- 1Libera Università Campus Bio-Medico di Roma, Rome, Italy
- 2Department of Neurology, University of California, Irvine, Irvine, CA, United States
- 3Mount Sinai West, New York City, NY, United States
This report describes the successful management of a case of central neurogenic hyperventilation (CNH) refractory to high dose sedation by increasing the mechanical dead space. A 46-year-old male presented with a history of multiple neurological symptoms. Following an extensive evaluation, he was diagnosed with primary diffuse CNS lymphoma and started on high dose steroids. After initial symptomatic improvement, the patient developed increasing respiratory distress and tachypnea. He was intubated and transferred to the neurointensive care unit (neuro ICU). While in the ICU the patient remained ventilator dependent with significant tachypnea and respiratory alkalosis resistant to fentanyl and propofol. This prompted an attempt to normalize the PaCO2 via an increase of the mechanical dead space. This approach successfully increased PaCO2 and bridged the patient until ongoing therapy for the underlying disease resolved the pervasive breathing pattern typical of CNH. Further investigation is warranted to evaluate this strategy, which upon review of the literature appears underused.
Introduction
Central neurogenic hyperventilation (CNH) is a rare condition characterized by hyperventilation that persists even during states of sleep, low PaCO2, and high arterial pH in the absence of toxic or metabolic etiologies. It was first described in patients exposed to acute anoxia by Plum and Swanson (1). It has subsequently been described in several other chronic, diffusely infiltrating processes such as diffusely infiltrating lymphomas, pontine glioma, medulloblastoma, anti-NMDAR encephalitis, multiple sclerosis, histiocytosis, and even a case of laryngeal carcinoma (2, 3). Unfortunately, the pathophysiological mechanisms remain poorly understood and the treatment options remain limited. Here we describe a patient with CNH that was refractory to conventional analgesics and sedatives but responded well to increasing the mechanical dead space.
Case
A 46-year-old male with no prior medical history presented to UC Irvine with a several month history of episodes of right and left hemiparesis, progressive bulbar weakness, paresthesia, dysarthria, and headache. He also reported a 30-pound unintentional weight loss. He was admitted to the neurology service and underwent an extensive evaluation for autoimmune, demyelinating, vascular, and neoplastic processes. MRI brain demonstrated several small discrete foci of restricted diffusion in the white matter, enhancement in large portions of the bilateral corticospinal tracts, and significant pontine involvement (Figure 1). Lumbar puncture demonstrated CSF pleocytosis and elevated protein. Histology of the right frontal brain lesion was consistent with diffuse large B-cell lymphoma (DLBCL), and a bone marrow biopsy demonstrated normocellular marrow. The patient was diagnosed with primary diffuse CNS lymphoma (PCNSL) and started on high dose steroid therapy.
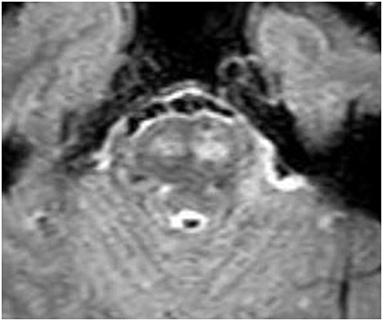
Figure 1. Fluid attenuated inversion recovery (FLAIR) demonstrating pontine hyperintensity from lymphoma involvement.
Early in the hospital course the patient was found to be in acute respiratory distress presenting with tachycardia, tachypnea, and increased work of breathing. He was emergently intubated and transferred to the neuro ICU. CT angiogram of the chest was negative for pulmonary embolism but demonstrated interval left lower lobe consolidation. The patient was placed on multiple conventional ventilator modes with a set tidal volume of 400 ml and pressure support of 5 cm H2O. Despite these standard settings, large tidal volumes were observed ranging from 700 to 1,400 ml. Initial arterial blood gas (ABG) was significant for a pH of 7.61 and PaCO2 of 13.1 mmHg. Due to the significant hypocarbia, a number of ventilator tubing segments were added to increase the mechanical dead space and increase the rebreathing of exhaled CO2. In addition, whole brain radiation and treatment with rituximab were initiated. Before these interventions, the patient's neurological exam was poor. He did not follow commands or open eyes to noxious stimuli. However, the patient had intact brainstem reflexes and trace extremity movement to noxious stimuli.
CO2 was monitored with ABG and immediate response to the increase in dead space was measured with VBG. Before intervention, VBG showed pH 7.57 and PvCO2 18.4 mmHg which immediately showed signs of improvement after 1 day. Two days after the increase in dead space a follow-up ABG showed pH 7.45 and PaCO2 30.5 mmHg. Over the coming days the patient required ventilator and dead space adjustments as he was weaned off of fentanyl and midazolam to dexmedetomidine and oxycodone.
