- 1Department of Neurology, Tianjin Neurological Institute, Tianjin Medical University General Hospital, Tianjin, China
- 2Department of Respiratory, Tianjin Medical University General Hospital, Tianjin, China
- 3Neurology Department of PKU Care CNOOC Hospital, Beijing, China
- 4Department of Neurology, Tianjin First Central Hospital, Tianjin, China
- 5Department of Neurology, Tianjin Haibin People's Hospital, Tianjin, China
- 6Department of Neurology, The Fifth Central Hospital of Tianjin, Binhai Hospital of Peking University, Tianjin, China
- 7Department of Neurology, The Second Hospital of Tianjin Medical University, Tianjin, China
- 8Department of Neurology, Tianjin Third Central Hospital, Tianjin, China
- 9Department of Neurology, Tianjin Fourth Center Hospital, Tianjin, China
- 10Department of Neuroscience, Carleton University, Ottawa, ON, Canada
Objective: The goal of this study is to evaluate pulmonary function and respiratory center drive in patients with early-stage idiopathic Parkinson's disease (IPD) to facilitate early diagnosis of Parkinson's Disease (PD).
Methods: 43 IPD patients (Hoehn and Yahr scale of 1) and 41 matched healthy individuals (e.g., age, sex, height, weight, BMI) were enrolled in this study. Motor status was evaluated using the Movement Disorders Society-Unified PD Rating Scale (MDS-UPDRS). Pulmonary function and respiratory center drive were measured using pulmonary function tests (PFT). All IPD patients were also subjected to a series of neuropsychological tests, including Non-Motor Symptoms Questionnaire (NMSQ), REM Sleep Behavior Disorder Screening Questionnaire (RBDSQ), Beck Depression Inventory (BDI) and Mini Mental State Examination (MMSE).
Results: IPD patients and healthy individuals have similar forced vital capacity (FVC), forced expiratory volume in 1s (FEV1), forced expiratory volume in 1s/forced vital capacity (FEV1/FVC), peak expiratory flow (PEF), and carbon monoxide diffusion capacity (DLCOcSB). Reduced respiratory muscle strength, maximal inspiratory pressure (PImax) and maximal expiratory pressure (PEmax) was seen in IPD patients (p = 0.000 and p = 0.002, respectively). Importantly, the airway occlusion pressure after 0.1 s (P0.1) and respiratory center output were notably higher in IPD patients (p = 0.000) with a remarkable separation of measured values compared to healthy controls.
Conclusion: Our findings suggest that abnormal pulmonary function is present in early stage IPD patients as evidenced by significant changes in PImax, PEmax, and P0.1. Most importantly, P0.1 may have the potential to assist with the identification of IPD in the early stage.
Introduction
Parkinson's disease (PD) is the second most common chronic and progressive neurodegenerative disease in the elderly (1). Although the pathogenesis of PD has been redefined with triggers (e.g., viral infections or environmental toxins), facilitators (e.g., systemic inflammation, mitochondrial dysfunction, and genetic facilitators), and aggravators (e.g., impaired autophagy, neuroinflammation), there is a complex interplay of genetic, aging, and environmental factors that result in PD (2). Consequently, clinical challenges remain because it is difficult to provide a definitive diagnosis at the earliest stage. This has led to an urgent yet unmet need to find a useful examination to facilitate the early diagnosis of PD (3).
Pulmonary function impairment has been studied in idiopathic Parkinson's disease (IPD), the most common type of Parkinsonism whereby the cause is unknown (4). Pulmonary dysfunction has been known as an autonomic disorder equated to non-motor symptoms of PD in several studies. Abnormalities involving obstructive, restrictive and mixed-type pulmonary dysfunction increases disability in PD patients (5). Moreover, respiratory complications, such as aspiration pneumonia and pulmonary embolism are associated with mortality of PD patients (6). A systematic review and meta-analysis suggested asymptomatic respiratory impairment can be discovered even in the initial stages of the disease course (7). In the past decades, research on PD and lung function has brought some meaningful discoveries. Based on the experimental designs and conclusions, these studies can be classified into four main topics: first is the effect of Levodopa (combined with different formulation) on pulmonary dysfunction in PD; the second is about dysregulated lung function, especially ventilatory dysfunction, diffusion impairment, obstructive, and restrictive disorders in PD; the third is the potential relationship between swallowing impairment and respiratory dysfunction in PD; the last is the therapeutic effect of rehabilitation (e.g., YOGA, Qigong) on pulmonary function in patients with PD (8–12). It is worth mentioning that most of these studies were performed in patients in the advanced stage of PD. Currently, whether pulmonary function is affected in early stage IPD patients remains elusive.
As a valid measurement of respiratory center drive, the airway occlusion pressure (P0.1) is a simple, convenient, non-invasive measurement index. It is described as the pressure developed in the occluded airway 100 ms after the onset of inspiration (13). P0.1 is commonly monitored in respiratory diseases, along with respiratory failure and chronic obstructive pulmonary disease (COPD). It is a sensitive indicator of respiratory drive to predict extubation failure in ICU (14). However, respiratory center drive in early IPD has never been studied.
In the present study, we therefore evaluated respiratory center drive and pulmonary function in early-stage IPD patients. Specifically, we examined the pulmonary ventilation function, respiratory muscle strength index, and respiratory drive in early IPD patients and healthy controls.
