- 1INSERM UMR 1105, Research Group on Multimodal Analysis of Brain Function, University of Picardie Jules Verne, Amiens, France
- 2INSERM UMR 1105, EFSN Pediatric, Amiens University Hospital, Amiens, France
Epilepsy with Centrotemporal Spikes (ECTS) is the most common form of self-limited focal epilepsy. The pathophysiological mechanisms by which ECTS induces neuropsychological impairment in 15–30% of affected children remain unclear. The objective of this study is to review the current state of knowledge concerning the brain structural and functional changes that may be involved in cognitive dysfunctions in ECTS. Structural brain imaging suggests the presence of subtle neurodevelopmental changes over the epileptogenic zone and over distant regions in ECTS. This structural remodeling likely occurs prior to the diagnosis and evolves over time, especially in patients with cognitive impairment, suggesting that the epileptogenic processes might interfere with the dynamics of the brain development and/or the normal maturation processes. Functional brain imaging demonstrates profound disorganization accentuated by interictal epileptic spikes (IES) in the epileptogenic zone and in remote networks in ECTS. Over the epileptogenic zone, the literature demonstrates changes in term of neuronal activity and synchronization, which are effective several hundred milliseconds before the IES. In the same time window, functional changes are also observed in bilateral distant networks, notably in the frontal and temporal lobes. Effective connectivity demonstrates that the epileptogenic zone constitutes the key area at the origin of IES propagation toward distant cortical regions, including frontal areas. Altogether, structural and functional network disorganizations, in terms of: (i) power spectral values, (ii) functional and effective connectivity, are likely to participate in the cognitive impairment commonly reported in children with ECTS. These results suggest a central and causal role of network disorganizations related to IES in the neuropsychological impairment described in ECTS children.
Highlights
- The epileptogenic zone and distant areas are structurally/functionally disorganized
- The functional disorganization is accentuated in the presence of IES on scalp EEG
- Distant desynchronization begins before IES
- A causal role of IES in ECTS neuropsychological impairment is highly suggested.
Introduction
According to the international classification of epilepsy (1), Epilepsy with CentroTemporal Spikes (ECTS) is the most common form of self-limited, drug-responsive, focal epilepsy (2–4). ECTS accounts for 13 to 23% of all cases of new-onset epilepsy in children (5–8) with an incidence of about 10–20/100 000 children under the age of 13 years (3, 8). The peak age of onset of ECTS is 7 to 8 years and ECTS is slightly more common in boys, with a sex ratio of 60:40 (9).
Clinical and Electroencephalographic Features of Typical ECTS
Diagnostic criteria are characterized by onset in childhood, absence of brain lesion on MRI neuroimaging, normal initial psychomotor development, normal pregnancy and delivery, sensorimotor seizures and focal centrotemporal or rolandic spikes and waves activated with sleep on inter-ictal EEG recording (2).
Neurological evaluation and cognitive development are normal, but cognitive impairments such as attention deficiency, visuomotor coordination impairment and specific learning disabilities have been described (see below).
Onset of seizures is usually between the ages of 4 and 10 years, and always before the age of 13 years (10). Seizures resolve by the age of 13 years. More than 90% of children experience active epilepsy for about 3 years (11). Seizures are uncommon, as more than 90% of children experience fewer than 5 seizures (12, 13) and they are usually closely related to sleep (55%) or appear during wakefulness or drowsiness (9). Only 30% of children experience seizures during wakefulness (2, 9). Eighty percent of seizures are focal with inconstant secondary generalization (13). Focal seizures involve sensorimotor areas. Motor signs affect the face (37%) and oropharyngeal muscles (53%), including guttural sounds, mouth movements, dysarthria and clonic jerks (40%) and may be preceded by sensory sensations such as hemifacial, perioral or intraoral paraesthesia, and jaw and tongue stiffness (13). The upper limb (20%) and rarely the lower limb (8%) are less frequently affected (13). Generalized seizures have been reported in 24–80% of patients (9, 13), but probably correspond to secondary generalization of focal seizures. Seizures usually last only a few minutes, and always, less than half an hour (9).
In typical ECTS, standard EEG shows centrotemporal spikes on normal background activity (8). Interictal Epileptic Spikes (IES) may be either isolated or occur in clusters and exhibit a high voltage biphasic wave with centrotemporal negativity followed by a positive frontal dipole (14–16). IES can be unilateral (60%) or bilateral (40%), synchronous or asynchronous between the two hemispheres (15, 17) and lateralization may change between different EEGs in the same patient. IES are frequently activated during non-REM sleep (15, 18–20) and diffuse in the ipsilateral and contralateral hemispheres (21). About 30% of patients only present spikes during sleep (18) EEG abnormalities resolve at puberty, but frequently persist after the period of seizures (11).
Typical ECTS in the Spectrum of Rolandic Epilepsy
Typical ECTS belongs to the spectrum of rolandic epilepsy, which encompasses typical ECTS, Atypical Benign Partial Epilepsy (ABPE), Landau-Kleffner syndrome (LKS) and epileptic encephalopathy with Continuous Spikes and Waves during slow-wave Sleep (CSWS) (22).
ABPE is defined by atypical seizures (long partial or generalized motor, atonic seizures or negative myoclonus, atypical absences, perioral myoclonia) (23, 24) associated with peri-ictal symptoms, such as transient oro-motor dysfunction. Atypical patterns are observed on EEG, characterized by an intermittent slow-wave focus, multiple asynchrony spike-wave foci, long spike-wave clusters, and “absence-like” spike-wave discharges (25, 26). LKS and CSWS share several common features with typical ECTS: onset of epilepsy during childhood, remission before the end of adolescence, rare seizures, and normal brain MRI. EEG abnormalities are associated with deterioration of cognitive functions that were previously normally acquired. LKS is defined by acquired epileptic aphasia with verbal auditory agnosia and behavioral disorders associated with a marked increase of IES during sleep, predominantly in the temporal areas. CSWS is characterized by continuous spike-and-wave discharges during slow-wave sleep, usually combined with global intellectual deterioration and epileptic seizures (27).
Typical ECTS and rolandic epilepsy spectrum are believed to represent a continuum of different clinical phenotypes of self-limited focal epilepsy (28) but some authors consider them to be fundamentally distinct conditions (9, 15, 29–31).
Genetic Origin of Typical ECTS
The genetic origin of ECTS was first suggested on the basis of clinical observations (32) comparing the incidence of ECTS in twins and families with sporadic ECTS (33–37). However, the direct causal role of monogenic mutations in typical ECTS has not been clearly demonstrated. In contrast with LKS and CSWS (38–42), monogenic mutations including GRIN2A (38, 39, 42), KCNQ2, KCNQ3 or duplications in PRRT2 gene (35, 43–48) have been detected in only a small proportion of children with typical ECTS. ECTS has therefore been attributed to a complex interplay between brain development, maturation processes and gene susceptibility with no evidence, at the present time, that genetic factors are paramount (49, 50).
Neuropsychological Impairment in Typical ECTS
Normal cognition was previously considered to be a prerequisite for the diagnosis of ECTS, which is why this form of epilepsy was initially called Benign Childhood Epilepsy with Centro-Temporal Spikes (BCECTS) (ILAE, 1985; ILAE, 1989). However, ~15–30% of affected children show some degree of neuropsychological impairment, including disorders of language processing (51–55), attention (56–58), and executive functions (59), verbal function (52, 60–64), short-term (52, 54), and working memories (65), psychiatric status and general cognitive functioning (66, 67). These neuropsychological impairments impact academic achievement as a result of specific learning disabilities (62, 68, 69).
The pathophysiological mechanisms by which ECTS induces neuropsychological impairment remain unclear. The neuropsychological impairments are not related to seizure characteristics (26) and are observed even in children with well-controlled seizures (70–72). The impact of antiepileptic drugs has not been clearly determined (73). The role of IES has been suggested but remains debated. The rate of occurrence of IES in ECTS does not appear to be sufficient to predict the individual cognitive outcome (26, 58, 62, 68, 73). The morphology of interictal abnormalities appears to be predictive of neurocognitive and clinical outcomes, as combinations of various interictal EEG parameters (slow wave focus, multiple asynchronous spike and wave foci, long rhythmic spikes and clusters, generalized spike and wave discharges, abundance of interictal abnormalities during sleep and wakefulness, persisting for several months) precede clinical worsening, but these parameters are considered to be diagnostic criteria for ABPE (26). The localization of interictal discharges might be partly related to the type of cognitive deficit (74). Nevertheless, the correlation between IES localization on the EEG and the neuropsychological profile of children with ECTS has yet to be clearly defined (75, 76).
Despite the large number of studies conducted since the first description of ECTS by Nayrac and Beaussart (77), the pathophysiological mechanisms that participate in ECTS and relative neurocognitive impairment have yet to be defined.
The objective of this study is to review the functional and structural disorganizations in the epileptogenic zone, but also in remote areas, that may be involved in cognitive dysfunctions in patients with typical ECTS. Before addressing these disorganizations, we will review the current state of knowledge concerning the epileptogenic zone responsible for structural and functional interactions with distant regions.
The Epileptogenic Zone and the Spike Onset Zone in ECTS
The epileptogenic zone is defined by the site of onset of epileptic seizures and their primary organization (78). Due to the rarity of seizures and the absence of intracranial recordings, the epileptogenic zone is difficult to localize in ECTS. In rare ictal scalp EEG recordings (25, 79–82), the onset of focal seizures was predominantly observed in the centro-temporal region (82), and occasionally in the parietal region (25) (Figure 1A). Despite small differences according to the epileptogenic zone, all EEG recordings show a similar pattern characterized by low amplitude focal alpha-beta activity (9–14 Hz), gradually increasing in amplitude and decreasing in frequency to the alpha band (6–8 Hz), followed by rapid rhythmic spikes (79). This ictal pattern mostly occurs after an increment of interictal spikes (79) together with a reversal of the IES dipole (81). An ictal electrical source imaging study also demonstrated an operculo-insular origin of the initial rhythmic ictal activity (79).
