- 1Department of Rehabilitation Sciences and Physiotherapy, Faculty of Medicine and Health Sciences, University of Antwerp, Antwerp, Belgium
- 2Multidisciplinary Motor Centre Antwerp (M2OCEAN), University of Antwerp, Antwerp, Belgium
- 3Department of Musculoskeletal Biology, University of Liverpool, Liverpool, United Kingdom
- 4School of Health Science, University of Liverpool, Liverpool, United Kingdom
- 5Applied Music Research Lab, Department of Music, University of Liverpool, Liverpool, United Kingdom
Objective: To investigate the effects of sound-based interventions (SBIs) on biomechanical parameters in stroke patients.
Methods: PubMed/Medline, Web of Science, the Physiotherapy Evidence Database (PEDro), and the Cochrane Library were searched until September 2019. Studies examining the effect of SBIs on kinematic, kinetic, and electromyographic outcome measures were included. Two independent reviewers performed the screening, and data extraction and risk-of-bias assessment were conducted with the PEDro and Newcastle–Ottawa scale. Disagreements were resolved by a third independent reviewer.
Results: Of the 858 studies obtained from all databases, 12 studies and 240 participants met the inclusion and exclusion criteria. Six studies investigated the effect of SBI on upper limb motor tasks, while six examined walking. Concerning quality assessment (Newcastle–Ottawa Quality Assessment Scale and PEDro), the nine cross-sectional studies had a median score of seven, while the randomized controlled trials had a median score of five (fair to good quality). In relation to upper limb motor tasks, only one study found improvements in cortical reorganization and increased central excitability and motor control during reaching after SBI (results of the other five studies were too diverse and lacked quality to substantiate their findings). In relation to walking, results were clearer: SBI led to improvements in knee flexion and gastrocnemius muscle activity.
Conclusion: Despite of the heterogeneity of the included studies, evidence was found demonstrating that SBI can induce biomechanical changes in motor behavior during walking in stroke patients. No conclusions could be formulated regarding reaching tasks. Additionally, directions for future research for understanding the underlying mechanism of the clinical improvements after SBI are: (1) using actual music pieces instead of rhythmic sound sequences and (2) examining sub-acute stroke rather than chronic stroke patients.
Introduction
Stroke is the most common life-threatening neurological disease and the main cause of long term disability in adults (1). After a stroke, only 30% of the survivors have no activity-limitations or participation restrictions (2). The remaining 70% of stroke survivors show persistent impairments in motor function.
It is clear that there is an urgent need for more effective and patient-tailored rehabilitation strategies. Music interventions seem to be beneficial for mobility, upper limb function, and quality of life after stroke (3–6). Results show that music interventions are beneficial for improving clinical outcome measures of both upper and lower extremities and spatiotemporal parameters such as walking speed and step length after stroke (3, 4, 6). Positive effects have also been found on communication-related and quality-of-life outcomes (3). Chen provided an overview of three meta-analyses discussing the effectiveness of music interventions after stroke and highlighted a gap in the literature (5)—the author concluded that although music interventions seem to be promising for enhancing clinical motor recovery after stroke, the quality of evidence is still rather low. It was suggested that more phase I and II studies are necessary as underlying mechanisms, and the nature (true motor recovery or compensation) of these improvements is still unclear (5). Indeed, without taking into account the movement quality of a certain task, it is impossible to discriminate between “true recovery” and “compensation” of motor patterns (7). True recovery would suggest that a patient is able to relearn elemental motor patterns, whereas compensatory strategies mean that an adaptation of remaining motor elements takes place (7). For example, reaching within arm's length can be achieved by either using only the upper limb or using increased sagittal trunk displacements to grasp the object. True recovery of reaching should consist of solely using shoulder flexion and elbow, wrist, and finger extension, while incorporating the trunk to grasp an object should be seen a compensatory strategy. A qualitative biomechanical analysis, assessing joint motion, inter-joint coordination, muscular behavior, or movement synergies, is a required first step in unraveling both concepts (7). Although biomechanical analysis alone cannot fully explain these concepts without an explanation of the underlying neural basis, it is a first step in understanding and explaining possible mechanisms. This additional analysis should be complementary to the extensive research on clinical outcome measures, since biomechanical analysis should always be related to clinical benefits—which have been thoroughly reported (3–6).
Music interventions can be categorized into two types of interventions—passive or receptive (i.e., listening to music) compared to active (e.g., produce music or have an active role during therapy) (8). This study focuses on the effects of passive interventions, as active music interventions, such as playing an instrument, make it difficult to distinguish the cause of the observed changes in motor behavior, which can be due to either the music heard or other behaviors associated with the therapy received. Similar to the review of Tang and Vezeau, the definition of music interventions in this study was extended to include rhythm, since these authors showed that little research has been performed on the role of music listening interventions (MLIs) with stroke patients (8). Hereinafter, we will refer to music listening and rhythmic sound sequence interventions as sound-based interventions (SBIs). It should be noted that interventions that use rhythmic sound sequences are also known as rhythmic auditory stimulation (RAS). Music interventions are able to facilitate and modulate neural plasticity (9, 10), one of the key elements in neurological rehabilitation (11). Activation of the motor regions together with the auditory cortex was seen after SBI, while only the auditory cortex was active before the intervention (9, 12). This suggests that music interventions induce brain reorganization processes and enhance neuronal co-activation and functional coupling of the auditory–motor network after stroke (9, 13, 14). Since music listening is related to several motor–cognitive functions such as memory, attention, semantic processing, and motor function (15), it may be an appropriate tool for neurological rehabilitation. The aims of this study are to investigate the effects of SBI on motor behaviors through a qualitative biomechanical analysis and to discern the underlying mechanisms of clinical improvements (i.e., distinguish between true recovery and compensation). To achieve these aims, we devised a three-part research question:
1) “Are motor behaviors altered during SBI?”
