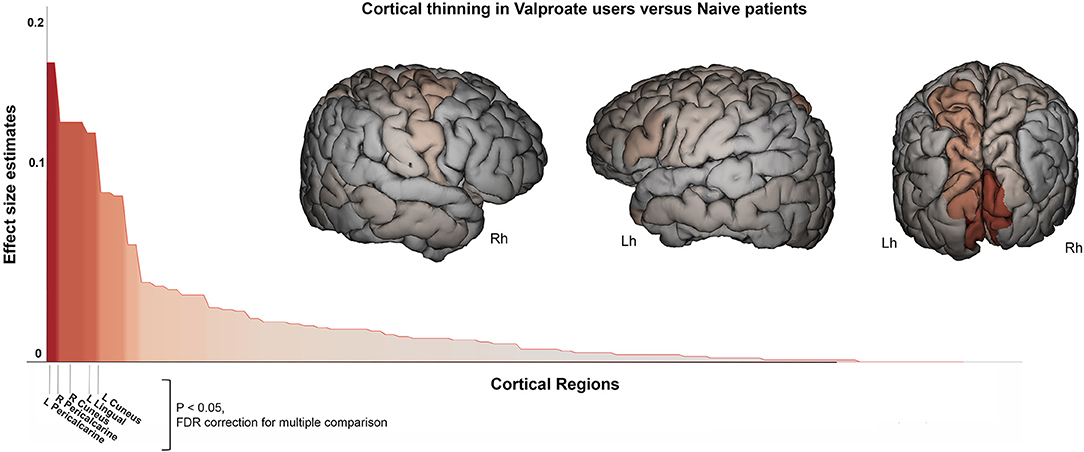
Figure 3. Cortical thickness difference between valproate users and drug-naïve patients. Surface brain template showing regions of cortical thinning in valproate users compared to drug-naïve patients. Brain images are generated using EnigmaViewer (https://www.nitrc.org/projects/enigmaviewer_20/); strength (color) of heat map is determined by the size of the regional effect size estimate. Effect size estimates (partial eta squared, y-axis) for cortical thickness differences across all brain regions is showed on the bar plots. Brain region with significant differences between groups (p < 0.05 FDR; ANCOVA analysis adjusted for age, sex, disease duration, and intracranial volume) are reported on the x-axis. Lh, left hemisphere; Rh, right hemisphere.
Finally, a subgroup analysis considering only patients using VPA monotherapy (n = 25) with respect to all other groups confirmed the occipital lobe cortical thickness reduction (in particular pericalcarine cortex) in VPA users (see Table 2).
Since dose-dependency has been reported in previous studies to increase the risks of congenital anomalies for VPA, and especially at doses above 800 mg/day (31), we also considered these possible dose-effects on cortical and subcortical brain measures. No correlations were found between cortical thickness measures and VPA total daily dose and VPA plasma concentration. Furthermore, no differences emerged when comparing VPA+ patients classified according to VPA total daily dose < or > 800 mg. Finally, no correlations were found between the length of VPA exposure (months) and cortical measures.
Subcortical Structures Analysis According to Valproate Exposure
As for cortical measures, direct comparison between VPA- and healthy controls and between VPA- and drug-naïve patients did not show any significant differences for subcortical structures.
On the other hand, the VPA+ group showed increased left (F = 17, p < 0.001) and right lateral (F = 12.2, p = 0.001) ventricle volume compared to VPA- (Table 3, Figure 4). Left (F = 12, p = 0.001) and right lateral (F = 12, p = 0.001) ventricles were also larger in the VPA+ vs. drug-naïve patients. The direct comparison between VPA+ and HC showed increased left (F = 20.3, p < 0.001) and right (F = 20.9, p < 0.001) lateral ventricle volume and also a reduction in the volumes of the right pallidum (F = 11.4, p = 0.001) and left hippocampus (F = 12.2, p = 0.001). We repeated the analysis adding also epilepsy type as covariate and the results did not change (Supplementary Table 2). The same difference emerged when comparing the VPA monotherapy subgroup with HC and with drug-naïve patients.