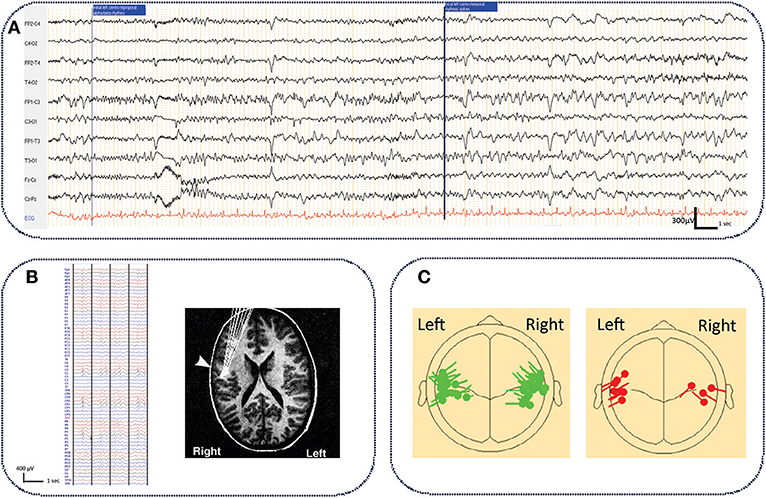
Figure 1. Typical ictal aspect on standard EEG in ECTS and electrical source imaging of IES in typical and atypical ECTS. (A) Typical aspect of ECTS seizure characterized by initial focal low amplitude alpha-beta activity (9–14 Hz), gradually increasing in amplitude and decreasing in frequency to the alpha band (6–8 Hz), followed by rapid rhythmic spikes. Longitudinal bipolar montage, electrodes positioned according to the international 10/20 system. Band pass (0.53–70 Hz), notch filter (50 Hz) (personal data). (B) Typical right centro-temporal IES on normal background activity recorded with HD EEG [averaging referential montage, band pass (0.53–70Hz)] and electrical source imaging (HD EEG) of IES with tangential dipoles located along the central sulcus with the negative pole situated in the posterior mid-temporal or central regions [adapted with authorization from Bourel-Ponchelet al. (83)]. (C) Comparison of typical (in green) and atypical generator (in red) of IES in ECTS and Atypical Benign Partial Epilepsy, respectively. Compared to ECTS, in Atypical Benign Partial Epilepsy, IES dipoles have a preferential posterior orientation [reproduced with authorization from Kim et al. (84). Averaged EEG spike dipole analysis may predict atypical outcome in Benign Childhood Epilepsy with Centrotemporal Spikes (BCECTS). Brain Dev. 38, 903–908].
The spike onset zone is defined by the site of generation of the IES. The epileptogenic zone is usually included in the spike onset zone (78). In ECTS, as in all forms of focal epilepsy, the epileptogenic zone could be approximated by the spike onset zone.
Studies using voltage gradient measures applied to IES in ECTS have defined the spike onset zone in the mid-centro-temporal region (85). Using Electrical Source Imaging, the source of the IES has been modeled by a single tangential dipole located along the central sulcus. This dipole is oriented tangentially to the surface of the scalp (86–88). The negative pole is situated in the posterior mid-temporal or central regions and a positive pole is centered more anteriorly, involving frontal areas (83, 88–90) (Figure 1B). Although the posterior side of the central sulcus was initially believed to generate the IES (90, 91), recent studies have proposed that the IES originate from the rolandic fissure of the somatosensory cortex (area 3b) (88–90, 92). More precisely, superior finger/hand areas have been proposed as a possible origin of IES, which may then propagate and extend along the precentral sulcus to the mouth/tongue area (93). Atypical generator characteristics have been proposed as criteria to help clinicians to rapidly discriminate typical ECTS from ABPE in individual patients (Figure 1C) (84, 94, 95). In these atypical generators, the dipoles have a preferential posterior orientation (84). Source locations are not tightly clustered around the central sulcus (95) and could be located around the rolandic sylvian regions, involving the motor cortex (91, 96) or even more lateral and inferior areas than those reported in typical ECTS (86).
Structural Disorganization in ECTS
As ECTS is defined as an idiopathic or self-limited epilepsy syndrome, no MRI abnormalities are expected. However, subtle structural abnormalities have been described in the epileptogenic zone and in distant brain structures, suggesting that the epileptogenic processes interfere with normal brain development and maturation.
During normal neurodevelopment and under physiological conditions, the gray matter volume increases until preadolescence and decreases thereafter, whereas the volume of white matter increases until adulthood (97–99). Synaptic exuberance and pruning are thought to contribute to these morphological changes (100, 101). Post-natal brain development is associated with the generation of excess neuronal synapses. In immature animals, the number of axonal branches, development of the dendritic spine and synaptic connections exceed those observed in mature animals. Connections may exist between parts of the brain that are not interconnected in mature animals. The generation of excess neuronal synapses is followed by decline until adulthood throughout the cerebral cortex (102–106). This selective elimination of synapses, called pruning, depends on synaptic activity and promote the maintenance of more active synapses, while removing less active synapses. Pruning is thought to be necessary to refine the emerging brain circuitry (107–110).
ECTS could impact this ongoing neurodevelopment in various ways. In newly diagnosed ECTS, neuroimaging studies have revealed differences (increases or decreases) in the thickness of the cortex (Table 1). These changes are not specifically observed in the central epileptogenic zone, as they have been observed in distant areas, notably in the frontal and temporal regions (112–115, 118) and in subcortical structures, especially the putamen (111, 114, 117). ECTS children also demonstrate white matter abnormalities [reduced fractional anisotropy (FA)] over the central epileptogenic zone (120) and over distant regions (the splenium of the ipsilateral corpus callosum) (113, 121). These differences in brain volume and brain structural connectivity suggest the presence of subtle neurodevelopmental changes between typical development in normal children and those with ECTS. This structural remodeling likely occurs prior to the diagnosis of ECTS following onset of the first seizure (61, 115, 118, 119).
Some studies have reported that this structural remodeling evolves over time (61, 115, 118, 119), as, during the active phase of ECTS (persistence of seizures and IES on interictal EEG), differences in cortical and subcortical thickness have been progressively observed compared to healthy age-matched controls, especially in patients with cognitive impairment (111, 115, 118, 120, 122) (Table 1). Following ECTS remission (115, 119), changes in gray matter growth, notably in the frontal lobe, tend to resolve, with more rapid recovery in ECTS patients with a shorter active seizure period (123) (Table 1). The epileptogenic processes related to synaptic hyperactivity and/or alterations of physiological pruning might affect the dynamics of the brain development and/or might interfere with the normal maturation processes (61, 109, 115, 118–120, 122, 124, 125).
Functional correlations between structural remodeling and cognitive deficits have been recently addressed (61, 113, 116, 119). A correlation between ADHD in ECTS children and structural impairment in the cortico-striatal circuitry has been inconsistently reported (113, 116). Similarly, a decrease in frontal and pre-frontal gray matter volume was more pronounced in ECTS with cognitive impairment and behavioral issues (119) (Table 1).
Functional Disorganization: From the Epileptogenic Zone to Distant Networks
Functional Disorganization in the Epileptogenic Zone
As expected, an increase in θ, α, and ß power spectra and cortical source densities (sensor space) (126) has been observed in the epileptogenic zone over the centro-temporal areas in the presence of IES (Figure 2). This increase in power spectrum over the centro-temporal areas was accompanied by higher phase synchronization when comparing EEG segments with and without IES in ECTS children (126, 128). In parallel, High frequency Oscillations (HFOs) (50–250 Hz) superimposed on top of IES were described in typical and atypical ECTS (129–131). The hypersynchronization due to IES is associated with increased local hemodynamic changes, as demonstrated by EEG-functional MRI (132–135) and EEG-functional Near InfraRed Spectroscopy (EEG-fNIRS) (136) (Figure 3A).
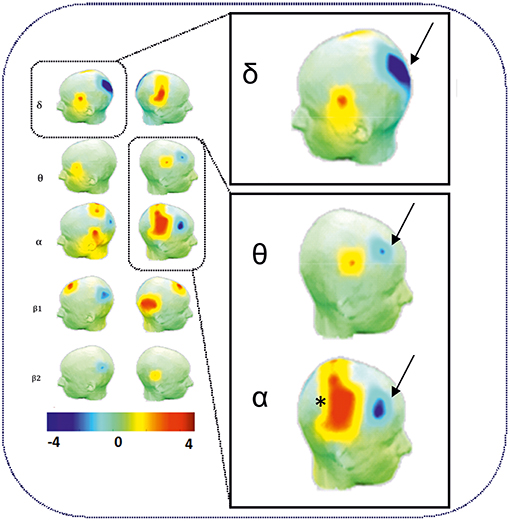
Figure 2. Power spectrum analysis of functional disorganization in the epileptogenic zone in remote regions. (Right Top) Increase in δ, θ, and α power spectra (arrows) in the epileptogenic zone over the centro-temporal areas in the presence of IES. (Right Bottom) Relative decrease in power value (arrows) in θ and α frequency bands in frontal areas and in δ bands in occipital areas. Statistical difference (t-value) maps of degree differences between HD EEG segments with IES and HD EEG segments without IES. The color bar indicates the t values projected onto a standardized head shape. The significant increase (indicated by red) and decrease (indicated by blue) in degree have been represented by positive and negative t values resulted from statistical comparisons between IES condition and no IES condition [adapted from Adebimpe et al. (127), reproduced under the Creative Commons CC-BY license] (126).
