- 1Department of Neurology, Kyungpook National University Hospital, Daegu, South Korea
- 2Department of Neurology, Asan Medical Center, University of Ulsan College of Medicine, Seoul, South Korea
- 3Department of Neurology & Parkinson's Disease Center, Guro Hospital, Korea University, Seoul, South Korea
- 4Department of Neurology, Dobong Hospital, Seoul, South Korea
- 5Department of Neurology, Bobath Memorial Hospital, Seongnam-si, South Korea
- 6Department of Neurology, Best Heals Hospital, Ansan-si, South Korea
- 7Department of Neurology, Metro Hospital, Anyang, South Korea
- 8Department of Neurology, The Good Light Hospital, Gwangju, South Korea
Background: Parkinson's disease (PD) is a common neurodegenerative disorder, characterized by a clinical symptomatology involving both motor and non-motor symptoms. Motor complications associated with long-term dopaminergic treatment include motor fluctuations and levodopa-induced dyskinesia (LID), which may have a major impact on the quality of life. The clinical features and onset time of motor complications in the disease course are heterogeneous, and the etiology remains unknown.
Objective: We aimed to identify genomic variants associated with the development of motor fluctuations and LID at 5 years after the onset of PD.
Methods: Genomic data were obtained using Affymetrix Axiom KORV1.1 array, including an imputation genome-wide association study (GWAS) grid and other GWAS loci; functional variants of the non-synonymous exome; pharmacogenetic variants; variants in genes involved in absorption, distribution, metabolism, and excretion of drugs; and expression quantitative trait loci in 741 patients with PD.
Results: FAM129B single-nucleotide polymorphism (SNP) rs10760490 was nominally associated with the occurrence of motor fluctuations at 5 years after the onset of PD [odds ratio (OR) = 2.9, 95% confidence interval (CI) = 1.8–4.8, P = 6.5 × 10−6]. GALNT14 SNP rs144125291 was significantly associated with the occurrence of LID (OR = 5.5, 95% CI = 2.9–10.3, P = 7.88 × 10−9) and was still significant after Bonferroni correction. Several other genetic variants were associated with the occurrence of motor fluctuations or LID, but the associations were not significant after Bonferroni correction.
Conclusion: This study identified new loci associated with the occurrence of motor fluctuations and LID at 5 years after the onset of PD. However, further studies are needed to confirm our findings.
Introduction
Parkinson's disease (PD) is a chronic, progressive neurodegenerative disorder characterized by a heterogeneous clinical symptomatology involving both motor and non-motor symptoms (1–3). The pathological hallmarks of PD are abnormal accumulation of alpha-synuclein (α-syn) aggregates, Lewy bodies, and Lewy neurites (4, 5). The α-synucleinopathy in PD involves not only dopaminergic neurons in the substantia nigra pars compacta of the midbrain but also other vulnerable neurotransmitter systems in the central nervous system (6, 7).
Levodopa is the most effective and potent medication for the treatment of motor symptoms of PD (8), and early treatment with levodopa increases life expectancy (9). However, long-term treatment of patients with PD with levodopa can result in the occurrence of motor fluctuations and dyskinesias. These late motor complications can become major causes of disability and reduce the quality of life of patients (10). To date, the pathophysiological mechanisms underlying motor fluctuations and levodopa-induced dyskinesia (LID) in patients with PD remain unclear.
Over the last two decades, rare variants of more than 20 genes have been reported to cause genetic PD (11). The common genetic risk factors for sporadic PD have been identified by genome-wide association studies (GWAS). To date, 90 independent genetic variants have been identified as risk factors for sporadic PD (12). Although previous GWAS and other genetic studies have indicated the importance of genetic contribution to the development of PD, the contribution of genetic factors to specific phenotypes of PD has not been well-studied. Identification of genetic risk factors for the major clinical phenotypes of PD may provide important insights into the underlying molecular mechanisms and valuable information for potential adjustments to overcome genetic heterogeneity in clinical trials. This GWAS aimed to identify the genetic variants associated with the occurrence of motor fluctuations and LID in patients with sporadic PD.
Materials and Methods
Patients
We included 741 patients who were diagnosed with PD (Supplementary Figure 1). Experienced movement disorder specialists (SJC, HSR, MJK, JK, and YJK) made the diagnosis of PD using the clinical diagnostic criteria of the United Kingdom Parkinson's Disease Society Brain Bank (13). All patients were enrolled from the clinical practice of the Department of Neurology of the Asan Medical Center, Seoul, South Korea, between January 1, 2011 and April 30, 2016. All patients were born and resided in South Korea. All patients were unrelated and ethnic Koreans without any foreign ancestry. The Institutional Review Board (IRB) of Asan Medical Center approved the study, and all patients provided an informed consent in accordance with the IRB regulations.
Clinical Assessment
Motor fluctuations were defined as alternating between periods of good motor symptom control (on-time) and periods of reduced motor symptom control (off-time), which were dependent on the scheduled intake time of levodopa and other dopaminergic medications (14). The time between the onset of PD motor symptoms and the occurrence of motor fluctuations was assessed in each patient.
LID was defined as involuntary choreiform or dystonic body movements, which occur most frequently when levodopa concentrations are at its highest (peak-dose dyskinesia) or, less commonly, at the beginning or end of levodopa administration, or both (diphasic dyskinesia) (14). The time between the onset of PD motor symptoms and the occurrence of LID was assessed in each patient. PD onset was defined as the onset of first motor symptoms in patients with PD.
The presence of motor fluctuations or LID was determined using the clinical history and Unified Parkinson's Disease Rating Scale (UPDRS) part IV. Dystonia that occurred in the morning before taking a medication was not considered as LID (15).
Genomic Analysis
Genotype data were obtained using the Korean Chip (K-CHIP), obtained from the K-CHIP consortium. K-CHIP was designed by the Center for Genome Science, Korea National Institute of Health, Korea (4845-301, 3000-3031) (www.cdc.go.kr). K-CHIP uses Affymetrix Axiom Customized Biobank Genotyping Arrays (Affymetrix, Santa Clara, CA, USA) and contains 827,783 variants. K-CHIP consists of an imputation GWAS grid [505,000 Asian-based grid with minor allele frequency (MAF) >5% in Asians]; exome contents [84,000 Korean-based grid with MAF >5%, in Koreans; 149,000 coding single-nucleotide polymorphisms (cSNPs); and insertions and deletions on the basis of data from 2000 whole exome sequences and 400 whole genome sequences with MAF> 0.1%]; new exome/loss of function contents (44,000 variants); expression quantitative trait loci (17,000 variants); absorption, distribution, metabolism, and excretion genes; and other miscellaneous variants.
Sample Quality Controls
The primary sample quality control was as follows: samples with low call rate (<0.95%) were excluded from the analysis because of the possibility of low DNA quality or experimental error; high heterozygosity was excluded from the analysis because of low DNA quality or possible contamination of samples. The entire sample distribution was checked, and low-quality samples were excluded if they deviated significantly from the entire sample distribution. SNP pruning was also performed. Because cryptic first-degree relative and multidimensional scaling (MDS) analyses are very time consuming when using whole data, only representative SNP information based on linkage disequilibrium were selected from the data. Due to the possibility of population stratification, samples that deviated from the whole sample were excluded from the analysis by assessing the MDS. If there were more than a certain number of SNPs with only one sample, the possibility of errors due to DNA quality and technical artifacts was excluded.
Secondary sample quality control consisted of genotype calling, excluding samples deemed to be of low quality based on the primary sample quality control criteria and sex-inconsistent samples. Samples that did not satisfy the quality control criteria after a repeat sample quality control were excluded. SNP data were excluded from the cryptic first-degree relative analysis because statistical analysis assumes the independence for each sample in most cases.