The patient required a tracheostomy due to prolonged ventilator time, but 2 weeks after the initiation of dead space therapy the patient was weaned from the ventilator with T-piece uncapped. He was off sedation and had been weaned off of opioids. The patient was transferred to the step-down unit with ABG showing pH 7.51 and PaCO2 28.5 mmHg without tachypnea. In those 2 weeks the patient's neurological exam had improved significantly, and he was awake, alert, and attending to the examiner. He followed motor commands in all extremities (distal strength better than proximal strength) and also followed commands to close eyes and stick out tongue. On discharge 1 week later (3 weeks after the increase in dead space) he was smiling and interactive with family.
Literature Review and Proposed Refractory Management
CNH is a rare neurologic complication first described by Plum and Swanson (1). Diagnostic criteria for CNH include hyperventilation that even during sleep, low PaCO2, high PaO2, and high arterial pH in the absence of drugs or metabolic causes (3). It is a diagnosis of exclusion, and its true incidence may be underestimated as its presenting signs are often attributed to other conditions which often delays diagnosis.
Several pathophysiological mechanisms have been proposed to explain CNH. In Plum and Swanson's initial report of nine comatose patients, the lesions common to the autopsied cases involved the medial pons, sparing the lateral pontine region, and the medulla oblongata. These findings led the authors to postulate that CNH is the result of an unrestrained stimulation of both the inspiratory and expiratory centers in the medulla via the lateral reticular formation in the pons and the laterally located descending neural pathways (1). However animal models in which the pontine respiratory group was disconnected from the medulla did not produce CNH (4). Bateman et al. on the basis of post-mortem neuropathological studies demonstrating brainstem sparing, further argued against the necessity of a direct destruction of the brainstem respiratory centers for the development of CNH (5).
Another hypothesis posits that the tumor may reduce local pH in the brainstem without altering overall CSF pH, resulting in hydrogen ions stimulating chemosensitive areas located in the ventral brainstem (2, 6). However, this explanation has been challenged as in vivo measurements of the pH in brain neoplasms using positron emission tomography (PET) have demonstrated that tumor pH was higher than in the rest of the brain (7). Finally, it has been proposed that the tumor microenvironment cytokines and other neuroactive chemicals may stimulate respiratory centers (2). Animal models have shown that microinjections of glutamate in the brainstem respiratory centers can induce respiration (8). Nevertheless, an unequivocal explanation has not yet been identified and there is still no clear understanding of the pathophysiologic sequence underlying CNH.
CNH is most common in comatose patients and very rare in an awake patients with only about 35 reports in the literature (3). Conversely, the patients who are alert at the onset of CNH are usually so despite very low PaCO2 concentration, suggesting that level of consciousness is more dependent on the underlying cause of the CNH rather than the degree of hypocarbia. Cancer-related CNH presents more commonly in a conscious patient. PCNSL accounts for half of such cases according to a recent review (2, 3). About 90% of PCNSLs are DLBCL and 10% are various other lymphomas such as Burkitt's lymphomas or T-cell lymphomas (9). Tumor-induced CNH usually constitutes a presenting symptom as well as an indication of the severity of the underlying disease, as it often precedes the cardiorespiratory deterioration. Prognosis in CNH and especially in CNH secondary to cancer, is usually very poor, with an overall survival after diagnosis of only a few months (3). Hypocapnia alone is unlikely responsible for the increase in mortality or morbidity, but studies of medical and surgical ICU patients with severe alkalemia has shown increased mortality with a rate of up to 80% above pH 7.65 (10, 11). Hypocapnia also leads to undesirable side effects such as impairment of tissue oxygenation (due to left shift of the oxygen dissociation), a decreased cerebral circulation, a resetting of the chemoreceptors and a compensatory distal renal acidosis (12–14).
A unique feature of DLBCL compared with other brain tumors is its unique sensitivity to steroids, although a multi-modality therapy is usually preferred due to the high probability of recurrence in patients treated solely with steroids. Hence the standard of treatment is a combination of corticosteroids, radiation therapy, and chemotherapy in order to increase overall survival and progression free survival (9). As reported above, the patient in our case showed a significant response to IV solumedrol administered shortly after diagnosis and then benefitted from 15 treatments of whole brain radiation and eight doses of weekly rituximab after the onset of CNH. As previously mentioned, treatment directed toward DLBCL ultimately resolved the patient's perverse breathing pattern; however, the patient required bridging therapy.
