- 1Department of Neurology, School of Medicine, College of Medicine, Taipei Medical University, Taipei, Taiwan
- 2Department of Neurology, Stroke Center, Shuang Ho Hospital, Taipei Medical University, New Taipei, Taiwan
- 3Department of Neurology, Faculty of Medicine, National Yang-Ming University School of Medicine, Taipei, Taiwan
- 4Department of Neurology, Neurological Institute, Taipei Veterans General Hospital, Taipei, Taiwan
- 5School of Public Health, College of Public Health, Taipei Medical University, Taipei, Taiwan
- 6Graduate Institute of Neural Regenerative Medicine, College of Medical Science and Technology, Taipei Medical University, Taipei, Taiwan
- 7Taipei Neuroscience Institute, Taipei Medical University, Taipei, Taiwan
Objectives: Post-stroke cognitive impairment (PSCI) is a common disease that may occur within 3 months after a stroke or even later. However, the mechanism of PSCI development is unclear. The present study investigated whether the levels of plasma amyloid beta-42 (Aβ42) and tau are associated with the onset of PSCI.
Methods: Fifty-five patients admitted within 7 days of acute ischemic stroke were enrolled and followed up for 1 year. Montreal Cognitive Assessment (MoCA) was administered at 3 months and 1 year, and plasma Aβ42 and tau levels were determined using an ultrasensitive immunoassay (immunomagnetic reduction) within 7 days of the stroke event and 3 months later.
Results: In this study, 13 of 55 patients developed PSCI (MoCA score <23) at 3 months. Seven patients with PSCI at 3 months recovered to a cognitively normal state at 1 year, whereas seven cognitively normal patients developed PSCI at 1 year. The patients with PSCI at 1 year had a higher incidence of cognitive function deterioration between 3 months and 1 year compared with those without PSCI at 1 year. Plasma Aβ42 and tau levels at 3 months were lower in the patients with PSCI at 1 year than in those without PSCI (Aβ42: 15.1 vs. 17.2 pg/mL, P = 0.013; tau: 16.7 vs. 19.9 pg/mL, P = 0.018). Low education levels and pre-existing white matter disease were the most significant predictors of PSCI at 3 months, and poor cognitive performance at 3 months and low plasma Aβ42 and tau levels at 3 months were the most significant predictors of PSCI at 1 year.
Conclusion: The pathogenesis of PSCI is complex and changes with time. Ischemia-induced Aβ42/tau pathology might be involved in PSCI development.
Introduction
Post-stroke cognitive impairment (PSCI) can occur immediately after a stroke or after a certain period. PSCI occurrence after a certain period is common, but it is often overlooked. Post-stroke dementia occurs in 7% of patients 1 year after a stroke (1); however, its incidence could be as high as 52% at 6 months after a stroke if mild PSCI is included in the diagnosis (2). PSCI is associated with impairment of activities of daily living, poor quality of life, and increased burden of care (3, 4). In addition, compelling evidence indicates that accelerated, continuous cognitive decline may occur following acute stroke events, even without clinically recurrent stroke occurrence (5). Consequently, in addition to the standard healthcare for stroke management, it is essential to detect and prevent PSCI. Age, hypertension, diabetes, and low education levels have been reported to be associated with PSCI (6). The roles of neuroinflammation and amyloid-related pathology in PSCI development have also been highlighted (7). However, the pathogenesis of late-onset PSCI remains unclear. In addition, precise biomarkers that could facilitate the prediction of PSCI following acute stroke events are lacking.
