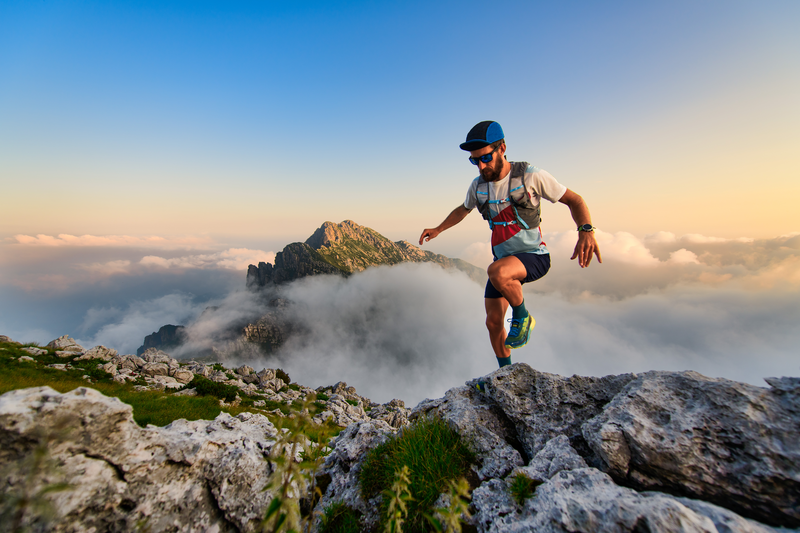
95% of researchers rate our articles as excellent or good
Learn more about the work of our research integrity team to safeguard the quality of each article we publish.
Find out more
HYPOTHESIS AND THEORY article
Front. Neurol. , 24 July 2019
Sec. Neurodegeneration
Volume 10 - 2019 | https://doi.org/10.3389/fneur.2019.00758
This article is part of the Research Topic The Role of Lipids in the Pathogenesis of Parkinson’s Disease View all 8 articles
Parkinson's disease (PD) is a common debilitating neurodegenerative disease caused by a loss of dopamine neurons in the substantia nigra within the central nervous system (CNS). The process leading to this neuronal loss is poorly understood. Seborrheic dermatitis (SD) is a common benign inflammatory condition of the skin which mainly affects lipid-rich regions of the head and trunk. SD is caused by over proliferation of the lipophilic fungus Malassezia. PD and SD are strongly associated. The increased PD risk following an SD diagnosis (OR = 1.69, 95% CI 1.36, 2.1; p < 0.001) reported by Tanner and colleagues remains unexplained. Malassezia were historically considered commensals confined to the skin. However, many recent studies report finding Malassezia in internal organs, including the CNS. This raises the possibility that Malassezia might be directly contributing to PD. Several lines of evidence support this hypothesis. AIDS is causally associated with both parkinsonism and SD, suggesting that weak T cell-mediated control of commensal microbes such as Malassezia might contribute to both. Genetic polymorphisms associated with PD (LRRK2, GBA, PINK1, SPG11, SNCA) increase availability of lipids within human cells, providing a suitable environment for Malassezia. Four LRRK2 polymorphisms which increase PD risk also increase Crohn's disease risk; Crohn's disease is strongly associated with an immune response against fungi, particularly Malassezia. Finally, Malassezia hypha formation and melanin synthesis are stimulated by L-DOPA, which could promote Malassezia invasiveness of dopamine neurons, and contribute to the accumulation of melanin in these neurons. Although Malassezia's presence in the substantia nigra remains to be confirmed, if Malassezia play a role in PD etiology, antifungal drugs should be tested as a possible therapeutic intervention.
Worldwide, about 20 out of 100,000 people are diagnosed with Parkinson's disease (PD) every year (1, 2). Prevalence in persons over 65 years of age is about 1.8% (3), and overall prevalence is expected to rise with aging demographics (4). Loss of dopamine in PD was discovered 60 years ago (5), and still provides the basis of therapy with its precursor, L-DOPA (6). However, the etiology of PD remains elusive, hindering the development of additional disease modifying treatments. Only 3–5% of PD cases are explained by known genetic variants (7), indicating that the majority of cases are sporadic and are likely influenced by environmental factors (7). An infectious component in PD has been suspected since the 1920s, because parkinsonism often followed encephalitis lethargica (8). The potential role of microbes and viruses in neurological diseases is becoming increasingly recognized, including in multiple sclerosis (9), Alzheimer's disease (10) and PD (11, 12). Some research groups specifically suspect fungal infections might be involved in central nervous system (CNS) diseases including Alzheimer's disease (13–15), amyotrophic lateral sclerosis (13, 16, 17), multiple sclerosis (18, 19), and schizophrenia (20–22).
Microbes and viruses are present in all humans from birth. While enteric bacteria have received the most attention from the research community, histological and molecular evidence of bacteria, fungi, and viruses have been found in organs previously considered mostly sterile, including the CNS (14–16, 23–25). Although most microbes are usually benign, some can become pathogenic under certain conditions. Propitious conditions allowing commensals to become pathogens are varied in nature, and include an overabundance of nutrients required for microbial growth, as well as immunodeficiency. Though immunodeficiency has been most widely studied in human immunodeficiency virus (HIV) patients (26–32), genetic or congenital immunological defects (33–37), the use of immunomodulatory drugs (38–40), and age-related immunosenescence (40, 41) all increase the risk of disease caused by normally well-tolerated microbes.
When both an overabundance of nutrients required for microbial growth and immunodeficiency occur in the same individual, this greatly favors microbial over proliferation. For example, the commensal lipophilic fungus Malassezia furfur can invade internal organs of immunodeficient infants who undergo lipid-rich parenteral nutrition, including the CNS (34). Seborrheic dermatitis (SD), a benign dermatological condition caused by over proliferation of Malassezia on the skin (29, 42, 43), is strongly associated with PD (44–46). Recent studies have found Malassezia DNA in the CNS of multiple sclerosis (MS) (23) and Alzheimer's disease patients (24). Could over proliferation of Malassezia in the CNS contribute to PD, as it does in SD and in immunodeficient infants undergoing lipid-rich parenteral nutrition? In this review, we explore this hypothesis in detail.
Despite being the most prevalent fungal genera in the human microbiome, Malassezia are little known outside the field of dermatology. Malassezia were first described in 1889, yet it took nearly a century to firmly establish their role in dandruff and seborrheic dermatitis (47). This delay can be attributed to Malassezia's difficulty growing in culture (they require just the right concentration of certain lipids) (48), their extremely high prevalence on the skin (~100% throughout life) (49), and their resistance to fungicides (commonly used antifungal drugs do not completely eradicate Malassezia and their populations rebound once such treatments are discontinued) (50). About 50% of adults have first-hand experience with Malassezia-associated symptoms: they use antifungal shampoo to keep their dandruff under control, and whenever they switch back to normal shampoo, Malassezia and dandruff return (47).
Until very recently, it was believed that Malassezia were restricted to the skin, except in rare immunocompromised or lipid-rich parenteral nutrition cases (34, 51). Due to improved microbe detection techniques, many groups now report finding Malassezia within the body of both healthy adults and immunocompetent patients with various ailments (14–16, 23–25, 33, 52–61). Malassezia's potential role in diseases of internal organs is just coming to light (19, 54, 62–64). It is important to note that Malassezia's presence is not synonymous with disease: in the vast majority of individuals, Malassezia colonize the body without causing symptoms. This means detecting Malassezia in a given organ is far from sufficient to prove their involvement in diseases of that organ. Additional lines of evidence are necessary to implicate them, for example the efficacy of antifungal drugs in treating the disease, immunological evidence (such as antibodies against Malassezia) and genetic evidence (such as genes affecting Malassezia's lipid supply or the immune response against fungi).
Since Malassezia are not well-known in the field of neurodegenerative disorders, this section is included as a primer on Malassezia's suspected role in SD, acquired immune deficiency syndrome (AIDS), Crohn's disease (CD), spondyloarthritides (SpA), and MS. Each of these conditions is informative for PD.
Malassezia's role in SD is now generally accepted (29, 42, 43, 50). Given the right conditions, Malassezia over proliferate on the skin (65), resulting in SD—though specific mechanisms are still open to debate (29, 42, 43, 50). Most SD cases respond well to topical fungicides which reduce Malassezia populations on affected patches of skin to levels tolerated by patients (29, 42, 43).
SD occurs mainly in lipid-rich skin regions, especially the face, trunk and scalp (29, 42, 43). Malassezia are lipid-dependent fungi: they lack key lipid metabolism genes (including fatty acid synthase, stearoyl-CoA desaturase, and enoyl-CoA isomerase), and thus depend on host lipids for survival (48). Skin lipid production varies during our lifetime, with a peak in the first year of life, followed by a second peak in adolescence (66–68): production is depressed during the rest of childhood, which corresponds to the period of lowest SD risk (69). In adults, the risk of SD increases substantially with age (69). This is unexpected because skin lipid levels slowly decline with age (67), so Malassezia should have increasing difficulty securing lipids in the elderly.