Management of CNH has been well-described in the literature. In brief, the cornerstone of treatment involves elucidating the underlying cause, treating it, and acutely bridging treatment with high dose sedation, whether it be benzodiazepines, propofol, or analgesia. However, herein we have identified a refractory entity; a patient presenting with an ABG showing pH of 7.61 and PaCO2 of 13.1 mmHg which did not respond to fentanyl or propofol. Very few cases have been reported with such refractoriness. One report of a patient with CNH secondary to lymphomatoid granulomatosis whose severe alkalosis was unresponsive to morphine was managed with intubation, mechanical ventilation and paralysis with pancuronium bromide (15). More recently, Jones et al. illustrated that the off-label administration of a single 2 mg/kg dose of ketamine in a patient with a sedation resistant CNH reduced hyperventilation and allowed successful extubation within 24 h (16).
Upon review, however, an increase in mechanical dead space has been scarcely acknowledged as a tool for managing hypocarbia and to our knowledge there have been no reports so far of its successful employment in the setting of CNH. The ventilator circuit is a mechanical dead space which is a frequently forgotten. Increased mechanical dead space facilitates greater rebreathing of exhaled PaCO2. Suwa et al. investigated the feasibility of restoring a low PaCO2 to normal by the addition of a mechanical dead space without changing the pattern of ventilation. Their population consisted of long-term mechanically ventilated patients, and they were able to formulate an equation to predict the increase in PaCO2 (12). Pauzner et al. mentioned in their case report a 2-h trial of breathing through increased dead space as one of the first attempts to control a resistant episode of tumor-induced CNH. Ultimately the patient responded to multiple doses of morphine that were given only after the failure of the aforementioned increase in dead space (17).
In our patient, a number of ventilator tubing segments were added to increase the mechanical dead space and increase the rebreathing of the exhaled CO2. The mechanisms involved in this are beyond the scope of this review; a simple heuristic, for every 100 mL (1 ft or 30.5 cm) of circuitry added the PaCO2 increases ~5 mmHg. We added ~6 ft of mechanical dead space to our patient's circuitry. The patient did not have an underlying lung disease. Therefore, this temporizing bridging modality was effective enough to wean the patient to 6 ft of mechanical T-piece dead space. This technique maintained a healthy pH, PaCO2, and PaO2 for ~2 weeks. As the patient's DLBCL became increasingly responsive to radiation and high dose steroids, the mechanical dead space was gradually weaned with stable ABG parameters.
The advantages of applying such a method derive from its roughly predictable results and minimal collateral effects, as well as the opportunity it may offer to administer smaller dosages of sedating pharmaceuticals in already compromised patients. These benefits and the relative low cost of this technique should warrant further investigations into the timing, sequencing, and optimal integration with the other measures employed in the management of CNH.
Conclusion
We report a patient with a central neurogenic hyperventilation secondary to a diffuse large B cell lymphoma resistant to high sedation. The refractory alkalemia was managed by treating the underlying lymphoma and bridging therapy with an increase of the mechanical dead space through the addition of ventilator tubing segments. The combination of these measures normalized the PaCO2 and pH, allowing the patient to be weaned off the ventilator. To our knowledge there have been no other successful employments of this technique in stabilizing patients during episodes of this abnormal breathing pattern.
Ethics Statement
Written informed consent was obtained from the participant/patient for both participation and publication of the case report.
Author Contributions
AS and MB: drafting, revising, and finalizing the manuscript. All others: reviewed and revised critically for important intellectual content.
Conflict of Interest Statement
The authors declare that the research was conducted in the absence of any commercial or financial relationships that could be construed as a potential conflict of interest.
Abbreviations
CNH, central neurogenic hyperventilation; DLBCL, diffuse large B-cell lymphoma; PCNSL, Primary CNS lymphoma; ABG, arterial blood gas; Neuro ICU, neurointensive care unit.