SNP Quality Controls
An SNPolisher analysis was performed to exclude low-quality SNPs. SNPs with low call rates were excluded when the call rate was <95% because errors in the calling process can occur due to probe design and clustering analysis problems. If the Hardy–Weinberg equilibrium (HWE) test P-value of a specific SNP is low, it indicates a probable error in the genotype clustering process; therefore, the HWE P < 10−6. If the frequency of a genetic variation is extremely different from that in Korean and Asian populations, there may be a genotype clustering error. Therefore, we excluded cases where the difference in MAF was >0.2. Both cases and controls were excluded if the MAF was <1%.
Statistical Analysis
The associations of each genetic variant with the occurrence of motor fluctuations and LID were investigated using multiple logistic regression models. We used the Cochran–Armitage trend test and the Jonckheere–Terpstra test, and adjusted all analyses by sex and age at onset of PD. For each genetic variant, we calculated the odds ratio (OR), 95% confidence interval (CI), and two-tailed P-value. For sensitivity analyses, similar analyses were performed for patients aged ≥50 years at onset of PD to further adjust for the effects of age at onset of PD on the occurrence of motor fluctuations and LID. The P-values from the primary analyses were assessed for significance using the Bonferroni correction for multiple comparisons. Clustering quality control was performed by visual inspection of analytic data of SNPs with a P < 0.0001. Markers that did not clearly separate between different genotypes and were not closely located in the same genotype were excluded (Supplementary Figure 2). Manhattan plots and quantile–quantile plots were constructed for P-values for all genotyped variants that passed quality controls.
The statistical analysis was performed using the PLINK program (version 1.90, NIH-NIDDK Laboratory of Biological Modeling, Bethesda, MD, USA), Haploview (version 4.2, Daly Lab at the Broad Institute, Cambridge, MA, USA), LocusZoom (version 1.4, University of Michigan, Department of Biostatistics, Center for Statistical Genetics, Ann Arbor, MI, USA), and R (version 3.1.2, Free Software Foundation, Inc., Boston, MA, USA).
Results
Patients
Clinical and genotyping data were obtained from 741 patients with PD who were followed for at least 5 years after the onset of PD. The demographic and clinical features of study patients are summarized in Table 1. The study group consisted of 325 men (43.9%) and 416 women (56.1%). The mean age at onset of PD was 57.1 years, while the mean disease duration from the onset of PD to the last follow-up was 10.8 ± 4.5 years.
Motor Fluctuations
Five years after the onset of PD, 219 (29.6%) patients exhibited motor fluctuations. No difference was observed between patients with PD with motor fluctuations (92 men, 42.0%) and those without motor fluctuations (233 men, 44.6%) (P = 0.480) in terms of sex. The mean age at onset of PD was lower in patients with motor fluctuations than in those without motor fluctuations (54.0 ± 10.3 years vs. 58.4 ± 9.7 years, P < 0.001). The mean disease duration between the onset of PD and the last follow-up was shorter in patients with motor fluctuations than in those without motor fluctuations (9.5 ± 4.0 years vs. 11.3 ± 4.6 years, P < 0.001; Supplementary Table 1). The 583,535 SNPs that passed quality controls were genotyped and analyzed. Quantile–quantile plots were made for the presence of LID at 5 years after onset of PD (Supplementary Figure 3A), and a Manhattan plot is described in Figure 1A. The top 20 SNPs associated with the occurrence of motor fluctuations are listed in Table 2. FAM129B SNP rs10760490 was nominally associated with the occurrence of motor fluctuations at 5 years after onset of PD (OR = 2.9, 95% CI = 1.8–4.8, P = 6.5 × 10−6). However, FAM129B SNP rs10760490 and other SNPs were not significant after Bonferroni correction (Table 2).
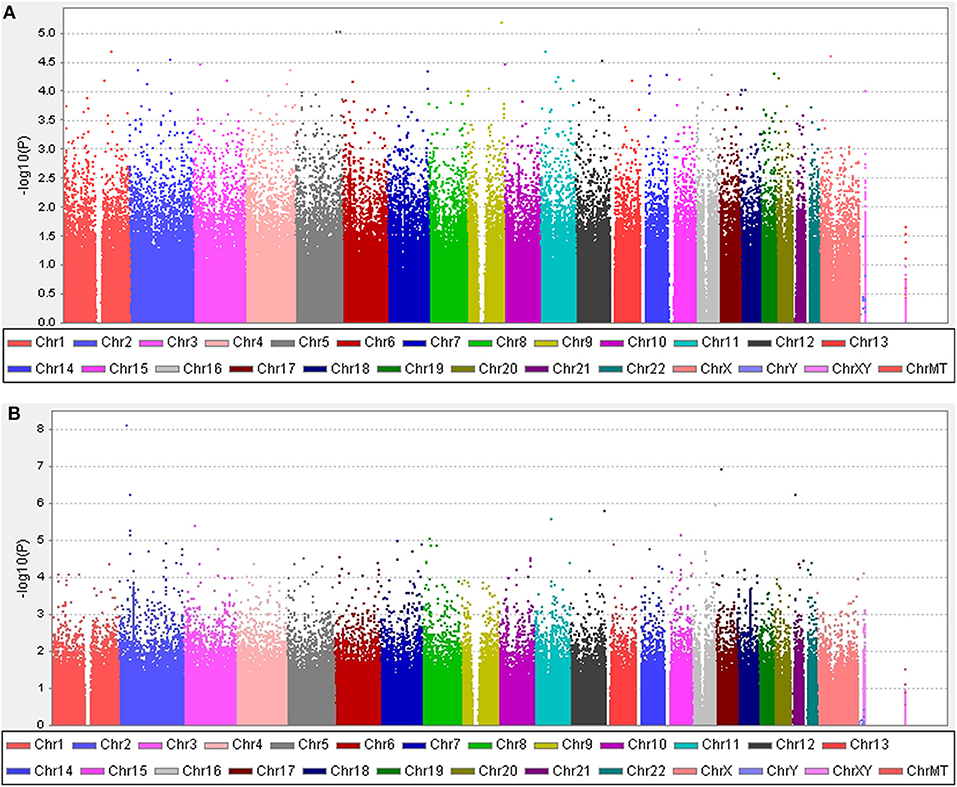
Figure 1. Manhattan plots. (A) The plot shows P-values for association analyses between 583,535 SNPs and the occurrence of motor fluctuations 5 years after the onset of Parkinson's disease (PD). The most significant association observed is with a locus on chromosome 9 (rs10760490). (B) The plot shows P-values for association analyses between 583,535 SNPs and the occurrence of levodopa-induced dyskinesia 5 years after the onset of PD. The most significant association observed is with a locus on chromosome 2 (rs144125291). P-values are log-transformed (y-axis) and plotted against chromosomal position (x-axis).