Azathioprine and cyclosporin, two immunosuppresive drugs which target T cells, substantially increase SD risk (39). Similarly, CD4+ T cell counts are inversely associated with SD risk and severity in AIDS patients (29). Peripheral blood mononuclear cells (PBMC) from SD patients produce less IL-2 and interferon gamma (IFNγ) when exposed to Malassezia antigens as compared to age-matched controls (70), suggesting a weak type 1 helper T cell (Th1) response against Malassezia is a characteristic of SD. In healthy individuals, thymic involution reduces naive T cell production, which results in a slow decline in T cell efficacy over our lifespan (71). This can be observed in part by measuring T cell receptor (TCR) diversity (71, 72). T cell immunosenescence can explain why SD risk increases with age, despite declining skin lipids. SD seems to be mainly due to the combination of ample lipids (29, 42, 43) and weak T cell-mediated control (70, 73, 74) of Malassezia, which together allow this fungus to over proliferate on the skin.
Seborrheic dermatitis (SD) is a well-known symptom associated with PD: PD patients have ~50% SD prevalence, while controls only have ~3% prevalence (44, 45). Though these are the most frequently cited figures, they are based on old studies whose accuracy has drawn criticism (75). We only found one recent study which measured the association between PD and SD (46). It reported that SD increases the risk of a subsequent PD diagnosis (OR = 1.69, 95% CI 1.36, 2.1; p < 0.001). This association remained significant when the SD diagnosis was made at least 5 years before the PD diagnosis, suggesting PD-associated treatments or behavior cannot explain it (46). This study reported an SD diagnosis rate of 4% prior to a PD diagnosis, as compared to 2.5% for age matched controls (46). This low rate of association indicates factors predisposing to SD and PD are mostly different. Of note, this report (46) is only available as an abstract and has not been published as a peer-reviewed article. Specific mechanisms underlying the association between SD and PD are not known (75). Nonetheless, this association suggests that mechanisms allowing Malassezia to over proliferate and cause SD (ample lipids and T cell immunosenescence) should be thoroughly investigated as possible mechanisms underlying PD as well.
Over proliferation of normally well-tolerated microbes is frequently observed in immunodeficient patients. The very first symptoms of AIDS often involve the over proliferation of fungi, such as Candida in the mouth (27), Malassezia on the skin (28, 29), or Pneumocystis in the lungs (30). Such fungi are normally present in healthy individuals (49, 76, 77), and are considered benign members of the human microbiome.
Latent microbes which are not considered part of the normal microbiome can also cause severe illness in AIDS patients. Toxoplasma gondii is a protist which colonizes the CNS of a large subset of the population for life (31). It rarely causes severe symptoms in immunocompetent individuals, but often causes life-threatening encephalitis in AIDS patients or during immunosuppression (31). Though much rarer today, Treponema pallidum (syphilis) also colonizes the CNS of infected individuals for life, typically without causing symptoms until old age (78). AIDS hastens neurosyphilis in previously asymptomatic Treponema pallidum carriers, placing young patients at an unusually high risk of this disease (32).
These well-studied secondary infections in AIDS indicate that CD4+ T cell-mediated control of latent microbes—including Toxoplasma gondii and Treponema pallidum in the CNS—is critical for our health. Malassezia have only recently been recognized as present within the body, so the effect of AIDS on Malassezia populations beyond the skin has not been investigated. The only such study we could find reported marked increase in Malassezia levels in stool of AIDS patients (51). This suggests CD4+ T cell-mediated control of Malassezia may be necessary to maintain well-tolerated Malassezia populations within the body, as well as on the skin. If Malassezia are involved in diseases of internal organs, AIDS would be expected to precipitate such diseases in genetically susceptible individuals—similarly to how it precipitates SD by allowing Malassezia to over proliferate on the skin. Interestingly, recent studies (reviewed in the next two sections) implicate Malassezia in arthritis (62) and demyelination (19), two common AIDS symptoms where no secondary infections were thought to be present.
AIDS increases the risk of developing early onset parkinsonism (79–82). Unlike sporadic PD, AIDS-associated parkinsonism progresses rapidly and affects young individuals (79). This raises the possibility that if Malassezia are present in the CNS (23, 24), their over proliferation might contribute to parkinsonism in AIDS patients, in a similar way that Malassezia on the skin contribute to SD in these patients (28, 29). This putative mechanism implies that poor CD4+ T cell-mediated control of Malassezia populations would be an important precipitating factor for PD and SD, occurring gradually in normal aging (through immunosenescence) and much more suddenly in AIDS (through lack of CD4+ T cells).
Spondyloarthritides (SpA) are a group chronic immune-mediated diseases mainly driven by alpha beta T cells recognizing intracellular peptides through HLA-B*27 presentation (62, 83). Affected organs include the spine, joints, skin, eyes, gut, and prostate (62). Historically, isolated inflammation of the eyes, gut, and skin—respectively acute anterior uveitis, inflammatory bowel disease (Crohn's disease [CD] and ulcerative colitis) and psoriasis—were considered separate diseases unrelated to SpA. However, SpA, acute anterior uveitis, inflammatory bowel disease and psoriasis run together in families (84), and share many polymorphisms in genes controlling T cell activation (85), strongly suggesting that they are the same immunological pathology (84). In particular, the fact that HLA-B*27 increases the risk of each disease strongly suggests the same antigens are being targeted (62). Varied lines of evidence support the presence of an elusive necessary intracellular fungal infection in each affected organ, which is efficiently detected by HLA-B*27 and CARD9 (62). CARD9 is an essential signaling protein for fungal immunity: homozygous loss-of-function CARD9 mutations cause severe mycoses (37). CARD9 polymorphisms are associated with inflammatory bowel disease and SpA (62, 64). Oral antifungal drugs are effective in psoriasis (86–89), psoriatic arthritis (89, 90), and likely in Crohn's disease as well (91).
In inflammatory bowel disease and psoriasis, strong evidence points to Malassezia as being the causative genus (62). Enteric Malassezia is strongly associated with CD (54, 64) and ulcerative colitis (63). Immune recognition of Malassezia occurs specifically through CARD9 in the gut, and knocking out CARD9 in mice abrogates colitis symptoms following exposure to Malassezia (64). In CD, CARD9 risk alleles increase PBMC secretion of tumor necrosis factor alpha (TNF-α) following Malassezia antigen challenges (64). Antibodies against Malassezia are associated with CD (64) and psoriasis (92, 93). Applying lysed Malassezia to the skin provokes psoriasis lesions in susceptible individuals (94). The only known fungus which is commonly present in the gut (61) and on the skin (49), and thus can explain why Crohn's patients develop psoriasis during vedolizumab treatment (95), is Malassezia. Finally, PBMCs in psoriasis react strongly to Malassezia antigens (96). These data suggest that a dysregulated immune response against Malassezia in the gut is causative in CD (64).
CD and SpA provide three key insights for PD. First, they demonstrate that Malassezia can cause diseases of internal organs in genetically predisposed individuals. Second, the increased risk of psoriasis in overweight individuals (97–100), and the increased risk of CD in carriers of certain LRRK2 alleles (101, 102) are most simply explained by enhanced lipid availability (103) which promotes Malassezia's growth by supplying it with lipids [the same LRRK2 alleles increase PD risk (101, 102)]. Third, intracellular melanin reminiscent of neuromelanin (104) is associated with inflammation of the prostate (104–106). Though indigenous production of melanin by human cells has been proposed as an explanation (104), a second possible origin would be from Malassezia which have colonized the prostate and CNS. Malassezia produce DOPA-melanin from L-DOPA (107). Both prostate epithelial cells and dopamine neurons contain intracellular lipid droplets which can fulfill Malassezia's requirement for lipids.
MS has many direct links with the immune response against fungi (18) and with SpA (62). The distribution of the age at onset of MS (108) is nearly identical to ankylosing spondylitis (109) and CD (110). The fungicidal compound dimethyl fumarate is effective in psoriasis (111), psoriatic arthritis (89, 90), and MS (112). MS is moderately associated with SpA (113, 114), inflammatory bowel disease (115, 116), and psoriasis (115, 117). These associations are surprising because SpA shares few genetic susceptibility loci with MS (85), and unlike SpA, MS is mainly B cell-mediated (18). The simplest explanation is that MS shares a necessary environmental factor with SpA (113), such as colonization of internal organs by Malassezia (19).
A recently published study compared fungi in the CNS of MS patients vs. controls, and found Malassezia in 9 of 10 MS cases, and in 1 of 9 controls (OR = 72, 95% CI 3.8–1,350, p = 0.0011) (23). Myelin producing Schwann cells are lipid-rich (118), thus can fulfill Malassezia's requirement for lipids.
Unlike in SpA, direct links between Malassezia and MS are currently limited to a single study (23). The role of Malassezia in MS is mainly supported by the many associations between MS and SpA (62), which suggest the same fungal infection is necessary for both (62). This means Malassezia likely cross the blood-brain-barrier and colonize the CNS. The closely related fungus Cryptococcus neoformans survives phagocytosis, and uses macrophages to move within the body and CNS (119). A recent study reported that Malassezia also survive phagocytosis (120), suggesting they might use macrophages to reach the CNS.
Seborrhea is defined as an elevated sebum secretion rate (SER) (121). To explain the association between SD and PD, Burton and colleagues proposed that SER could be increased by neuroendocrine stimulation via the parasympathetic system (122). Seventeen years later, the same group stated that “There is no evidence of a neural control of sebaceous function,” abandoning this hypothesis (123). This hypothesis was also excluded by Martignoni and colleagues who found little evidence of increased SER in PD (124), nor any relation with symptom severity or treatment type (124): “Sebum excretion does not appear to be related to the abnormalities of the autonomic nervous system” (124).
