- 1Department of Neurodegenerative Disorders Research, Institute of Biomedical Sciences, Graduate School of Medical Sciences, Tokushima University, Tokushima, Japan
- 2Department of Neurobiology and Therapeutics, Institute of Biomedical Sciences, Graduate School of Pharmaceutical Sciences, Tokushima University, Tokushima, Japan
- 3Parkinson's Disease and Dystonia Research Center, Tokushima University Hospital, Tokushima, Japan
Although the administration of dopamine precursor levodopa remains as the mainstay for the treatment of Parkinson's disease, long-term exposure to levodopa often causes a disabling complication, referred to as levodopa-induced dyskinesias. Therefore, the development of new therapeutic interventions to dampen levodopa-induced dyskinesias and parkinsonian motor deficits is needed in the treatment of Parkinson's disease. Intracerebral brain infusion has the merit of being able to specifically deliver any drug into any brain part. By using an intracerebral infusion system equipped with implantable, programmable, and refillable pumps, we show herein that continuous intrastriatal administration of memantine (MMT), which is a non-competitive N-methyl-D-aspartate receptor antagonist, attenuates levodopa-induced dyskinesias and parkinsonian signs in 6-hydroxydopamine-lesioned hemiparkinsonian mice that received daily levodopa treatment. Corroborating the general thought that overactivation of the striatal N-methyl-D-aspartate receptor function might generate levodopa-induced dyskinesias and parkinsonism, our results suggest that a continuous intrastriatal MMT infusion can be beneficial for the management of Parkinson's disease with levodopa-induced dyskinesias. Our study also provides indications for the prototypic use of pharmacological deep-brain modulation through intracerebral infusion systems for treating medically intractable movement disorders.
Introduction
The administration of the dopamine precursor levodopa is a powerful tool for treating Parkinson's disease; however long-term exposure to levodopa often provokes abnormal involuntary movements (AIMs), referred to as levodopa-induced dyskinesias (LIDs) (1). LID is a major cause of disability that occurs in >80% of Parkinson's disease patients after 5 years of daily levodopa treatment (2). Although electrical deep brain stimulation can produce a striking impact on Parkinson's disease with LIDs, its therapeutic efficacy for the quality of life has usually fallen below preoperative levels during long-term follow-up (3). Therefore, the development of alternative or adjunct therapeutic interventions for overcoming both LIDs and “OFF”-period symptoms is a pressing challenge in the treatment of Parkinson's disease.
Parkinsonian symptoms result from striatal dysfunction caused by an imbalance between dopamine and glutamate transmissions (4, 5). The dysregulation of corticostriatal glutamatergic inputs leading to maladaptive synaptic plasticity in the striatum is also a well-documented process underlying LID genesis (6). At the striatal postsynaptic level, the interactions of D1-type dopamine receptors with glutamate receptors, particularly N-methyl-D-aspartate (NMDA) receptors and metabotropic glutamate receptors, appear to be especially relevant (6–9). Accordingly, oral administration of compounds targeting NMDA receptors or specific subtypes of metabotropic glutamate receptors (mGluR4 and mGluR5) has been challenged in clinical practice (8). Currently, the oral administration of amantadine, which is a non-competitive NMDA receptor antagonist, represents the only recommended adjunct therapy for reducing LIDs in patients with Parkinson's disease (9). However, its low therapeutic efficacy and various adverse effects have limited its clinical use.
Applying the intracerebral brain infusion (iCBI) technique for brain disorder treatment has a long history in experimental and clinical studies on Parkinson's disease [for examples, see (10–12)]. Interestingly, Whone et al. (13) recently reported the clinical use of a new and sophisticated drug delivery system for intermittent intraputamenal infusion of the glial cell line-derived neurotrophic factor in patients with Parkinson's disease. The present study was undertaken to examine if the continuous intrastriatal infusion of memantine (MMT), which is a non-competitive NMDA receptor antagonist and clinically used for the treatment of dementia (14), through an iCBI system could attenuate levodopa-induced AIMs and parkinsonian signs in mice with hemiparkinsonism caused by 6-hydroxydopamine (6-OHDA) lesions. Our results suggest that continuous intrastriatal MMT infusion may represent a new therapeutic tool in treating intractable LIDs associated with Parkinson's disease.
Materials and Methods
Animals
Adult male C57BL/6 mice aged 8–9 weeks (Nihon SLC, Hamamatsu, Japan) were used. The mice were housed in a controlled environment (25 ± 1°C, 50 ± 10% humidity, and 12-h light/dark cycle) with access to food and tap water ad libitum. All experimental procedures were approved by the Institutional Animal Care and Use Committees of Tokushima University, Japan.
Nigrostriatal 6-OHDA Lesion
Under anesthesia with N2O and isoflurane (Sigma-Aldrich, St. Louis, MO, USA), the mice received an intraperitoneal (i.p.) injection of desipramine hydrochloride (an inhibitor of noradrenaline transporter, 1 mg/kg dissolved in 0.9 % saline, Wako, Osaka, Japan) and pargyline hydrochloride (an inhibitor of monoamine oxidase, 0.2 mg/kg dissolved in 0.9% saline, Sigma-Aldrich, St. Louis, MO, USA). We used these inhibitors for uptaking 6-OHDA selectively in brain dopaminergic neurons. After 15 min, they received stereotaxic injections of 6-OHDA HCl (Sigma-Aldrich; 3 μg dissolved in 0.2 μl of 0.9% saline containing 0.2% ascorbic acid) targeted into the medial forebrain bundle (MFB) on the right side. The target coordinates (AP = −1.2, L = +1.1, DV = +5.0) were according to the mouse brain atlas (15). After a recovery of 2 weeks, the 6-OHDA-lesioned mice, which showed over 80% of the spontaneous ipsilateral rotation and apomorphine (0.5 mg/kg; Sigma-Aldrich) injection-induced contralateral rotation (rotation check) at day 0 (Figure 1A), were processed for further studies.