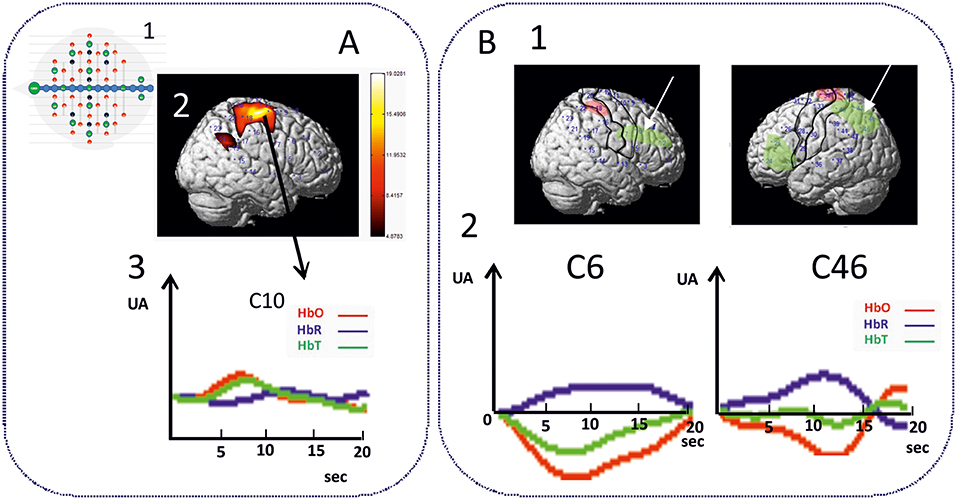
Figure 3. Hemodynamic response of functional disorganization in the epileptogenic zone (A) in remote regions (B). A1 High density EEG-fNIRS drawing of the bimodal EEG-fNIRS cap with optodes (detectors, green; emitters, red) and electrodes (black) positions. Twenty three channels per hemispheres have been considered corresponding to distances between emitting and detecting fibers (1.5 to 4 cm). A2 Hemodynamic responses related to right centro-temporal IES in a patient with typical ECTS. Statistical map of the hemodynamic response using a typical Hemodynamic Response Function (p < 0.05) and (3) Traces of the hemodynamic response after averaging (0-20 seconds) of the related channel 10 (C10). IES in ECTS are associated with a typical positive hemodynamic response, as demonstrated by EEG-fNIRS in the centro-temporal area [adapted from Bourel-Ponchel et al. [130]]. B1 Positive and negative neurovascular coupling areas (in red and green, respectively) calculated from a typical Hemodynamic Response Function (HRF) (p < 0.05). B2 Negative neurovascular coupling obtained with averaging method (range considered 0–20 s) in right frontal and left parieto-occipital areas (arrows). High density optical imaging found increase in HbR and decrease in HbO (corresponding to a negative BOLD) in the same regions (bi-frontal areas and left occipital areas) (136). x Axis: time range between 0 and 20 s (interval between vertical bars: 5 sec). y Axis: arbitrary unit (UA): relative concentrations variations related to IES. Red curve: HbO, Blue curve: HbR, Green curve: HbT.
However, IES can no longer be simply considered to be due to hypersynchronization of a single homogeneous population of neurons. Using time-frequency analysis, complex sequences of desynchronization-synchronization-desynchronization have been demonstrated surrounding the IES in ECTS (83) (Figure 4A). These sequences began 400 ms before the IES, suggesting complex disorganization of the network in the epileptogenic zone starting before the onset of the neuronal network process of the IES as recorded by EEG. These phenomena are not specific to ECTS, as they have also been described in animal models of epilepsy (137) and in non-idiopathic frontal epilepsy in children (138) and could be explained by various patterns of neuronal activation and deactivation occurring 400 ms before hypersynchronization (139). Interestingly, these swings in synchronization are observed in parallel with swings in membrane properties observed on Fast Optical Signal (FOS) recordings in animals and children with frontal epilepsy, which might result in sequences of shrinking, swelling and shrinking of the neuronal population involved in the IES process. These non-synaptic events around the IES likely modify the extracellular space and consequently the bioavailability of neurotransmitters that propels neurons to hypersynchronization (137, 138).
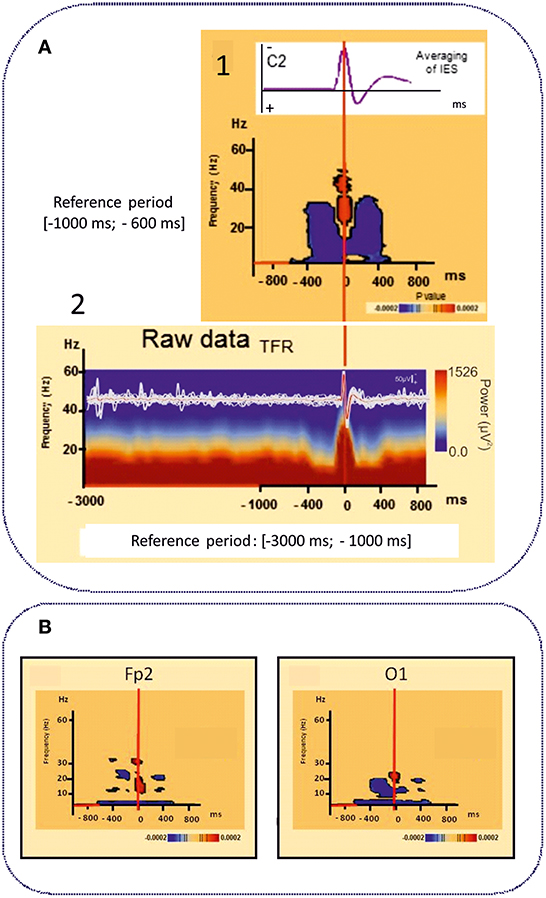
Figure 4. Time frequency analyses of functional disorganization in the epileptogenic zone (A) in in remote regions (B). A1 Averaging of the selected right centro-temporal IES in typical ECTS at electrode C2 [HD EEG, 64 electrodes positioned according to the 10/10 international system, band pass (0.53 −15 Hz), notch filter (50 Hz)]. Bellow, Time frequency statistical analysis at electrode C2 for the same patient [reference period (−1000; −600 ms), (p < 0.0002)]. A2 Raw data of the time frequency analysis [Reference period: (−3000 ms −1000 ms)] for the same patient. T0 was defined as the first negative deflexion of the spike. Time-frequency analysis revealed complex sequences of desynchronization (in blue) -synchronization (in red) -desynchronization (in blue) surrounding the IES in ECTS for frequencies range from 4 to 50 Hz independently of the baseline considered [−1000; −600 ms] (1) or [−3000 ms; −1000 ms]. This desynchronization was localized near the epileptogenic zone [adapted from Bourel-Ponchel et al. (83), reproduced under the Creative Commons CC-BY license] (83). (B) Time frequency representation of desynchronizations (in blue) occurring, in frontal and occipital areas, distant to the epileptogenic zone located in central area, involving low frequency bands (bellow 10 Hz), in the same time window as local alternation of synchrony around the IES (Figure 2) [−400 ms; +400 ms]. Significant statistical (p < 0.0002) results of time frequency analysis at electrodes Fp2 and O1 for the same patient (reference period [−1000; −600 ms] [adapted from Bourel-Ponchel et al. (83)], reproduced under the Creative Commons CC-BY license) (83).
Higher relative EEG power in the θ, α, and ß bands is still observed in the centro-temporal area regardless of the presence of IES in the EEG segments (126). Profound changes in terms of spectral power (126), phase synchronization (140), network degree and clustering coefficient (127, 141, 142) are observed simultaneously in the epileptogenic zone, independently of the presence of IES on EEG (Figure 2).
Altogether, these results indicate profound disorganization of the epileptogenic zone, not only in the time-window around the IES, but also during the period during which IES are not detected. IES are transient biomarkers of the local complex disorganization that is consubstantial to the epileptic network in ECTS. This disorganization is effective several hundred milliseconds before the IES (83) and consists of aberrant local synchronization/desynchronization and higher levels of neuronal activity (142–144) that do not always reach the threshold of an IES recorded on the scalp EEG.
From the Epileptogenic Zone to Distant Networks
Involvement of Distant Areas
Functional MRI and HD-EEG studies in the interictal state, regardless of the presence of IES, have demonstrated profound changes of the spectral power in distant areas to the spike onset zone (Figures 2, 4B). Desynchronization in the α and ß bands observed in bilateral frontal and parieto-occipital areas, suggests disengagement of the frontal and parieto-occipital cortices (127, 140, 142). Using fNIRS and fMRI (132, 133, 136), the hemodynamic response to IES (decrease in HbO, increase in HbR) in distant areas, including the frontal and parieto-occipital lobes, confirmed the widespread effect of IES on remote networks (Figure 3B).
Functional Connectivity
Functional connectivity measurements have been used to study functional alteration of the brain networks during the interictal state.
In the human brain, inhibitory and excitatory circuits interact by integrating information at local and global levels. It has been shown that the normal brain has a small-world functional topology, which can efficiently combine functionally specialized (segregated) modules with intermodular links (integrating). This type of organization reflects an optimal balance between functional integration and segregation (145, 146). This small-world functional topology is disrupted in ECTS.
A higher connection density around the epileptogenic zone, including the motor areas, the central region and the ipsilateral temporal region, has been described in all frequency bands (126). Functional connectivity using Lagged Phase Synchronization (LPS) analysis revealed higher θ and α and lower β LPS values (141). Functional connectivity changes around the IES were associated with lower segregation and higher integration, notably in the θ and α bands, compared to controls, suggesting that the ECTS brain network differs from the small-world features observed in healthy controls in a frequency-dependent manner. Loss of global processing and stronger integration have been observed in the epileptogenic zone, but also between remote brain regions, notably the frontal, parietal and temporal lobes (126, 127, 140–142, 147). These results indicate that the ECTS brain networks are less well-organized regardless of the presence or absence of IES and regardless of the frequency band, corresponding to alteration of global small-world properties toward a more random network (126).
Dynamics and Directionality of Interictal Connectivity
The direction of information flow between the various brain regions has also been studied in ECTS children using effective connectivity analysis. Tools such as Dynamic Causal Modeling (DCM) can be used to measure the effective connectivity between selected brain regions (central epileptic region, temporo-parietal junction, temporal pole and precuneus) (148). This study showed that central rolandic regions constitute the key zone of origin of IES propagation in ECTS. DCM analysis of the direction of flow of information between brain regions from a causal perspective showed that the central epileptogenic zone exerts a greater causal influence on ipsilateral and contralateral distant cortical areas including the prefrontal cortex, temporo-parietal junction and temporal pole (148, 149). In other words, changes in distant cortical regions, notably at the frontal and temporo-parietal junction and in the temporal pole, are driven by the epileptogenic zone (148, 150). Moreover, in an fMRI study, Wu et al. showed, in addition to the driving effect from the epileptogenic zone to cortical areas, a causal link from the epileptogenic zone to subcortical structures (bilateral putamen, caudate nuclei) and cerebellum (149).
Functional changes in terms of power spectral values, functional and effective connectivity and small-world properties can participate in the cognitive impairments commonly reported in children with ECTS. The effect of pathological epileptic HFO on cognitive function needs also to be considered.