2) “Are motor behaviors altered after SBI?”
3) “Is SBI effective in improving motor behaviors?”
In relation to (1), we will analyze studies that compared similar motor tasks with and without SBI and measured their effects during the delivery of the interventions. Concerning (2), we will look into pre/post-designs that were used to assess the impact of SBI on motor behaviors after the intervention (either immediately after the single intervention or after a longer period of treatment). Finally, regarding (3), we aimed to investigate whether SBI resulted in statistically significant improvements on biomechanical parameters when compared to a control training program. We hypothesize that SBI results in some form of true recovery concerning motor behavior.
Methods
Protocol and Registration
This review was conducted according to the Preferred Reporting Items for Systematic Review and Meta-Analysis Statement (PRISMA). The checklist can be found as Appendix S1 (16). The study was registered in the PROSPERO database (no. CRD42018115118).
Eligibility Criteria
Studies were included if they met the following PICOS criteria:
1) Population: The study population included adults (18 years old or older) diagnosed with ischemic or hemorrhagic stroke (no limitations were set on time since stroke or level of functioning).
2) Intervention: Interventions had to be sound-based, including music listening or listening to rhythmic sequences (MLI or RAS, which could be performed by various instruments, e.g., metronome, synthesizer). At least one group of participants had to perform a task in this condition.
3) Controls: A similar motor act had to be performed without listening to music or rhythmic sequences (control intervention).
4) Outcome: Instrumented 3-D movement analysis with or without electromyography (EMG) had to be performed during a motor task to evaluate the effect of the SBI intervention, and outcome measures had to assess motor function in a biomechanical manner (e.g., movement velocity, movement time, smoothness of motion, joint angles, muscle activity or muscle-related assessment).
5) Study design: All designs except for systematic reviews, meta-analyses, surveys, and case reports.
Studies were excluded using the following criteria:
1) The SBI was not adequately specified (e.g., lack of information concerning music/rhythm, motor task).
2) Studies pertaining to interventions that involved an active music engagement (i.e., singing, playing rhythms on musical instruments).
3) The rhythm was used as a real-time feedback mechanism to aid proprioception and knowledge of performance.
4) Outcome measures assessed by means of a clinical test or motion capture systems were inadequately specified, or only spatiotemporal parameters were investigated since this has been studied thoroughly by previous reviews (3–6).
5) Studies not written in English, Dutch, German, or French.
Information Sources
A systematic search strategy was conducted using the electronic databases of PubMed, Web of Science, Cochrane, and the Physiotherapy Evidence Database (PEDro). A combination of the following free text words and Medical Subject Headings were used: stroke, cerebrovascular disorder, music, rhythm, rhythmical auditory stimulation, music supported therapy, acoustic, muscle, EMG, kinematics, and biomechanics. The details of the final search strategy, performed in September 2019, can be found in Appendix S2.
Study Selection
The screening procedure was performed by two independent researchers (TVC and EC). To collect potentially relevant studies, eligibility was screened based on title and abstract based on the provided inclusion and exclusion criteria described above. Full texts were retrieved and evaluated based on the same eligibility criteria. Afterward, full texts were gathered and evaluated on the previously set inclusion criteria. Reference lists were manually screened to identify additional relevant studies. Discrepancies were discussed and resolved with consensus by a third independent person (KD).
Assessment of Quality
The risk of bias was assessed by two independent reviewers (TVC and KD) by using the Newcastle–Ottawa Quality Assessment Scale (NOS) (17, 18) and the PEDro scale (19). In case of uncertainty at any point during the scoring process, consensus was sought by a third reviewer (JO). The adapted version for cross-sectional studies by Herzog et al. (20) was employed. The NOS is an instrument that assesses the risk of bias by awarding a star for each answer that meets the criteria; a maximum of nine stars can be obtained: four stars for selection, two stars for comparability, and three stars for outcome. Each star given projects a low risk of bias for this criterion. As a criterion for quality, the Agency of Healthcare Research standards were used (21). Included studies were of good quality when they scored three or four stars in the selection domain, one or two in the comparability domain, and two or three in the outcome/exposure domain. Fair quality was assigned to studies that scored two stars in the selection domain, one or two in the comparability domain, and two or three in the outcome/exposure domain. Poor quality was assigned to studies that received zero or one star in the selection domain, zero stars in the comparability domain, and zero or one star in the outcome/exposure domain. For randomized controlled trials (RCTs), the PEDro scale was used, which assesses 11 items such as random allocation of the subjects, concealed allocation, and blinding of therapists and assessors (22). The total PEDro score was considered of good quality when it was six or higher (23).
Data Extraction and Analysis
Extracted data consisted of subject characteristics (age, gender, time post-stroke, inclusion criteria), outcome measures, motion capture, and sound apparatus (see Table 1). Results were mostly described as a comparison between performing a motor task with and without SBI or, in the case of an RCT study, as a difference between groups based on intervention (see Table 2). Furthermore, a general conclusion per study is also provided.