Subjects/Materials and Methods
Human Subjects
In this study, a total of 43 IPD patients (19 male, 24 female; mean age 62.60 ± 6.60 years) and 41 healthy control subjects (21 male, 20 female; mean age 61.39 ± 5.87 years) were enrolled from May 2017 to September 2018 at the Department of Neurology at the General Hospital, Tianjin Medical University, Tianjin, China. The main clinical characteristics of the study population are summarized in Table 1. There were no significant differences in the age, height, weight, and BMI between IPD patients and healthy controls (Table 2). All IPD patients have a mean disease duration of 1.67 ± 1.14 years and received regular dopaminergic treatment with a mean total levodopa equivalent dose (LED) 313.18 ± 206.50 mg according to conversion factors among dopamine drugs recommended by the Movement Disorder Society (15). All subjects signed written informed consent before being recruited for the study. The study was approved and performed in accordance with the guidelines of the Ethics Committee of the General Hospital of Tianjin Medical University.
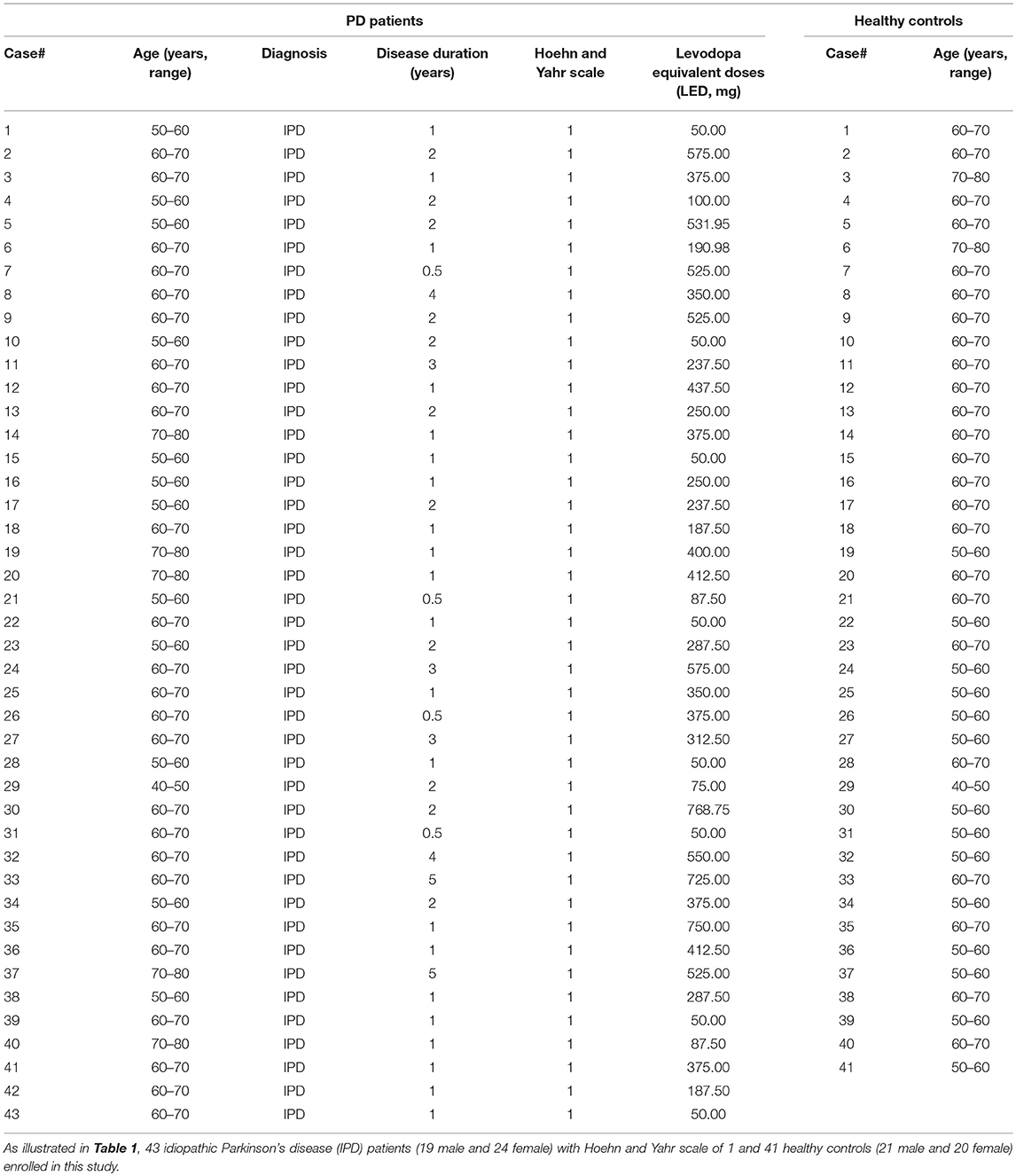
Table 1. Overview of the demographic and clinical characteristics of PD patients and healthy controls.
Inclusion Criteria of the PD Patients
All patients were diagnosed with IPD based on the clinical criteria of the United Kingdom PD Society Brain Bank (16). Motor status was evaluated using the Movement Disorders Society-Unified Parkinson's Disease Rating Scale (MDS-UPDRS). The Hoehn and Yahr stages of all patients were 1 (H&Y stage = 1), clinical signs and symptoms were unilateral involved only, usually with minimal or no functional impairment (17), and all these PD patients had no fluctuations in symptoms, such as on-off phenomena.
Exclusion Criteria of the PD Patients
IPD patients were excluded under the following conditions: (1) Suffering from any clinical neurological, respiratory, cardiovascular, or other systemic diseases verified by specialized physicians through clinical and appropriate instrumental diagnosis, such as cerebral infarction, cerebral hemorrhage, encephalitis, Myasthenia Gravis, COPD, asthma, emphysema, respiratory tract infection, anemia, coronary heart disease etc.; (2) Complaining of any heart and lung discomfort (e.g., dyspnea, palpitation, etc.); (3) History of smoking or lung, brain or cardiac surgery trauma; (4) Receiving any treatments (e.g., β-blocker, sedatives, hypnotics, antibiotic, NAIDS, etc.) that may influence pulmonary function; (5) Severe cognitive dysfunction or dementia according to the Mini-Mental State Examination (MMSE < 25) (18); (6) Other causes leading to the inability to tolerate the study or factors affecting lung function.