The epileptogenic zone induces functional changes in the frontal lobes, which play a major role in the processing and execution of higher cognitive skills and behavior (151). The centro-frontal pathway is altered in ECTS patients with an abnormal information flow arising from central areas during IES. Frontal deactivation and disturbances of connectivity are correlated with cognitive performances (140) and might explain learning, memory and attention difficulties in ECTS patients (73, 152–155). The causal link from the central to the frontal areas constitutes a source of abnormal information flow onto the frontal structures, suggesting that IES may play a role in alteration of the attention network (148). In addition to functional changes involving the centro-frontal network, decreased functional connectivity in the dorsal attention network (intraparietal sulcus and frontal eye fields of each hemisphere) associated with increased functional connectivity in the ventral attention network (temporo-parietal junction and ventral frontal cortex) might also be responsible for the attention deficit observed in ECTS (155). Functional connectivity disorders with the right inferior temporal cortex and bilateral primary auditory cortex may affect auditory processing in both hemispheres, resulting in language processing and speech processing deficits in ECTS patients (54, 141, 147, 156–158). The temporo-parieto-occipital areas are believed to be involved in auditory, visual, somatosensory and memory processes that can be impaired in ECTS children (142, 143). Finally, the reduced degree in occipital areas may explain the poor visuospatial memory observed in ECTS children (126, 159).
Cognition and Brain Maturation in Children With ECTS
In summary, the available literature demonstrates the existence of:
1) Complex functional disorganizations in the epileptogenic zone that are accentuated in the presence of IES on scalp EEG
2) Complex functional changes in terms of activity and synchronization in bilateral distant networks that are accentuated in the presence of IES on scalp EEG
3) Complex functional changes in distant areas beginning around 400 ms before the IES in the same time window as complex modifications of synchronization around IES (-400 ms; +400 ms) in the epileptogenic area
4) Complex functional connectivity changes including small-world network impairment
5) Impaired structural brain maturation in children with ECTS with no specificity for the epileptogenic area.
Altogether, these results suggest a central and causal role of the functional and structural disorganizations of the neuronal network that promote the IES in the neuropsychological impairment described in children with ECTS. Two non-exclusive hypotheses can be proposed to explain the pathophysiological mechanisms of the neuropsychological impairment. First, disorganizations related to IES might directly induce transient inhibition of the networks. Second, disorganizations related to IES might induce long-lasting effects on brain functioning and maturation.
Disorganizations of the Neuronal Network Related to IES-Induced Transient Network Inhibitions
This hypothesis is supported by clinical observations of transient cognitive impairment (TCI). Transient cognitive impairment (TCI) (160, 161) is characterized by brief temporary deficits of attention, visuospatial memory and learning strategies related to IES on EEG. TCI is observed during the active phase of ECTS and resolves after resolution of the EEG abnormalities (62, 162, 163). TCI is considered to be the direct consequence of IES on neuronal processing (74, 132, 158, 164, 165). TCI is likely related to transient disruption of brain function occurring around the IES in the epileptogenic zone and in distant functional networks. Time-frequency analyses have demonstrated that interferences in the epileptogenic areas and distant areas start 400 ms before IES (83). Interestingly, TCI started 100–200 ms before the IES (166) and can last for up to 2 s following a focal IES (160, 167–169), suggesting a direct link between complex changes in terms of synchronization around IES and TCI.
Disorganizations of the Neuronal Network Related to IES Induces Long-Lasting Effects on Brain Functioning and Maturation
In addition to the short-lasting effects, network disorganizations related to IES may have long-term effects on developing neural networks (170, 171).
This abnormal brain neurodevelopment could be related to reports that certain neuropsychological deficits persist despite return to a normal EEG and clinical remission (62, 162, 163), suggesting cumulative effects of IES on functional maturation of neuronal networks (149, 172–176). The early onset, at a critical period of maturation and the duration of active epilepsy should be considered when assessing the functional outcome and cognitive prognosis. Similarly, the potentiation of IES during NREM sleep needs to be considered. The degree of cognitive impairment is correlated to the amount of NREM sleep-related IES and associated with the involvement of a widespread cortico-subcortical network related to IES during (177). In addition, the rate of pathological epileptic HFO during NREM sleep seemed to be a marker of epilepsy severity. HFOs rate is higher in atypical BECTS and Electrical status epilepticus in sleep. Absence of HFO may predict a relative benign clinical entity, whereas in the presence of several ripples, the child is likely to have more seizures (129–131, 178).
Structural and functional disorganizations not related to IES but participating in neurocognitive impairment in ECTS should not be excluded.
Conclusion
The complexity of the interactions between the epileptogenic network and remote functional networks has been demonstrated in ECTS. Structural brain imaging suggests the presence of subtle neurodevelopmental changes over the epileptogenic zone and over distant regions in ECTS. Functional brain imaging demonstrates profound disorganization accentuated by IES in the epileptogenic zone which are effective several hundred milliseconds before the IES. In the same time window, desynchronization and changes in neuronal activity are observed in bilateral distant networks. These functional changes are associated with the alteration of global small-world properties toward a more random network; the direction of information flow between the brain regions demonstrates that the epileptogenic zone constitutes the key area at the origin of IES propagation toward distant cortical regions.
Altogether, these results support the idea that disorganizations of the neuronal network related to IES may disrupt local and distant networks with a possible impact on functional and the maturational processes. If the impact of IES on cognitive impairments remains debated, these results suggest a central and causal role of network disorganizations related to IES in the neuropsychological impairment described in ECTS children. These results suggest that therapeutic suppression of IES should reduce the risk of neuropsychological impairment in children with ECTS. However, the currently available antiepileptic drugs are not suitable and, specific treatments for disorganizations of the neuronal network related to IES need to be developed.
Author Contributions
EB-P, MM, AA, and FW conceived and designed the review, read and accepted the manuscript. EB-P, MM, FW wrote the paper.
Funding
This work was partially supported the the local PHRC CHU Amiens, N°2009-A00974-53.
Conflict of Interest Statement
The authors declare that the research was conducted in the absence of any commercial or financial relationships that could be construed as a potential conflict of interest.
Abbreviations
ABPE, Atypical Benign Partial Epilepsy; BCECTS, Benign Childhood Epilepsy with Centro-Temporal Spikes; CSWS, epileptic encephalopathy with Continuous Spikes and Waves during slow-wave Sleep; DCM, Dynamic Causal Modeling; ECTS, Epilepsy with Centrotemporal Spikes; FA, Fractional Anisotropy; HD EEG, High Density Electroencephalography; HFO, High frequency Oscillations; IES, Interictal Epileptic Spikes; LKS, Landau-Kleffner Syndrome; LPS, Lagged Phase Synchronization; MRI, Magnetic Resonance Imaging; fNIRS, functional Near InfraRed Spectroscopy; REM, Rapid Eyes Movements; TCI, Transient Cognitive Impairment.
References
1. Berg AT, Berkovic SF, Brodie MJ, Buchhalter J, Cross JH, van Emde Boas W, et al. Revised terminology and concepts for organization of seizures and epilepsies: report of the ILAE commission on classification and terminology, 2005-2009. Epilepsia. (2010) 51:676–85. doi: 10.1111/j.1528-1167.2010.02522.x
2. Beaussart M. Benign epilepsy of children with rolandic (centro-temporal) paroxysmal foci. A clinical entity. Study of 221 cases. Epilepsia. (1972) 13:795–811.
3. Larsson K, Eeg-Olofsson O. A population based study of epilepsy in children from a Swedish county. Eur J Paediatr Neurol. (2006) 10:107–13. doi: 10.1016/j.ejpn.2006.02.005
4. Scheffer IE, Berkovic S, Capovilla G, Connolly MB, French J, Guilhoto L, et al. ILAE classification of the epilepsies: position paper of the ILAE commission for classification and terminology. Epilepsia. (2017) 58:512–21. doi: 10.1111/epi.13709
5. Cavazzuti GB. Epidemiology of different types of epilepsy in school age children of Modena, Italy. Epilepsia. (1980) 21:57–62.
6. Chahine LM, Mikati MA. Benign pediatric localization-related epilepsies. Epileptic Disord. (2006) 8:243–58.
7. Eriksson KJ, Koivikko MJ. Prevalence, classification, and severity of epilepsy and epileptic syndromes in children. Epilepsia. (1997) 38:1275–82.
8. Heijbel J, Blom S, Bergfors PG. Benign epilepsy of children with centrotemporal EEG foci. A study of incidence rate in outpatient care. Epilepsia. (1975) 16:657–64.
9. Wirrell EC. Benign epilepsy of childhood with centrotemporal spikes. Epilepsia. (1998) 39 (Suppl 4):S32–41.
10. Beaumanoir A, Ballis T, Varfis G, Ansari K. Benign epilepsy of childhood with Rolandic spikes. A clinical, electroencephalographic, and telencephalographic study. Epilepsia. (1974) 15:301–15.
11. Bouma PA, Bovenkerk AC, Westendorp RG, Brouwer OF. The course of benign partial epilepsy of childhood with centrotemporal spikes: a meta-analysis. Neurology. (1997) 48:430–7.
12. Lerman P, Kivity S. Benign focal epilepsy of childhood. A follow-up study of 100 recovered patients. Arch. Neurol. (1975) 32:261–4.
13. Loiseau P, Beaussart M. The seizures of benign childhood epilepsy with Rolandic paroxysmal discharges. Epilepsia. (1973) 14:381–9.
14. Graf M, Lischka A, Gremel K. [Benign Rolandic epilepsy in children. Topographic EEG analysis] Wien Klin Wochenschr. (1990) 102:206–10.
15. Holmes GL. Rolandic epilepsy: clinical and electroencephalographic features. Epilepsy Res Suppl. (1992) 6:29–43.
16. Wong PK, Bencivenga R, Gregory D. Statistical classification of spikes in benign rolandic epilepsy. Brain Topogr. (1988) 1:123–9.
17. Loddenkemper T, Burgess RC, Syed T, Pestana EM. Lateralization of interictal EEG findings. J Clin Neurophysiol. (2007) 24:379–85. doi: 10.1097/WNP.0b013e31815607cc.
18. Blom S, Heijbel J. Benign epilepsy of children with centro-temporal EEG foci. Discharge rate during sleep. Epilepsia. (1975) 16:133–40.
19. Dalla Bernardina B, Pajno-Ferrara F, Beghini G. Proceedings: rolandic spike activation during sleep in children with and without epilepsy. Electroencephalogr Clin Neurophysiol. (1975) 39:537.
20. Rose D, Duron B. [Prognostic value of EEG abnormalities observed during sleep in epilepsy with rolandic spikes (RSE)]. Rev Electroencephalogr Neurophysiol Clin. (1984) 14:217–26.