The two largest studies of SER in PD found no association in women (124, 125). Both studies stratified male subjects and found an association in only a subset of men (124, 125). Smaller studies reported conflicting results (126–128). Some studies reported that the administration of levodopa decreased SER (122, 125, 129), while others found no effect (124, 130).
Seborrhea seems to have little to do with SD because SER is unrelated to SD (121, 123)—although seborrhea and SD occur in the same skin regions, increased lipid secretion rates are not a characteristic of SD, strongly suggesting that seborrhea and SD are completely different clinical entities (121, 123). Malassezia can cause SD under a wide range of sebum conditions (123), and a reduction in sebum level below the physiological range is required to abrogate SD symptoms (123). This lead Cowley and colleagues to propose that limited movement might be one of the main causes of SD observed in a variety of neurological disorders, including PD (123). This hypothesis is strongly supported by SD onset observed shortly after cervical cord injuries (131), which was attributed to changes in hygiene rather than changes in SER (131).
Though the link between subjective seborrhea and PD has been reported in older studies (75), the fact that seborrhea and SD were considered synonymous (75) means these studies cannot be used to evaluate SER in PD. Therefore, we have found no compelling evidence of increased SER in PD. If such an increase exists, it must be of a small magnitude, requiring large studies to detect, and measure.
Moreover, because SER is not associated with SD (121), small changes in SER are an unlikely explanation for the association between PD and SD (123). Changes in SER fail to explain the increase in SD risk with age (69): SER decreases 2-fold between puberty and 50 years of age (67). Weak T cell-mediated control of Malassezia thus appears to be the main contributing factor to SD in older adults (70, 73, 74). This means reduced movement is a much more plausible contributor to SD in neurological diseases (123) and spinal cord injuries (131) than increased SER. We propose two non-SER mechanisms which could explain how reduced movement increases SD risk: [1] changes in hygiene might promote Malassezia's growth, possibly by allowing sebum to accumulate; [2] reduced movement hampers lymph flow (132), which might prevent CD4+ T cells controlling Malassezia populations from activating and reaching the skin. Regardless of the mechanism of SD in movement-impaired individuals, an SD diagnosis increases the risk of a PD diagnosis many years later (46), suggesting that reduced movement cannot entirely explain the association between PD and SD.
Beyond the strong association between PD and SD, and preliminary evidence of Malassezia's presence inside the CNS, three additional lines of evidence support a direct contribution of Malassezia in PD: [1] many PD risk alleles affect lipid metabolism (Malassezia are lipophilic), [2] Malassezia invasiveness and melanin production are both stimulated by L-DOPA (L-DOPA is naturally abundant in the substantia nigra), and [3] low CD4+ T cell counts observed in PD might contribute to the over proliferation of microbes such as Malassezia.
Malassezia are lipid-dependent fungi which consume host lipids by secreting extracellular lipases (48, 50, 133). On the skin, they consume extracellular lipids in sebum (50), though they can also utilize intracellular lipids by invading keratinocytes (133). The best documented cases of Malassezia inside the body were reported following lipid-rich parenteral nutrition (34). In these cases, Malassezia were mainly found within the wall of arteries which contained lipid deposits, suggesting high lipid concentrations are required to sustain Malassezia in tissue (134).
If Malassezia reach the CNS, ample access to lipids might allow them to take-hold or over proliferate, as observed in arteries during lipid-rich parenteral nutrition and on the skin in SD. Among other cellular functions, genes associated with PD risk often impact lipid metabolism. Polymorphisms in GBA, LRRK2, and PINK1—three important genetic risk factors of PD (135)—increase the concentration of intracellular lipids. GBA risk alleles have been most studied in Gaucher's disease, an autosomal recessive disorder which is caused by an abnormal accumulation of lipids in lysosomes due to defects in the β-glucocerebrosidase enzyme (which GBA encodes) (136). β-glucocerebrosidase hydrolyses the beta-glucosidic linkage of glucocerebroside, breaking it into ceramide and glucose (136). This is a key step in lipid metabolism: glucocerebroside accumulates within cells—especially macrophages—in the absence of β-glucocerebrosidase (136). Glucocerebroside typically contain long chain saturated fatty acids (137). Malassezia lack fatty acid synthase genes, so they depend on an external source of long-chain saturated fatty acids to grow (48, 138). We speculate that cells which contain high amounts of glucocerebroside are more susceptible to colonization by Malassezia. LRRK2 risk allele Y1699C phosphorylates Rab8a at T72, which promotes formation of large lipid droplets (103). PINK1 risk alleles increase the size and count of lipid droplets by reducing PGC-1a-mediated mitochondrial fatty acid oxidation (139). The newly recognized genetic risk factor SPG11 also causes an abnormal accumulation of lipids in lysosomes (140). SNCA risk alleles, another important genetic risk factor of PD, render lipid droplets more permeable to lipases (141) The alpha-synuclein variants they encode are less effective at coating such droplets (141), perhaps giving Malassezia easier access to this essential nutrient source, which they consume by secreting lipases (48, 50, 133).
Interestingly, four LRRK2 risk alleles in PD also increase the risk of CD (101, 102). CD is likely caused by a T cell-mediated immune response against Malassezia in the gut (62, 64). It is important to note that only LRRK2 PD risk allele Y1699C has been tested for lipid-droplet formation, and that LRRK2 alleles associated with both CD and PD (N551K, R1398H, N2081D, G2019S) have not been tested for excess lipid-droplet formation. It is also important to note that the most important LRRK2 PD risk allele G2019S did not reach statistical significance in one of the CD studies (101): “The LRRK2 N2081D CD risk allele is located in the same kinase domain as G2019S, a mutation that is the major genetic cause of familial and sporadic PD […]. Notably, G2019S did not have nominally significant CD association (P = 0.12), likely because of subtle stochastic fluctuations in allele frequencies during imputation.” However, it amply reached statistical significance (p = 0.0014) in the other CD study (102).
Neuromelanin is abundantly present in neurons of the substantia nigra (142, 143). Though neuromelanin's precise role in PD pathogenicity is not known, neurons containing this pigment seem to die as the disease progresses (144, 145).
Neuromelanin concentration and distribution within dopamine neurons increase with age, beginning in childhood (142, 143). The origin and biochemical process through which neuromelanin is synthesized remains a matter of debate, though the simplest explanations of tyrosinase-mediated synthesis and autoxidation have been deemed unlikely (144). In contrast with peripheral melanin, neuromelanin is often collocated with lipids [in particular dolichols (146)] and is not produced by nor kept within melanosomes (144).
Intriguingly, Malassezia was found in vitro to produce melanin from exogenous L-DOPA (107). In addition, L-DOPA triggered hyphal growth (107) which is thought to be the most invasive Malassezia morphology, allowing it to penetrate host cells and tissue. This raises the possibility that Malassezia, if present in the CNS, would be more invasive in naturally L-DOPA rich regions such as catecholaminergic neurons of the substantia nigra (147). Neuromelanin in the substantia nigra might be produced by Malassezia following invasion of dopamine neurons, as Malassezia consume intracellular lipid droplets in these neurons. Since neurons are long-lived, melanin of fungal origin could accumulate following repeated transient exposure to Malassezia. A similar mechanism could explain the presence of melanin in the basal region of prostate secretory epithelial cells, where lipid droplets are found (104).
AIDS patients are at increased risk of parkinsonism (79): about 5–15% are affected (82). AIDS-induced parkinsonism differs from sporadic PD in several respects: bradykinesia and rigidity are usually symmetrical, postural instability and gait abnormalities occur earlier, and onset can occur at a much younger age (79).
A small post-mortem study reported a higher fraction of pigmented cells in the pars compacta of the substantia nigra in AIDS patients (as compared to age-matched controls) (80). It also reported lower neuronal density in AIDS patients, suggesting neuronal loss (80). Another small post-mortem study reported lower dopamine levels in the caudate nucleus tissue in AIDS patients (as compared to age-matched controls) (81), which was attributed to the loss of nigrostriatal dopamine neurons.
AIDS-induced parkinsonism usually occurs when CD4+ T cell counts are very low (82). Interestingly, peripheral blood CD4+ T cell counts in sporadic PD are also depressed (148–150), though not as much as in AIDS. This suggests CD4+ T cells are protective in PD, and prevent or slow dopamine neuron loss. Age-related CD4+ T cell immunosenescence could thus partly explain why PD risk increases with age.
While most human cells are genetically identical, CD4+ T cells have different TCR genes produced through V(D)J recombination, allowing subsets of CD4+ T cells to recognize specific MHC class II restricted peptides. This means a small subset of CD4+ T cells recognize Malassezia peptides, another small subset recognizes Candida peptides, and so on (Figure 1). In AIDS, CD4+ T cell subsets recognizing each microbial species are equally vulnerable to depletion (Figure 1). This results in accrued susceptibility to a variety of infections which would not usually be life-threatening, but can prove fatal when they affect a vital organ of an AIDS patient.