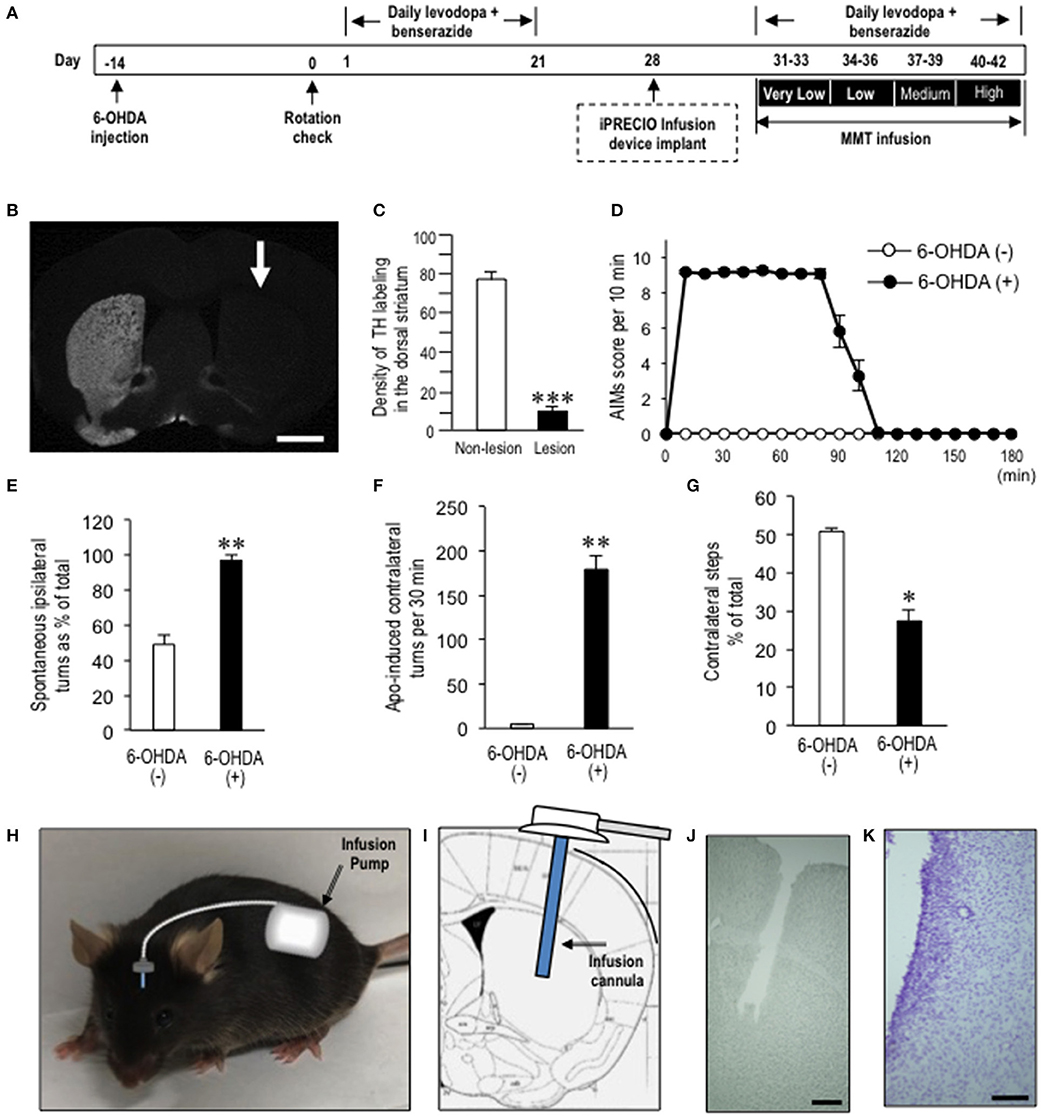
Figure 1. Generation of dyskinetic mice for the intracerebral brain infusion (iCBI) experiments. (A) Timeline of experimentations (see “Materials and Methods” section). Dyskinetic mice were implanted with programmable intracerebral brain infusion (iCBI) systems equipped with iPRECIOTM micro infusion pumps at day 28. After a recovery of 3 days, they restarted receiving daily levodopa/benserazide treatments for the next 12 days. Simultaneously, MMT-treated dyskinetic mice received continuous intrastriatal infusion of MMT 0.28 ng/μl at a flow rate of 1.0 μl/h for the first 3 days (days 31–33), MMT 1.12 ng/μl at a flow rate of 1.0 μl/h for the next 3 days (days 34–36), MMT 4.48 ng/μl at a flow rate of 1.0 μl/h for the next 3 days (days 37–39), and MMT 17.93 ng/μl a flow rate of 1.0 μl/h for the last 3 days (days 40–42). In parallel, PBS-treated dyskinetic mice received PBS under the same protocol. AIM scoring tests for MMT- or PBS-treated mice were done at days 33, 36, 39, and 42. (B) A representative photomicrograph of the striatum stained for tyrosine hydroxylase on the non-lesioned and lesioned (arrow) sides in mice with 6-OHDA-induced lesions. Scale bar = 2 mm. (C) Quantification of the mean staining intensity for tyrosine hydroxylase (TH) in the dorsolateral striatum on the non-lesioned (non-lesion) and lesioned (lesion) sides. In the Y-axis, the averaged pixel intensities in the measured area were expressed with arbitrary units. Data are means ± SEM (n = 7). Paired two-tailed t-test: ***P < 0.001 vs. non-lesion. (D) Time course of the abnormal involuntary movements (AIMs) scored every 10 min over a period of 180 min after the last levodopa administration in naïve mice and 6-hydroxydopamine (6-OHDA)-lesioned mice. Data are means ± SEM at each time point (naïve mice, n = 10; 6-OHDA-lesioned mice, n = 14). (E) Spontaneous rotations ipsilateral to 6-OHDA-lesioned side percent of total in mice with or without 6-OHDA lesions. 6-OHDA (–) (n = 6); 6-OHDA (+) (n = 6). Data are means ± SEM. **P < 0.01 vs. 6-OHDA (–); Mann-Whitney U-test. (F) Counts of apomorphine-induced contralateral turns per 30 min in mice with or without 6-OHDA lesions. 6-OHDA (–) (n = 6); 6-OHDA (+) (n = 6). Data are means ± SEM. **P < 0.01 vs. naïve controls; Mann-Whitney U-test. (G) Counts of hind limb steps. Steps of the contralateral hind limb as % of the total count in the naïve controls (n = 6) and Hemi-PD mice (n = 6). Data are means ± SEM. *P < 0.05 vs. naïve; Mann-Whitney U-test. (H–K) Schematic representation of the iCBI system, in which the pump and tubing were implanted completely under the skin (H). The infusion cannula implanted into the dopamine-depleted striatum (I), together with the image of striatum after fixation and removal of the cannula (J), and with a Nissl-stained image (K). Scale bars show 0.5 mm in (J) and 100 μm in (K).
Levodopa/Benserazide Treatments
Mice received intraperitoneal injections of levodopa (Sigma-Aldrich; 15 mg/kg) dissolved in 0.9% saline containing 0.5% carboxymethyl cellulose at 20 min after i.p. injections of benserazide HCl (Sigma-Aldrich; 12.5 mg/kg) dissolved in 0.9% saline.
Assessment of AIMs