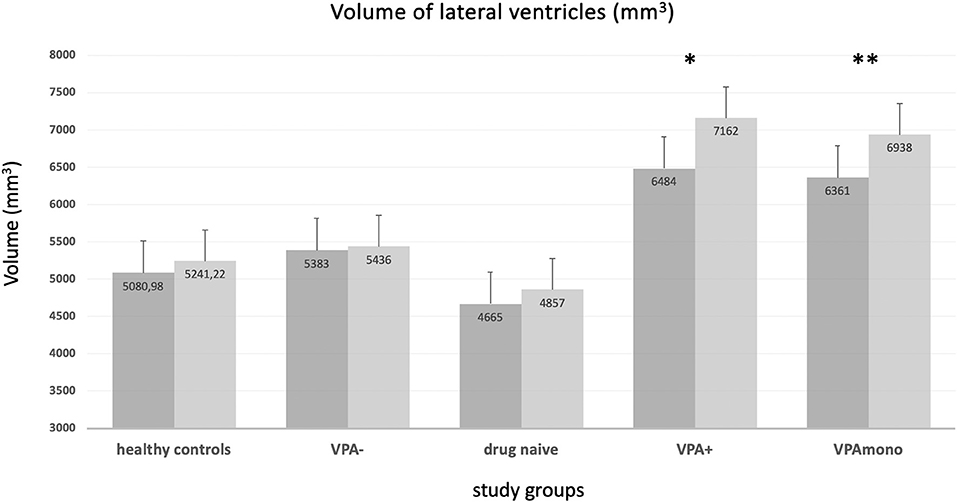
Figure 4. Volume of the lateral ventricles (means, mm3) in the different groups. VPA+ (*) and VPA mono (**) groups showed enlarged ventricular volumes (both right and left ventricle) with respect to every other group. No significant differences were present for healthy controls, VPA-, and drug-naïve groups (see text and Table 3). VPA+: epilepsy patients using valproate (in mono or polytherapy); VPA-, patients not using valproate; VPA mono, patients using valproate in monotherapy. Bars represent the standard error of the mean.
As for cortical thickness analysis, no correlations were found between subcortical measures and VPA total daily dose or VPA plasma concentration. No differences emerged when considering VPA+ patients classified according to VPA total daily dose < or > 800 mg. No correlations were found between duration of VPA exposure (months) and subcortical measures.
Past Exposure to Valproate
To evaluate possible long-term effects of VPA exposure on brain structure, we evaluated patients who were no longer VPA users at the time of MRI but who had been exposed to VPA in the past (n = 27; 11 females; mean age 24.2 ± 3.2; time since VPA withdrawal 6 months to 5 years). Cortical and subcortical measures of patients with past-exposure to VPA were compared to HC and to drug-naïve patients: there were no significant differences for any cortical brain parcellation or for any subcortical structure.
Discussion
The main study finding showed occipital lobe cortical changes, particularly in the visual cortex, and ventricular enlargement in people with epilepsy using VPA compared to those not on VPA. These differences were independent of clinical and demographic variables. Moreover, a direct comparison between patients with past exposure to VPA and controls' groups did not reveal significant differences, suggesting that valproate-related changes might be transient and reversible. Overall, even if the retrospective cross-sectional design of this study cannot establish a causal role of VPA on the observed brain structural changes, the observed findings are presumably related to VPA use and are not driven by epilepsy per se or by generalized or focal epilepsy phenotype. Notably, these results are consistent with previous case reports of “pseudoatrophy” and ventricular enlargement associated with cognitive impairment or parkinsonism in children and adults with epilepsy (12–14) and with clinical trials evaluating the effect of valproate use in Alzheimer's disease patients, showing accelerated brain volume loss and ventricular enlargement in VPA users compared to non-users (15, 16).
Since its original discovery in France in early 60 s as an analog of valeric acid, sodium valproate has become one of the mainstays for the treatment of different epilepsy syndromes in adults and children (1). However, despite its long-standing usage, its multi-faceted mechanism of action is still matter of debate.
Sodium valproate increases gamma-aminobutyric acid (GABA) synthesis and release and hence potentiates GABAergic transmission in several brain regions. It also attenuates neuronal excitation mediated by activation of N-methyl-d-aspartate glutamate receptors. Moreover, a direct action on blocking of voltage-dependent sodium channels was also reported (1).