Figure 1. Model of CD4+ T cell immunosenescence, which illustrates how CD4+ T sensitivity to different microbes can change. Each column represents a sub-population of naïve CD4+ T cells which has an identical T cell receptor (TCR). Some TCRs are cognate with Malassezia peptides (green), others with Candida peptides (orange) or Treponema peptides (blue). Recognized peptides will vary between individuals based on their MHC Class II alleles. This model can explain early onset seborrheic dermatitis, parkinsonism and neurosyphilis in AIDS patients, as the concentration of all TCRs decline. It can also explain why seborrheic dermatitis and Parkinson's disease are so strongly associated in immunocompetent individuals: the concentrations of TCRs cognate with Malassezia peptides decline, allowing Malassezia to over proliferate due to a weak CD4+ T cell-mediated immune response. This has been measured in seborrheic dermatitis (70), but not in Parkinson's disease. These data were manually generated for illustrative purposes only (they are not measurements from patients).
Though low CD4+ T cell counts in PD (148–150) suggest that the concentration of all CD4+ T cells matters, we propose that the concentration and efficacy of the CD4+ T cell subset which recognizes Malassezia peptides is the most important precipitating factor in PD and SD (Figure 2). This provides a simple explanation for the marked increase in risk of both PD and SD in AIDS patients, and for the strong epidemiological association between PD and SD in immunocompetent individuals.
Figure 2. These plots illustrate how the green region of Figure 1 is expected to vary with age (mainly due to thymic involution and immunosenescence). In the top plot, the seborrheic dermatitis (SD) threshold is reached first, which means SD will precede Parkinson's disease (PD). In the bottom plot, the PD threshold is reached first, which means PD will precede SD. These thresholds are likely influenced by genes controlling the strength of Th1 immunity against Malassezia. The PD threshold could also be affected by lipid levels (modulated by GBA, LRRK2, PINK1, SPG11) and lipid droplet accessibility by lipases (modulated by SNCA). The low rate of SD diagnosis preceding a PD diagnosis [4%] reported by Tanner and colleagues (46) suggests that PD and SD share few genetic/environmental risk factors, and that the PD threshold is usually crossed first in individuals who develop PD.
The strong epidemiological association between PD and SD suggests these two diseases share risk factors or underlying mechanisms (Table 1). The best established shared risk factor is AIDS, which greatly increases the risk of both parkinsonism and SD in young individuals. A weak CD4+ T cell-mediated immune response thus seems to be a key common mechanism. SD occurs mainly in lipid-rich areas of the skin (42), and several PD risk alleles either cause an abnormal accumulation of intracellular lipids (GBA, LRRK2, PINK1, SPG11) (103, 136, 139, 140) or increase lipase permeability of lipid droplets (SNCA) (141). This suggests a second shared mechanism is increased lipid availability. The main cause of SD is the over proliferation of the fungus Malassezia on the skin, which seems partly driven by a weak T cell-mediated immune response, and partly driven by an abundance of lipids which are required for Malassezia's growth. The main cause or causes of PD are not known.
Evidence related to CD, SpA, and MS reviewed here support both the presence and potential pathogenicity of Malassezia in internal organs, including in the CNS. PD and CD share LRRK2 susceptibility alleles (101, 102). Abnormal lipid droplet accumulation associated with the LRRK2 Y1699C allele (103) suggests a genetically determined increased in intracellular lipids might enable Malassezia over proliferation in the CNS and gut. It is important to note however that abnormal lipid droplet accumulation has not been tested for any other LRRK2 risk allele (103). Two very recent studies report finding Malassezia in the CNS in association with MS (23) and Alzheimer's disease (24). If Malassezia are found in the substantia nigra, this would strongly support the hypothesis that Malassezia are a necessary factor in PD.
Idiopathic AIDS symptoms such as parkinsonism, demyelination and arthritis could be explained by poor CD4+ T cell-mediated control of Malassezia populations in internal organs. Similarly, increased risk of PD and neurosyphilis in older adults could be explained by gradual immunosenescence, in particular the loss of CD4+ T cell subsets which control specific microbe populations such as Malassezia and Treponema. AIDS can be seen as a markedly accelerated form of immunosenescence, which precipitates certain diseases of aging in young individuals. The strong association between SD and PD, and the increased risk of SD and PD in AIDS patients, are most simply explained by waning CD4+ T cell control of Malassezia populations.
Using SD as a model disease (70), we do not expect to find strong immunological evidence of Malassezia's role in PD, because demonstrating a weak immune response is more difficult than demonstrating a robust response. This contrasts with SpA and MS where even small studies report strong adaptive immune responses against fungi (18, 62). For example, interferon gamma (IFNγ) released by PBMC exposed to Malassezia is markedly elevated in psoriasis (96)—so much that this assay can be used as a reliable biomarker of psoriasis (96). IFNγ released by PBMC exposed to Malassezia is moderately depressed in SD (70)—this could also be used as biomarker of SD, though it would be much less sensitive and specific than in psoriasis. These biomarkers suggest psoriasis is partly caused by an exaggerated Th1 response against Malassezia, while SD is partly caused by an insufficient Th1 immune response against Malassezia—and the Th1 response against Malassezia in healthy skin is just right. We expect to find moderately depressed IFNγ release when PBMC from PD patients are exposed to Malassezia, while responses to other microbes are expected to be similar. We also expect this biomarker to be predictive of future PD risk.
Once Malassezia's presence in the CNS is confirmed, and a weak Th1 response against Malassezia in PD patients who do not have SD is demonstrated, the key additional proof of Malassezia's involvement will be the efficacy of CNS-penetrant antifungal drugs such as voriconazole in preventing PD or slowing PD progression. It is imperative to confirm the presence of Malassezia in the CNS, especially in the substantia nigra. Metagenomics is a promising new approach to detect microbes in clinical specimens (152–155), and could be applied to post-mortem CNS tissue to test for Malassezia. Several important idiopathic diseases have recently been attributed to previously unsuspected infections, such as Helicobacter pylori in gastric ulcers and human papillomaviruses in cervical cancer. In both cases, these discoveries led to effective new treatments. Confirming Malassezia's role in PD would open many new treatment avenues.
First draft was written by ML after discussion with FC and JB-L. All the authors critiqued and revised the draft.
The authors declare that the research was conducted in the absence of any commercial or financial relationships that could be construed as a potential conflict of interest.
1. Twelves D, Perkins KS, Counsell C. Systematic review of incidence studies of Parkinson's disease. Mov Disord. (2003) 18:19–31. doi: 10.1002/mds.10305
2. Hirsch L, Jette N, Frolkis A, Steeves T, Pringsheim T. The incidence of Parkinson's disease: a systematic review and meta-analysis. Neuroepidemiology. (2016) 46:292–300. doi: 10.1159/000445751
3. de Rijk M, Launer L, Berger K, Breteler M, Dartigues J, Baldereschi M, et al. Prevalence of Parkinson's disease in Europe: a collaborative study of population-based cohorts. Neurologic diseases in the elderly research group. Neurology. (2000) 54(11 Suppl 5):S21–3.
4. Savica R, Grossardt BR, Bower JH, Ahlskog JE, Rocca WA. Time trends in the incidence of Parkinson disease. JAMA Neurol. (2016) 73:981–9. doi: 10.1001/jamaneurol.2016.0947
5. Ehringer H, Hornykiewicz O. Verteilung von Noradrenalin und Dopamin (3-Hydroxytyramin) im Gehirn des Menschen und ihr Verhalten bei erkrankungen des extrapyramidalen systems. Klinische Wochenschrift. (1960) 38:1236–9. doi: 10.1007/BF01485901
6. Birkmayer W, Hornykiewicz O. The L-3, 4-dioxyphenylalanine (DOPA)-effect in Parkinson-akinesia. Wiener klinische Wochenschrift. (1961) 73:787–8.