AIM scoring was performed after the last levodopa injection for 1 min every 10 min over a period of 150–180 min, as per our previous report (16). For the evaluation, each mouse was placed in a 12-cm-diameter glass cylinder. The following subtypes of AIMs were examined: axial (twisted posturing of the neck and the upper body toward the contralateral side), forelimb (jerky movements of the contralateral forelimb, and/or grabbing movement of the contralateral paw), and orolingual (jaw movements and tongue protrusion toward the contralateral side). Each subtype was scored as follows: 0: absent; 1: occasional; 2: frequent; 3: continuous; and 4: continuous, not interrupted by sensory stimuli.
Assessment of the Rotational Behaviors
Spontaneous rotational behaviors were assessed by measuring systemic turns ipsilateral to the 6-OHDA lesion (17) 6 h after the last levodopa administration. Apomorphine-induced rotations contralateral to the 6-OHDA lesion were also measured 30 min after the apomorphine (0.5 mg/kg) administration.
Assessment of Hind Limb Stepping
Video-based analysis of the hind limb stepping in mice was performed as reported previously (18). Mice were placed in 600-ml beaker, and the spontaneous steps of their hind limbs were video-recorded from the bottom for 5 min. Each hind limb was counted with a playback speed slowed to 0.3–0.5× using VLC media player. Total hind limb steps were summed for both the ipsilateral (intact) and contralateral (impaired) to the lesion side, and the number of contralateral steps was calculated as % of the total.
Placement of iCBI Devices and MMT Administration
The iCBI devices equipped with the iPRECIOTM programmable micro infusion pumps (Model SMP-300, Primetech Co., Tokyo, Japan) were implanted in the mice under anesthesia with isoflurane (Sigma-Aldrich). The infusion cannulas were implanted into the dopamine-depleted striatum at the stereotaxic coordinates: AP = +0.3; L = +2.3; and DV = +3.0. MMT HCl (Wako) was dissolved in 0.01 M phosphate-buffered saline at pH 7.2 (PBS), then applied for the infusion pumps. The used concentrations of MMT were determined by the solubility to PBS of MMT.
Immunohistochemistry and Digital Imaging
Immunostaining procedures and microscopic imaging were previously described (19). The mice (n = 7) were intraperitoneally injected with a lethal dose of pentobarbital (Sigma-Aldrich), and transcardially perfused with cold saline, followed by cold 4% paraformaldehyde in 0.1 M phosphate buffer (pH 7.2). The brains were removed, postfixed overnight in the same fixative at 4°C, and stored in a 10–30% sucrose gradient in 0.01 M phosphate buffered saline (pH 7.2) at 4°C for cryoprotection. Sections were cut on a cryostat at 16-μm thickness and stored in PBS containing 0.05% NaN3 until use. After blocking endogenous peroxidase activity, the free-floating sections were incubated in PBS containing 3% bovine serum albumin (PBS-BSA) for 60 min. They were then incubated in PBS-BSA with a rabbit polyclonal antibody tyrosine hydroxylase (TH) (1:200,000) (19) for 18 h. The bound antibody was detected using the Histofine Simple Stain Kit (Nichirei, Tokyo, Japan) and the tyramide signal amplification system with Cyanine3 (Perkin Elmer, Shelton, CT, USA). For Nissl staining, sections were stained with 0.1% cresyl violet acetate (Wako) dissolved in distilled water. Digital microscopy images were captured using an Olympus BX51 microscope (Olympus, Tokyo, Japan), imported into Adobe Photoshop CS4, and digitally processed for adjustments of contrast, brightness, and color balance.