21. Guerrini R, Pellacani S. Benign childhood focal epilepsies. Epilepsia. (2012) 53 (Suppl 4):9–18. doi: 10.1111/j.1528-1167.2012.03609.x
22. Gobbi G, Boni A, Filippini M. The spectrum of idiopathic Rolandic epilepsy syndromes and idiopathic occipital epilepsies: from the benign to the disabling. Epilepsia. (2006) 47 (Suppl 2):62–6. doi: 10.1111/j.1528-1167.2006.00693.x
23. Aicardi J, Chevrie JJ. Atypical benign partial epilepsy of childhood. Dev Med Child Neurol. (1982) 24:281–92.
24. Fejerman N. Atypical rolandic epilepsy. Epilepsia. (2009) 50 (Suppl 7):9–12. doi: 10.1111/j.1528-1167.2009.02210.x
25. de Saint-Martin A, Picard F, Hirsch E, Fischbach M. Canaux ioniques et épilepsies. Arch Pediatr. (2001) 8 (Suppl 2):296s−297s. doi: 10.1016/S0929-693X(01)80051-8
26. Massa R, de Saint-Martin A, Carcangiu R, Rudolf G, Seegmuller C, Kleitz C, et al. EEG criteria predictive of complicated evolution in idiopathic rolandic epilepsy. Neurology. (2001) 57:1071–9. doi: 10.1212/wnl.57.6.1071
27. Van Bogaert P. Epileptic encephalopathy with continuous spike-waves during slow-wave sleep including Landau-Kleffner syndrome. Handb Clin Neurol. (2013) 111:635–40. doi: 10.1016/B978-0-444-52891-9.00066-X
28. Rudolf G, Valenti MP, Hirsch E, Szepetowski P. From rolandic epilepsy to continuous spike-and-waves during sleep and Landau-Kleffner syndromes: insights into possible genetic factors. Epilepsia. (2009) 50 (Suppl 7):25–8. doi: 10.1111/j.1528-1167.2009.02214.x
29. De Negri M. Electrical status epilepticus during sleep (ESES). Different clinical syndromes: towards a unifying view? Brain Dev. (1997) 19:447–51.
30. Doose H, Baier WK. Benign partial epilepsy and related conditions: multifactorial pathogenesis with hereditary impairment of brain maturation. Eur J Pediatr. (1989) 149:152–8.
31. Morikawa T, Seino M, Yagi K. Is rolandic discharge a hallmark of benign partial epilepsy of childhood? Epilepsy Res Suppl. (1992) 6:59–69.
33. Bali B, Kull LL, Strug LJ, Clarke T, Murphy PL, Akman CI, et al. Autosomal dominant inheritance of centrotemporal sharp waves in rolandic epilepsy families. Epilepsia. (2007) 48:2266–72. doi: 10.1111/j.1528-1167.2007.01221.x
34. De Tiège X, Goldman S, Verheulpen D, Aeby A, Poznanski N, Van Bogaert P. Coexistence of idiopathic rolandic epilepsy and CSWS in two families. Epilepsia. (2006) 47:1723–7. doi: 10.1111/j.1528-1167.2006.00644.x
35. Dimassi S, Labalme A, Lesca G, Rudolf G, Bruneau N, Hirsch E, et al. A subset of genomic alterations detected in rolandic epilepsies contains candidate or known epilepsy genes including GRIN2A and PRRT2. Epilepsia. (2014) 55:370–8. doi: 10.1111/epi.12502
36. Neubauer BA, Fiedler B, Himmelein B, Kämpfer F, Lässker U, Schwabe G, et al. Centrotemporal spikes in families with rolandic epilepsy: linkage to chromosome 15q14. Neurology. (1998) 51:1608–12.
37. Vears DF, Tsai M-H, Sadleir LG, Grinton BE, Lillywhite LM, Carney PW, et al. Clinical genetic studies in benign childhood epilepsy with centrotemporal spikes. Epilepsia. (2012) 53:319–24. doi: 10.1111/j.1528-1167.2011.03368.x
38. Carvill GL, Regan BM, Yendle SC, O'Roak BJ, Lozovaya N, Bruneau N, et al. GRIN2A mutations cause epilepsy-aphasia spectrum disorders. Nat Genet. (2013) 45:1073–6. doi: 10.1038/ng.2727
39. Lemke JR, Lal D, Reinthaler EM, Steiner I, Nothnagel M, Alber M, et al. Mutations in GRIN2A cause idiopathic focal epilepsy with rolandic spikes. Nat Genet. (2013) 45:1067–72. doi: 10.1038/ng.2728
40. Lesca G, Rudolf G, Labalme A, Hirsch E, Arzimanoglou A, Genton P, et al. Epileptic encephalopathies of the Landau-Kleffner and continuous spike and waves during slow-wave sleep types: genomic dissection makes the link with autism. Epilepsia. (2012) 53:1526–38. doi: 10.1111/j.1528-1167.2012.03559.x
41. Reutlinger C, Helbig I, Gawelczyk B, Subero JIM, Tönnies H, Muhle H, et al. Deletions in 16p13 including GRIN2A in patients with intellectual disability, various dysmorphic features, and seizure disorders of the rolandic region. Epilepsia. (2010) 51:1870–3. doi: 10.1111/j.1528-1167.2010.02555.x
42. Lesca G, Rudolf G, Bruneau N, Lozovaya N, Labalme A, Boutry-Kryza N, et al. GRIN2A mutations in acquired epileptic aphasia and related childhood focal epilepsies and encephalopathies with speech and language dysfunction. Nat Genet. (2013) 45:1061–6. doi: 10.1038/ng.2726
43. Cloarec R, Bruneau N, Rudolf G, Massacrier A, Salmi M, Bataillard M, et al. PRRT2 links infantile convulsions and paroxysmal dyskinesia with migraine. Neurology. (2012) 79:2097–103. doi: 10.1212/WNL.0b013e3182752c46
44. Fusco C, Frattini D, Bassi MT. A novel KCNQ3 gene mutation in a child with infantile convulsions and partial epilepsy with centrotemporal spikes. Eur J Paediatr Neurol. (2015) 19:102–3. doi: 10.1016/j.ejpn.2014.08.006
45. Ishii A, Yasumoto S, Ihara Y, Inoue T, Fujita T, Nakamura N, et al. Genetic analysis of PRRT2 for benign infantile epilepsy, infantile convulsions with choreoathetosis syndrome, and benign convulsions with mild gastroenteritis. Brain Dev. (2013) 35:524–30. doi: 10.1016/j.braindev.2012.09.006
46. Neubauer BA, Waldegger S, Heinzinger J, Hahn A, Kurlemann G, Fiedler B, et al. KCNQ2 and KCNQ3 mutations contribute to different idiopathic epilepsy syndromes. Neurology. (2008) 71:177–83. doi: 10.1212/01.wnl.0000317090.92185.ec
47. Reinthaler EM, Lal D, Jurkowski W, Feucht M, Steinböck H, Gruber-Sedlmayr U, et al. Analysis of ELP4, SRPX2, and interacting genes in typical and atypical rolandic epilepsy. Epilepsia. (2014) 55:e89–93. doi: 10.1111/epi.12712
48. Singh NA, Westenskow P, Charlier C, Pappas C, Leslie J, Dillon J, et al. KCNQ2 and KCNQ3 potassium channel genes in benign familial neonatal convulsions: expansion of the functional and mutation spectrum. Brain. (2003) 126:2726–37. doi: 10.1093/brain/awg286
49. Dulac O, Milh M, Holmes GL. Brain maturation and epilepsy. Handb Clin Neurol. (2013) 111:441–6. doi: 10.1016/B978-0-444-52891-9.00047-6
50. Gkampeta A, Pavlou E. Emerging genetic influences in benign epilepsy with centro-temporal spikes - BECTS. Epilepsy Res. (2012) 101:197–201. doi: 10.1016/j.eplepsyres.2012.06.011
51. Berroya AG, McIntyre J, Webster R, Lah S, Sabaz M, Lawson J, et al. Speech and language deterioration in benign rolandic epilepsy. J Child Neurol. (2004) 19:53–8. doi: 10.1177/08830738040190010706
52. Danielsson J, Petermann F. Cognitive deficits in children with benign rolandic epilepsy of childhood or rolandic discharges: a study of children between 4 and 7 years of age with and without seizures compared with healthy controls. Epilepsy Behav. (2009) 16:646–51. doi: 10.1016/j.yebeh.2009.08.012
53. Monjauze C, Broadbent H, Boyd SG, Neville BGR, Baldeweg T. Language deficits and altered hemispheric lateralization in young people in remission from BECTS. Epilepsia. (2011) 52:e79–83. doi: 10.1111/j.1528-1167.2011.03105.x
54. Northcott E, Connolly AM, Berroya A, McIntyre J, Christie J, Taylor A, et al. Memory and phonological awareness in children with Benign Rolandic Epilepsy compared to a matched control group. Epilepsy Res. (2007) 75:57–62. doi: 10.1016/j.eplepsyres.2007.04.004
55. Staden U, Isaacs E, Boyd SG, Brandl U, Neville BG. Language dysfunction in children with Rolandic epilepsy. Neuropediatrics. (1998) 29:242–8. doi: 10.1055/s-2007-973569
56. Deltour L, Barathon M, Quaglino V, Vernier M-P, Despretz P, Boucart M, et al. Children with benign epilepsy with centrotemporal spikes (BECTS) show impaired attentional control: evidence from an attentional capture paradigm. Epileptic Disord. (2007) 9:32–8. doi: 10.1684/epd.2007.0066
57. Deltour L, Querné L, Vernier-Hauvette MP, Berquin P. Deficit of endogenous spatial orienting of attention in children with benign epilepsy with centrotemporal spikes (BECTS). Epilepsy Res. (2008) 79:112–9. doi: 10.1016/j.eplepsyres.2007.12.019
58. Kim E-H, Yum M-S, Kim H-W, Ko T-S. Attention-deficit/hyperactivity disorder and attention impairment in children with benign childhood epilepsy with centrotemporal spikes. Epilepsy Behav. (2014) 37:54–8. doi: 10.1016/j.yebeh.2014.05.030
59. Filippini M, Ardu E, Stefanelli S, Boni A, Gobbi G, Benso F. Neuropsychological profile in new-onset benign epilepsy with centrotemporal spikes (BECTS): Focusing on executive functions. Epilepsy Behav. (2016) 54:71–9. doi: 10.1016/j.yebeh.2015.11.010
60. Northcott E, Connolly AM, Berroya A, Sabaz M, McIntyre J, Christie J, et al. The neuropsychological and language profile of children with benign rolandic epilepsy. Epilepsia. (2005) 46:924–30. doi: 10.1111/j.1528-1167.2005.62304.x
61. Overvliet GM, Besseling RMH, Jansen JFA, van der Kruijs SJM, Vles JSH, Hofman PAM, et al. Early onset of cortical thinning in children with rolandic epilepsy. Neuroimage Clin. (2013) 2:434–9. doi: 10.1016/j.nicl.2013.03.008
62. Piccinelli P, Borgatti R, Aldini A, Bindelli D, Ferri M, Perna S, et al. Academic performance in children with rolandic epilepsy. Dev Med Child Neurol. (2008) 50:353–6. doi: 10.1111/j.1469-8749.2007.02040.x
63. Riva D, Vago C, Franceschetti S, Pantaleoni C, D'Arrigo S, Granata T, et al. Intellectual and language findings and their relationship to EEG characteristics in benign childhood epilepsy with centrotemporal spikes. Epilepsy Behav. (2007) 10:278–85. doi: 10.1016/j.yebeh.2006.12.003
64. Völkl-Kernstock S, Bauch-Prater S, Ponocny-Seliger E, Feucht M. Speech and school performance in children with benign partial epilepsy with centro-temporal spikes (BCECTS). Seizure. (2009) 18:320–6. doi: 10.1016/j.seizure.2008.11.011
65. Kárpáti J, Donauer N, Somogyi E, Kónya A. Working memory integration processes in benign childhood epilepsy with centrotemporal spikes. Cogn Behav Neurol. (2015) 28:207–14. doi: 10.1097/WNN.0000000000000075
66. Croona C, Kihlgren M, Lundberg S, Eeg-Olofsson O, Eeg-Olofsson KE. Neuropsychological findings in children with benign childhood epilepsy with centrotemporal spikes. Dev Med Child Neurol. (1999) 41:813–8.
