- 1Basic and Clinical Neuroscience, Institute of Psychiatry, Psychology and Neuroscience, King's College London, London, United Kingdom
- 2NIHR-Wellcome Trust King's Clinical Research Facility/SLaM Biomedical Research Centre, King's College Hospital, London, United Kingdom
- 3Department of Ophthalmology, King's College Hospital NHS Trust, London, United Kingdom
Neuroimaging plays an essential role in the diagnostic workup of idiopathic intracranial hypertension with the aims to exclude secondary causes of elevated intracranial pressure and to identify imaging signs that are commonly observed in this disorder. As a valuable expansion of brain imaging, the imaging of the retina using optical coherence tomography has been of increasing value. In particular, this is the case with the latest devices that allow a more accurate distinction between a reduction in retinal nerve fiber layer thickness due to an improvement of papilledema or due to a worsening caused by optic nerve atrophy. Although optical coherence tomography does not yet replace the other elements of the diagnostic workup, it is likely to play an increasing role in diagnosis and follow-up of idiopathic intracranial hypertension. The review focuses on the main findings in neuroimaging, including structural and vascular alterations as well as on the relevance of optical coherence tomography.
Introduction
Idiopathic intracranial hypertension (IIH) is defined as an elevation of intracranial pressure (ICP) in the absence of a brain lesion or any other secondary etiology (1). IIH generally affects obese young women of childbearing age. Given the relationship to obesity, the prevalence of IIH, which is currently estimated at 0.5–2.0 per 100,000 of the general population (2), is increasing along with the worldwide increasing incidence of obesity (3). The potential similarity of the clinical picture to primary headaches, in particular chronic migraine, probably results in IIH still being underdiagnosed (4).
In this article, we review our understanding of IIH with a special focus on the current imaging techniques and their utility in diagnosing and managing IIH.
Clinical Picture
The clinical picture of IIH is dominated by headache and ophthalmic features resulting from the pressure-induced papilledema (1, 5). Headache is the most common clinical symptom of IIH and a key factor in the reduction of quality of life (4, 6). The headache can vary substantially in its clinical presentation, hence, the relatively unspecific definition in the diagnostic criteria of the International Headache Society. Frequently, the headache has a migraine phenotype, raising the question to what extent is the headache primarily driven by the elevated ICP or by a pressure-induced exacerbation of a pre-existing migraine. This uncertainty is fuelled further by the fact that most IIH patients do not experience a sustained improvement of their headache once ICP is normalized with an adequate treatment. Despite causing major morbidity in IIH and having an immense impact on patients' quality of life, no clinical trials exist that focus exclusively on the headache component of IIH (7).
The second cardinal feature of IIH is the papilledema caused by the elevation of ICP. In the majority of cases, papilledema is bilateral, but in up to 4% of cases, it can be asymmetrical. If IIH is untreated, the papilledema leads to numerous visual symptoms, including visual field defects, obscurations, and ultimately to the complete loss of eyesight resulting from an atrophy of the optic nerve. Due to the potential irreversibility of visual symptoms, a quick and accurate assessment is essential in the diagnostic workup of IIH.
Pathophysiology
The pathophysiology remains unknown although our understanding has evolved significantly over the last decades. The first studies led to the belief that IIH may be due to increased CSF (8–10). This idea was refuted by Dandy and coworkers in the late 1930s as they saw no ventricular size alteration on ventriculography. The authors hypothesized IIH was related to an increased intracranial blood volume as a result of vasomotor control (11). Indeed, they suggested changes in the vascular bed would explain better the rapid changes in the intracranial pressure they observed. In the early 1950s, venography studies showed obstruction of the superior sagittal sinus (SSS) and dominant transverse sinus (12). Studies, including brain biopsy, in the late 1950s demonstrated intracellular and extracellular cerebral edema (13, 14). In the 1970s, after performing isotope cisternography and ventriculography, Johnston and coworkers hypothesized that a pressure increase within the SSS may lead to reduced CSF absorption (15). Raichle and coworkers, utilizing tracer techniques, showed a reduction in cerebral blood flow despite an increase in cerebral blood volume and pointed to an abnormality in the cerebral microvasculature (16). More recently, in 1995, with the use of cerebral venography and manometry, venous hypertension was shown in the SSS and the transverse sinus (17). In line with some studies performed in the 1930s and 1950s, a 3D volumetric MR imaging study showed normal ventricular volume in IIH. Nevertheless, the authors also observed increased extraventricular CSF volume (18). A phase-contrast MRI performed to measure the interaction between CSF and blood flows demonstrated the presence of a small phase shift of venous outflow leading to increased arteriovenous pulsatility, which ultimately would lead to an increase in CSF and (ICP) (19). The venous sinus stenosis hypothesis has led to venous sinus stenting as a therapy, the efficacy of which appears to be related to the pressure gradient prior to surgery (18).