The occipital, and particularly the visual cortex changes related to VPA exposure are of particular interest considering the established efficacy of this drug in the treatment of GGE, and in particular to reduce the photoparoxysmal response in photosensitive epilepsies (6), as well as its efficacy in reducing cortical excitability in migraine (7). It is of interest that valproate is largely used in treating patients with electroclinical syndromes for whom the visual system has been demonstrated to be the hub of ictogenesis. One is eyelid myoclonia with absence syndrome (Jeavons syndrome) in which the photoparoxysmal response is a cardinal feature of the syndrome itself. Notably, an increased thickness of the visual cortex has been demonstrated in these patients (compared to healthy controls and patients without photosensitivity) as well as an increased functional connectivity between parieto-occipital networks and the motor system (32–34). Another such syndrome is idiopathic occipital lobe epilepsy, a focal idiopathic epilepsy syndrome of childhood, in which the extrastriate visual cortices are involved in spike generation and propagation (35). Moreover, valproate has been demonstrated to modulate (decrease) the excessive functional connectivity between parieto-frontal and motor networks in patients with juvenile myoclonic epilepsy, the commonest GGE syndrome in adolescent and young adults, indicating a possible normalizing effect of this drug (36). Recently, reduced functional connectivity in primary visual and parietal brain networks was noted after administration of VPA in functional neuroimaging studies in the baboon model of genetic generalized epilepsy, suggesting a presumed therapeutic benefit in terms of seizure generation inhibition (37).
Considering the clinical effects of VPA on migraine and photosensitivity and the present findings of VPA-related thinning of posterior cortical regions, we hypothesize that some components of the mechanism of action of VPA are related to cortical structural changes, whether related to its action on GABA or ion-channels neurotransmission or not. Interestingly, vigabatrin, an irreversible GABA-transaminase inhibitor used as first-line treatment in children with infantile spasms, has been demonstrated to induce reversible subcortical alterations (age- and dose-related) in young infants (38, 39). The most common sites for abnormal MRI signal intensity and/or restricted diffusion were the basal ganglia, followed by the dorsal brainstem, dentate nuclei, and thalami. No study to our knowledge has investigated this effect by means of advanced quantitative MRI methods.
Although growing evidence points to a probable role of VPA in causing structural and functional brain changes, mechanisms underlying these changes are still poorly understood. A possible explanation involves inhibition of neurite outgrowth (40), but also osmotic changes (41), and neurotoxicity (42) have been hypothesized. Another proposed mechanism is through mitochondrial dysfunction, as suggested in patients with mitochondrial DNA mutation and VPA toxicity (43). More recently, converging evidence suggests a possible epigenetic effect of VPA on human central nervous system, mediated by histone deacetylase (HDACs) dysregulation (44). Indeed, systemic administration of valproate, a recognized global HDACs inhibitor, induced myelination dysregulation in animals' brain (45, 46), suggesting that chronic exposure to valproate may profoundly affect this process (47). Moreover, VPA treatment produced marked alterations in the expression of multiple genes, many of which are involved in transcription regulation, cell survival, ion homeostasis, cytoskeletal modifications and signal transduction (48). As suggested by Rosenzweig et al. (47) “VPA via its biochemical, molecular and epigenetic mechanisms may simultaneously act to promote neuronal survival and proliferation, while also negatively affecting differentiation of local oligodendrocyte progenitor cells.” The advent of several new imaging techniques, including DTI or myelin-specific MRI techniques, will enable in vivo visualization of any such effects of VPA. Unfortunately, in our study we did not collect specific MRI sequences to analyse white matter or tractography, but we can speculate that our finding of bilaterally larger lateral ventricle volume in patients taking VPA in comparison to controls' groups might reflect a global white matter change related to VPA role as pan-HDACs inhibitor of (re)myelination efficiency and homeostasis. Importantly, epigenetic effects are related to the duration of exposure to VPA. Therefore, the putative effects of valproate as an HDAC inhibitor could also explain the reversibility of the cortical and subcortical structural changes that we observed in the patients that were no longer VPA users.