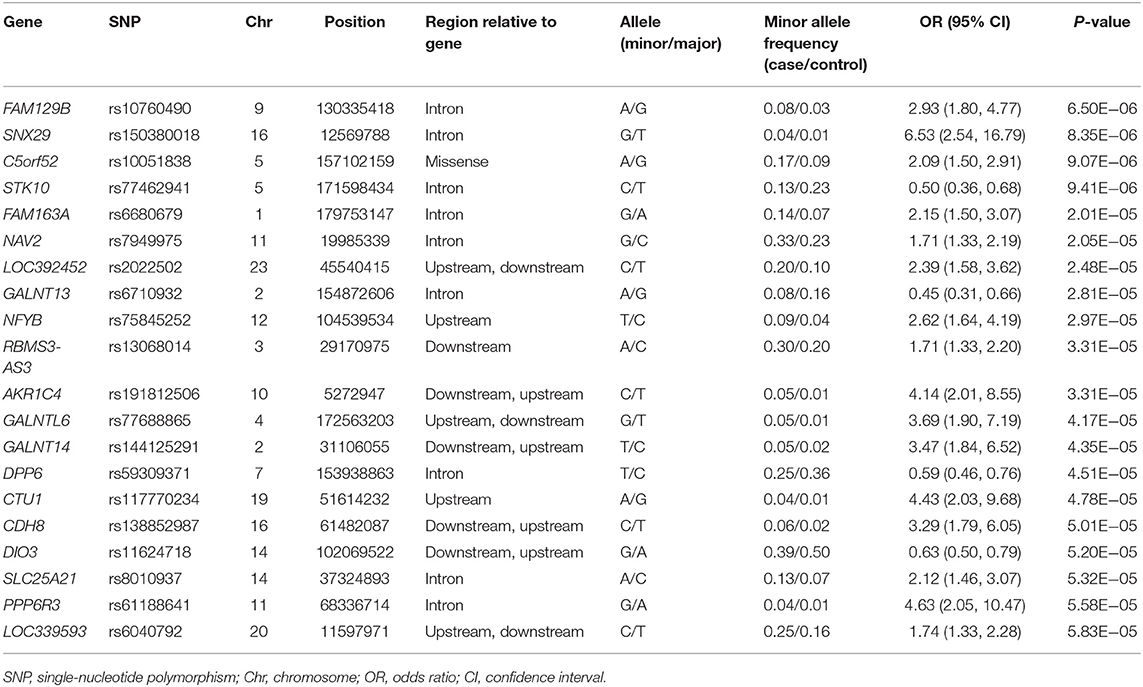
Table 2. Top 20 genomic variants associated with the occurrence of motor fluctuations, in decreasing order of statistical significance.
Levodopa-Induced Dyskinesia
Five years after the onset of PD, 172 patients had LID (23.2%). No difference was observed between patients with LID (75 men, 43.6%) and those without LID (250 men, 43.9%) (P = 0.892) in terms of sex. The mean age at onset of PD was lower in patients with LID than in those without (55.2 ± 10.7 years vs. 57.7 ± 9.8 years, P = 0.007). The mean duration between disease onset and the last follow-up was shorter in patients with LID than in those without LID (9.1 ± 3.5 years vs. 11.3 ± 4.6 years, P < 0.001; Supplementary Table 2). After quality controls, 583,379 SNPs were genotyped and analyzed. Quantile–quantile plots were made for the occurrence of LID (Supplementary Figure 3B), and a Manhattan plot is described in Figure 1B. The top 20 SNPs associated with the occurrence of LID 5 years after the onset of PD are listed in Table 3. The GALNT14 SNP rs144125291 had the lowest P-value and was significantly associated with LID even after Bonferroni correction (OR = 5.5, 95% CI = 2.9–10.3, P = 7.88 × 10−9; Table 3). The representative regional association plots of rs10495912, rs117999072, rs6737342, and rs6795866 showed other risk variants within 150 kb (Figures 2A–E).
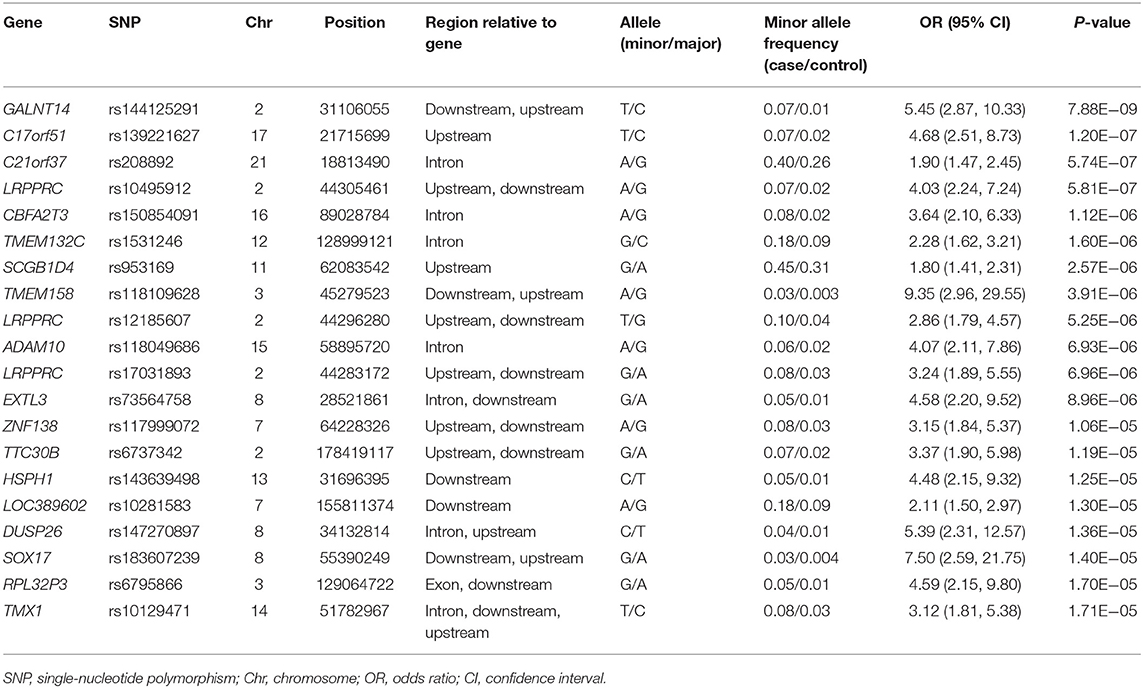
Table 3. Top 20 genomic variants associated with the occurrence of levodopa-induced dyskinesia, in decreasing order of statistical significance.
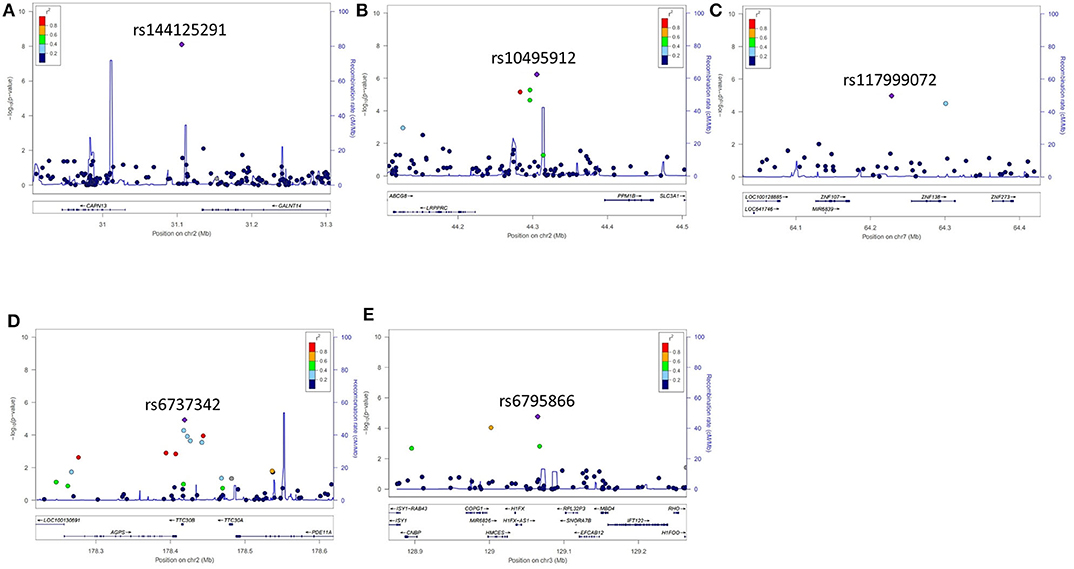
Figure 2. Representative regional plots for genetic variants that showed associations with the occurrence of levodopa-induced dyskinesia 5 years after the onset of Parkinson's disease. (A) rs144125291, (B) rs10495912, (C) rs117999072, (D) rs6737342, and (E) rs6795866.