Requirements for Healthy Subjects
The healthy controls were age, sex, and BMI matched with all PD patients. They were in healthy condition at the time of the study and were screened for the same exclusion criteria as the PD patients.
Instruments and Procedures
All the patients and healthy controls underwent pulmonary function tests (PFT) according to the standards set by the American Thoracic Society (ATS) under the guidance of a respiratory physician. The apparatus used in this study included a spirometer and a respiratory actuator (MasterScreen, JAEGER, Germany). To ensure research consistency, fixed check time (8:00–12:00 a.m.), place (in a quiet environment, reducing the impact on subjects), and indoor temperature (24–28°C) were kept constant. Before the test, these early-stage PD patients were required to stop the associated anti-Parkinson's disease treatment at least 12 h to avoid the impact of drugs on testing. Beyond that, all patients underwent related neuropsychological assessments. Parameters related to PFT and various symptom scales are as follows:
Parameters Related to PFT
Predicted values were automatically generated by the PFT equipment according to the height, weight and age of the examinees before the test. Following examination, the percentages of the measured value from the test compared to the predicted value from the computer were automatically calculated. In this study, these percentages were considered as the PFT measurements to be recorded and processed further.
Ventilation Function Indicators
The percentages of the predicted values of forced vital capacity (FVC%), forced expiratory volume in 1s (FEV1%), forced expiratory volume in 1s/forced vital capacity (FEV1/FVC%), peak expiratory flow (PEF%), total lung capacity (TLC%), residual volume (RV%), carbon monoxide diffusion capacity (DLCOcSB%) were evaluated as indicators of ventilation function.
Respiratory Muscle Strength Index
The percentages of the predicted values of maximal inspiratory pressure (PImax%) and maximal expiratory pressure (PEmax%) were measured to determine the respiratory muscle strength.
Respiratory Drive Index
The percentage of the predicted value of the airway occlusion pressure (P0.1%) was examined to determine the respiratory drive.
Neuropsychological Examination
To assess neuropsychological status, all patients also underwent the Non-Motor Symptoms Questionnaire (NMSQ), REM sleep behavior Disorder Screening Questionnaire (RBDSQ), Beck Depression Inventory (BDI), and Mini Mental State Examination (MMSE).
Statistical Analysis
Statistical analyses were performed using SPSS 22.0 (IBM, USA). All the summary results were presented as the mean ± standard deviation (SD). All data was first checked for any potential outliers and tested for normality using the Shapiro-Wilk normality test. Data Significance was then assessed by student's unpaired t-test for data with normal distributions. A value of p < 0.05 was considered to be statistically significant.
Results
Unaltered Pulmonary Ventilation Function in Early-Stage IPD Patients
We first assessed the pulmonary ventilation function in early-stage IPD patients and healthy controls by measuring FVC (%), FEV1 (%), FVC/FEVF1 (%), PEF (%), RV (%), TLC (%), and DLCOcSB (%) (Figure 1). Consistent with the early clinical stage of these IPD patients, we found no significant changes in ventilation function in IPD patients (FVC: 105.51 ± 17.24%; FEV1: 102.67 ± 18.50%; FVC/FEVF1: 79.27 ± 9.46%; PEF: 103.19 ± 20.67%; RV: 94.26 ± 17.09%; TLC: 92.04 ± 9.67%; DLCOcSB: 83.57 ± 15.55%; n = 43) compared to healthy controls (FVC: 104.38 ± 15.90%, p = 0.76; FEV1: 102.12 ± 13.93%, p = 0.88; FVC/FEVF1: 79.71 ± 6.10%, p = 0.80; PEF: 109.01 ± 13.99%, p = 0.13; RV: 97.64 ± 16.65%, p = 0.36; TLC: 93.21 ± 11.26 %, p = 0.61; DLCOcSB: 88.63 ± 14.09%, p = 0.12; n = 41). In addition, there were no significant correlations between pulmonary ventilation function tests and neuropsychological assessments (p > 0.05, Table 3).
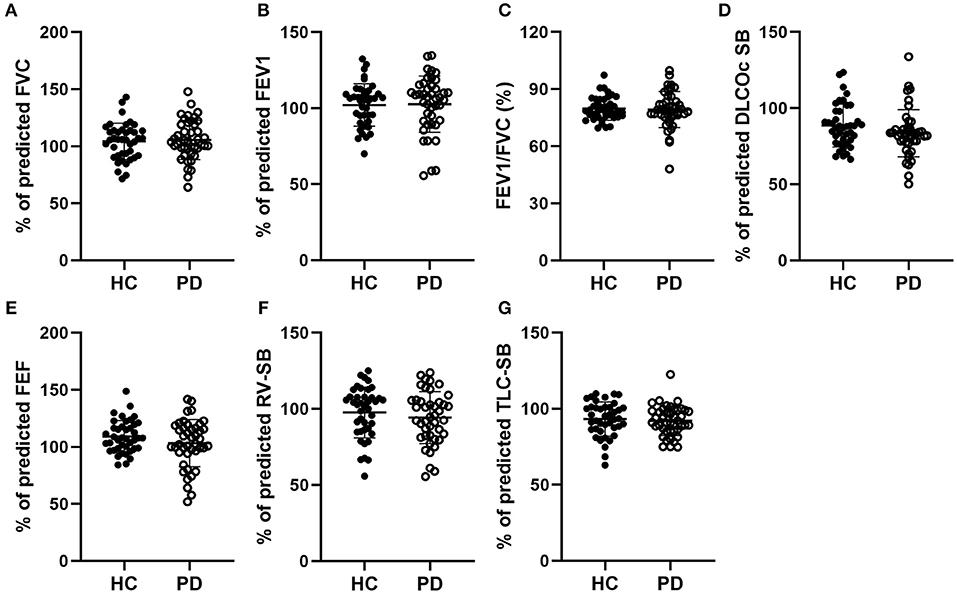
Figure 1. Comparisons of the pulmonary ventilation function between early-stage PD patients and age-matched healthy controls. (A–G) Percentage of predicted values of FVC (A), FEV1 (B), FEV1/FVC (C), DLCOc SB (D), FEF (E), RV-SB (F), and TLC-SB (G) showed no significant difference between early-stage PD patients (open circles, n = 43) and age-matched healthy controls (filled circles, n = 41, p > 0.05). Each symbol represents a single subject. Data are presented as mean ± SD.