For the analysis, the percentage of change between SBI and no-sound conditions was examined. Data figures can be found as Appendices S3–S5. To facilitate the qualitative interpretation of the RCTs, forest plots were created using RevMan 5.3 (37). The number of participants, mean differences, and standard deviations were inserted in the RevMan 5.3 template. When the necessary data were not available, authors were contacted to complete the data form. If authors did not respond, missing data were manually calculated using the RevMan 5.3 calculator, if possible. To calculate pooled effect sizes, inverse variance was used as statistical method, a random-effects model was used as an analysis model, and standardized mean differences (SMDs) were calculated as the effect measure. Heterogeneity between the studies was assessed using I2 statistics, together with magnitude and direction of effects for overlapping ranges (38, 39). Cochrane guidelines were used to interpret the heterogeneity: 0–40% (might not be important), 30–60% (may represent moderate heterogeneity), 50–90% (may represent substantial heterogeneity), and 75–100% (considerable heterogeneity) (39). Effect sizes were categorized as a standard mean effect size of 0 representing no change, 0.2 representing a small effect, 0.5 representing a medium effect, and 0.8 representing a large effect (40). Confidence intervals (CIs) were set to 95%.
Results
Study Selection
Of the 733 unique studies obtained from all databases, 13 studies met all inclusion criteria. The study selection process is depicted in Figure 1. Concerning the quality assessment (see Table 3), the nine cross-sectional studies had a median score of seven, with a maximum score of eight and a minimum of four. In total, five studies had a good methodological quality, while the others were of fair to poor quality. The majority of studies used a selected group of subjects who did not represent the target population, and no study justified their sample size. Concerning the quality assessment of the RCTs, a median score of five was observed, with a maximum of nine and a minimum of five (see Table 4). Most studies did not meet the criteria of blinding the subjects and therapist, as this does not seem possible with respect to treatment. It would be very difficult to blind people as to receiving or executing SBI compared to a placebo or no therapy.
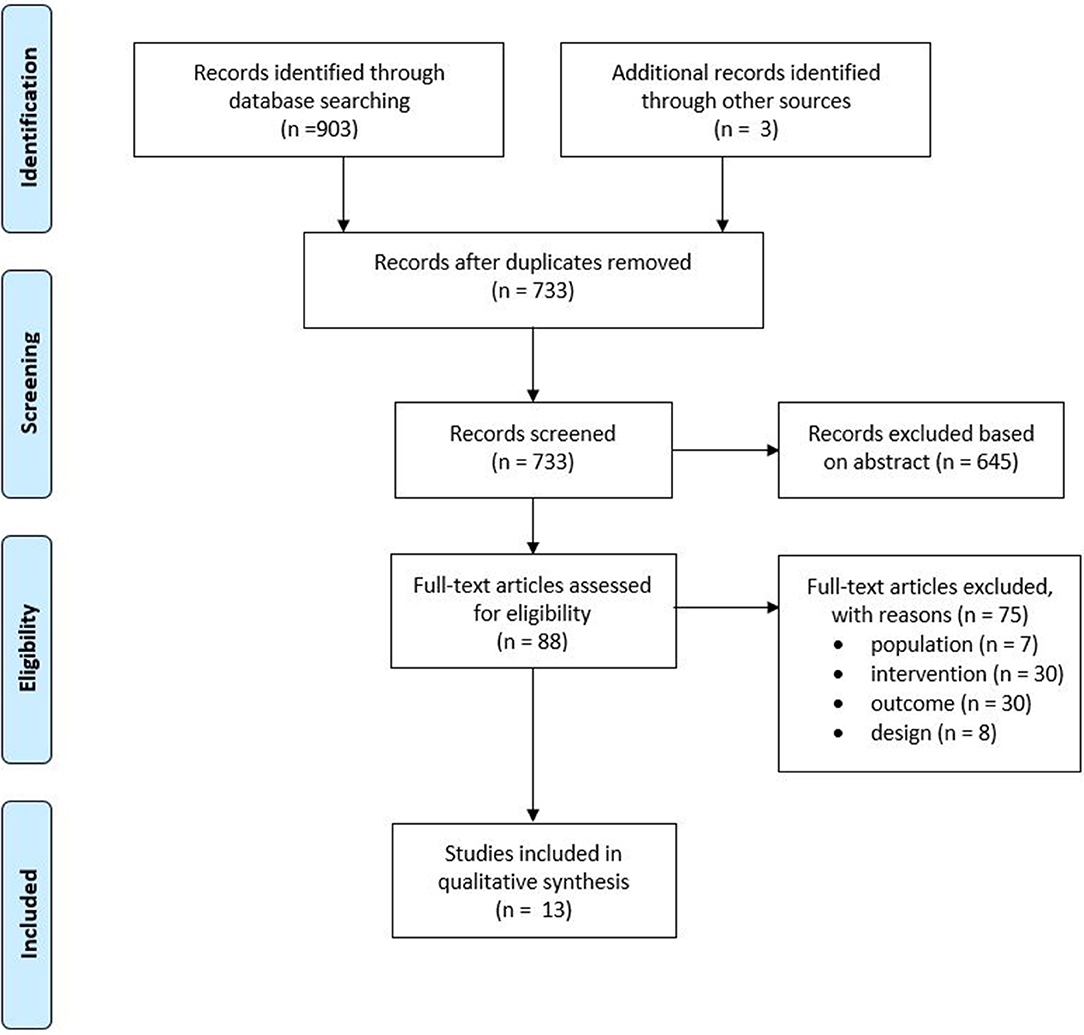
Figure 1. Preferred Reporting Items for Systematic Review and Meta-Analysis Statement (PRISMA) flowchart of the included studies.