Cerebral ischemia triggers amyloid beta-42 (Aβ42) deposition, alteration of tau phosphorylation, and neuroinflammation (7–9), which are also the core pathologies of Alzheimer's disease (AD). Therefore, in addition to damaging the brain directly, ischemia may induce neurodegenerative processes that lead to PSCI development. The levels of Aβ42 and tau in the cerebrospinal fluid (CSF) correlate well with the brain Aβ42 burden assessed using position emission tomography (PET) (10, 11). The Aβ42 burden on PET images is the gold standard maker for AD diagnosis; however, it is not widely applied in clinical practice. The Aβ42 level in the plasma correlates with the Aβ42 level in the CSF, and the Aβ42 levels in the plasma are lower in patients with AD than in patients with mild cognitive impairment or controls (12, 13). In addition, the plasma Aβ42 levels and Aβ42/Aβ40 ratios could reveal AD pathological change in PET among cognitively normal individuals with subjective cognitive decline (12). In addition, the levels of plasma tau are higher in patients with AD than in controls (14, 15). The Aβ42, Aβ40, and tau levels in peripheral blood can be quantified using ultrasensitive immunoassays such as single-molecule assay (SIMOA) or immunomagnetic reduction (IMR) assay. Aβ42 and tau plasma measurements are more practical than PET and CSF assessments in clinical settings. Therefore, plasma Aβ42 and tau are potential biomarkers of neurodegenerative diseases, including PSCI. The association between plasma Aβ42 levels and cognitive function was investigated in a cross-sectional study of stroke (16). A longitudinal study would clarify the temporal relationship between cognitive function deterioration and the plasma biomarkers of Aβ42 and tau.
The roles of plasma Aβ42 and tau in PSCI development are still unclear. We hypothesized that the levels of plasma Aβ42 and tau may predict the onset of PSCI. In the present study, we investigated whether plasma Aβ42 and tau levels at 3 months after an ischemic stroke are associated with the onset of PSCI at 3 months and 1 year.
Methods
Participants
The Institutional Review Board of Taipei Medical University approved the present study. Adult patients (≥20 years old) who were admitted to Taipei Medical University-Shuang Ho Hospital within 7 days of acute ischemic stroke were consecutively screened for eligibility in the present study. The exclusion criteria were as follows: (1) Patients with known cognitive impairment or a neurodegenerative disease that impaired regular daily activities before a stroke, (2) patients with large infarcts (≥1/3 middle cerebral artery territory) that caused immediate consciousness or cognitive impairment after a stroke, (3) patients with strategic infarcts involving the paramedian thalamus, hippocampus, or medial frontal cortex with a high risk of cognitive impairment, and (4) patients with severe language or physical disability that impeded neuropsychological testing. Each patient was assessed within 7 days of a stroke at admission and was followed up at 3 months and 1 year at the outpatient clinic. Sixty patients were enrolled into the study, and written informed consent was obtained from all patients or their legal guardians.
Clinical Characteristics
The patients' stroke severity was assessed at admission, 3 months, and 1 year using the National Institute of Health Stroke Scale (NIHSS), and the patients' cognitive functions were assessed using the Montreal Cognitive Assessment (MoCA) screening instrument (17) at 3 months and 1 year. A trained research assistant blinded to the patients' plasma biomarker data conducted assessments. The MoCA score was adjusted based on the patients' education levels. The patients who could not complete the MoCA test at 3 months due to physical disability (e.g., dominant hand weakness and unable to complete the drawing tasks) were excluded at 3 months (n = 5). Data of 55 patients were included in the final analysis. The patients with a MoCA score of <23 were considered as having cognitive impairment (18).
Brain magnetic resonance images were obtained once at admission, including T1- and T2-weighted images, T2 fluid-attenuated inversion recovery (T2 FLAIR) images, diffusion-weighted images (DWI), apparent diffusion coefficient maps, and time-of-flight magnetic resonance angiograms. Carotid Doppler ultrasonographs and electric cardiograms were also obtained once during admission. The lesion volume on DWI was calculated manually, pre-existing white matter hyperintensities in T2 FLAIR images were assessed using the Fazekas scale (19), and the etiologic subtype of stroke was classified based on the TOAST study (20) by an experienced neurologist blinded to the patients' outcomes.
Plasma Amyloid Beta and Tau Protein Analysis
The patients provided a 10-mL non-fasting venous blood sample within 7 days of the stroke event and 3 months later. The blood samples were centrifuged at 1,500 × g for 15 min and stored at −80°C. The blood samples were sent to MagQu Co. Ltd. (New Taipei City, Taiwan) for IMR assays, with blinding applied for the patients' identity and clinical information.