67. Weglage J, Demsky A, Pietsch M, Kurlemann G. Neuropsychological, intellectual, and behavioral findings in patients with centrotemporal spikes with and without seizures. Dev Med Child Neurol. (1997) 39:646–51.
68. Ebus SCM, Overvliet GM, Arends JBA, Aldenkamp AP. Reading performance in children with rolandic epilepsy correlates with nocturnal epileptiform activity, but not with epileptiform activity while awake. Epilepsy Behav. (2011) 22:518–22. doi: 10.1016/j.yebeh.2011.08.008
69. Tedrus GMAS, Fonseca LC, Melo EMV, Ximenes VL. Educational problems related to quantitative EEG changes in benign childhood epilepsy with centrotemporal spikes. Epilepsy Behav. (2009) 15:486–90. doi: 10.1016/j.yebeh.2009.06.010
70. Bailet LL, Turk WR. The impact of childhood epilepsy on neurocognitive and behavioral performance: a prospective longitudinal study. Epilepsia. (2000) 41:426–31.
71. Farwell JR, Dodrill CB, Batzel LW. Neuropsychological abilities of children with epilepsy. Epilepsia. (1985) 26:395–400.
72. Neyens LG, Aldenkamp AP, Meinardi HM. Prospective follow-up of intellectual development in children with a recent onset of epilepsy. Epilepsy Res. (1999) 34:85–90.
73. Vannest J, Tenney JR, Gelineau-Morel R, Maloney T, Glauser TA. Cognitive and behavioral outcomes in benign childhood epilepsy with centrotemporal spikes. Epilepsy Behav. (2015) 45:85–91. doi: 10.1016/j.yebeh.2015.01.041
74. Lillywhite LM, Saling MM, Harvey AS, Abbott DF, Archer JS, Vears DF, et al. Neuropsychological and functional MRI studies provide converging evidence of anterior language dysfunction in BECTS. Epilepsia. (2009) 50:2276–84. doi: 10.1111/j.1528-1167.2009.02065.x
75. Völkl-Kernstock S, Willinger U, Feucht M. Spacial perception and spatial memory in children with benign childhood epilepsy with centro-temporal spikes (BCECTS). Epilepsy Res. (2006) 72:39–48. doi: 10.1016/j.eplepsyres.2006.07.004
76. Weglage J, Demsky A, Pietsch M, Kurlemann G. Neuropsychological, intellectual, and behavioral findings in patients with centrotemporal spikes with and without seizures. Dev Med Child Neurol. (1997) 39:646–51.
77. Nayrac P, Beaussart M. [Pre-rolandic spike-waves: a very peculiar EEG reading; electroclinical study of 21 cases]. Rev Neurol. (1958) 99:201–6.
78. Talairach J, Bancaud J. Lesion, “irritative” zone and epileptogenic focus. Confin Neurol. (1966) 27:91–4.
79. Alving J, Fabricius M, Rosenzweig I, Beniczky S. Ictal source imaging and electroclinical correlation in self-limited epilepsy with centrotemporal spikes. Seizure. (2017) 52:7–10. doi: 10.1016/j.seizure.2017.09.006
80. Capovilla G, Beccaria F, Bianchi A, Canevini MP, Giordano L, Gobbi G, et al. Ictal EEG patterns in epilepsy with centro-temporal spikes. Brain Dev. (2011) 33:301–9. doi: 10.1016/j.braindev.2010.06.007
81. Gutierrez AR, Brick JF, Bodensteiner J. Dipole reversal: an ictal feature of benign partial epilepsy with centrotemporal spikes. Epilepsia. (1990) 31:544–8.
82. Sarkis RA, Loddenkemper T, Burgess RC, Wyllie E. Childhood absence epilepsy in patients with benign focal epileptiform discharges. Pediatr Neurol. (2009) 41:428–34. doi: 10.1016/j.pediatrneurol.2009.07.004
83. Bourel-Ponchel E, Mahmoudzadeh M, Berquin P, Wallois F. Local and distant dysregulation of synchronization around interictal spikes in BECTS. Front Neurosci. (2017) 11:59. doi: 10.3389/fnins.2017.00059
84. Kim H, Yoo IH, Lim BC, Hwang H, Chae J-H, Choi J, et al. Averaged EEG spike dipole analysis may predict atypical outcome in Benign Childhood Epilepsy with Centrotemporal Spikes (BCECTS). Brain Dev. (2016) 38:903–8. doi: 10.1016/j.braindev.2016.06.001
85. Lombroso CT. Sylvian seizures and midtemporal spike foci in children. Arch Neurol. (1967) 17:52–9.
86. Kakisaka Y, Iwasaki M, Haginoya K, Kanno A, Tsuchiya S, Nakasato N. Somatotopic distribution of peri-rolandic spikes may predict prognosis in pediatric-onset epilepsy with sensorimotor seizures. Clin Neurophysiol. (2011) 122:869–73. doi: 10.1016/j.clinph.2010.09.026
87. Kamada K, Möller M, Saguer M, Kassubek J, Kaltenhäuser M, Kober H, et al. Localization analysis of neuronal activities in benign rolandic epilepsy using magnetoencephalography. J Neurol Sci. (1998) 154:164–72.
88. Pataraia E, Feucht M, Lindinger G, Aull-Watschinger S, Baumgartner C. Combined electroencephalography and magnetoencephalography of interictal spikes in benign rolandic epilepsy of childhood. Clin Neurophysiol. (2008) 119:635–41. doi: 10.1016/j.clinph.2007.11.009
89. Ishitobi M, Nakasato N, Yamamoto K, Iinuma K. Opercular to interhemispheric source distribution of benign rolandic spikes of childhood. Neuroimage. (2005) 25:417–23. doi: 10.1016/j.neuroimage.2004.11.040
90. Minami T, Gondo K, Yamamoto T, Yanai S, Tasaki K, Ueda K. Magnetoencephalographic analysis of rolandic discharges in benign childhood epilepsy. Ann Neurol. (1996) 39:326–34. doi: 10.1002/ana.410390309
91. Kubota M, Takeshita K, Sakakihara Y, Yanagisawa M. Magnetoencephalographic study of giant somatosensory evoked responses in patients with rolandic epilepsy. J Child Neurol. (2000) 15:370–9. doi: 10.1177/088307380001500604
92. Lin YY, Shih YH, Chang KP, Lee WT, Yu HY, Hsieh JC, et al. MEG localization of rolandic spikes with respect to SI and SII cortices in benign rolandic epilepsy. Neuroimage. (2003) 20:2051–61. doi: 10.1016/j.neuroimage.2003.08.019
93. Huiskamp G, van Der Meij W, van Huffelen A, van Nieuwenhuizen O. High resolution spatio-temporal EEG-MEG analysis of rolandic spikes. J Clin Neurophysiol. (2004) 21:84–95. doi: 10.1097/00004691-200403000-00002
94. Kakisaka Y, Nakasato N, Haginoya K, Kanno A, Tsuchiya S. Sensorimotor seizures of pediatric onset with unusual posteriorly oriented rolandic spikes. Epilepsy Res. (2009) 84:153–8. doi: 10.1016/j.eplepsyres.2009.01.012
96. Shiraishi H, Haginoya K, Nakagawa E, Saitoh S, Kaneko Y, Nakasato N, et al. Magnetoencephalography localizing spike sources of atypical benign partial epilepsy. Brain Dev. (2014) 36:21–7. doi: 10.1016/j.braindev.2012.12.011
97. Aubert-Broche B, Fonov VS, García-Lorenzo D, Mouiha A, Guizard N, Coupé P, et al. A new method for structural volume analysis of longitudinal brain MRI data and its application in studying the growth trajectories of anatomical brain structures in childhood. Neuroimage. (2013) 82:393–402. doi: 10.1016/j.neuroimage.2013.05.065
98. Giedd J. Brain development, IX: human brain growth. Am J Psychiatry. (1999) 156:4. doi: 10.1176/ajp.156.1.4
99. Lenroot RK, Gogtay N, Greenstein DK, Wells EM, Wallace GL, Clasen LS, et al. Sexual dimorphism of brain developmental trajectories during childhood and adolescence. Neuroimage. (2007) 36:1065–73. doi: 10.1016/j.neuroimage.2007.03.053
100. Blakemore S-J, Burnett S, Dahl RE. The role of puberty in the developing adolescent brain. Hum Brain Mapp. (2010) 31:926–33. doi: 10.1002/hbm.21052
101. Knickmeyer RC, Gouttard S, Kang C, Evans D, Wilber K, Smith JK, et al. A structural MRI study of human brain development from birth to 2 years. J Neurosci. (2008) 28:12176–82. doi: 10.1523/JNEUROSCI.3479-08.2008
102. Bourgeois JP, Goldman-Rakic PS, Rakic P. Synaptogenesis in the prefrontal cortex of rhesus monkeys. Cereb Cortex. (1994) 4:78–96.