Recently, the role obesity may play has also been addressed. A pathophysiological link is supported by reports of patients whose CSF opening pressure was normalized following bariatric surgery (20). Indeed, two cases showed reduction in venous sinus pressure as measured by intracranial venography following surgery (21). As a causative factor, recent evidence points to androgen excess, specifically testosterone, concentrations of which were found to be higher in both blood and CSF as compared to obese females with and without polycystic ovary syndrome (22).
Structural MRI
Structural MRI is a key element in the diagnostic workup of IIH with the aim of ruling out a secondary cause of elevated ICP and to identify neuroimaging signs that are typically observed in IIH. One of the most suggestive neuroimaging abnormalities that is highly suggestive of IIH is the reduction of the midsagittal height of the pituitary gland (“empty sella”) (23). This is reflected in a significant reduction in its volume when performing an MR-based volumetric measurement (24). It is not entirely clear how a long-term increase of ICP causes the size reduction of the pituitary gland, but it is thought to be the result of a herniation of arachnocele through the diaphragma sellae (25). Interestingly, most abnormal morphometric neuroimaging findings do not improve after CSF pressure has been normalized and papilledema has resolved (26). However, healthy participants in research studies or patients who are scanned for a different reason may show an “empty sella.” Although, in the context of IIH, treatment should be based on the principle of treating clinical symptoms and not radiological signs, recent evidence suggests that a close follow-up of these patients may be recommendable (27).
Another typical neuroimaging finding in IIH is the distension of the optic nerve sheath (ONS) observed on T2-weighted MR-images (23, 24, 28). The distension of the ONS results from increased CSF pressure in the perioptic subarachnoid space. The adaptation of CSF pressure in the ONS to the ICP is not immediate due to the capillary CSF communication in the optic canal. For this reason, changes in the ONS are not seen in acute ICP changes (e.g., intracranial hemorrhage) (29) or within a few hours after normalization of ICP (30) although the exact time of the delay remains unknown. In contrast, although the ONS shows a macroscopic distention and the optic nerve may appear tortuous, the size and volume of the optic nerve remain unchanged (23, 24). However, when imaging is performed with diffusion tensor imaging (DTI) to analyze microstructural properties, changes in the optic nerve are identified (28, 30). These changes are in line with a microstructural tissue compression and are reversed after normalization of ICP (30). The fact that microstructural alterations within the optic nerve improve within 24 hours of lumbar puncture but the macroscopic size of the ONS does not highlights the delayed effect on the perioptic space after normalization of ICP and may suggest a higher sensitivity when imaging microscopic alterations using DTI compared to macroscopic changes in ONS using T2-weighted MRI. Nevertheless, the data from this study is based on a small number of patients and, therefore, requires a larger study to be confirmed (30). In line with microstructural imaging of the optic nerve, DTI of the optic disc shows abnormal values of fractional anisotropy in patients with IIH compared to healthy controls (31).
A posterior flattening of the optic globe is also commonly observed, but compared to the previously mentioned neuroimaging signs, it has an inferior sensitivity (23).
Finally, unilateral or bilateral transverse sinus stenoses (TSS) are commonly observed in IIH. Data on the prevalence of TSS in IIH vary substantially as MR-venography is frequently affected by imaging artifacts. It still remains controversial whether these are the cause or consequence of elevated ICP. However, increasing evidence suggest that TSS are secondary to increased ICP as they can resolve after normalization of ICP (32). The fact that bilateral stenting can resolve elevated ICP could be explained by a vicious cycle in which elevated ICP causes compression of the transverse sinuses, further aggravating the situation by obstructing venous outflow and thereby reducing the pressure gradient over the arachnoid villi (4, 33).
Optical Coherence Tomography
Optical coherence tomography (OCT) uses a low-energy near-infrared laser beam that is projected onto the retina, and the light reflected from the retina interacts with a reference laser beam to create an interference pattern, which is analyzed to determine the reflectance of retinal tissue at different depths (34). Up to 100,000 points are scanned per second, creating exquisitely detailed profiles (axial resolution currently up to 3 μm) from which thickness maps of different retinal layers can be derived. Modern spectral-domain and swept-source OCTs use en-face laser ophthalmoscopic images of fundus vessels to ensure that follow-up scans in a given patient are exactly aligned with baseline scans, allowing tiny changes in retinal elevation and the thickness of individual retinal layers to be reliably measured.