The technical details of IMR assays are described in previous studies (21, 22). In brief, IMR reagents consisting of Fe3O4 nanoparticles coated with Aβ42, Aβ40, or tau antibodies (MF-AB2-0060, MF-AB0-0060, and MF-TAU-0060 respectively, MagQu) were used to capture the antigens in the plasma. The antigen-bound nanoparticles became larger, clustered, and their movements were less responsive to an external magnetic field than the unbound ones. Reduction in magnetic susceptibility was measured using a magnetosusceptometer (XacPro-S, MagQu). The percentage reduction in magnetic susceptibility was referred to as IMR. The plasma antigen levels were determined using a logistic function of the concentration-dependent IMR signal. Aβ40 levels were measured only at 3 months after the stroke event. Each reported result of Aβ or tau in a patient was the average of duplicate measurements that had a coefficient of variation <15%.
Statistical Analysis
Data normality was determined using the Shapiro–Wilk test. Normally distributed data are expressed as means ± standard deviations, whereas non-normally distributed data are expressed as medians with inter-quartile ranges (IQRs). Demographic and laboratory data were compared between the patients with PSCI (MoCA score <23) and those without PSCI (MoCA score ≥ 23) using the t-test, Mann–Whitney U-test, or χ2 test, as applicable. The MoCA score and laboratory data at different time points were compared using the Wilcoxon signed-rank test. Correlations between variables were verified using the Spearman rank correlation coefficient. Univariate logistic regression was used to estimate the odds ratios of PSCI onset based on clinical characteristics and laboratory data. All variables were tested for multicollinearity (variance inflation factor ≥ 10). Multivariate logistic regression using automatic forward variable selection (enter if P < 0.10) was conducted to build a model including significant variables associated with PSCI. A P-value of < 0.05 was considered statistically significant. Statistical data were analyzed using MedCalc v18 (MedCalc Software bvba, Ostend, Belgium).
Results
The clinical characteristics of the patients with PSCI at 3 months (n = 13) and without PSCI at 3 months (n = 42) are summarized in Table 1. Most patients had mild stroke severity (median NIHSS score = 4 and median lesion volume = 0.5 cm3 in each group at admission) and small-vessel disease, which was consistent with our study inclusion and exclusion criteria. Education levels in the patients with PSCI at 3 months were significantly lower than those in the patients without PSCI at 3 months, whereas the scores of the Fazekas scale in the patients with PSCI at 3 months were significantly higher than those in the patients without PSCI at 3 months. Age, sex, stroke lesion side, stroke etiologic subtype, vascular comorbidities, NIHSS score within 7 days, NIHSS score at 3 months, hemoglobin A1c (HbA1c), lesion volume on DWI, plasma Aβ42, and tau levels within 7 days, and Aβ42, Aβ40, and tau levels at 3 months were not different between the patients with and without PSCI at 3 months.
The results of univariate and multivariate logistic regression analyses of variables predicting PSCI at 3 months are summarized in Table 2. In univariate analysis, the education level and Fazekas scale score were significant predictors, whereas age, diabetes mellitus, and lesion volume on DWI were predictors with borderline significance (P = 0.080, 0.077, and 0.059, respectively). The NIHSS score within 7 days or at 3 months, hypertension, hyperlipidemia, HbA1c, stroke lesion side, plasma Aβ42, and tau levels within 7 days, and plasma Aβ42, Aβ40, and tau levels at 3 months were not predictors of PSCI at 3 months. In multivariate analysis, only the education level and Fazekas scale score were included in the automatic forward variable selection model with an acceptable model fit (Nagelkerke R2 = 0.37, Hosmer–Lemeshow test P = 0.17). Therefore, the education level and pre-existing white matter disease (Fazekas scale score) were the most significant predictors of PSCI at 3 months. The receiver operating characteristic (ROC) curves of the education level, Fazekas scale score, and multivariate model for predicting PSCI at 3 months are displayed in Figure 1.
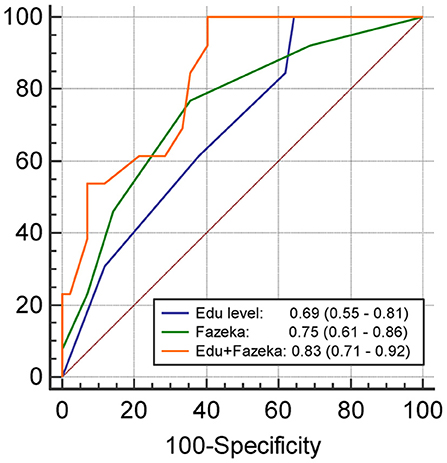
Figure 1. The receiver operating characteristics curve and the area under curves (95% confidence interval) of the variables predicting PSCI at 3 months. Edu, Education level (year); Fazeka, The score of Fazekas scale.