Statistical Analysis
All analyses were expressed as group mean ± SEM. We used paired two-tailed t-test or Mann-Whitney U-test for two-group comparisons. Multiple comparisons were analyzed using analyses of variance (ANOVA), followed by Bonferroni's post-hoc tests for pairwise comparisons. Statistical analyses were performed using GraphPad Prism 7 Software (GraphPad Software Inc., San Diego, CA, USA). P < 0.05 were considered statistically significant.
Results
Generation and Characterization of a Dyskinetic Mouse Model
The hemiparkinsonian mice were produced by unilateral injections of 6-OHDA targeted into the right MFB (Figure 1A). TH immunostaining revealed severe loss of striatal dopaminergic afferents in the mice with 6-OHDA lesions (Figure 1B). The quantifications revealed a >80% reduction of TH immunoreactivity in the dorsal striatum on the 6-OHDA-lesioned side compared to the non-lesioned side (Figure 1C; P < 0.001, paired two-tailed t-test). After a period of 3 weeks of levodopa/benserazide treatment, the AIM scoring tests revealed that all 6-OHDA-lesioned mice manifested LIDs. Figure 1D shows the time-sequential changes of AIMs in the dyskinetic mice, which were maximal 10–60 min after levodopa administration (i.e., “ON” period) and almost disappeared after 120 min.
Meanwhile, these dyskinetic mice also showed parkinsonian signs 6 h after levodopa administration (i.e., “OFF” period). The percentages of spontaneous rotations ipsilateral to the 6-OHDA-lesioned side (or right side) relative to the total rotations were significantly increased in mice with 6-OHDA lesions compared to mice without 6-OHDA lesions (Figure 1E; P < 0.01, Mann-Whitney U-test). Apomorphine-induced rotations contralateral to the 6-OHDA-lesioned side were also significantly increased in mice with 6-OHDA lesions as compared to mice without 6-OHDA lesions (Figure 1F; P < 0.01, Mann-Whitney U-test). Moreover, spontaneous use of hind limbs contralateral to the 6-OHDA-lesioned side was significantly decreased in mice with 6-OHDA lesions compared to mice without 6-OHDA lesions (Figure 1G; P < 0.05, Mann-Whitney U-test).
Intrastriatal MMT Infusion Attenuates LIDs in 6-OHDA-Lesioned Mice
To determine if intrastriatal MMT infusion could reduce LIDs in the dyskinetic mice, we used the programmable and refillable iCBI systems equipped with iPRECIOTM micro infusion pumps, which make it possible to perform quantitative pharmacology in single animals (20, 21). The iCBI devices (Figure 1H) with the infusion cannulas implanted into the dopamine-depleted striatum (Figures 1I–K) were placed at day 28 under the experimental protocols (Figure 1A). No severe damage of the tissue except for the cell clusters around the trace of infusion cannula, which may represent gliosis (Figure 1K), was observed. The levodopa/benserazide treatments were then restarted at day 31. Simultaneously, the MMT-treated dyskinetic mice received continuous intrastriatal infusion of MMT 0.28 ng/μl at a flow rate of 1.0 μl/h for the first 3 days (days 31–33), MMT 1.12 ng/μl at a flow rate of 1.0 μl/h for the next 3 days (days 34–36), MMT 4.48 ng/μl at a flow rate of 1.0 μl/h for the next 3 days (days 37–39), and MMT 17.93 ng/μl at a flow rate of 1.0 μl/h for the last 3 days (days 40–42). In parallel, the PBS-treated dyskinetic mice received PBS under the same protocol. Accordingly, the AIM scoring tests for the MMT- or PBS-treated mice were done at days 33 (Figure 2A), 36 (Figure 2B), 39 (Figure 2C), and 42 (Figure 2D). The two-way ANOVA on the total AIMs scores (Figure 2E) revealed a significant effect of the MMT infusion treatment [F(group×dose)3,48 = 5.856, P = 0.0017]. Compared to PBS infusion, MMT infusion significantly reduced AIMs at day 42 (Bonferroni post-hoc test: PBS day 42 vs. MMT day 42, ***P < 0.001). Moreover, a significant difference in the severity of AIMs was found at days 33, 36, and 42 (Bonferroni post-hoc test: MMT day 33 vs. MMT day 42, ##P < 0.01; MMT day 36 vs. MMT day 42, $$P < 0.01). Thus, intrastriatal MMT infusion significantly attenuated levodopa-induced AIMs in a dose-dependent manner.