OCT Measurement of Papilledema in IIH
OCT has a well-established role in assessing and monitoring papilledema (35–37). A number of different OCT scanning protocols are used to assess the optic disc in ophthalmology. The most widely used is a 3.4 mm line scan measuring retinal nerve fiber layer thickness (pRNFL). Papilledema causes thickening of the pRNFL, and greater thickness is associated with higher lumbar puncture opening pressure (35, 38, 39). In very early papilledema, retinal nerve fiber layer thickening may not extend far enough from the disc to be picked up by a pRNFL scan (40) although, in severe RNFL thickening, automated segmentation analysis is often unreliable, requiring manual correction to ensure valid longitudinal data (35).
A variety of OCT scanning strategies have been described to quantify the elevation and volume of the disc itself in papilledema, which may offer some advantages over conventional pRNFL scans, especially in very early swelling (41–45). It has been shown that treatment of IIH with acetazolamide, successful weight loss, or ventriculo-peritoneal shunt causes corresponding improvement in OCT measures of disc height, volume, and pRNFL (43, 44, 46, 47) (Figure 1).
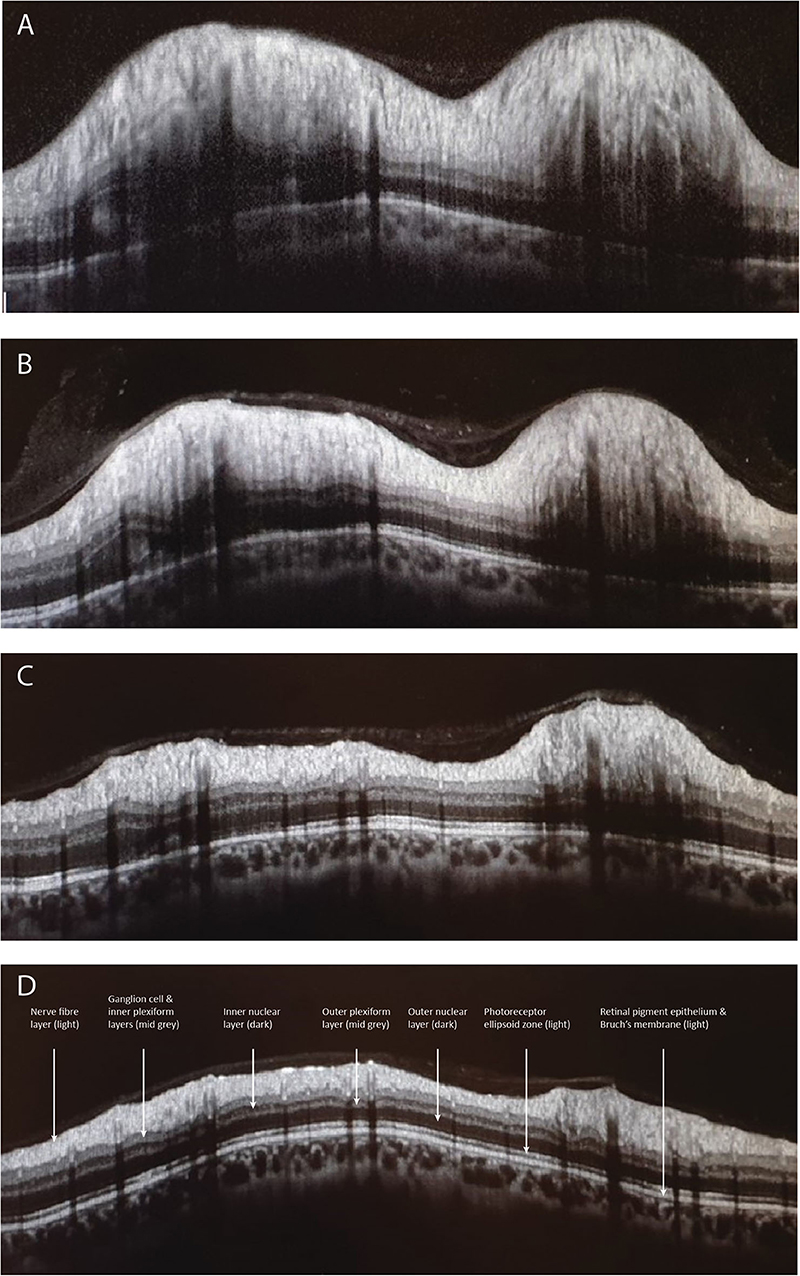
Figure 1. Depicts the peripapillary retinal nerve fiber thickness (pRNFL) scan performed with optical coherence tomography. Image (A) illustrates a pRNFL scan with severe disc swelling in IIH compared to day 5 (B), day 30 (C), and day 70 (D) after placing a ventriculoperitoneal shunt.