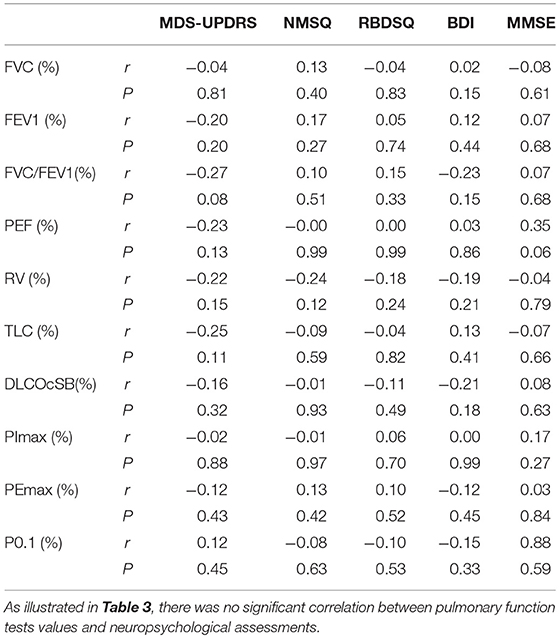
Table 3. Correlation analysis of pulmonary function tests (PFT) values and neuropsychological assessments.
Decreased Respiratory Muscle Strength in Early-Stage IPD Patients
We next evaluated respiratory muscle strength by assessing muscle strength index PImax and PEmax in both early-stage IPD patients and healthy controls. As shown Figures 2A,D, the early-stage IPD patients showed a significant reduction in both PImax (38.82 ± 16.87%, n = 43) and PEmax (68.13 ± 20.31%, n = 43) compared to healthy controls (PImax: 53.17 ± 16.00%, n = 41, p = 0.001; PEmax: 87.49 ± 32.46%, n = 41, p = 0.002). No significant correlations were found between PImax and neuropsychological assessments (MDS-UPDRS: p = 0.08, r = −0.02; NMSQ: p = 0.97, r = −0.01; RBDSQ: p = 0.70, r = 0.06; BDI: p = 0.99, r = 0.00; MMSE: p = 0.27, r = 0.17; Table 3), or between PEmax and neuropsychological assessments (MDS-UPDRS: p = 0.43, r = −0.12; NMSQ: p = 0.42, r = 0.13; RBDSQ: p = 0.52, r = 0.10; BDI: p = 0.45, r = −0.12; MMSE: p = 0.84, r = 0.03; Table 3). To further determine whether there is any sex-specific difference, we performed subgroup analysis by sex (Figures 2B,C,E,F). We found that both female and male early-stage IPD patients show a significant decrease in PImax (Female PD patients: 34.82 ± 16.39%, n = 24; Male PD patients: 43.87 ± 16.52%, n = 19, Figures 2B,C) and PEmax (Female PD patients: 71.60 ± 22.39%, n = 24; Male PD patients: 63.73 ± 16.91%, n = 19, Figures 2E,F) compared to female (PImax: 50.15 ± 14.73%, n = 20, p = 0.0024; PEmax: 98.16 ± 37.77%, n = 20, p = 0.006) and male (PImax: 56.06 ± 16.98%, n = 21, p = 0.027; PEmax: 77.33 ± 23.02%, n = 21, p = 0.042) healthy controls.
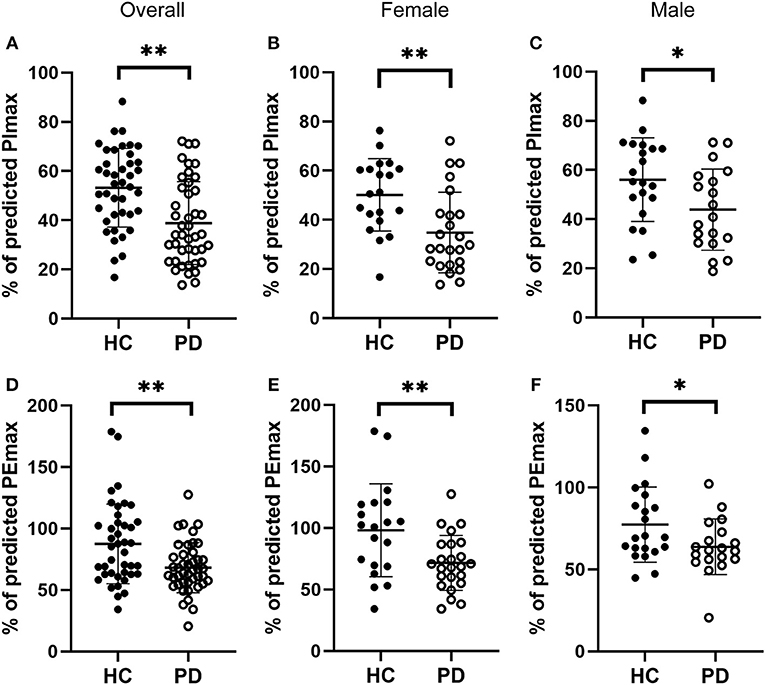
Figure 2. Comparisons of the respiratory muscle strength between early-stage PD patients and age-matched healthy controls. (A–C) Percentage of predicted values of maximal inspiratory pressure [PImax, (A)] was significantly lower in early-stage PD patients (open circles, n = 43) and age-matched healthy controls (filled circles, n = 41, **p < 0.001). Note that both female [(B), n = 24] and male [(C), n = 19] early-stage PD patients (open circles) showed significantly lower values of PImax compared to the corresponding healthy controls (filled circles; female n = 20, male n = 21, *p < 0.05, **p < 0.01). (D–F) Percentage of predicted values of maximal expiratory pressure [PEmax, (D)] was significantly lower in early-stage PD patients (open circles, n = 43) and age-matched healthy controls (filled circles, n = 41, **p < 0.001). Note that both female [(E), n = 24) and male [(F), n = 19] early-stage PD patients (open circles) showed significantly lower values of PEmax compared to the corresponding healthy controls (filled circles; female n = 20, male n = 21, *p < 0.05, **p < 0.01). Each symbol represents a single subject. Data are presented as mean ± SD.