Study Limitations
This study has several limitations. The first one as already pointed is the retrospective and cross-sectional design of the study. Therefore, a direct causation of VPA on the observed brain structural changes cannot be established. A prospective within-subject longitudinal study should be performed to address this question. Second, we did not collect neurobehavioral measures. This prevents to correlate cortical changes revealed by MRI and neuropsychological and behavioral features, as suggested by previous case reports (10, 14). Other limitations of this study concern the sample size of the different groups: even though the sample was larger with respect to previous MRI studies investigating AEDs effects on brain structure, it was still limited. However, it should be noted that detailed AED information is typically difficult to obtain in larger and multicentre retrospective datasets. Indeed, the only other study that evaluated with structural imaging the VPA effect in people with epilepsy included only seven/nine subjects and all were male (17). Finally, the duration of the disease was significantly different between groups, being shorter in drug-naïve patients and longer in other groups. To mitigate the influence of disease duration, we treated this variable as a confound in all statistical analyses. Moreover, the VPA users had shorter disease duration with respect to non-VPA users, suggesting that it is highly improbable that the observed VPA-related findings are a consequence of a longer disease duration in patients exposed to VPA.
Conclusion
We observed that occipital lobe cortical changes, particularly in the visual cortex, are present in people with epilepsy using VPA. These differences were independent from clinical and demographic variables, and specific for VPA use. The fact that these changes were not observed in patients with past exposure to valproate may suggest reversibility. We believe these findings are relevant both in relation to efficacy and to the adverse events profile of VPA use in people with epilepsy. Finally, the findings of the present study should also be taken into account as a potential confounding factor in any MRI morphometric study in which subjects taking VPA are included.
Data Availability Statement
The raw data supporting the conclusions of this article will be made available by the authors, without undue reservation.
Ethics Statement
The human ethics committee of the University of Modena and Reggio Emilia approved this study and written informed consent was obtained from all subjects.
Author Contributions
SM and MT: study design, drafting, and revising the manuscript. AV: data collection, data interpretation, and revising the manuscript. SS: data interpretation, drafting, and revising the manuscript. All authors contributed to discussion of the results and read and approved the final version of the manuscript.
Funding
Supported by a grant Dipartimenti di eccellenza 2018-2022, MIUR, Italy, to the Department of Biomedical, Metabolic and Neural Sciences. SS was supported by Epilepsy Society, UK. Part of this work was undertaken at University College London Hospitals, which received a proportion of funding from the NIHR Biomedical Research Centers funding scheme.
Conflict of Interest
SS has received honoraria or institutional grant support from UCB and Eisai. SM received research grant support from the Ministry of Health (MOH); has received personal compensation as scientific advisory board member for UCB and Eisai.
The remaining authors declare that the research was conducted in the absence of any commercial or financial relationships that could be construed as a potential conflict of interest.
Supplementary Material