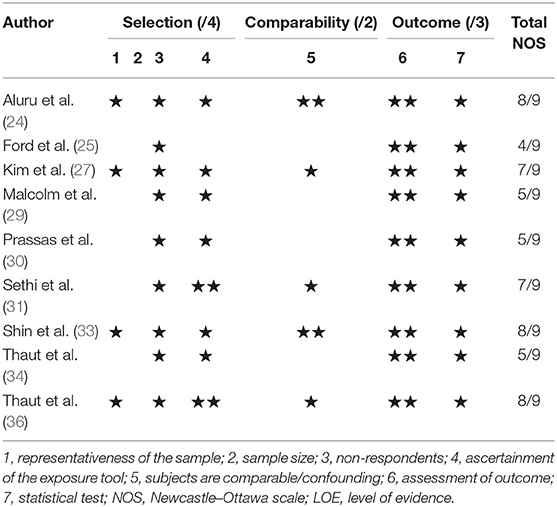
Table 3. Risk of bias for cross-sectional studies with the Newcastle–Ottawa Quality Assessment Scale.

Table 4. Risk of bias of randomized controlled trials with the Physiotherapy Evidence Database (PEDro) scale.
Study Characteristics
In total, data from 279 stroke survivors (98 females, 174 males) were included in this study. The examined participants had a mean age of 61 years (range: 44–73 years) and an average time post-stroke of 24 months (range: 16.1 days−5.5 years) (24–36). One study did not provide mean age and time post-stroke of their included population (25).
Four studies were RCTs investigating the effectiveness of SBI by comparing motor activities with and without sound (26, 28, 32, 35). Additionally, two studies examined the effectiveness of SBI by means of a pre/post-design (29, 33), and seven studies investigated the immediate effects of SBI on motor tasks in a cross-sectional study (24, 25, 27, 30, 31, 34, 36).
Motor tasks included both upper and lower limb tasks and varied across studies. Upper limb tasks were generally related to reaching exercises (27, 29, 31, 32, 36), but one study investigated wrist flexion/extension (24). Lower limb tasks mostly consisted of walking trials which could be performed over ground or on a treadmill (25, 26, 30, 33–35). One study examined standing balance in a static condition (28).
The majority of studies generated rhythmic sound sequences via a metronome (25–27, 29, 31, 36), two used an actual or a software package of a synthesizer/keyboard (28, 30), and two others a combination of both (33, 35). In these studies, a baseline assessment was performed to calculate the reach or step frequency. Afterward, an increased or decreased auditory frequency was provided to examine the influence of the rhythmic sounds' characteristics on the movement. These rhythmic sound sequences were generated via individual sounds generated by synthesizers or metronomes. In the study by Aluru et al. (24), patients were exposed to four different types of sounds: metronome sounds, a baby's laughter, self-selected music, and silence. Only one study used actual music—Thaut et al. (34) used a music piece of renaissance dance style orchestrated for woodwinds, harpsichord, and percussion. One study did not specify the manner of sound production or apparatus used yet elaborately described the motor task procedure, time of execution, and sound frequency (0.25–1.0/s) (32). The outcome measures and motion capture systems used to assess motor behavior can be found in Table 1.
Synthesis of Results
Research Question 1: Is Motor Behavior Altered During SBI?
The immediate effect of SBI on muscle activity was examined by three studies (24, 27, 34), on joint angles by four (24, 27, 30, 36), and temporal parameters during reaching by three studies (25, 31, 36) (see Appendix S3).
During SBI, significant differences in gastrocnemius muscle activity were found during walking compared to no sound, a 10.8% increase in amplitude at push-off, a 16.5% increase in amplitude variation, and a 10.4% decrease in amplitude during swing (34). Concerning upper limb muscle activity, a non-significant increase in the maximum voluntary isometric contraction of biceps activity of 0.34% and a significant increase in triceps activity of 18.18% were observed (27). Moreover, a decrease of 10% was found in the co-contraction ratio of the aforementioned muscles during reaching (27). Aluru et al. (24) investigated the changes of SBI between three types of stroke patients: spastic paresis, spastic co-contraction, and minimal paresis. They concluded that listening to a metronome or self-selected songs increased wrist flexor activity in patients with spastic paresis but not those with spastic co-contraction. For patients with minimal paresis, results were unclear (24).
During walking, the vertical displacements of the center of mass significantly decreased with 15.15% between SBI and the no-sound condition (30). However, no significant differences were found concerning range of motion (RoM) of the hip and knee, trunk, and pelvic tilt angle (30). Aluru et al. (24) concluded that patients with spastic paresis improved their wrist extension with a metronome during reaching, while the spastic co-contraction group did improve the amplitude of their wrist extension without cue. On the other hand, the minimal paresis group improved when listening to “happy” sounds (24).
Temporal parameters were only assessed during reaching. Studies found that the deviation of the optimal peak acceleration decreased by 76% (36) and peak velocity increased by 34% in the sound condition (31), while movement time did not significantly differ between the two conditions (36). Increased coordination of the arm/leg and trunk during walking (25) and decreased variability (range: 12.5–214%) of the upper limb during reaching (31, 36) were observed during SBI.
In summary, there is a small amount of evidence that after SBI, muscle activity of the gastrocnemius and RoM of the upper limb increased, while also normalizing acceleration, enhancing peak velocity, and decreasing variability.
Research Question 2: Is Motor Behavior Altered After SBI?
Only two studies examined the effects of SBI on motor behavior after a period of 10–28 days of therapy (29, 33) (see Appendix S4), and both examined different motor tasks that necessitated different outcome measures, which makes the comparison difficult. During reaching tasks, the segmental contribution of the trunk seemed to decrease, while the contribution of shoulder flexion increased (29). No significant differences were found pre- and post-SBI concerning the contribution of elbow extension (29). In addition, movement time decreased by 23%, while reach velocity increased by 20% (29). Of the 28 joint angle parameters during walking assessed by Shin et al. (33), only two were significant before and after SBI in stroke patients. Maximal knee flexion during mid-swing and maximal dorsiflexion during terminal stance increased by 13 and 17%, approximately (33).