During follow-up, the median MoCA scores of all participants were not different between 3 months and 1 year (25 vs. 25, P = 0.314). However, 7 of 42 patients without PSCI at 3 months exhibited cognitive function deterioration, which progressed to PSCI at 1 year. Conversely, 7 of 13 patients with PSCI at 3 months exhibited improvements in cognitive function, and PSCI was reversed at 1 year. A comparison of MoCA scores (positive values represented improvements, and negative values represented deterioration) between the patients with and without PSCI at 1 year is presented in Table 3. The incidence of cognitive function deterioration (MoCA score decreased by 2 points or more) was significantly higher in the patients with PSCI at 1 year than in the patients without PSCI at 1 year. In addition, MoCA scores at 3 months were significantly lower in the patients with PSCI at 1 year than in the patients without PSCI at 1 year. Figure 2 presents a flowchart of patient enrollment and changes in cognitive function.
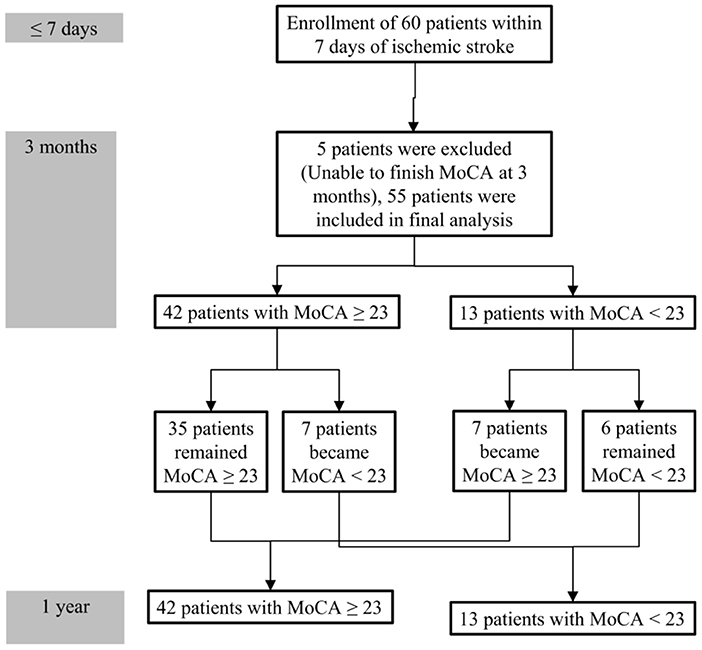
Figure 2. Flowchart of patient enrollment and cognitive function changes during the 1-year follow-up.
The clinical characteristics of the patients with PSCI at 1 year (n = 13) and without PSCI at 1 year (n = 42) are summarized in Table 4. Plasma Aβ42 and tau levels at 3 months as well as the Aβ42/40 ratio at 3 months in the patients with PSCI at 1 year were significantly lower than those in the patients without PSCI at 1 year. Age, sex, education level, stroke lesion side, stroke etiologic subtype, vascular comorbidities, HbA1c, NIHSS score within 7 days, NIHSS score at 3 months, NIHSS score at 1 year, lesion volume on DWI, and plasma Aβ42 and tau levels within 7 days were not different between the patients with and without PSCI at 1 year.