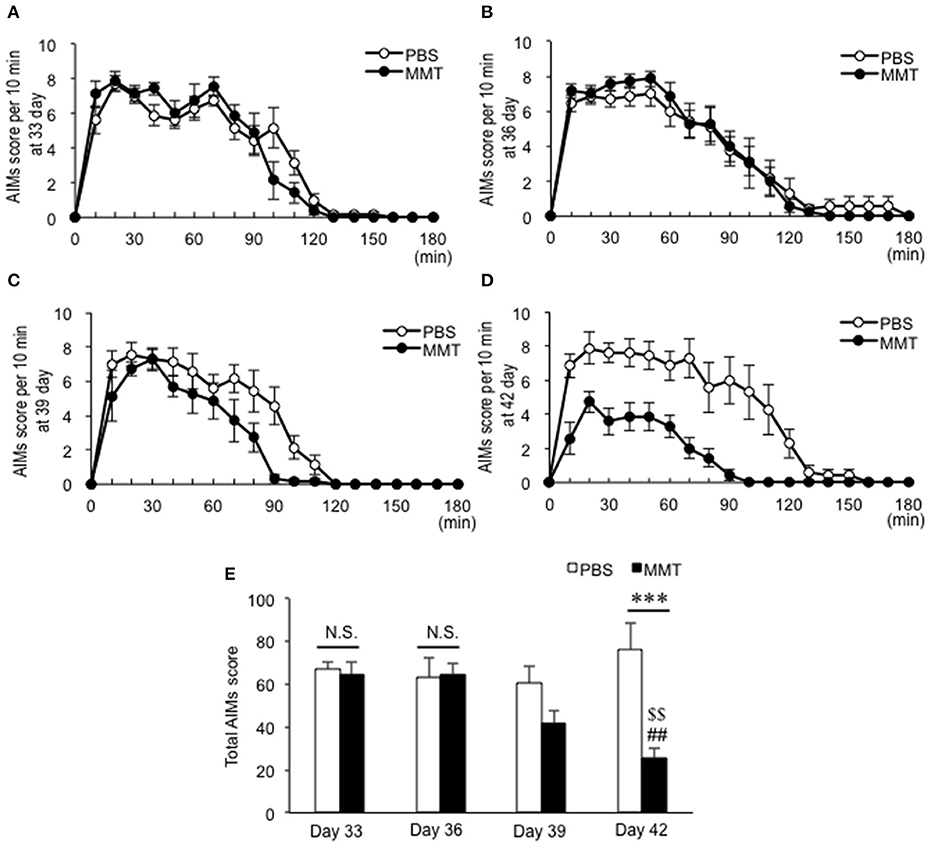
Figure 2. Effects of intrastriatal memantine (MMT) infusion on levodopa-induced abnormal involuntary movements (AIMs) in dyskinetic mice. (A–D) Time-sequential changes in the AIM scores every 10 min over a period of 180 min after the last levodopa administration in mice with intrastriatal infusion of PBS (n = 7) or MMT (n = 7) at day 33 (B), day 36 (C), day 39 (D), and day 42 (E). (E) Total AIMs score in mice with intrastriatal infusion of PBS or MMT at days 33, 36, 39, and 42. Data are means ± SEM. A significant effect of the MMT infusion treatment is found [two-way ANOVA: F(group×dose)3,48 = 5.856, P = 0.0017]. Bonferroni post-hoc test: PBS day 33 vs. MMT day 33, not significant (N.S.); PBS day 36 vs. MMT day 36, N.S.; and PBS day 42 vs. MMT day 42, ***P < 0.001. Significant differences in the severity of AIMs at days 33, 36, and 42 are found (Bonferroni post-hoc test: MMT day 33 vs. MMT day 42, ##P < 0.01; MMT day 36 vs. MMT day 42, $$P < 0.01).
Intrastriatal MMT Infusion Attenuates Parkinsonian Signs in 6-OHDA-Lesioned Mice
We next determined if intrastriatal MMT infusion could also affect parkinsonian signs in the dyskinetic mice. For this purpose, behavioral tests for hemiparkinsonism were done 6 h after the last levodopa/benserazide treatments at days 31 and 42 (Figure 1A). Notably, the two-way ANOVA on the percentage of the spontaneous ipsilateral rotations relative to the total rotations (Figure 3A) revealed a significant effect of the MMT infusion treatment [F (group×dose)1,24 = 10.25, P = 0.0038]. MMT infusion significantly normalized the rotational asymmetry at day 42 (Bonferroni post-hoc test: MMT day 31 vs. MMT day 42, **P < 0.01; PBS day 42 vs. MMT day 42, ###P < 0.001). The two-way ANOVA on the counts of apomorphine-induced contralateral turns (Figure 3B) also showed a significant effect of the MMT infusion treatment [F (group×dose)1,20 = 9.608, P = 0.0056]. MMT infusion significantly reversed the apomorphine-induced rotational behaviors at day 42 (Bonferroni post-hoc test: MMT day 31 vs. MMT day 42, *P < 0.05; PBS day 42 vs. MMT day 42, ##P < 0.01). The two-way ANOVA on the counts of spontaneous contralateral hind limb stepping (Figure 3C) also showed a significant effect of the MMT infusion treatment [F(group×dose)1,16 = 7.891, P = 0.0126]. MMT infusion significantly normalized the % of contralateral hind limb steps at day 42 (Bonferroni post-hoc test: MMT day 31 vs. MMT day 42, ***P < 0.001; PBS day 42 vs. MMT day 42, #P < 0.05). There was no significant difference between PBS and MMT groups in the total number of spontaneous turns (Figure 3D) nor of hind limb steps (Figure 3E). Thus, MMT infusion significantly improved not only AIMs but also parkinsonian signs in dyskinetic mice.