Sensitivity Analysis for Patients With PD Aged ≥50 Years at the Onset of PD
The clinical features of patients with PD are presented in Table 4 and Supplementary Tables 3, 4. Five years after the onset of PD, 141 (24.4%) of 578 patients with PD exhibited motor fluctuations. A Manhattan plot is described in Figure 3A. The top 20 SNPs associated with the occurrence of motor fluctuations are listed in Table 5. RABL6 SNP rs191519045 had the lowest P-value, but none of the SNPs were significant after Bonferroni correction. Representative regional association plots of rs72850586, rs76767606, and rs12408511 showed other risk variants within 150 kb (Supplementary Figures 4A–C).
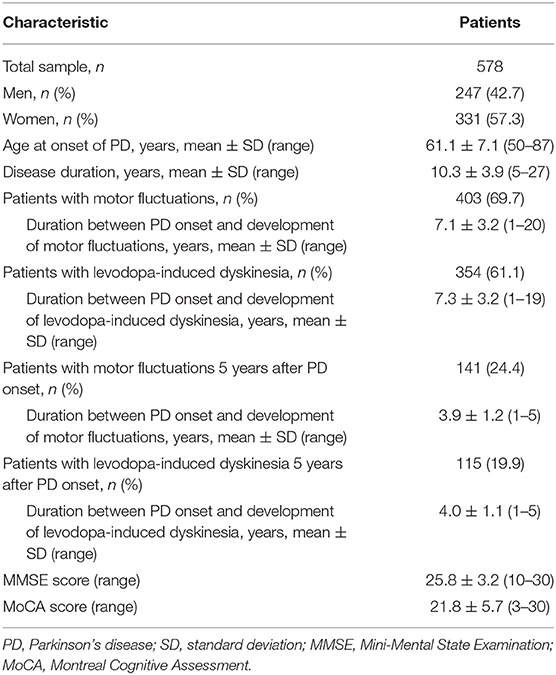
Table 4. Demographic and clinical characteristics of patients aged ≥50 years at onset of Parkinson's disease.
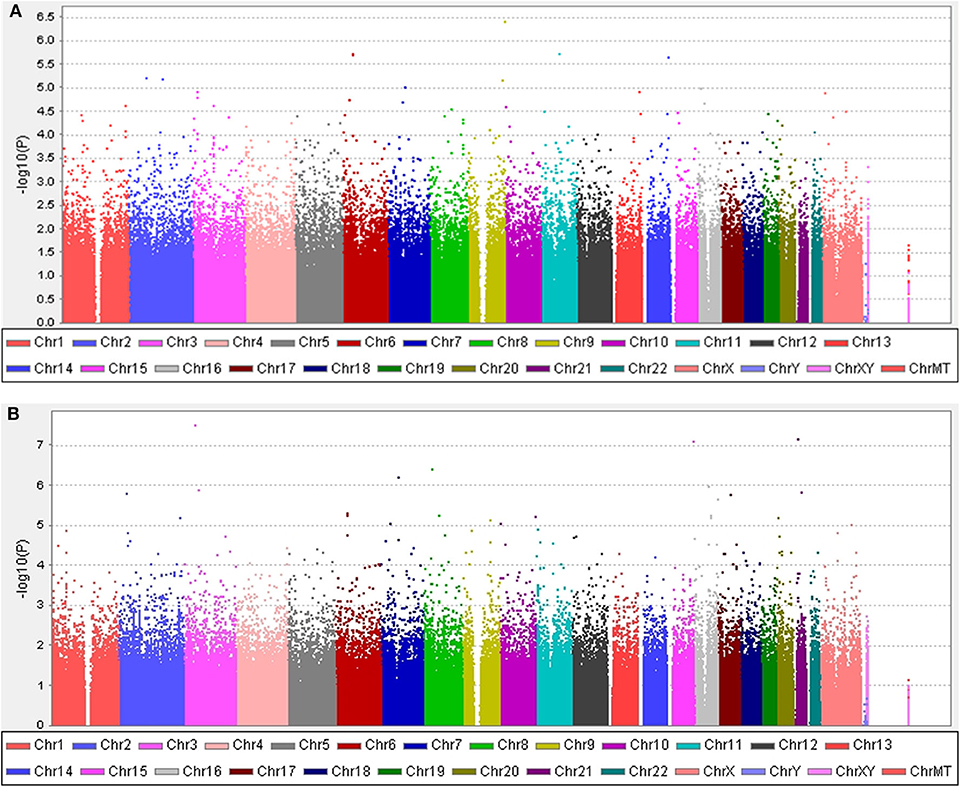
Figure 3. Manhattan plots. (A) The plot shows the P-values for association analyses between 580,128 SNPs and the occurrence of motor fluctuations 5 years after Parkinson's disease (PD) onset in patients aged ≥50 years at disease onset. The most significant association observed is with a locus on chromosome 9 (rs191519045). (B) The plot shows P-values for association analyses between 579,399 SNPs and the occurrence of levodopa-induced dyskinesia 5 years after onset of PD in patients aged ≥50 years at disease onset. The most significant association observed is with a locus on chromosome 3 (rs118109628). P-values are log-transformed (y-axis) and plotted against chromosomal position (x-axis).
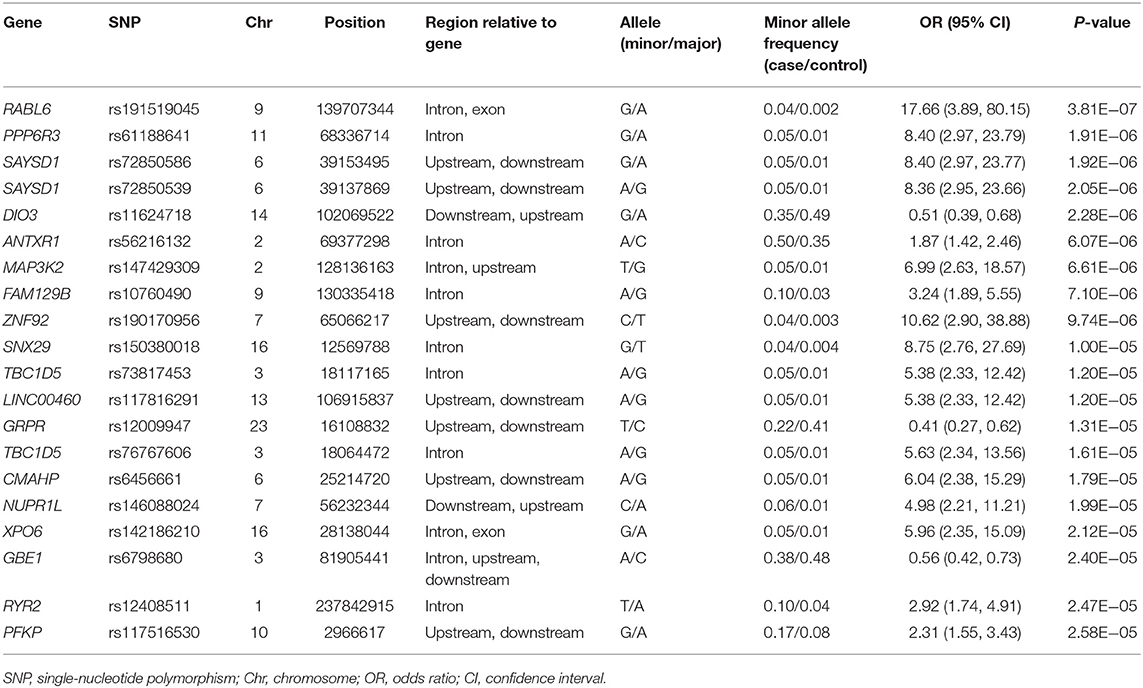
Table 5. Top 20 genomic variants associated with the occurrence of motor fluctuations in patients aged ≥50 years at onset of Parkinson's disease.