The Supplementary Material for this article can be found online at: https://www.frontiersin.org/articles/10.3389/fneur.2020.00622/full#supplementary-material
References
1. Perucca E. Pharmacological and therapeutic properties of valproate: a summary after 35 years of clinical experience. CNS Drugs. (2002) 16:695–714. doi: 10.2165/00023210-200216100-00004
2. Nevitt SJ, Sudell M, Weston J, Tudur Smith C, Marson AG. Antiepileptic drug monotherapy for epilepsy: a network meta-analysis of individual participant data cochrane database. Syst Rev. (2017) 12:CD011412. doi: 10.1002/14651858.CD011412.pub3
3. Marson AG, Al-Kharusi AM, Alwaidh M, Appleton R, Baker GA, Chadwick DW, et al. The SANAD study of effectiveness of valproate, lamotrigine, or topiramate for generalised and unclassifiable epilepsy: an unblinded randomised controlled trial. Lancet. (2007) 369:1016–26. doi: 10.1016/S0140-6736(07)60461-9
4. Harding GF, Edson A, Jeavons PM. Persistence of photosensitivity. Epilepsia. (1997) 38:663–9. doi: 10.1111/j.1528-1157.1997.tb01235.x
6. Verrotti A, Grosso S, D'Egidio C, Parisi P, Spalice A, Pavone P, et al. Valproate in adolescents with photosensitive epilepsy with generalized tonic-clonic seizures only. Eur J Paediatr Neurol. (2014) 18:13–8. doi: 10.1016/j.ejpn.2013.06.006
7. Bowyer SM, Mason KM, Moran JE, Tepley N, Mitsias PD. Cortical hyperexcitability in migraine patients before and after sodium valproate treatment. J Clin Neurophysiol. (2005) 22:65–7. doi: 10.1097/01.WNP.0000150928.23523.A9
8. Aurora SK, Cao Y, Bowyer SM, Welch KM. The occipital cortex is hyperexcitable in migraine: experimental evidence. Headache. (1999) 39:469–76. doi: 10.1046/j.1526-4610.1999.3907469.x
9. Zielman R, Wijnen JP, Webb A, Onderwater GLJ, Ronen I, Ferrari MD, et al. Cortical glutamate in migraine. Brain. (2017) 140:1859–71. doi: 10.1093/brain/awx130
10. Meador KJ, Baker GA, Browning N, Clayton-Smith J, Combs-Cantrell DT, Cohen M, et al. Cognitive function at 3 years of age after fetal exposure to antiepileptic drugs. N Engl J Med. (2009) 360:1597–605. doi: 10.1056/NEJMoa0803531
11. Wood AG, Chen J, Barton S, Nadebaum C, Anderson VA, Catroppa C, et al. Altered cortical thickness following prenatal sodium valproate exposure. Ann Clin Transl Neurol. (2014) 1:497–501. doi: 10.1002/acn3.74
12. Lovett M, Skidmore DL, Mohamed IS. Valproate-induced pseudoatrophy: expanding the clinical and imaging spectrum. Pediatr Neurol. (2014) 51:284–5. doi: 10.1016/j.pediatrneurol.2014.04.019
13. McLachlan RS. Pseudoatrophy of the brain with valproic acid monotherapy. Can J Neurol Sci. (1987) 14:294–6. doi: 10.1017/S0317167100026640
14. Papazian O, Canizales E, Alfonso I, Archila R, Duchowny M, Aicardi J. Reversible dementia and apparent brain atrophy during valproate therapy. Ann Neurol. (1995) 38:687–91. doi: 10.1002/ana.410380423
15. Fleisher AS, Truran D, Mai JT, Langbaum JB, Aisen PS, Cummings JL, et al. Chronic divalproex sodium use and brain atrophy in alzheimer disease. Neurology. (2011) 77:1263–71. doi: 10.1212/WNL.0b013e318230a16c
16. Tariot PN, Schneider LS, Cummings J, Thomas RG, Raman R, Jakimovich LJ, et al. Chronic divalproex sodium to attenuate agitation and clinical progression of alzheimer disease. Arch Gen Psychiatr. (2011) 68:853–61. doi: 10.1001/archgenpsychiatry.2011.72
17. Pardoe HR, Berg AT, Jackson GD. Sodium valproate use is associated with reduced parietal lobe thickness and brain volume. Neurology. (2013) 80:1895–900. doi: 10.1212/WNL.0b013e318292a2e5
18. Whelan CD, Altmann A, Botia JA, Jahanshad N, Hibar DP, Absil J, et al. Structural brain abnormalities in the common epilepsies assessed in a worldwide ENIGMA study. Brain. (2018) 141:391–408. doi: 10.1093/brain/awx341