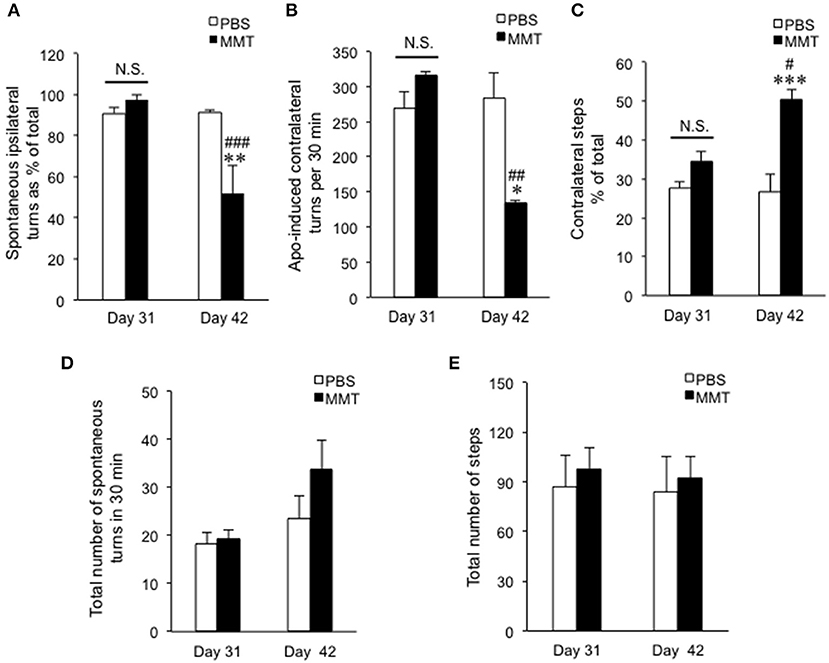
Figure 3. Effects of intrastriatal memantine (MMT) infusion on parkinsonian signs in the dyskinetic mice. (A) Spontaneous rotations ipsilateral to the 6-OHDA-lesioned side percent of the total in the groups of PBS infusion at day 31 (PBS day 31, n = 7), MMT infusion at day 31 (MMT day 31, n = 7), PBS infusion at day 42 (PBS day 42, n = 7), and MMT infusion at day 42 (MMT day 42, n = 7). Data are means ± SEM. A significant effect of MMT infusion [two-way ANOVA: F(group×dose)1,24 = 10.25, P = 0.0038] is observed. Bonferroni post-hoc test: not significant (N.S.); MMT day 31 vs. MMT day 42, **P < 0.01; PBS day 42 vs. MMT day 42, ###P < 0.001. (B) Counts of apomorphine-induced contralateral turns per 30 min in the groups of PBS day 31 (n = 6), MMT day 31 (n = 6), PBS day 42 (n = 6), and MMT day 42 (n = 6). Data are means ± SEM. A significant effect of MMT infusion [two-way ANOVA: F(group×dose)1,20 = 9.608, P = 0.0056] is found. Bonferroni post-hoc test: not significant (N.S.); MMT day 31 vs. MMT day 42, *P < 0.05; PBS day 42 vs. MMT day 42, ###P < 0.001. (C) Spontaneous contralateral hind limb steps as % of the total in PBS day 31 (n = 5), MMT day 31 (n = 5), PBS day 42 (n = 5), and MMT day 42 (n = 5). Data are means ± SEM. A significant effect of MMT infusion [two-way ANOVA: F(group×dose)1,16 = 7.891, P = 0.0126] is found. Bonferroni post-hoc test: not significant (N.S.); MMT day 31 vs. MMT day 42, ***P < 0.001; PBS day 42 vs. MMT day 42, #P < 0.05. (D) Total number of turns in spontaneous rotations summed with both ipsi- and contra-lateral directions. Data are means ± SEM. No significance was observed [two-way ANOVA: F(group×dose)1,24 = 6.507, P = 0.0434]. (E) Total number of steps summed with both ipsi- and contra-lateral hind limbs. Data are means ± SEM. No significance was observed [two-way ANOVA: F(group×dose)1,16 = 0.3957, P = 0.5634].
Discussion
The long-term and repeated pharmacological overactivation of striatal dopamine receptors, which leads to an altered glutamatergic transmission at several nodes of the corticobasal ganglia circuit, is generally viewed as the principal cause for LIDs in patients with advanced Parkinson's disease. We provide herein experimental evidence that intrastriatal MMT infusion exerts therapeutic effects on both levodopa-induced AIMs and parkinsonian signs in mice with hemiparkinsonism caused by 6-OHDA. Although there is a difficulty to distinguish improvement of akinesia from induction of dyskinesia in rodent models of Parkinson's disease, and further studies using primate models are essentially required, our finding in this study corroborates the general thought that the overactivation of striatal NMDA receptors, which mediate a slow, Ca2+-permeable component of excitatory synaptic transmission (22), might generate LIDs and “OFF”-period motor deficits in Parkinson's disease (4, 5, 7, 23). Indeed, clinical observations have shown the positive therapeutic potential of the NMDA receptor antagonists amantadine (24) and MMT (25–28) in the treatment of LIDs and parkinsonian symptoms in patients with Parkinson's disease. However, the oral administration of NMDA antagonists, particularly amantadine, causes considerable noncerebral side effects, such as cardiovascular, gastrointestinal, and skin reactions (8, 26). It also incites cerebral adverse effects of nonstriatal origin, such as psychosis, hallucinations, and learning and memory deficit, because of the wide distribution of NMDA receptors in the brain (29). Considering the structural similarity between amantadine and MMT, these central and systemic side effects limit the usable dose of NMDA antagonists and reduce their clinical impact (30). A recent report of meta-analysis comparing multiple medicines in Alzheimer's disease patients showed that oral administration of MMT is safe enough (31). However, it is not approved for the treatment of Parkinson's disease patients yet. In a previous study, although single injection of MMT or amantadine did reduce LIDs in the 6-OHDA-lesioned rats, this antidyskinetic effect has disappeared by subchronic administration for a few days (32). It indicates that drug tolerance to MMT or amantadine developed during the treatment. A clinical study also reported that the beneficial effect of amantadine on dyskinesia was lost within 8 months (33). The underlying mechanism of MMT or amantadine tolerance, including whether it is caused by central or peripheral reasons, is still unknown. Given that the drug tolerance by repeated oral or systemic administration limits the therapeutic efficacy, it is worth developing an alternative drug delivery system, such as iCBI, despite of the risks, such as complications accompanying physical invasion.