In summary, no consensus could be reached, due to the variety of outcome measures and motor tasks. However, there was a tendency for SBI to affect several biomechanical parameters.
Research Question 3: Is SBI Effective in Improving Motor Behavior?
In order to examine whether the observed effects in the pre/post-designs are due to SBI or natural recovery, results should be compared to a control group, which was the case for four studies (26, 28, 32, 35). Our analysis shows that there was a moderate effect of music listening on muscle activity (SMD 0.60, 95% CI 0.35–0.85) (26, 35), as depicted in Figure 2. The gastrocnemius muscle was the only one assessed in both studies, and a moderate effect was seen in favor of SBI (SMD 0.74, 95% CI 0.06–1.42). However, a considerable amount of heterogeneity was observed (I2 = 53%). No significant differences were observed for the tibialis anterior muscle and quadriceps muscle, while a large effect was observed for the hamstring muscles, SMD 0.89 (95% CI 0.36–1.43). Concerning joint angles during walking, SBI seemed to improve RoM of the knee by 25 and 39% in the control and the experimental group, respectively (26). No significant differences were found between treadmill training with and without RAS in static balance outcome measures as evaluated by means of center of pressure (COP)–based measures (28). Finally, Shahine and Shafshak's (32) was the only study looking at upper limb activities; bilateral arm training with SBI significantly increased central motor conduction time (which is the difference between the peripheral and cortical latency of the signal), motor-evoked potential resting threshold, and amplitude ratio compared to the control group (see Appendix S5).
In summary, there is a limited amount of evidence that SBI has a positive effect on motor behavior—muscle activity of the gastrocnemius and hamstring muscles improved, in addition to neurophysiological parameters and knee flexion amplitude.
Discussion
Do Sound-Based Interventions Influence Motor Behavior?
Although recent literature concluded that music interventions are beneficial for improving clinical outcome measures of both upper and lower extremities after stroke (3, 4, 6), little research has been performed concerning the underlying mechanisms of SBI. Although biomechanical analysis cannot fully distinguish between true recovery and compensation, as neural mechanisms should also be investigated (e.g., motor control), it is a first step in understanding and explaining the observed clinical improvements. We reviewed a total of 12 studies that provided biomechanical data obtained from 240 survivors during the execution of a variety of motor tasks. The overall risk of bias of the included studies was moderate; 10 studies were of good quality, while eight were of fair to poor quality. However, the great heterogeneity between studies, mainly due to the great variation in outcome measures and assessment at different time points, made comparison difficult.
1) Is motor behavior altered during SBI?—Yes!
High-quality studies all reported significant improvements in movement quality, e.g., decreased co-contraction, increased peak velocity, and decreased variability. So it seems that SBI might be able to improve movement control.
2) Is motor behavior altered after SBI?—Not clear…
Although two studies (each examining a different motor task) showed that several biomechanical parameters were improved after SBI, no clear conclusions could be made. However, there is a tendency for SBI to aid motor behavior.
3) Is SBI effective in improving motor behavior?—Yes, but more evidence is needed.
Studies examining the lower limbs showed signs of normalization of muscular activity and motor recruitment (26, 35), while no improvements were observed concerning static balance (28). Furthermore, only one study investigated upper limb movements (32). Therefore, more qualitative research is needed.
How Do Sound-Based Interventions Influence Walking and Reaching Performance?
1) The effect of SBI on walking
After stroke, problems with foot clearance are commonly reported, resulting in an increased fall risk (41–43). SBI seems to be able to improve clearance in swing by increasing knee flexion and push-off activity, which is beneficial during gait rehabilitation after stroke to decrease tripping and enhance walking speed. An effective push-off is important for leg swing acceleration and knee flexion (44, 45). These improvements might be one of the underlying causes to explain the significant changes seen in walking speed, stride length, gait cadence, and stride symmetry after music interventions in stroke patients (3). It seems that some form of true recovery might have taken place at the level of the knee; however, too little research has been performed in order to fully explain the mechanisms, as we still have no idea what drives this recovery process based on the available literature.
1. The effect of SBI on reaching tasks
Although improvements in motor control, cortical reorganization, and/or increased central excitability were observed during reaching, too little qualitative research was performed on kinematic parameters after SBI. It is suggested that MLI aids in motor planning activities, as listening to music engages a complex network of brain regions, in both the auditory and the motor system (46). To date, evidence is still too limited to conclude that SBI is able to induce true recovery after stroke. In addition, studies concerning neuroplasticity suggest that the first weeks after a stroke are crucial for inducing functional and structural cortical reorganization (47, 48), whereas the majority of studies assessed chronic stroke patients. Therefore, future research should include sub-acute stroke patients since cortical reorganization is more apparent in this population, which is a sign of true recovery. To date, no research has been performed on the effect of SBI during reaching in sub-acute stroke patients.
Music or Rhythmic Sound Sequences?—Directions for Future Research
Only two studies used actual music pieces or musical instruments as part of the intervention (24, 34). The majority of studies used a metronome or a synthesizer to play a certain rhythm. However, walking is a more dynamic situation which cannot be entirely explained as consecutive beats (49). Music, on the other hand, has a more complex auditory stream of rhythmic, dynamic, harmonic, and timbral structures. These different parameters can map different gait events, not just synchronizing heel contact to a beat, as exemplified by Rodger and Craig (49): “a patient may either lift his/her toe off with a beat, place the heel with a chord, or swing the leg with part of a melody, and still have the veridical experience of being in time with the sound”. Studies have already shown that moving to music compared to the sound of a metronome resulted in a faster walking speed and decreased synchronization errors (50–52). Furthermore, listening to music activates cortical and paralimbic areas related to neural systems of reward and emotion (53), which makes music an intervention that can be rewarding and motivating and at the same time regulate emotions, arousal, and cognitive functions (54), especially when patients are able to choose the music themselves (53, 55). When healthy volunteers listen to self-selected music, increased muscle activity and heart and respiration rate were observed compared to non-self-selected music (53). Even though the benefits of MLI are well-described in healthy adults, the lack of studies using actual music pieces in this review highlights the need for future research on MLIs.