Deformation of the Peripapillary Retina
A number of methods have been described for measuring deformation of the layers deep into the neural retina (peri-papillary retinal pigment epithelium and Bowman's membrane) toward the vitreous, equivalent to inward deformation of the posterior sclera seen on MRI. The degree of deformation is related to lumbar puncture opening pressure and improves with ICP-lowering treatment (36, 41, 43, 48–50).
Diagnosis of Pseudopapilledema
OCT can readily distinguish tilted discs, the crowded hypermetropic discs, and buried disc drusen from true papilledema. The use of enhanced depth imaging allows OCT to image as deep as the lamina cribrosa of the sclera to detect even very small drusen (51–53).
OCT Macular Ganglion Cell Layer Imaging in IIH
A significant challenge in monitoring IIH-related papilledema is to determine whether a reduction in the degree of disc or pRNFL swelling is due to improvement of edema due to falling ICP from successful treatment or, conversely, to the loss of RNFL fibers as optic atrophy develops. Macular OCT imaging is extremely helpful in this situation. The macular ganglion cell layer (mGCL), which contains the cell bodies of axons of the optic nerve, does not swell in papilledema. Disc damage due to papilledema causes early thinning of the mGCL before frank thinning of the pRNFL develops (47, 54) (Figure 2). Conversely, finding that a patient with chronic papilledema despite medical therapy has no thinning (or no progression of thinning) of the mGCL offers reassurance that the optic nerve is not losing axons at an abnormal rate.
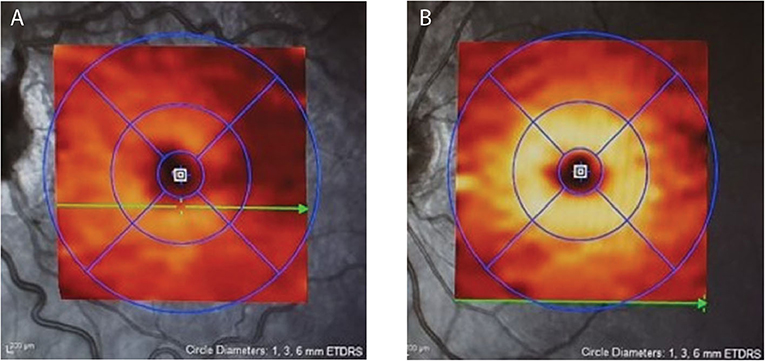
Figure 2. Depicts the moderate macular ganglion cell layer (mGCL) thinning in IIH (A) vs. a healthy control (B). Note on image A the dilated, tortuous veins resulting from papilledema.
Other Applications of OCT Systems in IIH
Various other OCT features may have value in diagnosing or monitoring IIH. These include imaging retinal and choroidal folds due to papilledema, of which some subtypes may improve with treatment (55, 56); OCT imaging of venular diameter, which increases in papilledema and decreases when elevated ICP is reduced (57); and OCT angiographic imaging of peripapillary capillaries, which have increased diameter and tortuosity in papilledema (58).
In patients suspected of having IIH without papilledema or in whom established optic atrophy prevents disc swelling, OCT systems can be used to obtain motion-stabilized laser ophthalmoscopic videos, which are extremely sensitive in detecting spontaneous retinal venous pulsation (SVP) (59). The presence of SVP signifies a healthy pressure gradient between the eye and the retrobulbar perineural CSF, and videography using OCT systems has shown that SVP reliably disappears when ICP becomes moderately elevated (60).
Conclusions
Neuroimaging in IIH has substantially improved diagnostic accuracy in IIH. Although it is unlikely that it will replace diagnostic lumbar puncture, it is feasible that, in a few years, improved MR imaging, including microstructural imaging as well as the rapidly improving quality of OCT imaging of papilledema, may offer a possibility to reduce the number of lumbar punctures for diagnostic follow-up as they could provide reliable markers that could be used in indirectly assessing ICP (32, 61).
Author Contributions
DM-A, JM, and JH performed the literature review and drafted the manuscript.