19. Berg AT, Berkovic SF, Brodie MJ, Buchhalter J, Cross JH, van Emde Boas W, et al. Revised terminology and concepts for organization of seizures and epilepsies: report of the ILAE commission on classification and Terminology, 2005-2009. Epilepsia. (2010) 51:676–85. doi: 10.1111/j.1528-1167.2010.02522.x
20. Scheffer IE, Berkovic S, Capovilla G, Connolly MB, French J, Guilhoto L, et al. ILAE classification of the epilepsies: position paper of the ILAE commission for classification and terminology. Epilepsia. (2017) 58:512–21. doi: 10.1111/epi.13709
22. Hibar DP, Westlye LT, Doan NT, Jahanshad N, Cheung JW, Ching CRK, et al. Cortical abnormalities in bipolar disorder: an MRI analysis of 6503 individuals from the ENIGMA Bipolar Disorder Working Group. Mol Psychiatr. (2018) 23:932–42. doi: 10.1038/mp.2017.73
23. Stein JL, Medland SE, Vasquez AA, Hibar DP, Senstad RE, Winkler AM, et al. Identification of common variants associated with human hippocampal and intracranial volumes. Nat Genet. (2012) 44:552–61. doi: 10.1038/ng.2250
24. Boedhoe PS, Schmaal L, Abe Y, Ameis SH, Arnold PD, Batistuzzo MC, et al. Distinct subcortical volume alterations in pediatric and adult OCD: a worldwide meta- and mega-analysis. Am J Psychiatr. (2017) 174:60–9. doi: 10.1176/appi.ajp.2016.16020201
25. Schmaal L, Veltman DJ, van Erp TG, Samann PG, Frodl T, Jahanshad N, et al. Subcortical brain alterations in major depressive disorder: findings from the ENIGMA major depressive disorder working group. Mol Psychiatr. (2016) 21:806–12. doi: 10.1038/mp.2015.69
26. Tondelli M, Vaudano AE, Ruggieri A, Meletti S. Cortical and subcortical brain alterations in juvenile absence epilepsy. Neuroimage Clin. (2016) 12:306–11. doi: 10.1016/j.nicl.2016.07.007
27. Desikan RS, Segonne F, Fischl B, Quinn BT, Dickerson BC, Blacker D, et al. An automated labelling system for subdividing the human cerebral cortex on MRI scans into gyral based regions of interest. Neuroimage. (2006) 31:968–80. doi: 10.1016/j.neuroimage.2006.01.021
28. Fischl B, van der Kouwe A, Destrieux C, Halgren E, Segonne F, Salat DH, et al. Automatically parcellating the human cerebral cortex. Cereb Cortex. (2004) 14:11–22. doi: 10.1093/cercor/bhg087
29. Fischl B, Salat DH, Busa E, Albert M, Dieterich M, Haselgrove C, et al. Whole brain segmentation: automated labeling of neuroanatomical structures in the human brain. Neuron. (2002) 33:341–55. doi: 10.1016/S0896-6273(02)00569-X
30. Bejamini Y, Hochberg Y. Controlling the False Discovery Rate: a practical and powerful approach to multiple testing. J Roy Stat Soc. (1995) 57:289–300. doi: 10.1111/j.2517-6161.1995.tb02031.x
31. Perucca E. Birth defects after prenatal exposure to antiepileptic drugs. Lancet Neurol. (2005) 4:781–6. doi: 10.1016/S1474-4422(05)70224-6
32. Meletti S, Vaudano AE. Comment on “Reflex epileptic mechanisms in humans: lessons about natural ictogenesis” by Peter Wolf. Epilepsy Behav. (2015) 52:275–6. doi: 10.1016/j.yebeh.2015.07.045
33. Vaudano AE, Ruggieri A, Avanzini P, Gessaroli G, Cantalupo G, Coppola A, et al. Photosensitive epilepsy is associated with reduced inhibition of alpha rhythm generating networks. Brain. (2017) 140:981–97. doi: 10.1093/brain/awx009
34. Vaudano AE, Ruggieri A, Tondelli M, Avanzini P, Benuzzi F, Gessaroli G, et al. The visual system in eyelid myoclonia with absences. Ann Neurol. (2014) 76:412–27. doi: 10.1002/ana.24236
35. Meletti S, Ruggieri A, Avanzini P, Caramaschi E, Filippini M, Bergonzini P, et al. Extrastriate visual cortex in idiopathic occipital epilepsies: the contribution of retinotopic areas to spike generation. Epilepsia. (2016) 57:896–906. doi: 10.1111/epi.13385