Limitations
There are a few limitations of this review that should be acknowledged. First, during the systematic literature search, only studies written in English, Dutch, German, or French were included. It is therefore possible that relevant studies and important information were missed during the search process. Second, some caution with these findings is required since conclusions were sometimes based on the results of a single study. The heterogeneity in outcome measures made it difficult to find comparable results. Third, although clinical improvements have been reported repeatedly, the amount of research regarding the quality of the movement after SBI is very limiting.
Conclusion
There is evidence concluding that SBI is able to induce some form of true recovery during walking after stroke, while it was difficult to provide evidence for reaching tasks. There was a great amount of heterogeneity between the included studies, hampering clear conclusions. At this point, it is still unknown what the underlying mechanisms of the observed improvements are. Several important gaps in the literature were determined, which necessitates further qualitative examination. Future research should include larger study samples, sub-acute stroke patients, and actual music pieces instead of only rhythmical sounds to examine music interventions after stroke.
Data Availability Statement
Publicly available datasets were analyzed in this study. All datasets analyzed for this study are included in the article/Supplementary Material.
Author Contributions
TV co-designed the study, carried out the screening procedure, performed the risk-of-bias assessment, was involved with data extraction and interpretation of data, and drafted the manuscript. KD'A co-designed the study, took part in the screening procedure, performed the risk-of-bias assessment, and was involved in data interpretation and drafting of the manuscript. JO'B took part in the screening procedure and risk-of-bias assessment and was involved with data interpretation and drafting of the manuscript. EC co-designed the study, carried out the screening procedure, and was involved in data interpretation and drafting of the manuscript. All authors gave final approval for publication.
Funding
This project was funded by the University of Liverpool's Research Development Initiative Fund and a mobility grant from the Flandres Research Foundation. Open access publication fees are supported by the University of Liverpool's Gold Open Access Institutional Fund.
Conflict of Interest
The authors declare that the research was conducted in the absence of any commercial or financial relationships that could be construed as a potential conflict of interest.
The reviewer AD and handling Editor declared their shared affiliation at the time of review.
Supplementary Material
The Supplementary Material for this article can be found online at: https://www.frontiersin.org/articles/10.3389/fneur.2019.01141/full#supplementary-material
References
1. Meijer R, Ihnenfeldt DS, de Groot IJ, van Limbeek J, Vermeulen M, de Haan RJ. Prognostic factors for ambulation and activities of daily living in the subacute phase after stroke. A systematic review of the literature. Clin Rehabil. (2003) 17:119–29. doi: 10.1191/0269215503cr585oa
3. Magee WL, Clark I, Tamplin J, Bradt J. Music interventions for acquired brain injury. Cochrane Database Syst Rev. (2017) 1:CD006787. doi: 10.1002/14651858.CD006787.pub3
4. Zhang Y, Cai J, Zhang Y, Ren T, Zhao M, Zhao Q. Improvement in stroke-induced motor dysfunction by music-supported therapy: a systematic review and meta-analysis. Sci Rep. (2016) 6:38521. doi: 10.1038/srep38521
5. Chen JL. Music-supported therapy for stroke motor recovery: theoretical and practical considerations. Ann N Y Acad Sci. (2018) 1423:13726. doi: 10.1111/nyas.13726
6. Yoo GE, Kim SJ. Rhythmic auditory cueing in motor rehabilitation for stroke patients: systematic review and meta-analysis. J Music Ther. (2016) 53:149–77. doi: 10.1093/jmt/thw003
7. Levin MF, Kleim JA, Wolf SL. What do motor “recovery” and “compensation” mean in patients following stroke? Neurorehabil Neural Repair. (2009) 23:313–9. doi: 10.1177/1545968308328727
8. Tang HY, Vezeau T. The use of music intervention in healthcare research: a narrative review of the literature. J Nurs Res. (2010) 18:174–90. doi: 10.1097/JNR.0b013e3181efe1b1
9. Francois C, Grau-Sanchez J, Duarte E, Rodriguez-Fornells A. Musical training as an alternative and effective method for neuro-education and neuro-rehabilitation. Front Psychol. (2015) 6:475. doi: 10.3389/fpsyg.2015.00475
10. Pantev C, Herholz SC. Plasticity of the human auditory cortex related to musical training. Neurosci Biobehav Rev. (2011) 35:2140–54. doi: 10.1016/j.neubiorev.2011.06.010
11. Li S. Spasticity, Motor Recovery, and Neural Plasticity after Stroke. Frontiers in neurology. (2017) 8:120. doi: 10.3389/fneur.2017.00120
12. Alluri V, Toiviainen P, Burunat I, Kliuchko M, Vuust P, Brattico E. Connectivity patterns during music listening: evidence for action-based processing in musicians. Hum Brain Mapp. (2017) 38:2955–70. doi: 10.1002/hbm.23565
13. Ripolles P, Rojo N, Grau-Sanchez J, Amengual JL, Camara E, Marco-Pallares J, et al. Music supported therapy promotes motor plasticity in individuals with chronic stroke. Brain Imaging Behav. (2016) 10:1289–307. doi: 10.1007/s11682-015-9498-x
14. Särkämö T, Tervaniemi M, Laitinen S, Forsblom A, Soinila S, Mikkonen M, et al. Music listening enhances cognitive recovery and mood after middle cerebral artery stroke. Brain. (2008) 131(Pt 3):866–76. doi: 10.1093/brain/awn013
15. Reybrouck M, Vuust P, Brattico E. Music and brain plasticity: how sounds trigger neurogenerative adaptations. In: Chaban VV, editor. Neuroplasticity - Insights of Neural Reorganization. London, UK: IntechOpen (2018). Available online at: https://www.intechopen.com/books/neuroplasticity-insights-of-neural-reorganization/music-and-brain-plasticity-how-sounds-trigger-neurogenerative-adaptations
16. Moher D, Liberati A, Tetzlaff J, Altman DG, Group P. Preferred reporting items for systematic reviews and meta-analyses: the PRISMA statement. PLoS Med. (2009) 6:e1000097. doi: 10.1371/journal.pmed.1000097
17. Luchini C, Stubbs B, Solmi M, Veronese N. Assessing the quality of studies in meta-analyses: advantages and limitations of the Newcastle Ottawa Scale. World J Meta-Anal. (2017) 5:80–4. doi: 10.13105/wjma.v5.i4.80
18. Hartling L, Milne A, Hamm MP, Vandermeer B, Ansari M, Tsertsvadze A, et al. Testing the Newcastle Ottawa Scale showed low reliability between individual reviewers. J Clin Epidemiol. (2013) 66:982–93. doi: 10.1016/j.jclinepi.2013.03.003
19. score SE-P.. (2019) Available online at: https://www.strokengine.ca/glossary/pedro-score/ (accessed September 23, 2019).