Increased Respiratory Drive in Early-Stage IPD Patients
Respiratory center drive, P0.1, is associated with the level of muscular inspiratory activity. As we found significant decreases in respiratory muscle strength, we next aimed to assess respiratory center drive in early-stage IPD patients as well as healthy controls (Figure 3). Indeed, we found that early-stage IPD patients showed significant increases in respiratory center drive P0.1 (156.78 ± 63.24%, n = 43) compared to age-matched controls (82.40 ± 13.14%, n = 41, p < 0.001, Figure 3A). Importantly, both female and male early-stage IPD patients show a significant increase in P0.1 (Female PD patients: 181.1 ± 73.00%, n = 24; Male PD patients: 126.1 ± 27.31%, n = 19, Figures 3B,C) compared to female (85.70 ± 10.78%, n = 20, p = 0.001) and male (79.26 ± 14.61%, n = 21, p = 0.001) healthy controls. It is worth mentioning that respiratory center drive P0.1 values of most male and female IPD patients were indeed separated from control values with minimal overlap. No significant correlations were found between P0.1 and neuropsychological assessments (MDS-UPDRS: p = 0.45, r = 0.12; NMSQ: p = 0.63, r = −0.08; RBDSQ: p = 0.53, r = −0.10; BDI: p = 0.33, r = −0.15; MMSE: p = 0.59, r = 0.88; Table 3).
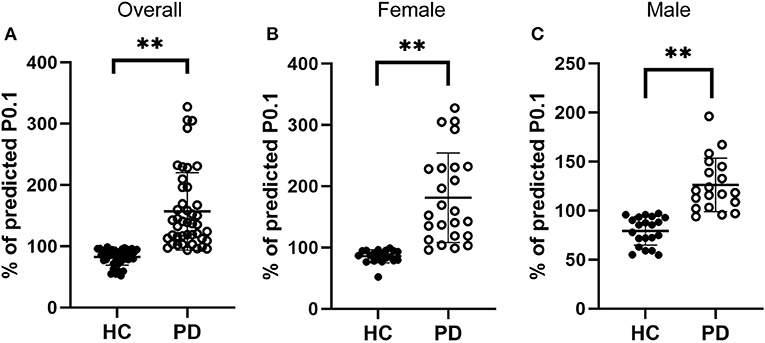
Figure 3. Comparisons of the respiratory drive between early-stage PD patients and age-matched healthy controls. (A) Percentage of predicted values of respiratory center drive (P0.1), was significantly higher in early-stage PD patients (open circles, n = 43) and age-matched healthy controls (filled circles, n = 41, **p < 0.001). (B–C) Both female [(B), n = 24] and male [(C), n = 19] early-stage PD patients (open circles) showed significantly larger values of PImax compared to the corresponding female [(B), n = 20] and male [(C), n = 21] healthy controls (filled circles, **p < 0.01). Each symbol represents a single subject. Data are presented as mean ± SD.
Discussion
The present study provides the first evidence of abnormal pulmonary function in the very early stage of IPD. As documented here, patients with early IPD had significantly lower respiratory muscle strength (PImax%, PEmax%) and higher central respiratory drive (P0.1%) than those in age-matched healthy controls. Importantly, the values of measured respiratory center drive P0.1 showed a remarkable separation between the early-stage IPD patients and healthy controls, indicating its potential utility for diagnosis of the early-stage IPD patients.
Abnormalities of peak expiratory and inspiratory flows (PEF, PIF) were found in patients with relatively severe PD and without clinical signs or symptoms of respiratory problem. These changes can be explained by “muscle weakness” and hypokinesia, two symptoms intrinsic to PD (19). What's more, levodopa improved significant variations in PEF by decreasing tremor and muscle rigidity, increasing coordination of muscles, and facilitating movement (20). In previous studies, upper airway dysfunction and a restrictive pattern are the main spirometric findings in PD. The main reasons might be a poor coordination or rigidity of respiratory muscles, which could limit forced respiratory movements (21). Unlike many previous studies, our data did not show any respiratory abnormalities including ventilation or diffusion dysfunction. This may be because the subjects in our study were very early stage patients (H&Y scale of 1) rather than more severe patients (H&Y scale of 2 or higher). Respiratory muscle strength, assessed by measuring the maximal inspiratory and expiratory mouth pressures (PImax and PEmax) was decreased in IPD patients. Furthermore, all these parameters of respiratory muscle performance tended to increase in the “on” period rather than the “off” period, although this increase did not reach statistical significance (22). This was due to poor posture restricting chest and abdominal movements, rigidity resulting in poor chest wall compliance and disrupted respiratory muscle coordination (23). Consistent with previous studies, our findings that the values of PImax and PEmax in early-stage IPD were significantly lower than the healthy controls suggest that inspiratory muscle strength appears to be impaired in IPD patients at very early stage (24). As previously thought, PD involves dysfunction in both movement and muscle tone in the trunk and limbs related to abnormal thoracoabdominal movements as well as incoordination of respiratory muscle (25).