Five years after the onset of PD, 115 (19.9%) of 578 patients with PD had LID. A Manhattan plot is described in Figure 3B. The 20 SNPs associated with the occurrence of LID are listed in Table 6. None of these SNPs were significant after Bonferroni correction. Regional association plots of rs117999072, rs149201992, and rs6907129 showed other risk variants within 150 kb (Supplementary Figures 4D–F).
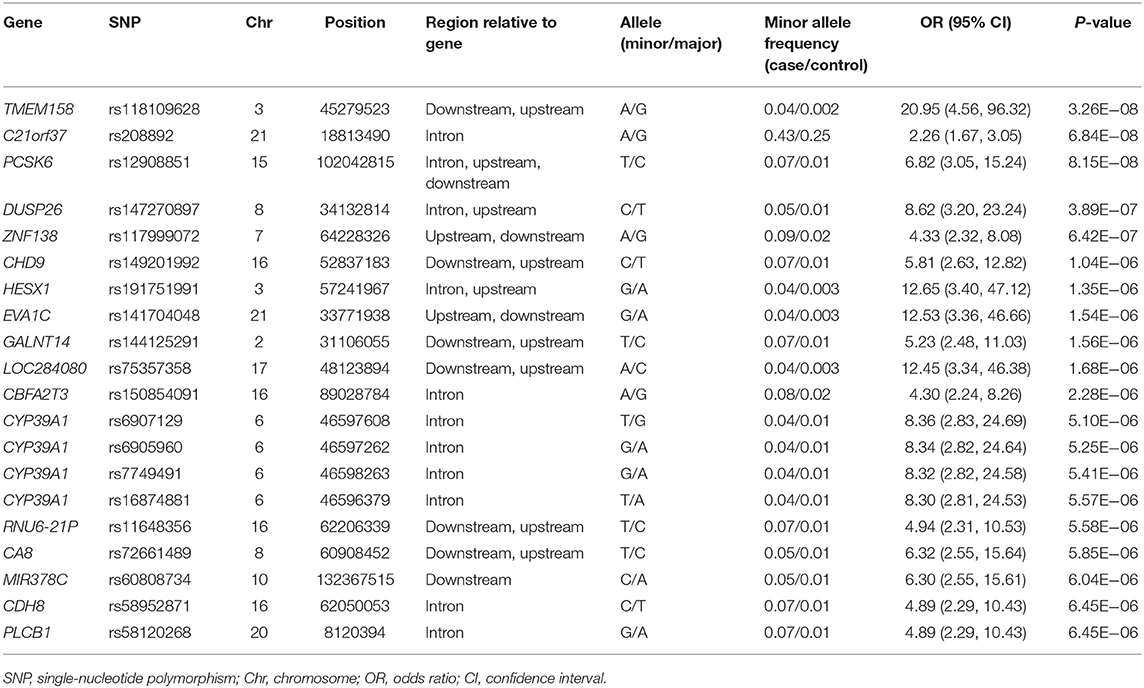
Table 6. Top 20 genomic variants associated with the occurrence of levodopa-induced dyskinesia in patients aged ≥50 years at the onset of Parkinson's disease.
Discussion
We found several genetic variants that showed associations with motor fluctuations and LID in patients with PD. The occurrence of motor fluctuations was associated with genetic variants in FAM129B, SNX29, C5orf52, and STK10 with P < 1.0 × 10−5, although the associations were not significant after Bonferroni correction. The occurrence of LID was most significantly associated with GALNT14 SNP rs144125291, and this association was significant after Bonferroni correction.
The pathophysiology of LID in PD is not well-understood. The functional state of the basal ganglia may be characterized by changes in the neuronal firing rate and oscillatory neuronal activity, which become excessive and possibly have a pathogenic role in the occurrence of abnormal corticostriatal connectivity (16). These mechanisms have been implicated in the pathophysiology of LID in PD. A polymorphism in brain-derived neurotrophic factor, recognized as modulating human cortical plasticity, affects the time to onset of LID in PD in addition to the response to rTMS (17, 18). Further studies using non-invasive brain stimulation techniques may be warranted to clarify the role of those genetic variants in LID.
GALNT14 SNP rs144125291 is located in the intergenic region 27,276 bases downstream of the gene variant for GALNT14. The GALNT14 gene encodes a Golgi protein that is a member of the polypeptide N-acetylgalactosaminyltransferase protein family (19). This enzyme catalyzes the transfer of N-acetyl-D-galactosamine to the hydroxyl group on serines and threonines in target peptides (19). Alterations in this gene may play a role in cancer progression and response to chemotherapy in several types of cancer (20–26). Some genes, such as LRRK2 and PRKN, may be associated with both cancer and PD (27–30). GALNT14 contributes to breast cancer invasion by altering cell proliferation and motility, by altering the expression levels of EMT genes, and by stimulating MMP-2 activity (31). MMP-2 is reported to play a role in the inflammatory response (32). GALNT14 may also cause abundant post-translational modifications, such as glycosylation, which is closely related to tumor growth and metastasis as well as resistance to chemotherapy (33). The development of LID in patients with PD is also related to altered post-synaptic transcription factors and maladaptive plasticity in the nigrostriatal neurons (34). Although the precise pathogenic mechanisms of LID remain unclear, chronic inflammation in the brain and altered post-synaptic plasticity may play key roles in the development of LID (34–36). GALNT14 SNP rs144125291 may affect the basal level of neuroinflammation in the brain or maladaptive post-synaptic plasticity. However, further functional studies are needed to elucidate the precise role of GALNT14 in LID.
Several other genes also showed possible association with the occurrence of LID, including LRPPRC. LRPPRC SNP rs10495912 showed a possible association with LID and is an intergenic variant located 60,028 bases upstream of LRPPRC. LRPPRC encodes a leucine-rich pentatricopeptide motif-containing protein that predominantly localizes to the mitochondria. The pentatricopeptide repeat (PPR) protein family plays a major role in RNA stability, regulation, processing, splicing, translation, and editing (37). LRPPRC regulates energy metabolism, and the maturation and export of nuclear mRNA. LRPPRC mutations have been found to cause Leigh syndrome in a French–Canadian population and are associated with reduced levels of LRPPRC and lower steady-state levels of mitochondrial transcripts (38). Leigh syndrome is an inherited neurometabolic disorder characterized by the occurrence of severe and deadly acidotic crises due to a tissue-specific deficiency in cytochrome c oxidase (38). An LRPPRC intronic variant can affect the normal splicing of LRPPRC and has been associated with susceptibility to PD (39). Mitochondrial susceptibility in the putamen is reported to play a role in the development of dyskinesia in patients with PD (40), suggesting that abnormal energy metabolism caused by LRPPRC variants may be associated with the occurrence of LID. However, further genetic and functional studies are needed to elucidate the role of LRPPRC in the development of LID.