The results of univariate and multivariate logistic regression analyses of variables predicting PSCI at 1 year are presented in Table 5. In univariate analysis, the MoCA score at 3 months, plasma Aβ42 and tau levels at 3 months, and Aβ42/40 ratio at 3 months were significant predictors, whereas the education level was a predictor with borderline significance (P = 0.092). Age, sex, vascular comorbidities, HbA1c, lesion volume on DWI, stroke lesion side, NIHSS score at any time point, Fazekas scale score, and plasma Aβ42 and tau levels within 7 days were not predictors of PSCI at 1 year. In multivariate analysis, plasma Aβ42, tau, and Aβ42/40 ratio were included in separate models because of their high association (correlation coefficient of plasma Aβ42 and tau within 7 days = 0.69, P < 0.001; correlation coefficient of plasma Aβ42 and tau at 3 months = 0.89, P < 0.001). MoCA scores at 3 months and the plasma Aβ42 level at 3 months as well as MoCA scores at 3 months and the plasma tau level at 3 months were included in the automatic forward variable selection models with good model fits (Nagelkerke R2 = 0.43 and 0.45, and Hosmer–Lemeshow test P = 0.55 and 0.52, respectively). However, the Aβ42/40 ratio at 3 months became non-significant after adjusting for MoCA scores at 3 months. Therefore, MoCA scores at 3 months and plasma Aβ42 and tau levels at 3 months were the most significant and independent predictors of PSCI at 1 year. The ROC curves of MoCA scores at 3 months, Aβ42 levels at 3 months, Aβ42/40 ratios at 3 months, and tau levels at 3 months for predicting PSCI at 1 year are displayed in Figure 3. In addition, the ROC curves of multivariate models to predict PSCI at 1 year are displayed in Figure 4.
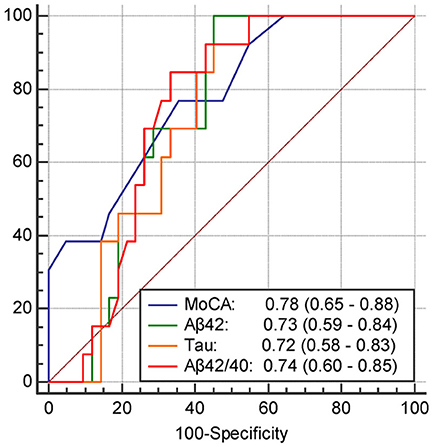
Figure 3. The receiver operating characteristics curve and the area under curves (95% confidence interval) of the variables predicting PSCI at 1 year. MoCA, The score of MoCA at 3 months; Aβ42, The plasma Aβ42 level at 3 months; tau, The plasma tau level at 3 months; Aβ42/40, The Aβ42/40 ratio at 3 months.
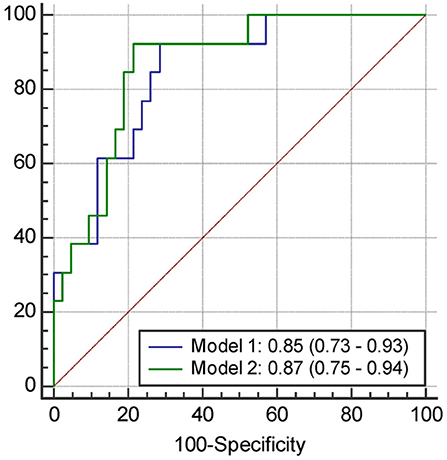
Figure 4. The receiver operating characteristics curve and the area under curves (95% confidence interval) of the variables predicting PSCI at 1 year. Model 1, Including the score of MoCA at 3 months and plasma Aβ42 level at 3 months; Model 2, Including the score of MoCA at 3 months and plasma tau level at 3 months.
Discussion
The present study followed up 55 patients with acute ischemic stroke for 1 year to investigate the risk factors for and plasma biomarkers of PSCI. Although the average MoCA scores and the incidence of PSCI in all participants did not change between 3 months and 1 year, cognitive function improvements were observed in some patients, whereas cognitive function deterioration was observed in some patients. The patients with PSCI at 1 year had more prominent cognitive function decline than the patients without PSCI, which implied that a degenerative process occurred in the patients with PSCI at 1 year. Plasma Aβ42 and tau levels at 3 months were significant predictors of PSCI at 1 year, but not at 3 months, implying that ischemic-induced AD pathology may be one mechanism of PSCI development after 3 months of stroke.