Respiratory center drive, or P0.1, is associated with the level of muscular inspiratory activity. It involves the nerve impulse that the respiratory center gives out to stimulate the inspiratory muscle to contract when we inhale. It is mainly affected by sensitivity of chemoreceptors in vivo, partial pressure of arterial oxygen CO2, resistance to inhalation, and abnormal function of inspiratory muscles (26). The output of the respiratory center can be estimated by many parameters, such as ventilation, inspiratory muscle power or EMG of the diaphragm. However, P0.1, the airway occlusion pressure (the negative airway pressure generated during the first 100 ms of an occluded inspiration) represents the most important index of the output of the respiratory centers (27). In many studies, P0.1 is a useful parameter in setting the level of pressure support ventilation in mechanical ventilation (28). Additionally, P0.1 can be used as a good indicator of postextubation respiratory distress after extubation (29). The most striking finding of our study was significant changes in respiratory center drive in patients with early stage IPD. The percentage of the predicted values of P0.1 (P0.1%) in IPD group was significantly higher than in the control group (p < 0.001). In our study, factors such as respiratory diseases or smoking which can influence P0.1 were excluded, suggesting that the abnormal respiratory drive was, therefore, mainly related to IPD itself. Based on relevant literature, there are two possible mechanisms at play. First is abnormal respiratory muscle strength of PD patients (peripheral mechanisms). Second is abnormalities in the respiratory center itself (central mechanisms). Abnormalities of the chest wall or its musculature can change lung volumes without necessarily altering expiratory flow (30). PD with a restrictive pattern of pulmonary dysfunction could probably be explained by abnormally low chest wall compliance secondary to chest wall rigidity (31). As described in the diagnostic criteria, as PD patients manifest their core symptoms, such as rigidity and bradykinesia, their movements become slow, clumsy, inflexible and uncoordinated, which impairs the tone, contractility, and coordination of thoracic musculature. As a result, the respiratory mechanics and pulmonary function are affected. The decrease of respiratory muscle force may cause an increase in compensation of central respiratory driving force to maintain adequate physiological ventilation. This central self-regulation mechanism is common in respiratory diseases such as COPD and asthma (32). In our study, the H&Y scale of IPD patients is 1 (the course of illness is extremely short), so the impairment of common lung ventilation (restrictive, obstructive, and mixed), is not evident or within the scope of compensation. So, P0.1 is a useful and sensitive measurement in early-stage IPD (28). Another mechanism involved is the problem with the respiratory center itself. Based on the Braak hypothesis, which suggests that alpha-synuclein aggregation starts from olfactory bulb and dorsal vagus nucleus, and develops gradually up and forward along the brain stem, there is early brainstem involvement in PD patients (33). Some researchers suggest that there is a depletion of the chemosensitive glutamatergic neurons in the dorsolateral pons and the ventrolateral medulla in both PD and MSA patients, which may trigger the impairment of respiratory muscle control (34). In a recent study by Lee et al. involvement of the central autonomic network and gray matter loss may underlie the respiratory dysfunction in PD patients (35). In addition, autonomic nerve dysfunction, such as vagus nerve damage, leads to increased upper airway muscle tone, resulting in uncoordinated local movements and weak muscular contraction (36, 37). There is currently little evidence of brainstem-mediated respiratory dysfunction, however it seems reasonable that as PD progresses along with further loss of dopaminergic cells in the midbrain, basal ganglia and medulla, respiratory function and ventilation will deteriorate (38).
The early diagnosis of PD will greatly help optimize therapeutic strategies, especially in cases with very mild or a-typical motor and non-motor symptoms. That being said, we have found significant increases in respiratory center drive (P0.1) and decreases in respiratory muscle strength PImax and PEmax in early-stage IPD patients. Importantly, measured P0.1 values in both female and male early-stage IPD patients were remarkably separated from those in healthy controls, while measured PImax and PEmax values showed moderate overlap between early-stage IPD patients and healthy controls. Therefore, our data strongly supports P0.1 as a safe, non-invasive and convenient measurement for early-stage IPD in clinical practice.
Data Availability
All datasets generated for this study are included in the manuscript and/or the supplementary files.
Ethics Statement
This study was carried out in accordance with the recommendations of the Ethics Committee of the General Hospital of Tianjin Medical University with written informed consent from all subjects. All subjects gave written informed consent in accordance with the Declaration of Helsinki. The protocol was approved by the Ethics Committee of the General Hospital of Tianjin Medical University.
Author Contributions
WZ, LZ, HS, and XZ: designed experiments. WZ, LZ, NZ, EH, QL, TW, CM, BL, CL, YD, JZ, and XL: carried out data collections. WZ, LZ, AR, HS, and XZ: analyzed experimental results and wrote the manuscript.
Funding
This study was supported in part by Tianjin Municipal Science and Technology Commission Foundation Grants (No: 15JCYBJC50000) and The Science and Technology Development Fund of Tianjin Education Commission for Higher Education (No: 2017ZD10) and fundamental research funds for the central universities in Peking Union Medical College (No: 3332018181) and Ministry of Science and Technology of China (Nos: 2016YFC1306500, 2016YFC1306504). HS holds a Canada Research chair in developmental neuroscience.