Of the genes associated with the occurrence of motor fluctuations, FAM129B showed the lowest P-value (OR = 2.93, 95% CI = 1.8–4.8, P = 6.5 × 10−6). Knockdown of FAM129B in HeLa cells accelerates the onset of apoptosis induced by TNF-α (41). Activation of the inflammatory response is closely associated with the pathogenesis of PD, and the increased release of pro-inflammatory cytokines such as TNF-α, interleukin-1β, and interferon-γ has been observed in the post-mortem brain of a PD patient (42). In addition to susceptibility to PD, neuroinflammation in the striatum as well as in the substantia nigra pars compacta may play an important role in the development of motor fluctuations in PD via presynaptic and post-synaptic mechanisms. The storage hypothesis for motor fluctuations posits that the loss of presynaptic dopaminergic terminals reduces the capacity for storage of dopamine in the striatum, thereby inhibiting the ability to compensate for oscillations in plasma levodopa levels, and neuroinflammation may contribute to this effect (43). Neuroinflammation and chronic overproduction and abnormal release of TNF-α by microglia may also contribute to the post-synaptic mechanisms of motor fluctuations, which may be associated with complex striatal functional abnormalities in basal ganglia motor circuits (44). Further functional studies are necessary to investigate the precise role of FAM129B in neuroinflammation in PD.
TBC1D5, which showed a possible association with the occurrence of motor fluctuations in patients with PD aged over 50 years, functions as a GTPase-activating protein for RAB7 and inhibits recruitment of the VPS35/VPS29/VPS26 subcomplex to membranes (45). The retromer complex is a key component of the endosomal protein sorting machinery and mediates cargo selection through a trimeric complex comprising VPS35/VPS29/VPS26, which is recruited to endosomes by binding to RAB7a and SNX3 (46). This retromer function is closely linked to PD. VPS35 mutations are a rare cause of autosomal dominant late-onset PD. The clinical features of PD with VPS35 mutations were as follows: lower onset age, good response to levodopa, and motor complications (47). VPS13C mutations are a rare cause of autosomal recessive early-onset PD. The clinical features of PD with VPS13C mutations suggested that the progression is rapid and severe (48). Thus, VPS-related variants might be associated with motor complications in patients with PD. RYR2, which also associates with the occurrence of motor fluctuations in patients with PD aged over 50 years (P = 2.5 × 10−5), encodes a ryanodine receptor. Ryanodine receptors are intracellular calcium release channels found in the endoplasmic reticulum of all cells, with RYR2 predominating among the three isoforms (RYR1, RYR2, and RYR3) (49). When cellular Ca2+-regulating systems are compromised, synaptic dysfunction, impaired plasticity, and neuronal degeneration occur, such as in PD (50). Functional studies are needed to clarify the roles of TBC1D5 and RYR2 in the occurrence of motor fluctuations in PD.
The genetic association studies using a small number of pre-specified genetic region were able to determine the genetic risk variants for LID. A previous study reported that the Val158Met variant of catechol-O-methyltransferase was associated with LID (51). In another previous study, 229 (45.5%) of 503 Korean patients with PD experienced LID during the mean disease duration of 10.9 years (52). In their candidate gene association study, only the p.S9G variant of dopamine receptor D3 was associated with the occurrence of diphasic dyskinesia (52). However, these studies had limitations as only a limited number of candidate genes were selected due to their incomplete understanding of the pathophysiology of motor complications. Our GWAS investigated a genome-wide set of genetic variants, and this hypothesis-free GWAS may provide a comprehensive evaluation of genetic risk factors for motor complications.
This study has limitations. First, our study used retrospective clinical data. Motor fluctuations and LID are closely related to the pattern and dosage of dopaminergic medications, which were not randomized due to the inherent limitations of a retrospective study. The prevalence of motor fluctuations (29.6%) and LID (23.2%) was slightly lower in the present study than in the previous clinical studies (53, 54); however, this rate of motor complications may be dependent on the patterns of prescribing dopaminergic medications (55, 56). Recently, the prevalence of motor complications is now ~20–28%, which is comparable to what we observed (57, 58). Motor fluctuations and LID are complex phenomena where several factors may contribute to their development and further studies are required to better understand their pathophysiology (59). Second, we assessed the UPDRS for the evaluation of LID, but we did not use more specific assessment tools, such as Unified Dyskinesia Rating Scale, due to practical issues. Hence, future studies should perform a more detailed clinical assessment of LID. Third, our sample size was small compared with that of the traditional GWAS. Deep phenotyping in larger samples is challenging; thus, a well-designed GWAS on clinically important issues should be conducted.
In conclusion, this study provides new insights into the genetic contributions to motor fluctuations and LID in PD. Future collaborative longitudinal genomic studies are needed to further investigate the genetic risk factors associated with motor fluctuations and LID in patients with PD.
Data Availability Statement
The datasets presented in this study can be found in online repositories: https://www.ncbi.nlm.nih.gov/SNP/snp_viewBatch.cgi?sbid=1063124. The names of the repository/repositories and accession number(s) can be found in the article/Supplementary Material.
Ethics Statement
The studies involving human participants were reviewed and approved by the Institutional Review Board (IRB) of Asan Medical Center. The patients/participants provided their written informed consent to participate in this study.
Author Contributions
SC contributed to the conception, organization, execution of the research project, design, execution, review, critique of the statistical analysis, writing of the first draft, and review and critique of the manuscript. H-SR contributed to the execution of the research project, design, execution, review, critique of the statistical analysis, writing of the first draft, and review and critique of the manuscript. KP, NC, JiK, Y-MP, SJ, M-JK, YK, JuK, KK, and S-BK contributed to the execution of the research project, design, execution, review, critique of the statistical analysis, and review and critique of the manuscript.
Funding
This work was supported by a grant of the Korea Healthcare Technology R & D Project, Ministry of Health and Welfare, Republic of Korea (HI17C0328).
Conflict of Interest
The authors declare that the research was conducted in the absence of any commercial or financial relationships that could be construed as a potential conflict of interest.