20. Herzog R, Alvarez-Pasquin MJ, Diaz C, Del Barrio JL, Estrada JM, Gil A. Are healthcare workers' intentions to vaccinate related to their knowledge, beliefs and attitudes? A systematic review. BMC Public Health. (2013) 13:154. doi: 10.1186/1471-2458-13-154
21. Hartling L, Hamm M, Milne A, Vandermeer B, Santaguida PL, Ansari M, et al. Validity and Inter-Rater Reliability Testing of Quality Assessment Instruments. Rockville, MD: AHRQ Methods for Effective Health Care (2012).
22. Maher CG, Sherrington C, Herbert RD, Moseley AM, Elkins M. Reliability of the PEDro scale for rating quality of randomized controlled trials. Phys Ther. (2003) 83:713–21. doi: 10.1093/ptj/83.8.713
23. Armijo-Olivo S, da Costa BR, Cummings GG, Ha C, Fuentes J, Saltaji H, et al. PEDro or cochrane to assess the quality of clinical trials? A meta-epidemiological study. PLoS ONE. (2015) 10:e0132634. doi: 10.1371/journal.pone.0132634
24. Aluru V, Lu Y, Leung A, Verghese J, Raghavan P. Effect of auditory constraints on motor performance depends on stage of recovery post-stroke. Front Neurol. (2014) 5:106. doi: 10.3389/fneur.2014.00106
25. Ford MP, Wagenaar RC, Newell KM. The effects of auditory rhythms and instruction on walking patterns in individuals post stroke. Gait Posture. (2007) 26:150–5. doi: 10.1016/j.gaitpost.2006.08.007
26. Kim JS, Oh DW, Kim SY, Choi JD. Visual and kinesthetic locomotor imagery training integrated with auditory step rhythm for walking performance of patients with chronic stroke. Clin Rehabil. (2011) 25:134–45. doi: 10.1177/0269215510380822
27. Kim JR, Jung MY, Yoo EY, Park JH, Kim SH, Lee J. Effects of rhythmic auditory stimulation during hemiplegic arm reaching in individuals with stroke: an exploratory study. Hong Kong J Occup Ther. (2014) 24:64–71. doi: 10.1016/j.hkjot.2014.11.002
28. Mainka S, Wissel J, Voller H, Evers S. The use of rhythmic auditory stimulation to optimize treadmill training for stroke patients: a randomized controlled trial. Front Neurol. (2018) 9:755. doi: 10.3389/fneur.2018.00755
29. Malcolm MP, Massie C, Thaut M. Rhythmic auditory-motor entrainment improves hemiparetic arm kinematics during reaching movements: a pilot study. Top Stroke Rehabil. (2009) 16:69–79. doi: 10.1310/tsr1601-69
30. Prassas S, Thaut M, McIntosh G, Rice R. Effect of auditory rhythmic cuing on gait kinematic parameters of stroke patients. Gait Posture. (1997) 6:218–23. doi: 10.1016/S0966-6362(97)00010-6
31. Sethi A, Stergiou N, Patterson TS, Patten C, Richards LG. Speed and rhythm affect temporal structure of variability in reaching poststroke: a pilot study. J Motor Behav. (2017) 49:35–45. doi: 10.1080/00222895.2016.1219304
32. Shahine E, Shafshak T. The effect of repetitive bilateral arm training with rhythmic auditory cueing on motor performance and central motor changes in patients with chronic stroke. Egypti Rheumatol Rehabil. (2014) 2014:8–13. doi: 10.4103/1110-161X.128128
33. Shin YK, Chong HJ, Kim SJ, Cho SR. Effect of rhythmic auditory stimulation on hemiplegic gait patterns. Yonsei Med J. (2015) 56:1703–13. doi: 10.3349/ymj.2015.56.6.1703
34. Thaut M, McIntosh G, Prassas S, Ruth R. Effect of rhythmic auditory cuing on temporal stride parameters and EMG patterns in hemiparetic gait of stroke patients. Neurorehabil Neural Repair. (1993) 7:9–16. doi: 10.1177/136140969300700103
35. Thaut M, McIntosh G, Rice R. Rhythmic facilitation of gait training in hemiparetic stroke rehabilitation. J Neurol Sci. (1997) 151:207–12. doi: 10.1016/S0022-510X(97)00146-9
36. Thaut MH, Kenyon GP, Hurt CP, McIntosh GC, Hoemberg V. Kinematic optimization of spatiotemporal patterns in paretic arm training with stroke patients. Neuropsychologia. (2002) 40:1073–81. doi: 10.1016/S0028-3932(01)00141-5