7. Keller MF, Saad M, Bras J, Bettella F, Nicolaou N, Simón-Sánchez J, et al. Using genome-wide complex trait analysis to quantify ‘missing heritability’in Parkinson's disease. Hum Mol Genet. (2012) 21:4996–5009. doi: 10.1093/hmg/dds335
8. Dickman MS. von Economo encephalitis. Arch Neurol. (2001) 58:1696–8. doi: 10.1001/archneur.58.10.1696
9. Laurence M, Benito-León J. Epstein–barr virus and multiple sclerosis: updating pender's hypothesis. Multiple Scler Related Disord. (2017) 16:8–14. doi: 10.1016/j.msard.2017.05.009
10. Itzhaki RF, Lathe R, Balin BJ, Ball MJ, Bearer EL, Braak H, et al. Microbes and Alzheimer's disease. J Alzheimer Dis. (2016) 51:979–84. doi: 10.3233/JAD-160152
11. Woulfe JM, Gray MT, Gray DA, Munoz DG, Middeldorp JM. Hypothesis: a role for EBV-induced molecular mimicry in Parkinson's disease. Parkinson Related Disord. (2014) 20:685–94. doi: 10.1016/j.parkreldis.2014.02.031
12. Friedland RP, Chapman MR. The role of microbial amyloid in neurodegeneration. PLoS Pathog. (2017) 13:e1006654. doi: 10.1371/journal.ppat.1006654
13. Pisa D, Alonso R, Rábano A, Carrasco L. Corpora amylacea of brain tissue from neurodegenerative diseases are stained with specific antifungal antibodies. Front Neurosci. (2016) 10:86. doi: 10.3389/fnins.2016.00086
14. Alonso R, Pisa D, Marina AI, Morato E, Rábano A, Carrasco L. Fungal infection in patients with alzheimer's disease. J Alzheimer Dis. (2014) 41:301–11. doi: 10.3233/JAD-132681
15. Alonso R, Pisa D, Rábano A, Carrasco L. Alzheimer's disease and disseminated mycoses. Eur J Clin Microbiol. (2014) 33:1125–32. doi: 10.1007/s10096–013-2045-z
16. Alonso R, Pisa D, Fernández-Fernández AM, Rábano A, Carrasco L. Fungal infection in neural tissue of patients with amyotrophic lateral sclerosis. Neurobiol Dis. (2017) 108:249–60. doi: 10.1016/j.nbd.2017.09.001
17. Alonso R, Pisa D, Marina AI, Morato E, Rábano A, Rodal I, et al. Evidence for fungal infection in cerebrospinal fluid and brain tissue from patients with amyotrophic lateral sclerosis. Int J Biol Sci. (2015) 11:546–58. doi: 10.7150/ijbs.11084
18. Benito-Leon J, Laurence M. The role of fungi in the etiology of multiple sclerosis. Front Neurol. (2017) 8:535. doi: 10.3389/fneur.2017.00535
19. Benito-León J, Laurence M. Malassezia in the central nervous system and multiple sclerosis. Infection. (2018) 46:443–59. doi: 10.1007/s15010-018-1196-3
20. Severance EG, Alaedini A, Yang S, Halling M, Gressitt KL, Stallings CR, et al. Gastrointestinal inflammation and associated immune activation in schizophrenia. Schizophrenia Res. (2012) 138:48–53. doi: 10.1016/j.schres.2012.02.025
21. Severance EG, Gressitt KL, Stallings CR, Katsafanas E, Schweinfurth LA, Savage CL, et al. Candida albicans exposures, sex specificity and cognitive deficits in schizophrenia and bipolar disorder. Npj Schizophr. (2016) 2:16018. doi: 10.1038/npjschz.2016.18
22. Severance EG, Gressitt KL, Stallings CR, Katsafanas E, Schweinfurth LA, Savage CL, et al. Probiotic normalization of Candida albicans in schizophrenia: a randomized, placebo-controlled, longitudinal pilot study. Brain Behav Immun. (2017) 62:41–5. doi: 10.1016/j.bbi.2016.11.019
23. Alonso R, Fernández-Fernández AM, Pisa D, Carrasco L. Multiple sclerosis and mixed microbial infections. Direct identification of fungi and bacteria in nervous tissue. Neurobio Dis. (2018) 117:42–61. doi: 10.1016/j.nbd.2018.05.022
24. Alonso R, Pisa D, Fernández-Fernández AM, Carrasco L. Infection of fungi and bacteria in brain tissue from elderly persons and patients with alzheimer's disease. Front Aging Neurosci. (2018) 10:159. doi: 10.3389/fnagi.2018.00159
25. Alonso R, Pisa D, Aguado B, Carrasco L. Identification of fungal species in brain tissue from alzheimer's disease by next-generation sequencing. J Alzheimer Dis. (2017) 58:55–67. doi: 10.3233/JAD-170058
26. Deeks SG. HIV infection, inflammation, immunosenescence, and aging. Annu Rev Med. (2011) 62:141–55. doi: 10.1146/annurev-med-042909-093756
27. Coleman DC, Bennett DE, Sullivan DJ, Gallagher PJ, Henman MC, Shanley DB, et al. Oral Candida in HIV infection and AIDS: new perspectives/new approaches. Crit Rev Microbiol. (1993) 19:61–82. doi: 10.3109/10408419309113523
28. Berger RS, Stoner MF, Hobbs ER, Hayes TJ, Boswell RN. Cutaneous manifestations of early human immunodeficiency virus exposure. J Am Acad Dermatol. (1988) 19:298–303. doi: 10.1016/S0190-9622(88)70175-9
29. Borda LJ, Wikramanayake TC. Seborrheic dermatitis and dandruff: a comprehensive review. J Clin Invest Dermatol. (2015) 3:10. doi: 10.13188/2373-1044.1000019
30. Selik RM, Starcher ET, Curran JW. Opportunistic diseases reported in AIDS patients: frequencies, associations, and trends. AIDS. (1987) 1:175–82.
31. Velimirovic B. Toxoplasmosis in immunosuppression and AIDs. Infection. (1984) 12:315–7. doi: 10.1007/BF01651143
32. Flood JM, Weinstock HS, Guroy ME, Bayne L, Simon RP, et al. Neurosyphilis during the AIDS epidemic, San Francisco, 1985–1992. J Infect Dis. (1998) 177:931–40. doi: 10.1086/515245
33. Abusleme L, Diaz PI, Freeman AF, Greenwell-Wild T, Brenchley L, Desai JV, et al. Human defects in STAT3 promote oral mucosal fungal and bacterial dysbiosis. JCI Insight. (2018) 3:122061. doi: 10.1172/jci.insight.122061
34. Shek YH, Tucker MC, Viciana AL, Manz HJ, Connor DH. Malassezia furfur—disseminated infection in premature infants. Am J Clin Pathol. (1989) 92:595–603. doi: 10.1093/ajcp/92.5.595
35. Smeekens SP, Plantinga TS, van de Veerdonk FL, Heinhuis B, Hoischen A, Joosten LA, et al. STAT1 hyperphosphorylation and defective IL12R/IL23R signaling underlie defective immunity in autosomal dominant chronic mucocutaneous candidiasis. PLoS ONE. (2011) 6:e29248. doi: 10.1371/journal.pone.0029248
36. Puel A, Cypowyj S, Bustamante J, Wright JF, Liu L, Lim HK, et al. Chronic mucocutaneous candidiasis in humans with inborn errors of interleukin-17 immunity. Science. (2011) 332:65–8. doi: 10.1126/science.1200439
37. Glocker EO, Hennigs A, Nabavi M, Schäffer AA, Woellner C, Salzer U, et al. A homozygous CARD9 mutation in a family with susceptibility to fungal infections. N Engl J Med. (2009) 361:1727–35. doi: 10.1056/NEJMoa0810719
38. Saunte D, Mrowietz U, Puig L, Zachariae C. Candida infections in patients with psoriasis and psoriatic arthritis treated with interleukin-17 inhibitors and their practical management. Br J Dermatol. (2017) 177:47–62. doi: 10.1111/bjd.15015
39. Lally A, Casabonne D, Newton R, Wojnarowska F. Seborrheic dermatitis among Oxford renal transplant recipients. J Eur Acad Dermatol Venereol. (2010) 24:561–4. doi: 10.1111/j.1468-3083.2009.03470.x
40. Grebenciucova E, Reder AT, Bernard JT. Immunologic mechanisms of fingolimod and the role of immunosenescence in the risk of cryptococcal infection: a case report and review of literature. Multiple Sclerosis Related Disord. (2016) 9:158–62. doi: 10.1016/j.msard.2016.07.015
41. Martín S, Pérez A, Aldecoa C. Sepsis and immunosenescence in the elderly patient: a review. Front Med. (2017) 4:20. doi: 10.3389/fmed.2017.00020
42. Gupta A, Bluhm R. Seborrheic dermatitis. J Eur Acad Dermatol Venereol. (2004) 18:13–26. doi: 10.1111/j.1468-3083.2004.00693.x
43. Gupta AK, Madzia S, Batra R. Etiology and management of seborrheic dermatitis. Dermatology. (2004) 208:89–93. doi: 10.1159/000076478
44. Arsenijevic VSA, Milobratovic D, Barac AM, Vekic B, Marinkovic J, Kostic VS. A laboratory-based study on patients with Parkinson's disease and seborrheic dermatitis: the presence and density of Malassezia yeasts, their different species and enzymes production. BMC Dermatol. (2014) 14:5. doi: 10.1186/1471-5945-14-5
45. A.-Ravn H, Thyssen JP, Egeberg A. Skin disorders in Parkinson's disease: potential biomarkers and risk factors. Clin Cosmet Invest Dermatol. (2017) 10:87–92. doi: 10.2147/CCID.S130319
46. Tanner C, Albers K, Goldman S, Fross R, Leimpeter A, Klingman J, et al. Seborrheic dermatitis and risk of future Parkinson's disease (PD)(S42. 001). Neurology. (2012) 78(1 Suppl):S42. 001. doi: 10.1212/WNL.78.1_MeetingAbstracts.S42.001
47. Shuster S. The aetiology of dandruff and the mode of action of therapeutic agents. Br J Dermatol. (1984) 111:235–42. doi: 10.1111/j.1365-2133.1984.tb04050.x
48. Celis Ramírez AM. Unraveling lipid metabolism in lipid-dependent pathogenic Malassezia yeasts. (Ph.D. thesis). Microbiology Group. Utretcht: Utrecht University (2017). p. 150.