Conflict of Interest Statement
The authors declare that the research was conducted in the absence of any commercial or financial relationships that could be construed as a potential conflict of interest.
Acknowledgments
We thank our patients and healthy controls for their participation in this study. We thank Wei Liu, Peng Zhao, Yu Wang, Yanan Sun, Mengya Xing, and Junfeng Yang for their assistance with data collection. We thank Dr. Q. Liu for helpful discussion and K. Wood for editorial assistance.
References
1. de Lau LM, Breteler MM. Epidemiology of Parkinson's disease. Lancet Neurol. (2006) 5:525–35. doi: 10.1016/S1474-4422(06)70471-9
2. Johnson ME, Stecher B, Labrie V, Brundin L, Brundin P. Triggers, facilitators, and aggravators: redefining Parkinson's disease pathogenesis. Trends Neurosci. (2019) 42:4–13. doi: 10.1016/j.tins.2018.09.007
3. Kalia LV, Lang AE. Parkinson's disease. Lancet. (2015) 386:896–912. doi: 10.1016/S0140-6736(14)61393-3
4. Lilker ES, Woolf CR. Pulmonary function in Parkinson's syndrome: the effect of thalamotomy. Can Med Assoc J. (1968) 99:752–7.
5. Sabate M, Rodriguez M, Mendez E, Enriquez E, Gonzalez I. Obstructive and restrictive pulmonary dysfunction increases disability in Parkinson disease. Arch Phys Med Rehabil. (1996) 77:29–34. doi: 10.1016/S0003-9993(96)90216-6
7. Monteiro L, Souza-Machado A, Valderramas S, Melo A. The effect of levodopa on pulmonary function in Parkinson's disease: a systematic review and meta-analysis. Clin Ther. (2012) 34:1049–55. doi: 10.1016/j.clinthera.2012.03.001
8. De Pandis MF, Starace A, Stefanelli F, Marruzzo P, Meoli I, De Simone G, et al. Modification of respiratory function parameters in patients with severe Parkinson's disease. Neurol Sci. (2002) 23(suppl. 2):S69–70. doi: 10.1007/s100720200074
9. Pal PK, Sathyaprabha TN, Tuhina P, Thennarasu K. Pattern of subclinical pulmonary dysfunctions in Parkinson's disease and the effect of levodopa. Mov Disord. (2007) 22:420–4. doi: 10.1002/mds.21330
10. LeWitt PA, Pahwa R, Sedkov A, Corbin A, Batycky R, Murck H. Pulmonary safety and tolerability of inhaled levodopa (CVT-301) administered to patients with Parkinson's disease. J Aerosol Med Pulm Drug Deliv. (2018) 31:155–61. doi: 10.1089/jamp.2016.1354
11. Colgrove Y, Sharma N, Robbins K, Wagner K. A randomized controlled pilot study of the therapeutic effects of yoga in people with Parkinson′s disease. Int J Yoga. (2015) 8:74–9. doi: 10.4103/0973-6131.146070
12. Burini D, Farabollini B, Iacucci S, Rimatori C. A randomised controlled cross-over trial of aerobic training versus Qigong in advanced Parkinson's disease. Eura Medicophys. (2006) 42:231–8.
13. Telias I, Damiani F, Brochard L. The airway occlusion pressure (P0. 1) to monitor respiratory drive during mechanical ventilation: increasing awareness of a not-so-new problem. Intens Care Med. (2018) 44:1532–5. doi: 10.1007/s00134-018-5045-8
14. Fernandez R, Raurich JM, Mut T, Blanco J, Santos A, Villagra A. Extubation failure: diagnostic value of occlusion pressure (P0. 1) and P0. 1-derived parameters. Intens Care Med. (2004) 30:234–40. doi: 10.1007/s00134-003-2070-y
15. Tomlinson CL, Stowe R, Patel S, Rick C, Gray R, Clarke CE. Systematic review of levodopa dose equivalency reporting in Parkinson's disease. Mov Disord. (2010) 25:2649–53. doi: 10.1002/mds.23429
16. Hughes AJ, Daniel SE, Kilford L, Lees AJ. Accuracy of clinical diagnosis of idiopathic Parkinson's disease: a clinico-pathological study of 100 cases. J Neurol Neurosurg Psychiatry. (1992) 55:181–4. doi: 10.1136/jnnp.55.3.181
17. Hoehn MM, Yahr MD. Parkinsonism: onset, progression and mortality. Neurology. (1967) 17:427–42. doi: 10.1212/WNL.17.5.427
18. Folstein MF, Folstein SE, McHugh PR. “Mini-mental state”. A practical method for grading the cognitive state of patients for the clinician. J Psychiatr Res. (1975) 12:189–98. doi: 10.1016/0022-3956(75)90026-6
19. Hovestadt A, Bogaard JM, Meerwaldt JD, van der Meché FG, Stigt J. Pulmonary function in Parkinson's disease. J Neurol Neurosurg Psychiatry. (1989) 52:329–33. doi: 10.1136/jnnp.52.3.329
20. Herer B, Arnulf I, Housset B. Effects of levodopa on pulmonary function in Parkinson's disease. Chest. (2001) 119:387–93. doi: 10.1378/chest.119.2.387
21. Izquierdo-Alonso JL, Jimenez-Jimenez FJ, Cabrera-Valdivia F, Mansilla-Lesmes M. Airway dysfunction in patients with Parkinson's disease. Lung. (1994) 172:47–55. doi: 10.1007/BF00186168
22. Weiner P, Inzelberg R, Davidovich A, Nisipeanu P, Magadle R, Berar-Yanay N, et al. Respiratory muscle performance and the perception of dyspnea in Parkinson's disease. Can J Neurol Sci. (2002) 29:68–72. doi: 10.1017/S031716710000175X