The iCBI system makes it possible to deliver appropriately adjusted doses of desired drugs into specific brain regions. Therefore, intrastriatal infusion of NMDA antagonists through the iCBI system may provide higher therapeutic efficacy while reducing the adverse side effects of their oral or systemic administration in the treatment of LIDs. For the issue of drug tolerance, longer iCBI treatment of NMDA antagonists than this study should be examined. With the hope that programmable iCBI devices with a clinical application can be developed, we suggest the pharmacological deep-brain modulation as an alternative therapeutic approach for the treatment of Parkinson's disease and, potentially, other movement disorders.
Data Availability Statement
The datasets generated for this study are available on request to the corresponding author.
Ethics Statement
The animal study was reviewed and approved by The Institutional Animal Care and Use Committees of Tokushima University, Japan.
Author Contributions
SG contributed to the conception and design of the study. MO, YZ, RT, JK, and SG contributed to the acquisition and analysis of data. MO, JK, and SG contributed to the drafting of a significant portion of the manuscript.
Funding
This work was supported in part by grants from the Ministry of Education, Culture, Sports, Science and Technology of Japan (grants-in-aid for Scientific Research Nos. 26430054 and 16k10788); Japan Agency for Medical Research and Development (AMED; No. 16ek0109182h0001); and the Research Cluster of Tokushima University (No. 1702004).
Conflict of Interest
The authors declare that the research was conducted in the absence of any commercial or financial relationships that could be construed as a potential conflict of interest.
References
1. Jenner P. Molecular mechanisms of L-DOPA-induced dyskinesia. Nat Rev Neurosci. (2008) 9:665–77. doi: 10.1038/nrn2471
2. Ahlskog JE, Muenter MD. Frequency of levodopa-related dyskinesias and motor fluctuations as estimated from the cumulative literature. Mov Disord. (2001) 16:448–58. doi: 10.1002/mds.1090
3. Limousin P, Foltynie T. Long-term outcomes of deep brain stimulation in Parkinson disease. Nat Rev Neurol. (2019) 15:234–42. doi: 10.1038/s41582-019-0145-9
4. Greengard P. The neurobiology of slow synaptic transmission. Science. (2001) 294:1024–30. doi: 10.1126/science.294.5544.1024
5. Brichta L, Greengard P, Flajolet M. Advances in the pharmacological treatment of Parkinson's disease: targeting neurotransmitter systems. Trends Neurosci. (2013) 36:543–54. doi: 10.1016/j.tins.2013.06.003
6. Picconi B, Hernández LF, Obeso JA, Calabresi P. Motor complications in Parkinson's disease: striatal molecular and electrophysiological mechanisms of dyskinesias. Mov Disord. (2018) 33:867–76. doi: 10.1002/mds.27261
7. Goto S. Striatal Gαolf/cAMP signal-dependent mechanism to generate levodopa-induced dyskinesia in Parkinson's disease. Front Cell Neurosci. (2017) 11:364. doi: 10.3389/fncel.2017.00364
8. Litim N, Morissette M, Di Paolo T. Metabotropic glutamate receptors as therapeutic targets in Parkinson's disease: an update from the last 5 years of research. Neuropharmacology. (2017) 115:166–79. doi: 10.1016/j.neuropharm.2016.03.036
9. Mellone M, Gardoni F. Glutamatergic mechanisms in L-DOPA-induced dyskinesia and therapeutic implications. J Neural Transm. (2018) 125:1225–36. doi: 10.1007/s00702-018-1846-8
10. Woiciechowsky C, Guilarte TR, May CH, Vesper J, Wagner HN Jr, Vogel S. Intrastriatal dopamine infusion reverses compensatory increases in D2-dopamine receptors in the 6-OHDA lesioned rat. Neurodegeneration. (1995) 4:161–9. doi: 10.1006/neur.1995.0020
11. Carta M, Lindgren HS, Lundblad M, Stancampiano R, Fadda F, Cenci MA. Role of striatal L-DOPA in the production of dyskinesia in 6-hydroxydopamine lesioned rats. J Neurochem. (2006) 96:1718–27. doi: 10.1111/j.1471-4159.2006.03696.x
12. Lang AE, Gill S, Patel NK, Lozano A, Nutt JG, Penn R, et al. Randomized controlled trial of intraputamenal glial cell line-derived neurotrophic factor infusion in Parkinson's disease. Ann Neurol. (2006) 59:459–66. doi: 10.1002/ana.20737
13. Whone A, Luz M, Boca M, Woolley M, Mooney L, Dharia S, et al. Randomized trial of intermittent intraputaminal glial cell line-derived neurotrophic factor in Parkinson's disease. Brain. (2019) 142:512–25. doi: 10.1093/brain/awz023
14. Parsons CG, Danysz W, Quack G. Memantine is a clinically well tolerated N-methyl-D-aspartate (NMDA) receptor antagonist–a review of preclinical data. Neuropharmacology. (1999) 38:735–67. doi: 10.1016/S0028-3908(99)00019-2
15. Paxinos G, Franklin KBJ. The Mouse Brain in Stereotaxic Coordinates, 2 ed. San Diego, CA: Academic Press (2001).
16. Morigaki R, Okita S, Goto S. Dopamine-induced changes in Gαolf protein levels in striatonigral and striatopallidal medium spiny neurons underlie the genesis of L-DOPA-induced dyskinesia in parkinsonian mice. Front Cell Neurosci. (2017) 11:26. doi: 10.3389/fncel.2017.00026
17. Boix J, Padel T, Paul G. A partial lesion model of Parkinson's disease in mice – characterization of a 6-OHDA-induced medial forebrain bundle lesion. Behav Brain Res. (2015) 284:196–206. doi: 10.1016/j.bbr.2015.01.053
18. Ogawa M, Zhou Y, Tsuji R, Goto S, Kasahara J. Video-based assessments of the hind limb stepping in a mouse model of hemi-parkinsonism. Neurosci Res. (2019). doi: 10.1016/j.neures.2019.05.002. [Epub ahead of print].