Conflict of Interest
JH is consulting for and/or serves on advisory boards of Allergan, Autonomic Technologies, Inc. (ATI), Chordate Medical AB, Eli Lilly, Hormosan Pharma, Novartis and Teva. He has received honoraria for speaking from Allergan, Chordate Medical AB, Novartis and Teva. He received personal fees for Medico-Legal Work as well as from Sage Publishing, Springer Healthcare and Quintessence Publishing.
The remaining authors declare that the research was conducted in the absence of any commercial or financial relationships that could be construed as a potential conflict of interest.
References
1. Hoffmann J, Mollan SP, Paemeleire K, Lampl C, Jensen RH, Sinclair AJ. European headache federation guideline on idiopathic intracranial hypertension. J Headache Pain. (2018) 19:93. doi: 10.1186/s10194-018-0919-2
2. Markey KA, Mollan SP, Jensen RH, Sinclair AJ. Understanding idiopathic intracranial hypertension: mechanisms, management, and future directions. Lancet Neurol. (2016) 15:78–91. doi: 10.1016/S1474-4422(15)00298-7
3. Almarzouqi SJ, Morgan ML, Lee AG. Idiopathic intracranial hypertension in the Middle East: A growing concern. Saudi J Ophthalmol. (2015) 29:26–31. doi: 10.1016/j.sjopt.2014.09.013
4. Hoffmann J, Goadsby PJ. Update on intracranial hypertension and hypotension. Curr Opin Neurol. (2013) 26:240–7. doi: 10.1097/WCO.0b013e328360eccc
5. Friedman DI, Liu GT, Digre KB. Revised diagnostic criteria for the pseudotumor cerebri syndrome in adults and children. Neurology. (2013) 81:1159–65. doi: 10.1212/WNL.0b013e3182a55f17
6. Mulla Y, Markey KA, Woolley RL, Patel S, Mollan SP, Sinclair AJ. Headache determines quality of life in idiopathic intracranial hypertension. J Headache Pain. (2015) 16:45. doi: 10.1186/s10194-015-0521-9
7. Mollan SP, Hoffmann J, Sinclair AJ. Advances in the understanding of headache in idiopathic intracranial hypertension. Curr Opin Neurol. (2019) 32:92–8. doi: 10.1097/WCO.0000000000000651
8. Bandyopadhyay S. Pseudotumor cerebri. Arch Neurol. (2001) 58:1699–701. doi: 10.1001/archneur.58.10.1699
9. Degnan AJ, Levy LM. Pseudotumor cerebri: brief review of clinical syndrome and imaging findings. AJNR Am J Neuroradiol. (2011) 32:1986–93. doi: 10.3174/ajnr.A2404
10. Johnston I. The historical development of the pseudotumor concept. Neurosurg Focus. (2001) 11:E2. doi: 10.3171/foc.2001.11.2.3
11. Dandy WE. Intracranial pressure without brain tumor: diagnosis and treatment. Ann Surg. (1937) 106:492–513. doi: 10.1097/00000658-193710000-00002
12. Ray BS, Dunbar HS. Thrombosis of the dural venous sinuses as a cause of pseudotumor cerebri. Ann Surg. (1951) 134:376–86. doi: 10.1097/00000658-195113430-00009
13. Foley J. Benign forms of intracranial hypertension; toxic and otitic hydrocephalus. Brain. (1955) 78:1–41. doi: 10.1093/brain/78.1.1
14. Joynt RJ, Sahs AL. Brain swelling of unknown cause. Neurology. (1956) 6:801–3. doi: 10.1212/WNL.6.11.791
15. Johnston I, Paterson A. Benign intracranial hypertension. II. CSF pressure and circulation. Brain. (1974) 97:301–12. doi: 10.1093/brain/97.1.301
16. Raichle ME, Grubb RL Jr, Phelps ME, Gado MH, Caronna JJ. Cerebral hemodynamics and metabolism in pseudotumor cerebri. Ann Neurol. (1978) 4:104–11. doi: 10.1002/ana.410040203
17. King JO, Mitchell PJ, Thomson KR, Tress BM. Cerebral venography and manometry in idiopathic intracranial hypertension. Neurology. (1995) 45:2224–8. doi: 10.1212/WNL.45.12.2224
18. McDougall CM, Ban VS, Beecher J, Pride L, Welch BG. Fifty shades of gradients: does the pressure gradient in venous sinus stenting for idiopathic intracranial hypertension matter? A systematic review. J Neurosurg. (2018) 130:999. doi: 10.3171/2017.8.JNS17459