Supplementary Material
The Supplementary Material for this article can be found online at: https://www.frontiersin.org/articles/10.3389/fneur.2020.00570/full#supplementary-material
References
1. Lang AE, Lozano AM. Parkinson's disease. First of two parts. N Engl J Med. (1998) 339:1044–53. doi: 10.1056/NEJM199810083391506
2. Lang AE, Lozano AM. Parkinson's disease. Second of two parts. N Engl J Med. (1998) 339:1130–43. doi: 10.1056/NEJM199810153391607
3. Holloway RG, Shoulson I, Kieburtz K, McDermott M, Shinaman A, Kamp C, et al. Pramipexole vs levodopa as initial treatment for Parkinson disease - a 4-year randomized controlled trial. Arch Neurol. (2004) 61:1044–53. doi: 10.1001/archneur.61.7.1044
4. Spillantini MG, Schmidt ML, Lee VM, Trojanowski JQ, Jakes R, Goedert M. Alpha-synuclein in Lewy bodies. Nature. (1997) 388:839–40. doi: 10.1038/42166
5. Braak H, Del Tredici K, Rub U, de Vos RA, Jansen Steur EN, Braak E. Staging of brain pathology related to sporadic Parkinson's disease. Neurobiol Aging. (2003) 24:197–211. doi: 10.1016/S0197-4580(02)00065-9
6. Jellinger KA. Neuropathology of nonmotor symptoms of Parkinson's disease. Int Rev Neurobiol. (2017) 133:13–62. doi: 10.1016/bs.irn.2017.05.005
7. Dickson DW. Neuropathology of Parkinson disease. Parkinsonism Relat Disord. (2018) 46(Suppl. 1):S30–3. doi: 10.1016/j.parkreldis.2017.07.033
8. Cotzias GC, Van Woert MH, Schiffer LM. Aromatic amino acids and modification of Parkinsonism. N Engl J Med. (1967) 276:374–9. doi: 10.1056/NEJM196702162760703
9. Diamond SG, Markham CH, Hoehn MM, McDowell FH, Muenter MD. Multi-center study of Parkinson mortality with early versus later dopa treatment. Ann Neurol. (1987) 22:8–12. doi: 10.1002/ana.410220105
10. Chapuis S, Ouchchane L, Metz O, Gerbaud L, Durif F. Impact of the motor complications of Parkinson's disease on the quality of life. Mov Disord. (2005) 20:224–30. doi: 10.1002/mds.20279
11. Blauwendraat C, Nalls MA, Singleton AB. The genetic architecture of Parkinson's disease. Lancet Neurol. (2019) 19:170–78. doi: 10.1016/S1474-4422(19)30287-X
12. Nalls MA, Blauwendraat C, Vallerga CL, Heilbron K, Bandres-Ciga S, Chang D, et al. Identification of novel risk loci, causal insights, and heritable risk for Parkinson's disease: a meta-analysis of genome-wide association studies. Lancet Neurol. (2019) 18:1091–102. doi: 10.1016/S1474-4422(19)30320-5
13. Gibb WR, Lees AJ. The relevance of the Lewy body to the pathogenesis of idiopathic Parkinson's disease. J Neurol Neurosurg Psychiatry. (1988) 51:745–52. doi: 10.1136/jnnp.51.6.745
14. Kalia LV, Lang AE. Parkinson's disease. Lancet. (2015) 386:896–912. doi: 10.1016/S0140-6736(14)61393-3
15. Shoulson I, Oakes D, Fahn S, Lang A, Langston JW, LeWitt P, et al. Impact of sustained deprenyl (selegiline) in levodopa-treated Parkinson's disease: a randomized placebo-controlled extension of the deprenyl and tocopherol antioxidative therapy of Parkinsonism trial. Ann Neurol. (2002) 51:604–12. doi: 10.1002/ana.10191
16. Alonso-Frech F, Zamarbide I, Alegre M, Rodríguez-Oroz MC, Guridi J, Manrique M, et al. Slow oscillatory activity and levodopa-induced dyskinesias in Parkinson's disease. Brain. (2006) 129:1748–57. doi: 10.1093/brain/awl103
17. Foltynie T, Cheeran B, Williams-Gray CH, Edwards MJ, Schneider SA, Weinberger D, et al. BDNF val66met influences time to onset of levodopa induced dyskinesia in Parkinson's disease. J Neurol Neurosurg Psychiatry. (2009) 80:141–4. doi: 10.1136/jnnp.2008.154294
18. Cheeran B, Talelli P, Mori F, Koch G, Suppa A, Edwards M, et al. A common polymorphism in the brain-derived neurotrophic factor gene (BDNF) modulates human cortical plasticity the response to rTMS. J Physiol. (2009) 586:5717–25. doi: 10.1113/jphysiol.2008.159905
19. Bennett EP, Mandel U, Clausen H, Gerken TA, Fritz TA, Tabak LA. Control of mucin-type O-glycosylation: a classification of the polypeptide GalNAc-transferase gene family. Glycobiology. (2012) 22:736–56. doi: 10.1093/glycob/cwr182
20. Song KH, Park MS, Nandu TS, Gadad S, Kim SC, Kim MY. GALNT14 promotes lung-specific breast cancer metastasis by modulating self-renewal and interaction with the lung microenvironment. Nat Commun. (2016) 7:13796. doi: 10.1038/ncomms13796
21. Liang KH, Yeh TS, Wu RC, Yeh CN, Yeh CT. GALNT14 genotype is associated with perineural invasion, lymph node metastasis and overall survival in resected cholangiocarcinoma. Oncol Lett. (2017) 13:4215–23. doi: 10.3892/ol.2017.5991
22. De Mariano M, Gallesio R, Chierici M, Furlanello C, Conte M, Garaventa A, et al. Identification of GALNT14 as a novel neuroblastoma predisposition gene. Oncotarget. (2015) 6:26335–46. doi: 10.18632/oncotarget.4501
23. Liang KH, Lin CC, Yeh CT. GALNT14 SNP as a potential predictor of response to combination chemotherapy using 5-FU, mitoxantrone and cisplatin in advanced HCC. Pharmacogenomics. (2011) 12:1061–73. doi: 10.2217/pgs.11.43
24. Lin WR, Chiang JM, Liang KH, Lim SN, Lai MW, Tsou YK, et al. GALNT14 genotype predicts postoperative outcome of stage III colorectal cancer with oxaliplatin as adjuvant chemotherapy. Medicine. (2016) 95:e3487. doi: 10.1097/MD.0000000000003487
25. Soria JC, Mark Z, Zatloukal P, Szima B, Albert I, Juhasz E, et al. Randomized phase II study of dulanermin in combination with paclitaxel, carboplatin, and bevacizumab in advanced non-small-cell lung cancer. J Clin Oncol. (2011) 29:4442–51. doi: 10.1200/JCO.2011.37.2623
26. Tsou YK, Liang KH, Lin WR, Chang HK, Tseng CK, Yeh CT. GALNT14 genotype as a response predictor for concurrent chemoradiotherapy in advanced esophageal squamous cell carcinoma. Oncotarget. (2017) 8:29151–60. doi: 10.18632/oncotarget.16253
27. Liu M, Shen C, Wang C. Long noncoding RNA LINC01133 confers tumor-suppressive functions in ovarian cancer by regulating leucine-rich repeat kinase 2 as an miR-205 sponge. Am J Pathol. (2019) 189:2323–39. doi: 10.1016/j.ajpath.2019.07.020
28. Gu S, Chen J, Zhou Q, Yan M, He J, Han X, et al. LRRK2 is associated with recurrence-free survival in intrahepatic cholangiocarcinoma and downregulation of LRRK2 suppresses tumor progress in vitro. Dig Dis Sci. (2019) 65:500–8. doi: 10.1007/s10620-019-05806-0
29. Agalliu I, Ortega RA, Luciano MS, Mirelman A, Pont-Sunyer C, Brockmann K, et al. Cancer outcomes among Parkinson's disease patients with leucine rich repeat kinase 2 mutations, idiopathic Parkinson's disease patients, and nonaffected controls. Mov Disord. (2019) 34:1392–8. doi: 10.1002/mds.27807
30. Takeda M, Koseki J, Takahashi H, Miyoshi N, Nishida N, Nishimura J, et al. Disruption of endolysosomal RAB5/7 efficiently eliminates colorectal cancer stem cells. Cancer Res. (2019) 79:1426–37. doi: 10.1158/0008-5472.CAN-18-2192