23. Sawan T, Harris ML, Kobylecki C, Baijens L, van Hooren M, Michou E. Lung function testing on and off dopaminergic medication in Parkinson's disease patients with and without dysphagia. Mov Disord Clin Pract. (2016) 3:146–50. doi: 10.1002/mdc3.12251
24. Baille G, Perez T, Devos D, Deken V, Defebvre L, Moreau C. Early occurrence of inspiratory muscle weakness in Parkinson's disease. PLoS ONE. (2018) 13:e190400. doi: 10.1371/journal.pone.0190400
25. Tamaki A, Matsuo Y, Yanagihara T, Abe K. Influence of thoracoabdominal movement on pulmonary function in patients with Parkinson's disease: comparison with healthy subjects. Neurorehabil Neural Repair. (2000) 14:43–7. doi: 10.1177/154596830001400105
26. Grant JL, Lucier E, Mahnke ML. Estimation of respiratory drive with carbon dioxide and helium. Ann Intern Med. (1971) 74:62–6. doi: 10.7326/0003-4819-74-1-62
27. Whitelaw WA, Derenne JP, Milic-Emili J. Occlusion pressure as a measure of respiratory center output in conscious man. Respir Physiol. (1975) 23:181–99. doi: 10.1016/0034-5687(75)90059-6
28. Alberti A, Gallo F, Fongaro A, Valenti S, Rossi A. P0. 1 is a useful parameter in setting the level of pressure support ventilation. Intensive Care Med. (1995) 21:547–53. doi: 10.1007/BF01700158
29. Hilbert G, Gruson D, Portel L, Vargas F, Gbikpi-Benissan G, Cardinaud JP. Airway occlusion pressure at 0. 1 s (P0. 1) after extubation: an early indicator of postextubation hypercapnic respiratory insufficiency. Intensive Care Med. (1998) 24:1277–82. doi: 10.1007/s001340050762
30. Obenour WH, Stevens PM, Cohen AA, McCutchen JJ. The causes of abnormal pulmonary function in Parkinson's disease. Am Rev Respir Dis. (1972) 105:382–7.
31. Sathyaprabha TN, Kapavarapu PK, Pall PK, Thennarasu K, Raju TR. Pulmonary functions in Parkinson's disease. Indian J Chest Dis Allied Sci. (2005) 47:251–7.
32. Franczuk M, Radwan L, Maszczyk Z, Kazimierczak A, Kus J, Koziorowski A, et al. Pattern of breathing and obstruction pressure in patients with airway obstruction after inhalation of bronchodilators. Pneumonol Alergol Pol. (1997) 65:457–64.
33. Braak H, Del TK, Rub U, de Vos RA, Jansen SE, Braak E. Staging of brain pathology related to sporadic Parkinson's disease. Neurobiol Aging. (2003) 24:197–211. doi: 10.1016/S0197-4580(02)00065-9
34. Wang Y, Shao W, Gao L, Lu J, Gu H, Sun L, et al. Abnormal pulmonary function and respiratory muscle strength findings in chinese patients with Parkinson's disease and multiple system atrophy–comparison with normal elderly. PLoS ONE. (2014) 9:e116123. doi: 10.1371/journal.pone.0116123
35. Lee S, Chen M, Chiang P, Chen H, Chou K, Chen Y, et al. Reduced gray matter volume and respiratory dysfunction in Parkinson's disease: a voxel-based morphometry study. BMC Neurol. (2018) 18:73. doi: 10.1186/s12883-018-1074-8
36. Vincken WG, Gauthier SG, Dollfuss RE, Hanson RE, Darauay CM, Cosio MG. Involvement of upper-airway muscles in extrapyramidal disorders. A cause of airflow limitation. N Engl J Med. (1984) 311:438–42. doi: 10.1056/NEJM198408163110704
37. Tsai CC, Wu MN, Liou LM, Chang YP. Levodopa reverse stridor and prevent subsequent endotracheal intubation in Parkinson disease patients with bilateral vocal cord palsy: a case report. Medicine. (2016) 95:e5559. doi: 10.1097/MD.0000000000005559
Keywords: Parkinson's disease, respiratory center drive, respiratory muscle strength, P0.1, biomarker
Citation: Zhang W, Zhang L, Zhou N, Huang E, Li Q, Wang T, Ma C, Li B, Li C, Du Y, Zhang J, Lei X, Ross A, Sun H and Zhu X (2019) Dysregulation of Respiratory Center Drive (P0.1) and Muscle Strength in Patients With Early Stage Idiopathic Parkinson's Disease. Front. Neurol. 10:724. doi: 10.3389/fneur.2019.00724
Received: 08 March 2019; Accepted: 18 June 2019;
Published: 03 July 2019.
Edited by:
Davide Martino, King's College London, United KingdomReviewed by:
Alessandro Tessitore, University of Campania Luigi Vanvitelli, ItalyVeronica Andrea Bruno, University of Calgary, Canada
Copyright © 2019 Zhang, Zhang, Zhou, Huang, Li, Wang, Ma, Li, Li, Du, Zhang, Lei, Ross, Sun and Zhu. This is an open-access article distributed under the terms of the Creative Commons Attribution License (CC BY). The use, distribution or reproduction in other forums is permitted, provided the original author(s) and the copyright owner(s) are credited and that the original publication in this journal is cited, in accordance with accepted academic practice. No use, distribution or reproduction is permitted which does not comply with these terms.
*Correspondence: Hongyu Sun, hongyu.sun@carleton.ca; Xiaodong Zhu, zxd3516@tmu.edu.cn
†These authors have contributed equally to this work