49. Findley K, Oh J, Yang J, Conlan S, Deming C, Meyer JA, et al. Topographic diversity of fungal and bacterial communities in human skin. Nature. (2013) 498:367–70. doi: 10.1038/nature12171
50. Theelen B, Cafarchia C, Gaitanis G, Bassukas ID, Boekhout T, Dawson TL Jr. Malassezia ecology, pathophysiology, and treatment. Med Mycol. (2018) 56(Suppl_1):10–25. doi: 10.1093/mmy/myx134
51. Hamad I, Abdallah RA, Ravaux I, Mokhtari S, Tissot-Dupont H, Michelle C, et al. Metabarcoding analysis of eukaryotic microbiota in the gut of HIV-infected patients. PLoS ONE. (2018) 13:e0191913. doi: 10.1371/journal.pone.0191913
52. Dupuy AK, David MS, Li L, Heider TN, Peterson JD, Montano EA, et al. Redefining the human oral mycobiome with improved practices in amplicon-based taxonomy: discovery of Malassezia as a prominent commensal. PLoS ONE. (2014) 9:e90899. doi: 10.1371/journal.pone.0090899
53. Cleland EJ, Bassioni A, Boase S, Dowd S, Vreugde S, Wormald PJ. The fungal microbiome in chronic rhinosinusitis: richness, diversity, postoperative changes and patient outcomes. Int Forum Allergy Rhinol. (2014) 259–65. doi: 10.1002/alr.21297
54. Kellermayer R, Mir SA, Nagy-Szakal D, Cox SB, Dowd SE, Kaplan JL, et al. Microbiota separation and C-reactive protein elevation in treatment naïve pediatric granulomatous Crohn disease. J Pediatr Gastroenterol Nutr. (2012) 55:243–50. doi: 10.1097/MPG.0b013e3182617c16
55. Hallen-Adams HE, Kachman SD, Kim J, Legge RM, Martínez I. Fungi inhabiting the healthy human gastrointestinal tract: a diverse and dynamic community. Fungal Ecol. (2015) 15:9–17. doi: 10.1016/j.funeco.2015.01.006
56. Suhr MJ, Banjara N, Hallen-Adams HE. Sequence-based methods for detecting and evaluating the human gut mycobiome. Lett Appl Microbiol. (2016) 62:209–15. doi: 10.1111/lam.12539
57. Sokol H, Leducq V, Aschard H, Pham PH, Jegou S, Landman C, et al. Fungal microbiota dysbiosis in IBD. Gut. (2017) 66:1039–48. doi: 10.1136/gutjnl-2015-310746
58. Liguori G, Lamas B, Richard ML, Brandi G, Da Costa G, Hoffmann TW, et al. Fungal dysbiosis in mucosa-associated microbiota of Crohn's disease patients. J Crohn Colitis. (2015) 10:296–305. doi: 10.1093/ecco-jcc/jjv209
59. Boix-Amorós A, Martinez-Costa C, Querol A, Collado MC, Mira A. Multiple approaches detect the presence of fungi in human breastmilk samples from healthy mothers. Sci Rep. (2017) 7:13016. doi: 10.1038/s41598-017-13270-x
60. van Woerden HC, Gregory C, Brown R, Marchesi JR, Hoogendoorn B, Matthews IP. Differences in fungi present in induced sputum samples from asthma patients and non-atopic controls: a community based case control study. BMC Infect Dis. (2013) 13:69. doi: 10.1186/1471-2334-13-69
61. Nash AK, Auchtung TA, Wong MC, Smith DP, Gesell JR, Ross MC, et al. The gut mycobiome of the human microbiome project healthy cohort. Microbiome. (2017) 5:153. doi: 10.1186/s40168-017-0373-4
62. Laurence M, Asquith M, Rosenbaum JT. Spondyloarthritis, acute anterior uveitis and fungi: updating the catterall king hypothesis. Front Med. (2018) 5:80. doi: 10.3389/fmed.2018.00080
63. Richard M. Malassezia in the human gut: friend or foe or artefact? In: ISHAM 2018 - Malassezia Workshop. Westerdijk Fungal Biodiversity Institute, Utrecht, Netherlands (2018).
64. Limon JJ, Tang J, Li D, Wolf AJ, Michelsen KS, Funari V, et al. Malassezia is associated with crohn's disease and exacerbates colitis in mouse models. Cell Host Microbe. (2019) 25:377–88.e6. doi: 10.1016/j.chom.2019.01.007
65. Tajima M, Sugita T, Nishikawa A, Tsuboi R. Molecular analysis of Malassezia microflora in seborrheic dermatitis patients: comparison with other diseases and healthy subjects. J Invest Dermatol. (2008) 128:345–51. doi: 10.1038/sj.jid.5701017
66. Ramasastry P, Downing DT, Pochi PE, Strauss JS. Chemical composition of human skin surface lipids from birth to puberty. J Invest Dermatol. (1970) 54:139–44. doi: 10.1111/1523-1747.ep12257164
67. Jacobsen E, Billings JK, Frantz RA, Kinney CK, Stewart ME, Downing DT. Age-related changes in sebaceous wax ester secretion rates in men and women. J Invest Dermatol. (1985) 85:483–5. doi: 10.1111/1523-1747.ep12277224
68. Ro BI, Dawson TL. The role of sebaceous gland activity and scalp microfloral metabolism in the etiology of seborrheic dermatitis and dandruff. J Invest Dermatol Symp Proc. (2005) 10:194–7. doi: 10.1111/j.1087-0024.2005.10104.x
69. Furue M, Yamazaki S, Jimbow K, Tsuchida T, Amagai M, Tanaka T, et al. Prevalence of dermatological disorders in Japan: a nationwide, cross-sectional, seasonal, multicenter, hospital-based study. J Dermatol. (2011) 38:310–20. doi: 10.1111/j.1346-8138.2011.01209.x
70. Neuber K, Kröger S, Gruseck E, Abeck D, Ring J. Effects of Pityrosporum ovale on proliferation, immunoglobulin (IgA, G, M) synthesis and cytokine (IL-2, IL-10, IFNγ) production of peripheral blood mononuclear cells from patients with seborrhoeic dermatitis. Arch Dermatol Res. (1996) 288:532–6. doi: 10.1007/BF02505250
71. Palmer D. The effect of age on thymic function. Front Immunol. (2013) 4:316. doi: 10.3389/fimmu.2013.00316
72. Izraelson M, Nakonechnaya TO, Davydov AN, Dronina MA, Miskevich DA, Mamedov IZ, et al. T cell immunity does not age in a long-lived rodent species. BioRxiv. (2018) 259374. doi: 10.1101/259374
73. Bergbrant IM, Johansson S, Robbins D, Scheynius A, Faergemann J, Söderström T. An immunological study in patients with seborrhoeic dermatitis. Clin Exp Dermatol. (1991) 16:331–8. doi: 10.1111/j.1365-2230.1991.tb00395.x
74. Wikler J, Trago EP, Nieboer C Cell-Mediated Deficiency To Pityrosporum Orbiculare In Patients With Seborrheic Dermatitis. In: Journal of Investigative Dermatology. Cambridge, MA: Blackwell Science Inc (1989) 92:541.
75. Mastrolonardo M, Diaferio A, Logroscino G. Seborrheic dermatitis, increased sebum excretion, and Parkinson's disease: a survey of (im) possible links. Med Hypotheses. (2003) 60:907–11. doi: 10.1016/S0306-9877(03)00094-X
76. Ponce CA, Gallo M, Bustamante R, Vargas SL. Pneumocystis colonization is highly prevalent in the autopsied lungs of the general population. Clin Infect Dis. (2010) 50:347–53. doi: 10.1086/649868
77. Akpan A, Morgan R. Oral candidiasis. Postgrad Med J. (2002) 78:455–9. doi: 10.1136/pmj.78.922.455
78. Hook EW III, Marra CM. Acquired syphilis in adults. N Engl J Med. (1992) 326:1060–9. doi: 10.1056/NEJM199204163261606
79. Tse W, Cersosimo MG, Gracies JM, Morgello S, Olanow CW, Koller W. Movement disorders and AIDS: a review. Parkinson Relat Disord. (2004) 10:323–34. doi: 10.1016/j.parkreldis.2004.03.001
80. Reyes M, Faraldi F, Senseng C, Flowers C, Fariello R. Nigral degeneration in acquired immune deficiency syndrome (AIDS). Acta Neuropathol. (1991) 82:39–44. doi: 10.1007/BF00310921
81. Sardar AM, Czudek C, Reynolds GP. Dopamine deficits in the brain: the neurochemical basis of parkinsonian symptoms in AIDS. NeuroRep. (1996) 7:910–2. doi: 10.1097/00001756-199603220-00015
82. Mirsattari SM, Power C, Nath A. Parkinsonism with HIV infection. Mov Disord. (1998) 13:684–9. doi: 10.1002/mds.870130413
83. Evans DM, Spencer CC, Pointon JJ, Su Z, Harvey D, Kochan G, et al. Interaction between ERAP1 and HLA-B27 in ankylosing spondylitis implicates peptide handling in the mechanism for HLA-B27 in disease susceptibility. Nat Genet. (2011) 43:761–7. doi: 10.1038/ng0911-919a
84. Elewaut D. Linking Crohn's disease and ankylosing spondylitis: it's all about genes! PLoS Genet. (2010) 6:e1001223. doi: 10.1371/journal.pgen.1001223
85. International Genetics of Ankylosing Spondylitis Consortium (IGAS)1, Cortes A, Hadler J, Pointon JP, Robinson PC, Karaderi T, et al. Identification of multiple risk variants for ankylosing spondylitis through high-density genotyping of immune-related loci. Nat Genet. (2013) 45:730–8. doi: 10.1038/ng.2667
86. Rosenberg EW, Belew PW. Improvement of psoriasis of the scalp with ketoconazole. Arch Dermatol. (1982) 118:370–1. doi: 10.1001/archderm.118.6.370
87. Crutcher N, Rosenberg EW, Belew PW, Skinner RB Jr, Eaglstein NF, Baker SM. Oral nystatin in the treatment of psoriasis. Arch Dermatol. (1984) 120:435–6. doi: 10.1001/archderm.1984.01650400017002
88. Ascioglu O, Soyuer U, Aktas E. Improvement of psoriasis with oral nystatin. In: Tümbay E, Seeliger HPR, Anǧ Ö editors. Candida and Candidamycosis. Boston, MA: Springer (1991) 279–81.