19. Capel C, Baroncini M, Gondry-Jouet C, Bouzerar R, Czosnyka M, Czosnyka Z, et al. Cerebrospinal fluid and cerebral blood flows in idiopathic intracranial hypertension. Acta Neurochir Suppl. (2018) 126:237–41. doi: 10.1007/978-3-319-65798-1_48
20. Sugerman HJ, Felton WL 3rd, Salvant JB Jr, Sismanis A, Kellum JM. Effects of surgically induced weight loss on idiopathic intracranial hypertension in morbid obesity. Neurology. (1995) 45:1655–9. doi: 10.1212/WNL.45.9.1655
21. Nadkarni T, Rekate HL, Wallace D. Resolution of pseudotumor cerebri after bariatric surgery for related obesity. J Neurosurg. (2004) 101:878–80. doi: 10.3171/jns.2004.101.5.0878
22. O'Reilly MW, Westgate CS, Hornby C, Botfield H, Taylor AE, Markey K, et al. A unique androgen excess signature in idiopathic intracranial hypertension is linked to cerebrospinal fluid dynamics. JCI Insight. (2019) 4:e125348. doi: 10.1172/jci.insight.125348
23. Hoffmann J, Huppertz HJ, Schmidt C, Kunte H, Harms L, Klingebiel R, et al. Morphometric and volumetric MRI changes in idiopathic intracranial hypertension. Cephalalgia. (2013) 33:1075–84. doi: 10.1177/0333102413484095
24. Hoffmann J, Schmidt C, Kunte H, Klingebiel R, Harms L, Huppertz H-J, et al. Volumetric assessment of optic nerve sheath and hypophysis in idiopathic intracranial hypertension. AJNR Am J Neuroradiol. (2014) 35:513–8. doi: 10.3174/ajnr.A3694
25. Brodsky MC, Vaphiades M. Magnetic resonance imaging in pseudotumor cerebri. Ophthalmology. (1998) 105:1686–93. doi: 10.1016/S0161-6420(98)99039-X
26. Chang RO, Marshall BK, Yahyavi N, Sharma A, Huecker J, Gordon MO, et al. Neuroimaging features of idiopathic intracranial hypertension persist after resolution of papilloedema. Neuroophthalmology. (2016) 40:165–70. doi: 10.1080/01658107.2016.1179767
27. Yilmaz A, Gok M, Altas H, Yildirim T, Kaygisiz S, Isik HS. Retinal nerve fibre and ganglion cell inner plexiform layer analysis by optical coherence tomography in asymptomatic empty sella patients. Int J Neurosci. (2020) 130:45–51. doi: 10.1080/00207454.2019.1660328
28. Schmidt C, Wiener E, Lüdemann L, Kunte H, Kreutz KM, Becker N, et al. Does IIH alter brain microstructures? - A DTI-based approach. Headache. (2017) 57:746–55. doi: 10.1111/head.13039
29. Patterson DF, Ho ML, Leavitt JA, Smischney NJ, Hocker SE, Wijdicks EF, et al. Comparison of Ocular Ultrasonography and Magnetic Resonance Imaging for Detection of Increased Intracranial Pressure. Front Neurol. (2018) 9:278. doi: 10.3389/fneur.2018.00278
30. Hoffmann J, Kreutz KM, Csapó-Schmidt C, Becker N, Kunte H, Fekonja LS, et al. The effect of CSF drain on the optic nerve in idiopathic intracranial hypertension. J Headac Pain. (2019) 20:59. doi: 10.1186/s10194-019-1004-1
31. Razek AAKA, Batouty N, Fathy W, Bassiouny R. Diffusion tensor imaging of the optic disc in idiopathic intracranial hypertension. Neuroradiology. (2018) 60:1159–66. doi: 10.1007/s00234-018-2078-1
32. Lublinsky S, Kesler A, Friedman A, Horev A, Shelef I. Quantifying response to intracranial pressure normalization in idiopathic intracranial hypertension via dynamic neuroimaging. J Magn Reson Imaging. (2018) 47:913–27. doi: 10.1002/jmri.25857
33. Ahmed RM, Wilkinson M, Parker GD, Thurtell MJ, Macdonald J, McCluskey PJ, et al. Transverse sinus stenting for idiopathic intracranial hypertension: a review of 52 patients and of model predictions. AJNR Am J Neuroradiol. (2011) 32:1408–14. doi: 10.3174/ajnr.A2575
34. Fercher AF, Hitzenberger CK, Drexler W, Kamp G, Sattmann H. In vivo optical coherence tomography. Am J Ophthalmol. (1993) 116:113–4. doi: 10.1016/S0002-9394(14)71762-3