The prevalence of PSCI in previous studies varied from 20% to 70% based on different definitions of PSCI and observational timing. Most studies have adopted cross-sectional designs and have evaluated patients at 3 months after a stroke (23–29), whereas some studies have applied longitudinal designs (30–33). In addition, commonly applied diagnostic tools include Diagnostic and Statistical Manual of Mental Disorders (DSM), Informant Questionnaire on Cognitive Decline in the Elderly (IQCODE), Mini-Mental State Examination (MMSE), or MoCA. In studies applying an MMSE score of <26 or an MoCA score <26 as the indicator of PSCI, the prevalence of PSCI was ~50% (23, 33). However, in studies applying an MMSE score of <24 as the indicator of PSCI, the prevalence ranged from 20 to 30% (27, 28, 30). In the present study, we selected an MoCA score <23 as the indicator of PSCI because an updated systemic review recommended the adoption of the MoCA score of 23 as the cutoff, considering its overall superior diagnostic accuracy and lower false positive rates compared with the initially recommended cutoff score of 26 (18). The PSCI prevalence in our study (13 of 55; 24%) is consistent with the results of other studies that have applied similar diagnostic criteria.
In the longitudinal studies, the PSCI prevalence remained relatively stable over months to 1 year, and improvements in cognitive function were observed in some patients, whereas cognitive function deterioration was observed in some patients (30, 31, 33). The findings are consistent with the results of our study: 7 of 13 patients with PSCI at 3 months recovered to a cognitively normal state at 1 year, and 7 of 42 cognitively normal patients at 3 months developed PSCI at 1 year.
Changes in cognitive function after a stroke occur at different stages, and it is critical to identify the risk factors for cognitive function deterioration. In the present study, a low education level and pre-existing white matter disease were the major risk factors for PSCI at 3 months; this finding is consistent with those of previous studies (28, 29). Age, diabetes mellitus, and stroke lesion volume were non-significantly associated with PSCI at 3 months in the present study; however, they have been reported as significant risk factors for PSCI in some studies (33, 34). Age and education level are not treatable risk factors, but vascular comorbidities are treated regularly in all patients with stroke; however, the incidence of PSCI remains high. Therefore, it is necessary to explore novel treatable risk factors.
Regarding the changes in MoCA scores from 3 months to 1 year, most patients with PSCI at 1 year (10 of 13) had MoCA scores that decreased by 2 points or more, and in most patients without PSCI at 1 year (39 of 42), their MoCA scores remained unchanged (score ± 1 point) or increased (by 2 points or more) (Table 3). Therefore, the development of PSCI from 3 months to 1 year could be a degenerative process. In patients with PSCI at 1 year, poor cognitive performance at 3 months is a recognized risk factor (30, 33), whereas the decrease in plasma Aβ42 or tau levels at 3 months has not been reported as a risk factor previously.
Decreased levels of plasma Aβ42 have been observed in patients with AD, which is consistent with increased Aβ42 deposition in the brain and a decreased Aβ42 level in the CSF (12, 13). The findings further suggest that Aβ42 deposition in the brain may cause PSCI development at 1 year. Animal studies have suggested that cerebral ischemia induces the deposition of Aβ42 (35, 36). In addition, Aβ42 deposition has been observed in one-third of patients with stroke and dementia using PET (37). Vascular risk factors increase the incidence of AD, and patients with AD are at higher risk of subsequent stroke (38). Therefore, AD pathology and ischemia are associated. However, it remains unclear whether AD pathology is linked to the onset of PSCI. In the present study, in the patients admitted within 7 days, plasma Aβ42 levels did not vary between patients with and without PSCI; however, low plasma Aβ42 levels at 3 months were associated with PSCI development at 1 year. Plasma Aβ42 levels exhibited a decreasing trend from 7 days to 3 months in the patients with PSCI at 1 year, although the decreases were non-significant (16.4 vs. 15.1, P = 0.102). Therefore, in the patients with PSCI in the present study, stroke potentially induced AD pathology, and AD pathology potentially did not exist before stroke occurrence.
The decreased plasma tau levels in the patients with PSCI in the present study may not be due to AD. In addition, plasma tau levels in patients with AD were higher than those in cognitively normal controls; however, the overlap in plasma tau levels between patients with AD and controls was high, and it has been reported that plasma tau levels partly reflect AD pathology (14). It has also been reported that plasma tau levels are positively associated with plasma Aβ42 levels, are not positively associated with CSF tau level levels, and are not different between patients with and without AD pathology (12). It is unclear whether the plasma tau levels are associated with the degree of taupathy in the brain. Therefore, in the present study, the decreased levels of plasma tau in the patients with PSCI could be explained by its association with the decreased plasma Aβ42 levels (12). Further investigations on the factors influencing plasma tau levels are required.