19. Morigaki R, Goto S. Putaminal mosaic visualized by tyrosine hydroxylase immunohistochemistry in the human neostriatum. Front Neuroanat. (2016) 10:34. doi: 10.3389/fnana.2016.00034
20. Abe C, Tashiro T, Tanaka K, Ogihara R, Morita H. A novel type of implantable and programmable infusion pump for small laboratory animals. J Pharmacol Toxicol Methods. (2009) 59:7–12. doi: 10.1016/j.vascn.2008.09.002
21. Tan T, Watts SW, Davis RP. Drug delivery: enabling technology for drug discovery and development. iPRECIO micro infusion pump: programmable, refillable, and implantable. Front Pharmacol. (2011) 2:44. doi: 10.3389/fphar.2011.00044
22. Traynelis SF, Wollmuth LP, McBain CJ, Menniti FS, Vance KM, Oqden KK, et al. Glutamate receptor ion channels: structure, regulation, and function. Pharmacol Rev. (2010) 62:405–96. doi: 10.1124/pr.109.002451
23. Ahmed I, Bose SK, Pavese N, Ramlackhansingh A, Turkheimer F, Hotton G, et al. Glutamate NMDA receptor dysregulation in Parkinson's disease with dyskinesias. Brain. (2011) 134:979–86. doi: 10.1093/brain/awr028
24. Perez-Lloret S, Rascol O. Efficacy and safety of amantadine for the treatment of L-DOPA-induced dyskinesia. J Neural Transm. (2018) 125:1237–50. doi: 10.1007/s00702-018-1869-1
25. Varanese S, Howard J, Di Rocco A. NMDA antagonist memantine improves levodopa-induced dyskinesias and “on-off” phenomena in Parkinson's disease. Mov Disord. (2010) 25:508–10. doi: 10.1002/mds.22917
26. Moreau C, Delval A, Tiffreau V, Defebvre L, Dujardin K, Duhamel A, et al. Memantine for axial signs in Parkinson's disease: a randomised, double-blind, placebo-controlled pilot study. J Neurol Neurosurg Psychiatry. (2013) 84:552–5. doi: 10.1136/jnnp-2012-303182
27. Vidal EI, Fukushima FB, Valle AP, Villas Boas PJ. Unexpected improvement in levodopa-induced dyskinesia and on-off phenomena after introduction of memantine for treatment of Parkinson's disease dementia. J Am Geriatr Soc. (2013) 61:170–2. doi: 10.1111/jgs.12058
28. Wictorin K, Widner H. Memantine and reduced time with dyskinesia in Parkinson's disease. Acta Neurol Scand. (2016) 133:355–60. doi: 10.1111/ane.12468
29. Paoletti P. Molecular basis of NMDA receptor functional diversity. Eur J Neurosci. (2011) 33:1351–65. doi: 10.1111/j.1460-9568.2011.07628.x
30. Pahwa R, Tanner CM, Hauser RA, Isaacson SH, Nausieda PA, Truong DD, et al. ADS-5102 (amantadine) extended-release capsules for levodopa-induced dyskinesia in Parkinson disease (EASE LID Study): a randomized clinical trial. JAMA Neurol. (2017) 74:941–9. doi: 10.1001/jamaneurol.2017.0943
31. Li D, Zhang Y, Zhang W, Zhao P. Meta-analysis of randomized controlled trials on the efficacy and safety of donepezil, galantamine, rivastigmine, and memantine for the treatment of Alzheimer's disease. Front Neurosci. (2019) 13:472. doi: 10.3389/fnins.2019.00472
32. Tronci E, Fidalgo C, Zianni E, Collu M, Stancampiano R, Morelli M, et al. Effect of memantine on L-DOPA-induced dyskinesia in the 6-OHDA-lesioned rat model of Parkinson's disease. Neuroscience. (2014) 265:245–52. doi: 10.1016/j.neuroscience.2014.01.042
33. Thomas A, Iacno D, Luciano AL, Armellino K, Di Iorio A, Onofrj M. Duration of amantadine benefit on dyskinesia of severe Parkinson's disease. J Neurol Neurosurg Psychiatry. (2004) 75:141–3. Available online at: https://jnnp.bmj.com/content/75/1/141.long.
Keywords: intracerebral brain infusion, levodopa-induced dyskinesia, memantine, N-methyl-D-aspartate receptor, Parkinson's disease
Citation: Ogawa M, Zhou Y, Tsuji R, Kasahara J and Goto S (2019) Intrastriatal Memantine Infusion Dampens Levodopa-Induced Dyskinesia and Motor Deficits in a Mouse Model of Hemiparkinsonism. Front. Neurol. 10:1258. doi: 10.3389/fneur.2019.01258
Received: 02 August 2019; Accepted: 13 November 2019;
Published: 05 December 2019.
Edited by:
Steven Frucht, Mount Sinai Hospital, United StatesCopyright © 2019 Ogawa, Zhou, Tsuji, Kasahara and Goto. This is an open-access article distributed under the terms of the Creative Commons Attribution License (CC BY). The use, distribution or reproduction in other forums is permitted, provided the original author(s) and the copyright owner(s) are credited and that the original publication in this journal is cited, in accordance with accepted academic practice. No use, distribution or reproduction is permitted which does not comply with these terms.
*Correspondence: Satoshi Goto, sgoto@tokushima-u.ac.jp