36. Vollmar C, O'Muircheartaigh J, Barker GJ, Symms MR, Thompson P, Kumari V, et al. Motor system hyperconnectivity in juvenile myoclonic epilepsy: a cognitive functional magnetic resonance imaging study. Brain. (2011) 134:1710–9. doi: 10.1093/brain/awr098
37. Salinas FS, Szabo CA. Resting-state functional connectivity changes due to acute and short-term valproic acid administration in the baboon model of GGE. Neuroimage Clin. (2017) 16:132–41. doi: 10.1016/j.nicl.2017.07.013
38. Dracopoulos A, Widjaja E, Raybaud C, Westall CA, Snead OC. Vigabatrin-associated reversible MRI signal changes in patients with infantile spasms. Epilepsia. (2010) 51:1297–304. doi: 10.1111/j.1528-1167.2010.02564.x
39. Pearl PL, Vezina LG, Saneto RP, McCarter R, Molloy-Wells E, Heffron A, et al. Cerebral MRI abnormalities associated with vigabatrin therapy. Epilepsia. (2009) 50:184–94. doi: 10.1111/j.1528-1167.2008.01728.x
40. Qian Y, Zheng Y, Tiffany-Castiglioni E. Valproate reversibly reduces neurite outgrowth by human SY5Y neuroblastoma cells. Brain Res. (2009) 1302:21–33. doi: 10.1016/j.brainres.2009.09.051
41. Garcia M, Huppertz HJ, Ziyeh S, Buechert M, Schumacher M, Mader I. Valproate-induced metabolic changes in patients with epilepsy: assessment with H-MRS. Epilepsia. (2009) 50:486–92. doi: 10.1111/j.1528-1167.2008.01801.x
42. Petroff OA, Rothman DL, Behar KL, Hyder F, Mattson RH. Effects of valproate and other antiepileptic drugs on brain glutamate, glutamine, and GABA in patients with refractory complex partial seizures. Seizure. (1999) 8:120–7. doi: 10.1053/seiz.1999.0267
43. Komulainen T, Lodge T, Hinttala R, Bolszak M, Pietila M, Koivunen P, et al. Sodium valproate induces mitochondrial respiration dysfunction in HepG2 in vitro cell model. Toxicology. (2015) 331:47–56. doi: 10.1016/j.tox.2015.03.001
44. Shen S, Sandoval J, Swiss VA, Li J, Dupree J, Franklin RJ, et al. Age-dependent epigenetic control of differentiation inhibitors is critical for remyelination efficiency. Nat Neurosci. (2008) 11:1024–34. doi: 10.1038/nn.2172
45. Jacob C, Lebrun-Julien F, Suter U. How histone deacetylases control myelination. Mol Neurobiol. (2011) 44:303–12. doi: 10.1007/s12035-011-8198-9
46. Shen S, Li J, Casaccia-Bonnefil P. Histone modifications affect timing of oligodendrocyte progenitor differentiation in the developing rat brain. J Cell Biol. (2005) 169:577–89. doi: 10.1083/jcb.200412101
47. Rosenzweig I, Vukadinovic Z, Turner AJ, Catani M. Neuroconnectivity and valproic acid: the myelin hypothesis. Neurosci Biobehav Rev. (2012) 36:1848–56. doi: 10.1016/j.neubiorev.2012.05.006
Keywords: valproate, epilepsy, brain morphometry, cortical thickness, brain structure
Citation: Tondelli M, Vaudano AE, Sisodiya SM and Meletti S (2020) Valproate Use Is Associated With Posterior Cortical Thinning and Ventricular Enlargement in Epilepsy Patients. Front. Neurol. 11:622. doi: 10.3389/fneur.2020.00622
Received: 07 April 2020; Accepted: 27 May 2020;
Published: 02 July 2020.
Edited by:
Eliane Kobayashi, McGill University, CanadaReviewed by:
Vincenzo Belcastro, Ospedale Sant Anna, ItalyAntonietta Coppola, University of Naples Federico II, Italy
Copyright © 2020 Tondelli, Vaudano, Sisodiya and Meletti. This is an open-access article distributed under the terms of the Creative Commons Attribution License (CC BY). The use, distribution or reproduction in other forums is permitted, provided the original author(s) and the copyright owner(s) are credited and that the original publication in this journal is cited, in accordance with accepted academic practice. No use, distribution or reproduction is permitted which does not comply with these terms.
*Correspondence: Stefano Meletti, stefano.meletti@unimore.it
†ORCID: Manuela Tondelli orcid.org/0000-0001-6698-5437
Stefano Meletti orcid.org/0000-0003-0334-539X