89. Altmeyer PJ, Mattlies U, Pawlak F, Hoffmann K, Frosch PJ, Ruppert P, et al. Antipsoriatic effect of fumaric acid derivatives: results of a multicenter double-blind study in 100 patients. J Am Acad Dermatol. (1994) 30:977–81. doi: 10.1016/S0190-9622(94)70121-0
90. Peeters A, Dukmans B, Van der Schroeff J. Fumaric acid therapy for psoriatic arthritis. A randomized, double-blind, placebo-controlled study. Rheumatology. (1992) 31:502–4. doi: 10.1093/rheumatology/31.7.502
91. Samuel S, Loftus E Jr, Sandborn W. The effects of itraconazole on inflammatory bowel disease activity in patients treated for histoplasmosis. Aliment Pharmacol Therapeut. (2010) 32:1207–9. doi: 10.1111/j.1365-2036.2010.04444.x
92. Squiquera L, Galimberti R, Morelli L, Plotkin L, Milicich R, Kowalckzuk A, et al. Antibodies to proteins from Pityrosporum ovale in the sera from patients with psoriasis. Clin Exp Dermatol. (1994) 19:289–93. doi: 10.1111/j.1365-2230.1994.tb01197.x
93. Liang Y, Wen H, Xiao R. Serum levels of antibodies for IgG, IgA, and IgM against the fungi antigen in psoriasis vulgaris. Bull Human Med Univer. (2003) 28:638–40.
94. Lober CW, Belew PW, Rosenberg EW, Bale G. Patch tests with killed sonicated microflora in patients with psoriasis. Arch Dermatol. (1982) 118:322–5. doi: 10.1001/archderm.118.5.322
95. Tadbiri S, Peyrin-Biroulet L, Serrero M, Filippi J, Pariente B, Roblin X, et al. Impact of vedolizumab therapy on extra-intestinal manifestations in patients with inflammatory bowel disease: a multicentre cohort study nested in the OBSERV-IBD cohort. Aliment Pharmacol Therapeut. (2018) 47:485–93. doi: 10.1111/apt.14419
96. Kanda N, Tani K, Enomoto U, Nakai K, Watanabe S. The skin fungus-induced Th1-and Th2-related cytokine, chemokine and prostaglandin E2 production in peripheral blood mononuclear cells from patients with atopic dermatitis and psoriasis vulgaris. Clin Exp Allergy. (2002) 32:1243–50. doi: 10.1046/j.1365-2745.2002.01459.x
97. Gelfand JM, Yeung H. Metabolic syndrome in patients with psoriatic disease. J Rheumatol Suppl. (2012) 89:24–8. doi: 10.3899/jrheum.120237
98. Setty AR, Curhan G, Choi HK. Obesity, waist circumference, weight change, and the risk of psoriasis in women: Nurses' Health Study II. Arch Int Med. (2007) 167:1670–5. doi: 10.1001/archinte.167.15.1670
99. Romero-Talamás H, Aminian A, Corcelles R, Fernandez AP, Schauer PR, Brethauer S Psoriasis improvement after bariatric surgery. Surg Obesity Relat Dis. (2014) 10:1155–9. doi: 10.1016/j.soard.2014.03.025
100. Budu-Aggrey A, Brumpton B, Tyrrell J, Watkins S, Modalsli EH, Celis-Morales C, et al. Evidence of a causal relationship between body mass index and psoriasis: a Mendelian randomization study. PLoS Med. (2019) 16:e1002739. doi: 10.1371/journal.pmed.1002739
101. Hui KY, Fernandez-Hernandez H, Hu J, Schaffner A, Pankratz N, Hsu NY, et al. Functional variants in the LRRK2 gene confer shared effects on risk for Crohn's disease and Parkinson's disease. Sci Transl Med. (2018) 10:eaai7795. doi: 10.1126/scitranslmed.aai7795
102. Rivas MA, Avila BE, Koskela J, Huang H, Stevens C, Pirinen M, et al. Insights into the genetic epidemiology of Crohn's and rare diseases in the Ashkenazi Jewish population. PLoS Genet. (2018) 14:e1007329. doi: 10.1371/journal.pgen.1007329
103. Yu M, Arshad M, Wang W, Zhao D, Xu L, Zhou L. LRRK2 mediated Rab8a phosphorylation promotes lipid storage. Lipids Health Dis. (2018) 17:34. doi: 10.1186/s12944-018-0684-x
104. Seman G, Gallager HS, Johnson DE. Melanin-like pigment in the human prostate. Prostate. (1982) 3:59–72. doi: 10.1002/pros.2990030109
105. Guillan R, Zelman S. The incidence and probable origin of melanin in the prostate. J Urol. (1970) 104:151–3. doi: 10.1016/S0022-5347(17)61689-6
106. Maksem JA, Johenning PW, Galang CF. Prostatitis and aspiration biopsy cytology of prostate. Urology. (1988) 32:263–8. doi: 10.1016/0090-4295(88)90398-6
107. Youngchim S, Nosanchuk JD, Pornsuwan S, Kajiwara S, Vanittanakom N. The role of L-DOPA on melanization and mycelial production in Malassezia furfur. PLoS ONE. (2013) 8:e63764. doi: 10.1371/journal.pone.0063764
108. Confavreux C, Vukusic S. Natural history of multiple sclerosis: a unifying concept. Brain. (2006) 129(Pt 3):606–16. doi: 10.1093/brain/awl007
109. Feldtkeller E, Khan MAD, van der Heijde S, van der L, Braun J. Age at disease onset and diagnosis delay in HLA-B27 negative vs. positive patients with ankylosing spondylitis. Rheumatol Int. (2003) 23:61–6. doi: 10.1007/s00296–002-0237–4
110. Chouraki V, Savoye G, Dauchet L, Vernier-Massouille G, Dupas JL, Merle V, et al. The changing pattern of Crohn's disease incidence in northern France: a continuing increase in the 10- to 19-year-old age bracket (1988–2007). Aliment Pharmacol Therapeut. (2011) 33:1133–42. doi: 10.1111/j.1365-2036.2011.04628.x
111. Balak D, Fallah Arani S, Hajdarbegovic E, Hagemans C, Bramer W, Thio H, et al. Efficacy, effectiveness and safety of fumaric acid esters in the treatment of psoriasis: a systematic review of randomized and observational studies. Br J Dermatol. (2016) 175:250–62. doi: 10.1111/bjd.14500
112. Gold R, Kappos L, Arnold DL, Bar-Or A, Giovannoni G, Selmaj K, et al. Placebo-controlled phase 3 study of oral BG-12 for relapsing multiple sclerosis. N Engl J Med. (2012) 367:1098–107. doi: 10.1056/NEJMoa1114287
113. Hanrahan P, Russell A, McLean D. Ankylosing spondylitis and multiple sclerosis: an apparent association? J Rheumatol. (1988) 15:1512–4.
114. Edwards L, Constantinescu C. A prospective study of conditions associated with multiple sclerosis in a cohort of 658 consecutive outpatients attending a multiple sclerosis clinic. Multiple Sclerosis J. (2004) 10:575–81. doi: 10.1191/1352458504ms1087oa
115. Dobson R, Giovannoni G. Autoimmune disease in people with multiple sclerosis and their relatives: a systematic review and meta-analysis. J Neurol. (2013) 260:1272–85. doi: 10.1007/s00415-012-6790-1
116. Kosmidou M, Katsanos AH, Katsanos KH, Kyritsis AP, Tsivgoulis G, Christodoulou D, et al. Multiple sclerosis and inflammatory bowel diseases: a systematic review and meta-analysis. J Neurol. (2017) 264:254–9. doi: 10.1007/s00415-016-8340-8
117. Egeberg A, Mallbris L, Gislason GH, Skov L, Hansen PR. Risk of multiple sclerosis in patients with psoriasis: a Danish nationwide cohort study. J Invest Dermatol. (2016) 136:93–8. doi: 10.1038/JID.2015.350
118. O'Brien JS, Sampson EL. Lipid composition of the normal human brain: gray matter, white matter, and myelin. J Lipid Res. (1965) 6:537–44.
119. Ma H. Intracellular parasitism of macrophages by Cryptococcus. (Ph.D. thesis). University of Birmingham (2009). p. 209.