103. Bourgeois JP, Rakic P. Changes of synaptic density in the primary visual cortex of the macaque monkey from fetal to adult stage. J Neurosci. (1993) 13:2801–20.
104. Huttenlocher PR, Dabholkar AS. Regional differences in synaptogenesis in human cerebral cortex. J Comp Neurol. (1997) 387:167–78.
105. Huttenlocher PR, de Courten C. The development of synapses in striate cortex of man. Hum Neurobiol. (1987) 6:1–9.
106. Zecevic N, Bourgeois JP, Rakic P. Changes in synaptic density in motor cortex of rhesus monkey during fetal and postnatal life. Brain Res Dev Brain Res. (1989) 50:11–32.
107. Innocenti GM, Price DJ. Exuberance in the development of cortical networks. Nat Rev Neurosci. (2005) 6:955–65. doi: 10.1038/nrn1790
108. Johnson MH. Functional brain development in humans. Nat Rev Neurosci. (2001) 2:475–83. doi: 10.1038/35081509
109. Neniskyte U, Gross CT. Errant gardeners: glial-cell-dependent synaptic pruning and neurodevelopmental disorders. Nat Rev Neurosci. (2017) 18:658–70. doi: 10.1038/nrn.2017.110
110. Riccomagno MM, Kolodkin AL. Sculpting neural circuits by axon and dendrite pruning. Annu Rev Cell Dev Biol. (2015) 31:779–805. doi: 10.1146/annurev-cellbio-100913-013038
111. Lin JJ, Riley JD, Hsu DA, Stafstrom CE, Dabbs K, Becker T, et al. Striatal hypertrophy and its cognitive effects in new-onset benign epilepsy with centrotemporal spikes. Epilepsia. (2012) 53:677–85. doi: 10.1111/j.1528-1167.2012.03422.x
112. Hermann BP, Dabbs K, Becker T, Jones JE, Myers y, Gutierrez A, Wendt G, et al. Brain development in children with new onset epilepsy: a prospective controlled cohort investigation. Epilepsia. (2010) 51:2038–46. doi: 10.1111/j.1528-1167.2010.02563.x
113. Kim E-H, Yum M-S, Shim W-H, Yoon H-K, Lee Y-J, Ko T-S. Structural abnormalities in benign childhood epilepsy with centrotemporal spikes (BCECTS). Seizure. (2015) 27:40–6. doi: 10.1016/j.seizure.2015.02.027
114. Luo C, Zhang Y, Cao W, Huang Y, Yang F, Wang J, et al. Altered structural and functional feature of striato-cortical circuit in benign epilepsy with centrotemporal spikes. Int J Neural Syst. (2015) 25:1550027. doi: 10.1142/S0129065715500276
115. Pardoe HR, Berg AT, Archer JS, Fulbright RK, Jackson GD. A neurodevelopmental basis for BECTS: evidence from structural MRI. Epilepsy Res. (2013) 105:133–9. doi: 10.1016/j.eplepsyres.2012.11.008
116. Saute R, Dabbs K, Jones JE, Jackson DC, Seidenberg M, Hermann BP. Brain morphology in children with epilepsy and ADHD. PLoS ONE. (2014) 9:e95269. doi: 10.1371/journal.pone.0095269
117. Shakeri M, Datta AN, Malfait D, Oser N, Létourneau-Guillon L, Major P, et al. Sub-cortical brain morphometry and its relationship with cognition in rolandic epilepsy. Epilepsy Res. (2017) 138:39–45. doi: 10.1016/j.eplepsyres.2017.09.007
118. Garcia-Ramos C, Jackson DC, Lin JJ, Dabbs K, Jones JE, Hsu DA, et al. Cognition and brain development in children with benign epilepsy with centrotemporal spikes. Epilepsia. (2015) 56:1615–22. doi: 10.1111/epi.13125
119. Kanemura H, Hata S, Aoyagi K, Sugita K, Aihara M. Serial changes of prefrontal lobe growth in the patients with benign childhood epilepsy with centrotemporal spikes presenting with cognitive impairments/behavioral problems. Brain Dev. (2011) 33:106–13. doi: 10.1016/j.braindev.2010.03.005
120. Ciumas C, Saignavongs M, Ilski F, Herbillon V, Laurent A, Lothe A, et al. White matter development in children with benign childhood epilepsy with centro-temporal spikes. Brain. (2014) 137:1095–106. doi: 10.1093/brain/awu039
121. Xiao F, Chen Q, Yu X, Tang Y, Luo C, Fang J, et al. Hemispheric lateralization of microstructural white matter abnormalities in children with active benign childhood epilepsy with centrotemporal spikes (BECTS): a preliminary DTI study. J Neurol Sci. (2014) 336:171–9. doi: 10.1016/j.jns.2013.10.033
122. Ciumas C, Laurent A, Saignavongs M, Ilski F, de Bellescize J, Panagiotakaki E, et al. Behavioral and fMRI responses to fearful faces are altered in benign childhood epilepsy with centrotemporal spikes (BCECTS). Epilepsia. (2017) 58:1716–27. doi: 10.1111/epi.13858
123. Kanemura H, Aihara M. Growth disturbance of frontal lobe in BCECTS presenting with frontal dysfunction. Brain Dev. (2009) 31:771–4. doi: 10.1016/j.braindev.2008.12.007
124. Besseling RMH, Jansen JFA, Overvliet GM, van der Kruijs SJM, Ebus SCM, de Louw AJA, et al. Delayed convergence between brain network structure and function in rolandic epilepsy. Front Hum Neurosci. (2014) 8:704. doi: 10.3389/fnhum.2014.00704
125. Prince DA. Benign focal epilepsies of childhood: genetically determined pathophysiology–epilepsy that comes and goes. Epilepsia. (2000) 41:1085–7.
126. Adebimpe A, Aarabi A, Bourel-Ponchel E, Mahmoudzadeh M, Wallois F. Functional brain dysfunction in patients with benign childhood epilepsy as revealed by graph theory. PLoS ONE. (2015) 10:e0139228. doi: 10.1371/journal.pone.0139228
127. Adebimpe A, Aarabi A, Bourel-Ponchel E, Mahmoudzadeh M, Wallois F. EEG resting state analysis of cortical sources in patients with benign epilepsy with centrotemporal spikes. Neuroimage Clin. (2015) 9:275–82. doi: 10.1016/j.nicl.2015.08.014
128. Avanzini G, Manganotti P, Meletti S, Moshé SL, Panzica F, Wolf P, et al. The system epilepsies: a pathophysiological hypothesis. Epilepsia. (2012) 53:771–78. doi: 10.1111/j.1528-1167.2012.03462.x
129. van Klink NE, van 't Klooster MA, Leijten FS, Jacobs J, Braun KP, Zijlmans M. Ripples on rolandic spikes: a marker of epilepsy severity. Epilepsia. (2016) 57:1179–89. doi: 10.1111/epi.13423
130. Qian P, Li H, Xue J, Yang Z. Scalp-recorded high-frequency oscillations in atypical benign partial epilepsy. Clin Neurophysiol. (2016) 12:3306–13. doi: 10.1016/j.clinph.2016.07.013
131. Kobayashi K, Yoshinaga H, Toda Y, Inoue T, Oka M, Ohtsuka Y. High-frequency oscillations in idiopathic partial epilepsy of childhood. Epilepsia. (2011) 52:1812–19. doi: 10.1111/j.1528-1167.2011.03169.x
132. Archer JS, Briellman RS, Abbott DF, Syngeniotis A, Wellard RM, Jackson GD. Benign epilepsy with centro-temporal spikes: spike triggered fMRI shows somato-sensory cortex activity. Epilepsia. (2003) 44:200–4.
133. Boor S, Vucurevic G, Pfleiderer C, Stoeter P, Kutschke G, Boor R. EEG-related functional MRI in benign childhood epilepsy with centrotemporal spikes. Epilepsia. (2003) 44:688–92.
134. Lengler U, Kafadar I, Neubauer BA, Krakow K. fMRI correlates of interictal epileptic activity in patients with idiopathic benign focal epilepsy of childhood. A simultaneous EEG-functional MRI study. Epilepsy Res. (2007) 75:29–38. doi: 10.1016/j.eplepsyres.2007.03.016
135. Moeller F, Stephani U, Siniatchkin M. Simultaneous EEG and fMRI recordings (EEG-fMRI) in children with epilepsy. Epilepsia. (2013) 54:971–82. doi: 10.1111/epi.12197
136. Bourel-Ponchel E. Exploration de L'unité Neurovasculaire Dans L'Épilepsie de l'Enfant : Approche Multimodale Haute Densité Couplant l'EEG à l'Imagerie Optique Fonctionnelle. (2013) Available online at: http://www.theses.fr/2013AMIED011 (accessed April 30, 2019).