In the present study, the levels of plasma tau were more than 15 pg/mL on average; however, the average plasma tau levels measured using SIOMA have been reported to be ~4 pg/mL (39). The use of different assay technologies may result in inconsistency in the reported levels. In previous studies, the levels of plasma tau measured using IMR have been reported to be higher than 10 pg/mL (15, 40, 41). In the present study, the plasma tau levels obtained using IMR are higher than those obtained using SIMOA in other studies. The major reason may be that in SIMOA, magnetic beads are used to collect tau protein molecules and concentrate/purify low levels of the tau protein. The collected tau protein molecules are delivered to the wells, in which optical labels bind with the tau molecules to generate optical signals. During the process, the loss of tau protein molecules occurs. Conversely, in IMR, there is neither a concentration/purification step nor a delivery step, and the loss of tau protein molecules in IMR is lower than that in SIMOA. From a molecular structure perspective, two epitopes of the tau protein are required for SIMOA, whereas only one epitope is required for IMR. The tau protein is easily truncated. Although SIMOA cannot detect truncated tau protein molecules, IMR can do so. Therefore, IMR provides higher levels of the tau protein than SIMOA.
The present study had several limitations. First, the sample size was small, and a larger sample size is required to validate the results reported. Second, most patients in the present study had small-vessel disease with mild severity, which may influence the generalizability of the findings. However, large infarct caused by major artery occlusion or cardioembolism often causes PSCI instantly, which is not the target of most studies of PSCI. Third, we did not perform CSF analysis or PET, and plasma Aβ42 and tau levels are indirect measures of the condition of the central nervous system. However, it would be challenging to track changes in Aβ42 and tau levels based on CSF analysis or PET due to their invasiveness and high costs. Fourth, we used MoCA alone to diagnose PSCI. Multiple factors may influence the accuracy of a single neuropsychological test in the diagnosis of PSCI, including education level, physiological condition, and sensitivity or specificity of the test itself. The application of other evaluation methods in addition to MoCA, such as the NINDS-Canadian Stroke Network (NINDS-CSN) neuropsychological battery, could enhance the accuracy of the diagnosis.
Conclusions
Changes in cognitive function occur at different stages after stroke. The pathogenesis of PSCI is complex. A low education level and pre-existing white matter disease are the key factors influencing PSCI development at 3 months, and ischemia-induced AD pathology is associated with PSCI development at 1 year. Such knowledge could facilitate the identification of patients at risk of developing PSCI. Exercise or cognitive training programs in addition to aggressive control of vascular risk factors may prevent PSCI in such patients.
Data Availability
The datasets generated for this study are available on request to the corresponding author.
Ethics Statement
The Institutional Review Board of Taipei Medical University approved this study. Written informed consent was obtained from all patients or their legal guardians.
Author Contributions
N-FC, H-YC, and C-JH: conceptualization and methodology. N-FC and C-JH: data curation and writing–original draft. N-FC, S-PC, and C-JH: formal analysis. H-YC and C-JH: funding acquisition and supervision. N-FC, S-PC, L-KH, LC, and C-JH: investigation. Y-RC: project administration. S-PC, L-KH, LC, Y-RC, H-YC, and C-JH: resources. N-FC, S-PC, L-KH, LC, Y-RC, H-YC, and C-JH: writing–review and editing.
Funding
This study was supported by Academia Sinica Stroke Biosignature Project (BM10701010021), Taiwan Ministry of Science and Technology (MOST) Clinical Trial Consortium for Stroke (MOST 107-2321-B-039-004, 106-2314-B-038-001, 107-2314-B-038-050).
Conflict of Interest Statement
The authors declare that the research was conducted in the absence of any commercial or financial relationships that could be construed as a potential conflict of interest.