120. Moreira RTdF, Lallo MA, Alvares-Saraiva AM, Hurtado EC P, Konno FT, Spadacci-Morena D. Dichotomous response of Malassezia-infected macrophages to Malassezia pachydermatis and Malassezia furfur. Med Mycol. (2018) 57:628–35. doi: 10.1093/mmy/myy104
121. Burton J, Pye R. Seborrhoea is not a feature of seborrhoeic dermatitis. Br Med J. (1983) 286:1169–70. doi: 10.1136/bmj.286.6372.1169
122. Burton J, Cartlidge M, Cartlidge N, Shuster S. Sebum excretion in Parkinsonism. Br J Dermatol. (1973) 88:263–6.
123. Cowley N, Farr P, Shuster S. The permissive effect of sebum in seborrhoeic dermatitis, an explanation of the rash in neurological disorders. Br J Dermatol. (1990) 122:71–6. doi: 10.1111/j.1365-2133.1990.tb08241.x
124. Martignoni E, Godi L, Pacchetti C, Berardesca E, Vignoli G, Albani G, et al. Is seborrhea a sign of autonomic impairment in Parkinson's disease? J Neural Transm. (1997) 104:1295–304. doi: 10.1007/BF01294730
125. Villares J, Carlini E. Sebum secretion in idiopathic Parkinson's disease: effect of anticholinergic and dopaminergic drugs. Acta Neurol Scand. (1989) 80:57–63. doi: 10.1111/j.1600-0404.1989.tb03843.x
126. Burton J, Cartlidge M, Shuster S. Effect of L-dopa on the seborrhoea of Parkinsonism. Br J Dermatol. (1973) 88:475–9. doi: 10.1111/j.1365-2133.1973.tb15453.x
127. Streifler M, Avrami E, Rabey J. L-dopa and the secretion of sebum in Parkinsonian patients. Eur Neurol. (1980) 19:43–8. doi: 10.1159/000115127
128. Appenzeller O, Goss J. Autonomic dysfunction in Parkinson's syndrome. Transact Am Neurol Assoc. (1970) 95:200.
129. Kohn SR, Pochi PE, Strauss JS, Sax DS, Feldman RG, Timberlake WH. Sebaceous gland secretion in Parkinson's disease during L-dopa treatment. J Invest Dermatol. (1973) 60:134–6. doi: 10.1111/1523-1747.ep12682040
130. Cotterill J, Cunliffe W, Williamson B, Arrowsmith W, Cook J, Sumner D. Sebum-excretion rate and skin-surface lipid composition in Parkinson's disease before and during therapy with levodopa. Lancet. (1971) 297:1271–2. doi: 10.1016/S0140-6736(71)91782-X
131. Wilson CL, Walshe M. Incidence of seborrhoeic dermatitis in spinal injury patients. Br J Dermatol. (1988) 119:48. doi: 10.1111/j.1365-2133.1988.tb05386.x
132. Schmid-Schonbein GW. Microlymphatics and lymph flow. Physiol Rev. (1990) 70:987–1028. doi: 10.1152/physrev.1990.70.4.987
133. Borgers M, Cauwenbergh G, Van De Ven MA, Hernanz AP, Degreef H. Pityriasis versicolor and Pityrosporum ovale. Int J Dermatol. (1987) 26:586–9. doi: 10.1111/j.1365-4362.1987.tb02311.x
134. Redline RW, Redline SS, Boxerbaum B, Dahms BB. Systemic malassezia furfur infections in patients receiving intralipid therapy. Hum Pathol. (1985) 16:815–22. doi: 10.1016/S0046-8177(85)80253-7
135. Trinh J, Farrer M. Advances in the genetics of Parkinson disease. Nat Rev Neurol. (2013) 9:445. doi: 10.1038/nrneurol.2013.132
136. Hruska KS, LaMarca ME, Scott CR, Sidransky E. Gaucher disease: mutation and polymorphism spectrum in the glucocerebrosidase gene (GBA). Hum Mutat. (2008) 29:567–83. doi: 10.1002/humu.20676
137. Hirabayashi Y, Hamaoka A, Matsumoto M, Nishimura K. An improved method for the separation of molecular species of cerebrosides. Lipids. (1986) 21:710–4. doi: 10.1007/BF02537245
138. Dawson TL Jr. Malassezia globosa and restricta: breakthrough understanding of the etiology and treatment of dandruff and seborrheic dermatitis through whole-genome analysis. J Invest Dermatol Symp Proc. (2007) 12:15–9. doi: 10.1038/sj.jidsymp.5650049
139. Choi J, Ravipati A, Nimmagadda V, Schubert M, Castellani RJ, Russell JW. Potential roles of PINK1 for increased PGC-1α-mediated mitochondrial fatty acid oxidation and their associations with Alzheimer disease and diabetes. Mitochondrion. (2014) 18:41–8. doi: 10.1016/j.mito.2014.09.005
140. Branchu J, Boutry M, Sourd L, Depp M, Leone C, Corriger A, et al. Loss of spatacsin function alters lysosomal lipid clearance leading to upper and lower motor neuron degeneration. Neurobiol Dis. (2017) 102:21–37. doi: 10.1016/j.nbd.2017.02.007
141. Cole NB, Murphy DD, Grider T, Rueter S, Brasaemle D, Nussbaum RL. Lipid droplet binding and oligomerization properties of the Parkinson's disease protein α-synuclein. J Biol Chem. (2002) 277:6344–52. doi: 10.1074/jbc.M108414200
142. Fedorow H, Halliday G, Rickert C, Gerlach M, Riederer P, Double K. Evidence for specific phases in the development of human neuromelanin. Neurobiol Aging. (2006) 27:506–12. doi: 10.1016/j.neurobiolaging.2005.02.015
143. Halliday G, Fedorow H, Rickert C, Gerlach M, Riederer P, Double K. Evidence for specific phases in the development of human neuromelanin. J Neural Transm. (2006) 113:721–8. doi: 10.1007/s00702-006-0449-y
144. Fedorow H, Tribl F, Halliday G, Gerlach M, Riederer P, Double K. Neuromelanin in human dopamine neurons: comparison with peripheral melanins and relevance to Parkinson's disease. Progress Neurobiol. (2005) 75:109–24. doi: 10.1016/j.pneurobio.2005.02.001
145. Haining RL, Achat-Mendes C. Neuromelanin, one of the most overlooked molecules in modern medicine, is not a spectator. Neural Regener Res. (2017) 12:372–5. doi: 10.4103/1673-5374.202928
146. Engelen M, Vanna R, Bellei C, Zucca FA, Wakamatsu K, Monzani E, et al. Neuromelanins of human brain have soluble and insoluble components with dolichols attached to the melanic structure. PLoS ONE. (2012) 7:e48490. doi: 10.1371/journal.pone.0048490
147. Mons N, Tison F, Geffard M. Identification of L-dopa-dopamine and L-dopa cell bodies in the rat mesencephalic dopaminergic cell systems. Synapse. (1989) 4:99–105. doi: 10.1002/syn.890040203
148. Kustrimovic N, Rasini E, Legnaro M, Bombelli R, Aleksic I, Blandini F, et al. Dopaminergic receptors on CD4+ T naive and memory lymphocytes correlate with motor impairment in patients with Parkinson's disease. Sci Rep. (2016) 6:33738. doi: 10.1038/srep33738
149. Stevens CH, Rowe D, Morel-Kopp M-C, Orr C, Russell T, Ranola M, et al. Reduced T helper and B lymphocytes in Parkinson's disease. J Neuroimmunol. (2012) 252:95–9. doi: 10.1016/j.jneuroim.2012.07.015
150. Saunders JAH, Estes KA, Kosloski LM, Allen HE, Dempsey KM, Torres-Russotto DR, et al. CD4+ regulatory and effector/memory T cell subsets profile motor dysfunction in Parkinson's disease. J Neuroimmun Pharmacol. (2012) 7:927–38. doi: 10.1007/s11481-012-9402-z
151. Sidransky E, Lopez G. The link between the GBA gene and parkinsonism. Lancet Neurol. (2012) 11:986–98. doi: 10.1016/S1474-4422(12)70190-4
152. Palacios G, Druce J, Du L, Tran T, Birch C, Briese T, et al. A new arenavirus in a cluster of fatal transplant-associated diseases. N Engl J Med. (2008) 358:991–8. doi: 10.1056/NEJMoa073785
153. Lipkin WI. Microbe hunting. Microbiol Molecul Biol Rev. (2010) 74:363–77. doi: 10.1128/MMBR.00007-10
154. Cottier F, Srinivasan KG, Yurieva M, Liao W, Poidinger M, Zolezzi F, et al. Advantages of meta-total RNA sequencing (MeTRS) over shotgun metagenomics and amplicon-based sequencing in the profiling of complex microbial communities. NPJ Biofilms Microbiomes. (2018) 4:2. doi: 10.1038/s41522-017-0046-x
Keywords: Parkinson's disease, seborrheic dermatitis, Malassezia, immunodeficiency, immunosenescence
Citation: Laurence M, Benito-León J and Calon F (2019) Malassezia and Parkinson's Disease. Front. Neurol. 10:758. doi: 10.3389/fneur.2019.00758
Received: 28 October 2018; Accepted: 01 July 2019;
Published: 24 July 2019.
Edited by:
Veerle Baekelandt, KU Leuven, BelgiumReviewed by:
Laura Civiero, University of Padova, ItalyCopyright © 2019 Laurence, Benito-León and Calon. This is an open-access article distributed under the terms of the Creative Commons Attribution License (CC BY). The use, distribution or reproduction in other forums is permitted, provided the original author(s) and the copyright owner(s) are credited and that the original publication in this journal is cited, in accordance with accepted academic practice. No use, distribution or reproduction is permitted which does not comply with these terms.
*Correspondence: Martin Laurence, bWxhdXJlbmNlQHNoaXBzaGF3LmNvbQ==
Disclaimer: All claims expressed in this article are solely those of the authors and do not necessarily represent those of their affiliated organizations, or those of the publisher, the editors and the reviewers. Any product that may be evaluated in this article or claim that may be made by its manufacturer is not guaranteed or endorsed by the publisher.
Research integrity at Frontiers
Learn more about the work of our research integrity team to safeguard the quality of each article we publish.