37. Review Manager (RevMan). Computer Program. Version 5.3. Copenhagen: The Nordic Cochrane Centre TCC (2014).
38. Higgins JP, Thompson SG. Quantifying heterogeneity in a meta-analysis. Stat Med. (2002) 21:1539–58. doi: 10.1002/sim.1186
39. Higgins JPT, Thomas J, Chandler J, Cumpston M, Li T, Page MJ. (eds.). Cochrane Handbook for Systematic Reviews of Interventions. 2nd ed. Chichester: John Wiley & Sons (2019).
40. Cohen J. Statistical Power Analysis for the Behavioral Sciences: Erlbaum Associates. Hoboken, NJ: Taylor and Francis (1988).
41. Barrett RS, Mills PM, Begg RK. A systematic review of the effect of ageing and falls history on minimum foot clearance characteristics during level walking. Gait Posture. (2010) 32:429–35. doi: 10.1016/j.gaitpost.2010.07.010
42. Matsuda F, Mukaino M, Ohtsuka K, Tanikawa H, Tsuchiyama K, Teranishi T, et al. Analysis of strategies used by hemiplegic stroke patients to achieve toe clearance. Japan J Comp Rehabil Sci. (2016) 7:111–8. doi: 10.11336/jjcrs.7.111
43. Matsuda F, Mukaino M, Ohtsuka K, Tanikawa H, Tsuchiyama K, Teranishi T, et al. Biomechanical factors behind toe clearance during the swing phase in hemiparetic patients. Top Stroke Rehabil. (2017) 24:177–82. doi: 10.1080/10749357.2016.1234192
44. Li L, Landin D, Grodesky J, Myers J. The function of gastrocnemius as a knee flexor at selected knee and ankle angles. J Electromyogr Kinesiol. (2002) 12:385–90. doi: 10.1016/S1050-6411(02)00049-4
45. Zelik KE, Adamczyk PG. A unified perspective on ankle push-off in human walking. J Exp Biol. (2016) 219(Pt 23):3676–83. doi: 10.1242/jeb.140376
46. Gordon CL, Cobb PR, Balasubramaniam R. Recruitment of the motor system during music listening: an ALE meta-analysis of fMRI data. PLoS ONE. (2018) 13:e0207213. doi: 10.1371/journal.pone.0207213
47. Krakauer JW, Carmichael ST, Corbett D, Wittenberg GF. Getting neurorehabilitation right: what can be learned from animal models? Neurorehabil Neural Repair. (2012) 26:923–31. doi: 10.1177/1545968312440745
48. Murphy TH, Corbett D. Plasticity during stroke recovery: from synapse to behaviour. Nat Rev Neurosci. (2009) 10:861–72. doi: 10.1038/nrn2735
49. Rodger MW, Craig CM. Beyond the metronome: auditory events and music may afford more than just interval durations as gait cues in Parkinson's disease. Front Neurosci. (2016) 10:272. doi: 10.3389/fnins.2016.00272
50. Wittwer JE, Webster KE, Hill K. Music and metronome cues produce different effects on gait spatiotemporal measures but not gait variability in healthy older adults. Gait Posture. (2013) 37:219–22. doi: 10.1016/j.gaitpost.2012.07.006
51. Styns F, van Noorden L, Moelants D, Leman M. Walking on music. Hum Mov Sci. (2007) 26:769–85. doi: 10.1016/j.humov.2007.07.007
52. Thaut MH, Rathbun JA, Miller RA. Music versus metronome timekeeper in a rhythmic motor task. Int J Arts Med. (1997) 5:4–12.
53. Blood AJ, Zatorre RJ. Intensely pleasurable responses to music correlate with activity in brain regions implicated in reward and emotion. Proc Natl Acad Sci USA. (2001) 98:11818–23. doi: 10.1073/pnas.191355898
54. Sarkamo T. Music for the ageing brain: cognitive, emotional, social, and neural benefits of musical leisure activities in stroke and dementia. Dementia. (2018) 17:670–85. doi: 10.1177/1471301217729237
Keywords: sound-based interventions, biomechanics, music, sound, stroke—diagnosis, therapy, stroke rehabilitation
Citation: Van Criekinge T, D'Août K, O'Brien J and Coutinho E (2019) The Influence of Sound-Based Interventions on Motor Behavior After Stroke: A Systematic Review. Front. Neurol. 10:1141. doi: 10.3389/fneur.2019.01141
Received: 16 May 2019; Accepted: 11 October 2019;
Published: 01 November 2019.
Edited by:
Margit Alt Murphy, University of Gothenburg, SwedenReviewed by:
Gabriela Lopes Dos Santos, Federal University of São Carlos, BrazilAnna Danielsson, University of Gothenburg, Sweden
Copyright © 2019 Van Criekinge, D'Août, O'Brien and Coutinho. This is an open-access article distributed under the terms of the Creative Commons Attribution License (CC BY). The use, distribution or reproduction in other forums is permitted, provided the original author(s) and the copyright owner(s) are credited and that the original publication in this journal is cited, in accordance with accepted academic practice. No use, distribution or reproduction is permitted which does not comply with these terms.
*Correspondence: Eduardo Coutinho, e.coutinho@liverpool.ac.uk