References
1. Plum F, Swanson AG. Central neurogenic hyperventilation in man. AMA Arch Neurol Psychiatry. (1959) 81:535–49. doi: 10.1001/archneurpsyc.1959.02340170001001
2. Tarulli AW, Lim C, Bui JD, Saper CB, Alexander MP. Central neurogenic hyperventilation. Arch Neurol. (2005) 62:1632–4. doi: 10.1001/archneur.62.10.1632
3. Pantelyat A, Galetta SL, Pruitt A. Central neurogenic hyperventilation: a sign of CNS lymphoma. Neurol Clin Pract. (2014) 4:474–7. doi: 10.1212/CPJ.0000000000000087
4. Bianchi AL, Denavit-Saubie M, Champagnat J. Central control of breathing in mammals: neuronal circuitry, membrane properties, and neurotransmitters. Physiol Rev. (1995) 75:1–45. doi: 10.1152/physrev.1995.75.1.1
5. Bateman DE, Gibson GJ, Hudgson P, Tomlinson BE. Central neurogenic hyperventilation in a conscious patient with a primary cerebral lymphoma. Ann Neurol. (1985) 17:402–5. doi: 10.1002/ana.410170418
6. Mulkey DK, Stornetta RL, Weston MC, Simmons JR, Parker A, Bayliss DA, et al. Respiratory control by ventral surface chemoreceptor neurons in rats. Nat Neurosci. (2004) 7:1360–9. doi: 10.1038/nn1357
7. Arnold JB, Kraig RP, Rottenberg DA. In vivo measurement of regional brain and tumor pH using [14C]dimethyloxazolidinedione and quantitative autoradiography. II: characterization of the extracellular fluid compartment using pH-sensitive microelectrodes and [14C]sucrose. J Cereb Blood Flow Metab. (1986) 6:435–40. doi: 10.1038/jcbfm.1986.76
8. Takayama K, Miura M. Respiratory responses to microinjection of excitatory amino acid agonists in ventrolateral regions of the lateral parabrachial nucleus in the cat. Brain Res. (1993) 604:217–23. doi: 10.1016/0006-8993(93)90372-T
9. Batchelor T, Loeffler JS. Primary CNS lymphoma. J Clin Oncol. (2006) 24:1281–8. doi: 10.1200/JCO.2005.04.8819
10. Tripathy S. Extreme metabolic alkalosis in intensive care. Indian J Crit Care Med. (2009) 13:217–20. doi: 10.4103/0972-5229.60175
11. Anderson LE, Henrich WL. Alkalemia-associated morbidity and mortality in medical and surgical patients. South Med J. (1987) 80:729–33. doi: 10.1097/00007611-198706000-00016
12. Suwa K, Bendixen HH. Change in PaCO2 with mechanical dead space during artificial ventilation. J Appl Physiol. (1968) 24:556–62. doi: 10.1152/jappl.1968.24.4.556
13. Ledet D, Delos Santos NM, Khan R, Gajjar A, Broniscer A. Central neurogenic hyperventilation and renal tubular acidosis in children with pontine gliomas. Neurology. (2014) 82:1099–100. doi: 10.1212/WNL.0000000000000252
14. Meng L, Gelb AW. Regulation of cerebral autoregulation by carbon dioxide. Anesthesiology. (2015) 122:196–205. doi: 10.1097/ALN.0000000000000506
15. Sunderrajan EV, Passamonte PM. Lymphomatoid granulomatosis presenting as central neurogenic hyperventilation. Chest. (1984) 86:634–6. doi: 10.1378/chest.86.4.634
16. Jones GM, Wiss AL, Goyal N, Chang JJ. Successful use of ketamine for central neurogenic hyperventilation: a case report. Neurohospitalist. (2017) 7:192–5. doi: 10.1177/1941874417697025
Keywords: mechanical ventilation, lymphoma, central neurogenic hyperventilation, neuro-oncology, neurocritical care, critical care
Citation: Sweidan AJ, Bower MM, Paullus J, Sterpi M, Stern-Nezer S, Dastur C, Yu W and Groysman LI (2019) Refractory Central Neurogenic Hyperventilation: A Novel Approach Utilizing Mechanical Dead Space. Front. Neurol. 10:937. doi: 10.3389/fneur.2019.00937
Received: 15 March 2019; Accepted: 12 August 2019;
Published: 04 September 2019.
Edited by:
Fernando Goldenberg, The University of Chicago Pritzker School of Medicine, United StatesReviewed by:
Benjamin Aaron Emanuel, The Keck School of Medicine at University of Southern California, United StatesMinjee Kim, Northwestern University, United States
Copyright © 2019 Sweidan, Bower, Paullus, Sterpi, Stern-Nezer, Dastur, Yu and Groysman. This is an open-access article distributed under the terms of the Creative Commons Attribution License (CC BY). The use, distribution or reproduction in other forums is permitted, provided the original author(s) and the copyright owner(s) are credited and that the original publication in this journal is cited, in accordance with accepted academic practice. No use, distribution or reproduction is permitted which does not comply with these terms.
*Correspondence: Matthew M. Bower, Ym93ZXJtMUB1Y2kuZWR1