137. Manoochehri M, Mahmoudzadeh M, Osharina V, Wallois F. Shedding light on interictal epileptic spikes: an in vivo study using fast optical signal and electrocorticography. Epilepsia. (2017) 58:608–16. doi: 10.1111/epi.13689
138. Manoochehri M, Mahmoudzadeh M, Bourel-Ponchel E, Wallois F. Cortical light scattering during interictal epileptic spikes in frontal lobe epilepsy in children: a fast optical signal and electroencephalographic study. Epilepsia. (2017) 58:2064–72. doi: 10.1111/epi.13926
139. Keller CJ, Truccolo W, Gale JT, Eskandar E, Thesen T, Carlson C, et al. Heterogeneous neuronal firing patterns during interictal epileptiform discharges in the human cortex. Brain. (2010) 133:1668–81. doi: 10.1093/brain/awq112
140. Zeng H, Ramos CG, Nair VA, Hu Y, Liao J, La C, et al. Regional homogeneity (ReHo) changes in new onset versus chronic benign epilepsy of childhood with centrotemporal spikes (BECTS): A resting state fMRI study. Epilepsy Res. (2015) 116:79–85. doi: 10.1016/j.eplepsyres.2015.06.017
141. Adebimpe A, Aarabi A, Bourel-Ponchel E, Mahmoudzadeh M, Wallois F. EEG resting state functional connectivity analysis in children with benign epilepsy with centrotemporal spikes. Front Neurosci. (2016) 10:143. doi: 10.3389/fnins.2016.00143
142. Tang Y-L, Ji G-J, Yu Y, Wang J, Wang Z-J, Zang Y-F, et al. Altered regional homogeneity in rolandic epilepsy: a resting-state FMRI study. Biomed Res Int. (2014) 2014:960395. doi: 10.1155/2014/960395
143. Besseling RMH, Jansen JFA, Overvliet GM, van der Kruijs SJM, Ebus SCM, de Louw A, et al. Reduced structural connectivity between sensorimotor and language areas in rolandic epilepsy. PLoS ONE. (2013) 8:e83568. doi: 10.1371/journal.pone.0083568
144. Besseling RMH, Jansen JFA, Overvliet GM, van der Kruijs SJM, Vles JSH, Ebus SCM, et al. Reduced functional integration of the sensorimotor and language network in rolandic epilepsy. Neuroimage Clin. (2013) 2:239–46. doi: 10.1016/j.nicl.2013.01.004
145. Bassett DS, Bullmore E. Small-world brain networks. Neuroscientist. (2006) 12:512–23. doi: 10.1177/1073858406293182
146. Ponten SC, Bartolomei F, Stam CJ. Small-world networks and epilepsy: graph theoretical analysis of intracerebrally recorded mesial temporal lobe seizures. Clin Neurophysiol. (2007) 118:918–27. doi: 10.1016/j.clinph.2006.12.002
147. Xiao F, An D, Lei D, Li L, Chen S, Wu X, et al. Real-time effects of centrotemporal spikes on cognition in rolandic epilepsy: an EEG-fMRI study. Neurology. (2016) 86:544–51. doi: 10.1212/WNL.0000000000002358
148. Adebimpe A, Bourel-Ponchel E, Wallois F. Identifying neural drivers of benign childhood epilepsy with centrotemporal spikes. Neuroimage Clin. (2018) 17:739–50. doi: 10.1016/j.nicl.2017.11.024
149. Wu Y, Ji G-J, Zang Y-F, Liao W, Jin Z, Liu Y-L, et al. Local activity and causal connectivity in children with benign Epilepsy with centrotemporal spikes. PLoS ONE. (2015) 10:e0134361. doi: 10.1371/journal.pone.0134361
150. Kiebel SJ, Garrido MI, Moran RJ, Friston KJ. Dynamic causal modelling for EEG and MEG. Cogn Neurodyn. (2008) 2:121–36. doi: 10.1007/s11571-008-9038-0
151. Stuss DT. Functions of the frontal lobes: relation to executive functions. J Int Neuropsychol Soc. (2011) 17:759–65. doi: 10.1017/S1355617711000695
152. Datta AN, Oser N, Bauder F, Maier O, Martin F, Ramelli GP, et al. Cognitive impairment and cortical reorganization in children with benign epilepsy with centrotemporal spikes. Epilepsia. (2013) 54:487–94. doi: 10.1111/epi.12067
153. Malfait D, Tucholka A, Mendizabal S, Tremblay J, Poulin C, Oskoui M, et al. fMRI brain response during sentence reading comprehension in children with benign epilepsy with centro-temporal spikes. Epilepsy Res. (2015) 117:42–51. doi: 10.1016/j.eplepsyres.2015.08.009
154. Verrotti A, Filippini M, Matricardi S, Agostinelli MF, Gobbi G. Memory impairment and Benign Epilepsy with centrotemporal spike (BECTS): a growing suspicion. Brain Cogn. (2014) 84:123–31. doi: 10.1016/j.bandc.2013.11.014
155. Xiao F, Li L, An D, Lei D, Tang Y, Yang T, et al. Altered attention networks in benign childhood epilepsy with centrotemporal spikes (BECTS): a resting-state fMRI study. Epilepsy Behav. (2015) 45:234–41. doi: 10.1016/j.yebeh.2015.01.016
156. Baglietto MG, Battaglia FM, Nobili L, Tortorelli S, De Negri E, Calevo MG, et al. Neuropsychological disorders related to interictal epileptic discharges during sleep in benign epilepsy of childhood with centrotemporal or Rolandic spikes. Dev Med Child Neurol. (2001) 43:407–12.
157. Liasis A, Bamiou DE, Boyd S, Towell A. Evidence for a neurophysiologic auditory deficit in children with benign epilepsy with centro-temporal spikes. J Neural Transm. (2006) 113:939–49. doi: 10.1007/s00702-005-0357-6
158. Metz-Lutz MN, Kleitz C, de Saint Martin A, Massa R, Hirsch E, Marescaux C. Cognitive development in benign focal epilepsies of childhood. Dev Neurosci. (1999) 21:182–90. doi: 10.1159/000017397
159. Cohen M. Auditory/verbal and visual/spatial memory in children with complex partial epilepsy of temporal lobe origin. Brain Cogn. (1992) 20:315–26.
160. Aarts JH, Binnie CD, Smit AM, Wilkins AJ. Selective cognitive impairment during focal and generalized epileptiform EEG activity. Brain. (1984) 107:293–308.
161. Binnie CD. Cognitive impairment during epileptiform discharges: is it ever justifiable to treat the EEG? Lancet Neurol. (2003) 2:725–30.
162. Carlsson G, Igelbrink-Schulze N, Neubauer BA, Stephani U. Neuropsychological long-term outcome of rolandic EEG traits. Epileptic Disord. (2000) 2 Suppl 1:S63–66.
163. Dubois CM, Zesiger P, Perez ER, Ingvar MM, Deonna T. Acquired epileptic dysgraphia: a longitudinal study. Dev Med Child Neurol. (2003) 45:807–12.
164. Chevalier H, Metz-Lutz MN, Segalowitz SJ. Impulsivity and control of inhibition in benign focal childhood epilepsy (BFCE). Brain Cogn. (2000) 43:86–90.
165. Maquet P, Metz-Lutz MN, de Saint-Martin A, Marescaux C, Hirsch E. Isotope tracer-techniques in rolandic epilepsy and its variants. Epileptic Disord. (2000) 2 (Suppl 1):S55–57.
166. Shewmon DA, Erwin RJ. Focal spike-induced cerebral dysfunction is related to the after-coming slow wave. Ann Neurol. (1988) 23:131–7. doi: 10.1002/ana.410230205
167. Fonseca LC, Tedrus GMAS, Pacheco EMC. Epileptiform EEG discharges in benign childhood epilepsy with centrotemporal spikes: reactivity and transitory cognitive impairment. Epilepsy Behav. (2007) 11:65–70. doi: 10.1016/j.yebeh.2007.04.001
168. Kleen JK, Scott RC, Holmes GL, Lenck-Santini PP. Hippocampal interictal spikes disrupt cognition in rats. Ann Neurol. (2010) 67:250–7. doi: 10.1002/ana.21896
169. Van Bogaert P, Urbain C, Galer S, Ligot N, Peigneux P, De Tiège X. Impact of focal interictal epileptiform discharges on behaviour and cognition in children. Neurophysiol Clin. (2012) 42:53–8. doi: 10.1016/j.neucli.2011.11.004
170. Baumbach HD, Chow KL. Visuocortical epileptiform discharges in rabbits: differential effects on neuronal development in the lateral geniculate nucleus and superior colliculus. Brain Res. (1981) 209:61–76.
171. Crabtree JW, Chow KL, Ostrach LH, Baumbach HD. Development of receptive field properties in the visual cortex of rabbits subjected to early epileptiform cortical discharges. Brain Res. (1981) 227:269–81.
172. Badawy RAB, Johnson KA, Cook MJ, Harvey AS. A mechanistic appraisal of cognitive dysfunction in epilepsy. Neurosci Biobehav Rev. (2012) 36:1885–96. doi: 10.1016/j.neubiorev.2012.05.002
173. Haut SR, Velísková J, Moshé SL. Susceptibility of immature and adult brains to seizure effects. Lancet Neurol. (2004) 3:608–17. doi: 10.1016/S1474-4422(04)00881-6
174. Hermann B, Seidenberg M, Bell B, Rutecki P, Sheth R, Ruggles K, et al. The neurodevelopmental impact of childhood-onset temporal lobe epilepsy on brain structure and function. Epilepsia. (2002) 43:1062–71.
175. Maltoni L, Posar A, Parmeggiani A. Long-term follow-up of cognitive functions in patients with continuous spike-waves during sleep (CSWS). Epilepsy Behav. (2016) 60:211–7. doi: 10.1016/j.yebeh.2016.04.006
176. Stafstrom CE, Holmes GL. Effects of uncontrolled seizures. Neural changes in animal models. Adv Exp Med Biol. (2002) 497:171–94.
177. Mirandola L, Cantalupo G, Vaudano AE, Anvanzini P, Ruggieri A, Pisani, et al. Centro-temporal spikes during NREM sleep : the promoting action of thalamus revealed by simultaneous EEG and fMRI coregistration. Epilepsy Behav Case Rep. (2013) 7:106–9. doi: 10.1016/j.ebcr.2013.06.005
Keywords: benign epilepsy with centro temporal spike, neurocognitive impairment, interictal epileptic spike, high density EEG, time frequency analysis, connectivity
Citation: Bourel-Ponchel E, Mahmoudzadeh M, Adebimpe A and Wallois F (2019) Functional and Structural Network Disorganizations in Typical Epilepsy With Centro-Temporal Spikes and Impact on Cognitive Neurodevelopment. Front. Neurol. 10:809. doi: 10.3389/fneur.2019.00809
Received: 02 April 2019; Accepted: 15 July 2019;
Published: 29 August 2019.
Edited by:
Marino M. Bianchin, Federal University of Rio Grande do Sul, BrazilReviewed by:
Peter Halasz, Hungarian Society for Sleep Medicine, HungaryDetlev Boison, Rutgers University, United States
Copyright © 2019 Bourel-Ponchel, Mahmoudzadeh, Adebimpe and Wallois. This is an open-access article distributed under the terms of the Creative Commons Attribution License (CC BY). The use, distribution or reproduction in other forums is permitted, provided the original author(s) and the copyright owner(s) are credited and that the original publication in this journal is cited, in accordance with accepted academic practice. No use, distribution or reproduction is permitted which does not comply with these terms.
*Correspondence: Emilie Bourel-Ponchel, emilie.bourel@u-picardie.fr