31. Tian HN, Zuo T, Wang XF, An LF, Xie YY, Wang JH, et al. GALNT14 mediates tumor invasion and migration in breast cancer cell MCF-7. Mol Carcinogen. (2015) 54:1159–71. doi: 10.1002/mc.22186
32. Singh D, Srivastava SK, Chaudhuri TK, Upadhyay G. Multifaceted role of matrix metalloproteinases (MMPs). Front Mol Biosci. (2015) 2:19. doi: 10.3389/fmolb.2015.00019
33. Lau KS, Dennis JW. N-glycans in cancer progression. Glycobiology. (2008) 18:750–60. doi: 10.1093/glycob/cwn071
34. Cenci MA, Lundblad M. Post- versus presynaptic plasticity in L-DOPA-induced dyskinesia. J Neurochem. (2006) 99:381–92. doi: 10.1111/j.1471-4159.2006.04124.x
35. Del-Bel E, Bortolanza M, Dos-Santos-Pereira M, Bariotto K, Raisman-Vozari R. l-DOPA-induced dyskinesia in Parkinson's disease: are neuroinflammation and astrocytes key elements? Synapse. (2016) 70:479–500. doi: 10.1002/syn.21941
36. Pisanu A, Boi L, Mulas G, Spiga S, Fenu S, Carta AR. Neuroinflammation in L-DOPA-induced dyskinesia: beyond the immune function. J Neural Transm. (2018) 125:1287–97. doi: 10.1007/s00702-018-1874-4
37. Cui J, Wang L, Ren X, Zhang Y, Zhang H. LRPPRC: a multifunctional protein involved in energy metabolism and human disease. Front Physiol. (2019) 10:595. doi: 10.3389/fphys.2019.00595
38. Burelle Y, Bemeur C, Rivard ME, Thompson Legault J, Boucher G, Consortium L, et al. Mitochondrial vulnerability and increased susceptibility to nutrient-induced cytotoxicity in fibroblasts from Leigh syndrome French Canadian patients. PLoS ONE. (2015) 10:e0120767. doi: 10.1371/journal.pone.0120767
39. Gaweda-Walerych K, Mohagheghi F, Zekanowski C, Buratti E. Parkinson's disease-related gene variants influence pre-mRNA splicing processes. Neurobiol Aging. (2016) 47:127–38. doi: 10.1016/j.neurobiolaging.2016.07.014
40. Naydenov AV, Vassoler F, Luksik AS, Kaczmarska J, Konradi C. Mitochondrial abnormalities in the putamen in Parkinson's disease dyskinesia. Acta Neuropathol. (2010) 120:623–31. doi: 10.1007/s00401-010-0740-8
41. Chen S, Evans HG, Evans DR. FAM129B/MINERVA, a novel adherens junction-associated protein, suppresses apoptosis in HeLa cells. J Biol Chem. (2011) 286:10201–9. doi: 10.1074/jbc.M110.175273
42. Kannarkat GT, Boss JM, Tansey MG. The role of innate and adaptive immunity in Parkinson's disease. J Parkinsons Dis. (2013) 3:493–514. doi: 10.3233/JPD-130250
43. Chou KL, Stacy M, Simuni T, Miyasaki J, Oertel WH, Sethi K, et al. The spectrum of “off” in Parkinson's disease: what have we learned over 40 years? Parkinsonism Relat Disord. (2018) 51:9–16. doi: 10.1016/j.parkreldis.2018.02.001
44. Obeso JA, Rodriguez-Oroz M, Marin C, Alonso F, Zamarbide I, Lanciego JL, et al. The origin of motor fluctuations in Parkinson's disease: importance of dopaminergic innervation and basal ganglia circuits. Neurology. (2004) 62(1 Suppl. 1):S17–30. doi: 10.1212/WNL.62.1_suppl_1.S17
45. Seaman MN, Harbour ME, Tattersall D, Read E, Bright N. Membrane recruitment of the cargo-selective retromer subcomplex is catalysed by the small GTPase Rab7 and inhibited by the Rab-GAP TBC1D5. J Cell Sci. (2009) 122:2371–82. doi: 10.1242/jcs.048686
46. Seaman MNJ, Mukadam AS, Breusegem SY. Inhibition of TBC1D5 activates Rab7a and can enhance the function of the retromer cargo-selective complex. J Cell Sci. (2018) 131:jcs.217398. doi: 10.1242/jcs.217398
47. Deng H, Gao K, Jankovic J. The VPS35 gene and Parkinson's disease. Mov Disord. (2013) 25:569–75. doi: 10.1002/mds.25430
48. Puschmann A. New genes causing hereditary Parkinson's disease or Parkinsonism. Curr Neurol Neurosci Rep. (2017) 17:66. doi: 10.1007/s11910-017-0780-8
49. Abu-Omar N, Das J, Szeto V, Feng ZP. Neuronal ryanodine receptors in development and aging. Mol Neurobiol. (2018) 55:1183–92. doi: 10.1007/s12035-016-0375-4
50. Mattson MP. Calcium and neurodegeneration. Aging Cell. (2007) 6:337–50. doi: 10.1111/j.1474-9726.2007.00275.x
51. de Lau LM, Verbaan D, Marinus J, Heutink P, van Hilten JJ. Catechol-O-methyltransferase Val158Met and the risk of dyskinesias in Parkinson's disease. Mov Disord. (2012) 27:132–5. doi: 10.1002/mds.23805
52. Lee JY, Cho J, Lee EK, Park SS, Jeon BS. Differential genetic susceptibility in diphasic and peak-dose dyskinesias in Parkinson's disease. Mov Disord. (2011) 26:73–9. doi: 10.1002/mds.23400
53. Ahlskog JE, Muenter MD. Frequency of levodopa-related dyskinesias and motor fluctuations as estimated from the cumulative literature. Mov Disord. (2001) 16:448–58. doi: 10.1002/mds.1090
54. Fabbrini G, Defazio G, Colosimo C, Suppa A, Beradelli A. Onset and spread of dyskinesia and motor symptoms in Parkinson's disease. Mov Disord. (2009) 24:2091–6. doi: 10.1002/mds.22703
55. Chaudhuri KR, Jenner P, Antonini A. Should there be less emphasis on levodopa-induced dyskinesia in Parkinson's disease? Mov Disord. (2019) 34:816–9. doi: 10.1002/mds.27691
56. Cenci MA, Riggare S, Pahwa R, Eidelberg D, Hauser RA. Dyskinesia matters. Mov Disord. (2019) 35:392–96. doi: 10.1002/mds.27959
57. Schrag A, Ben-Shlomo Y, Quinn N. How common are complications of Parkinson's disease? J Neurol. (2002) 249:419–23. doi: 10.1007/s004150200032
58. Scott NW, Macleod AD, Counsell CE. Motor complications in an incident Parkinson's disease cohort. Eur J Neurol. (2016) 23:304–12. doi: 10.1111/ene.12751
Keywords: genome-wide association study, genomic variants, Parkinson's disease, motor fluctuations, levodopa-induced dyskinesia
Citation: Ryu H-S, Park KW, Choi N, Kim J, Park Y-M, Jo S, Kim M-J, Kim YJ, Kim J, Kim K, Koh S-B and Chung SJ (2020) Genomic Analysis Identifies New Loci Associated With Motor Complications in Parkinson's Disease. Front. Neurol. 11:570. doi: 10.3389/fneur.2020.00570
Received: 23 January 2020; Accepted: 19 May 2020;
Published: 07 July 2020.
Edited by:
Nobutaka Hattori, Juntendo University, JapanReviewed by:
Graziella Mangone, INSERM CIC1422 CIC Pitié Neurosciences, FranceLuca Marsili, University of Cincinnati, United States
Copyright © 2020 Ryu, Park, Choi, Kim, Park, Jo, Kim, Kim, Kim, Kim, Koh and Chung. This is an open-access article distributed under the terms of the Creative Commons Attribution License (CC BY). The use, distribution or reproduction in other forums is permitted, provided the original author(s) and the copyright owner(s) are credited and that the original publication in this journal is cited, in accordance with accepted academic practice. No use, distribution or reproduction is permitted which does not comply with these terms.
*Correspondence: Sun Ju Chung, sjchung@amc.seoul.kr