35. Scott CJ, Kardon RH, Lee AG, Frisen L, Wall M. Diagnosis and grading of papilledema in patients with raised intracranial pressure using optical coherence tomography vs clinical expert assessment using a clinical staging scale. Arch Ophthalmol. (2010) 128:705–11. doi: 10.1001/archophthalmol.2010.94
36. Kupersmith MJ, Sibony P, Mandel G, Durbin M, Kardon RH. Optical coherence tomography of the swollen optic nerve head: deformation of the peripapillary retinal pigment epithelium layer in papilledema. Invest Ophthalmol Vis Sci. (2011) 52:6558–64. doi: 10.1167/iovs.10-6782
37. Sheils CR, Fischer WS, Hollar RA, Blanchard LM, Feldon SE. The relationship between optic disc volume, area, and frisen score in patients with idiopathic intracranial hypertension. Am J Ophthalmol. (2018) 195:101–9. doi: 10.1016/j.ajo.2018.07.032
38. Rebolleda G, Muñoz-Negrete FJ. Follow-up of mild papilledema in idiopathic intracranial hypertension with optical coherence tomography. Invest Ophthalmol Vis Sci. (2009) 50:5197–200. doi: 10.1167/iovs.08-2528
39. Skau M, Yri H, Sander B, Gerds TA, Milea D, Jensen R. Diagnostic value of optical coherence tomography for intracranial pressure in idiopathic intracranial hypertension. Graefes Arch Clin Exp Ophthalmol. (2013) 251:567–74. doi: 10.1007/s00417-012-2039-z
40. Pardon LP, Cheng H, Tang RA, Saenz R, Frishman LJ, Patel NB. Custom optical coherence tomography parameters for distinguishing papilledema from pseudopapilledema. Optom Vis Sci. (2019) 96:599–608. doi: 10.1097/OPX.0000000000001408
41. Patel MD, Malhotra K, Shirazi Z, Moss HE. Methods for quantifying optic disc volume and peripapillary deflection volume using radial optical coherence tomography scans and association with intracranial pressure. Front Neurol. (2019) 10:798. doi: 10.3389/fneur.2019.00798
42. Kaufhold F, Kadas EM, Schmidt C, Kunte H, Hoffmann J, Zimmermann H, et al. Optic nerve head quantification in idiopathic intracranial hypertension by spectral domain OCT. PLoS ONE. (2012) 7:e36965. doi: 10.1371/journal.pone.0036965
43. Wang JK, Kardon RH, Ledolter J, Sibony PA, Kupersmith MJ, Garvin MK. Peripapillary retinal pigment epithelium layer shape changes from acetazolamide treatment in the idiopathic intracranial hypertension treatment trial. Invest Ophthalmol Vis Sci. (2017) 58:2554–65. doi: 10.1167/iovs.16-21089
44. Albrecht P, Blasberg C, Ringelstein M, Muller AK, Finis D, Guthoff R, et al. Optical coherence tomography for the diagnosis and monitoring of idiopathic intracranial hypertension. J Neurol. (2017) 264:1370–80. doi: 10.1007/s00415-017-8532-x
45. Patel MD, Khushzad F, Moss HE. Comparison of cross sectional optical coherence tomography images of elevated optic nerve heads across acquisition devices and scan protocols. Eye Vis (Lond). (2018) 5:17. doi: 10.1186/s40662-018-0112-3
46. Auinger P, Durbin M, Feldon S, Garvin M, Kardon R, Keltner J, et al. Papilledema outcomes from the optical coherence tomography substudy of the idiopathic intracranial hypertension treatment trial. Ophthalmology. (2015) 122:1939–45.e2. doi: 10.1016/j.ophtha.2015.06.003
47. Athappilly G, Garcia-Basterra I, Machado-Miller F, Hedges TR, Mendoza-Santiesteban C, Vuong L. Ganglion cell complex analysis as a potential indicator of early neuronal loss in idiopathic intracranial hypertension. Neuroophthalmology. (2019) 43:10–7. doi: 10.1080/01658107.2018.1476558
48. Sibony P, Kupersmith MJ, Honkanen R, Rohlf FJ, Torab-Parhiz A. Effects of lowering cerebrospinal fluid pressure on the shape of the peripapillary retina in intracranial hypertension. Invest Ophthalmol Vis Sci. (2014) 55:8223–31. doi: 10.1167/iovs.14-15298
49. Malhotra K, Patel MD, Shirazi Z, Moss HE. Association between peripapillary bruch's membrane shape and intracranial pressure: effect of image acquisition pattern and image analysis method, a preliminary study. Front Neurol. (2018) 9:1137. doi: 10.3389/fneur.2018.01137
50. Gampa A, Vangipuram G, Shirazi Z, Moss HE. Quantitative association between peripapillary bruch's membrane shape and intracranial pressure. Invest Ophthalmol Vis Sci. (2017) 58:2739–45. doi: 10.1167/iovs.17-21592
51. Fard MA, Fakhree S, Abdi P, Hassanpoor N, Subramanian PS. Quantification of peripapillary total retinal volume in pseudopapilledema and mild papilledema using spectral-domain optical coherence tomography. Am J Ophthalmol. (2014) 158:136–43. doi: 10.1016/j.ajo.2014.03.008
52. Traber GL, Weber KP, Sabah M, Keane PA, Plant GT. Enhanced depth imaging optical coherence tomography of optic nerve head drusen: a comparison of cases with and without visual field loss. Ophthalmology. (2017) 124:66–73. doi: 10.1016/j.ophtha.2016.09.022
53. Malmqvist L, Bursztyn L, Costello F, Digre K, Fraser JA, Fraser C, et al. The optic disc drusen studies consortium recommendations for diagnosis of optic disc drusen using optical coherence tomography. J Neuro-Ophthalmol. (2018) 38:299–307. doi: 10.1097/WNO.0000000000000585
54. Marzoli SB, Ciasca P, Curone M, Cammarata G, Melzi L, Criscuoli A, et al. Quantitative analysis of optic nerve damage in idiopathic intracranial hypertension (IIH) at diagnosis. Neurol Sci. (2013) 34 Suppl 1:S143–5. doi: 10.1007/s10072-013-1373-1
55. Sibony PA, Kupersmith MJ, Feldon SE, Wang J-K, Garvin M, Trial OSGftNIIHT. Retinal and choroidal folds in papilledema. Invest Ophthalmol Vis Sci. (2015) 56:5670–80. doi: 10.1167/iovs.15-17459
56. Kupersmith MJ, Sibony PA, Feldon SE, Wang JK, Garvin M, Kardon R. The effect of treatment of idiopathic intracranial hypertension on prevalence of retinal and choroidal folds. Am J Ophthalmol. (2017) 176:77–86. doi: 10.1016/j.ajo.2016.12.017
57. Moss HE, Vangipuram G, Shirazi Z, Shahidi M. Retinal vessel diameters change within 1 hour of intracranial pressure lowering. Transl Vis Sci Technol. (2018) 7:6. doi: 10.1167/tvst.7.2.6
58. Rougier M-B, Le Goff M, Korobelnik J-F. Optical coherence tomography angiography at the acute phase of optic disc edema. Eye Vis. (2018) 5:15. doi: 10.1186/s40662-018-0109-y
59. McHugh JA, D'Antona L, Toma AK, Bremner FD. Spontaneous venous pulsations detected with infrared videography. J Neuroophthalmol. (2019) 40:174–77. doi: 10.1097/WNO.0000000000000815
60. D'Antona L, McHugh JA, Ricciardi F, Thorne LW, Matharu MS, Watkins LD, et al. Association of intracranial pressure and spontaneous retinal venous pulsation. JAMA Neurology. (2019) 76:1502–5. doi: 10.1001/jamaneurol.2019.2935
Keywords: headache, idiopathic intracranial hypertension (iih), neuroimaging, optical coherence tomography, pain
Citation: Moreno-Ajona D, McHugh JA and Hoffmann J (2020) An Update on Imaging in Idiopathic Intracranial Hypertension. Front. Neurol. 11:453. doi: 10.3389/fneur.2020.00453
Received: 24 December 2019; Accepted: 28 April 2020;
Published: 10 June 2020.
Edited by:
Roberta Messina, Vita-Salute San Raffaele University, ItalyCopyright © 2020 Moreno-Ajona, McHugh and Hoffmann. This is an open-access article distributed under the terms of the Creative Commons Attribution License (CC BY). The use, distribution or reproduction in other forums is permitted, provided the original author(s) and the copyright owner(s) are credited and that the original publication in this journal is cited, in accordance with accepted academic practice. No use, distribution or reproduction is permitted which does not comply with these terms.
*Correspondence: Jan Hoffmann, amFuLmhvZmZtYW5uQGtjbC5hYy51aw==