References
1. Pendlebury ST, Rothwell PM. Prevalence, incidence, and factors associated with pre-stroke and post-stroke dementia: a systematic review and meta-analysis. Lancet Neurol. (2009) 8:1006–18. doi: 10.1016/S1474-4422(09)70236-4
2. Mijajlović MD, Pavlović A, Brainin M, Heiss W-D, Quinn TJ, Ihle-Hansen HB, et al. Post-stroke dementia – a comprehensive review. BMC Med. (2017) 15:11. doi: 10.1186/s12916-017-0779-7
3. Cumming TB, Brodtmann A, Darby D, Bernhardt J. The importance of cognition to quality of life after stroke. J Psychosom Res. (2014) 77:374–9. doi: 10.1016/j.jpsychores.2014.08.009
4. Stephens S, Kenny RA, Rowan E, Kalaria RN, Bradbury M, Pearce R, et al. Association between mild vascular cognitive impairment and impaired activities of daily living in older stroke survivors without dementia. J Am Geriatr Soc. (2005) 53:103–7. doi: 10.1111/j.1532-5415.2005.53019.x
5. Levine DA, Galecki AT, Langa KM, Unverzagt FW, Kabeto MU, Giordani B, et al. Trajectory of cognitive decline after incident stroke. JAMA J Am Med Assoc. (2015) 314:41–51. doi: 10.1001/jama.2015.6968
6. Dichgans M, Leys D. Vascular cognitive impairment. Circ Res. (2017) 120:573–91. doi: 10.1161/CIRCRESAHA.116.308426
7. Whitehead SN, Thiel A, Cechetto DF, Heiss W-D, Hachinski V, Whitehead SN. Amyloid burden, neuroinflammation, and links to cognitive decline after ischemic stroke. Stroke. (2014) 45:2825–9. doi: 10.1161/STROKEAHA.114.004285
8. Mailliot C, Podevin-Dimster V, Rosenthal RE, Sergeant N, Delacourte A, Fiskum G, et al. Rapid tau protein dephosphorylation and differential rephosphorylation during cardiac arrest-induced cerebral ischemia and reperfusion. J Cereb Blood Flow Metab. (2000) 20:543–9. doi: 10.1097/00004647-200003000-00013
9. Rosenberg GA, Bjerke M, Wallin A. Multimodal markers of inflammation in the subcortical ischemic vascular disease type of vascular cognitive impairment. Stroke. (2014) 45:1531–8. doi: 10.1161/STROKEAHA.113.004534
10. Landau SM, Lu M, Joshi AD, Pontecorvo M, Mintun MA, Trojanowski JQ, et al. Comparing positron emission tomography imaging and cerebrospinal fluid measurements of β-amyloid. Ann Neurol. (2013) 74:826–36. doi: 10.1002/ana.23908
11. LaJoie R, Bejanin A, Fagan AM, Ayakta N, Baker SL, Bourakova V, et al. Associations between [18F]AV1451 tau PET and CSF measures of tau pathology in a clinical sample. Neurology. (2018) 90:e282–90. doi: 10.1212/WNL.0000000000004860
12. Verberk IMW, Slot RE, Verfaillie SCJ, Heijst H, Prins ND, vanBerckel BNM, et al. Plasma amyloid as prescreener for the earliest alzheimer pathological changes. Ann Neurol. (2018) 84:648–58. doi: 10.1002/ana.25334
13. Hanon O, Vidal JS, Lehmann S, Bombois S, Allinquant B, Tréluyer JM, et al. Plasma amyloid levels within the Alzheimer's process and correlations with central biomarkers. Alzheimers Dement. (2018) 14:858–68. doi: 10.1016/j.jalz.2018.01.004
14. Mattsson N, Zetterberg H, Janelidze S, Insel PS, Andreasson U, Stomrud E, et al. Plasma tau in Alzheimer disease. Neurology. (2016) 87:1827–35. doi: 10.1212/WNL.0000000000003246
15. Yang CC, Chiu MJ, Chen TF, Chang HL, Liu BH, Yang SY. Assay of plasma phosphorylated tau protein (threonine 181) and total tau protein in early-stage Alzheimer's disease. J Alzheimers Dis. (2018) 61:1323–32. doi: 10.3233/JAD-170810
16. Tang S-C, Yang K-C, Chen C-H, Yang S-Y, Chiu M-J, Wu C-C, et al. Plasma β-amyloids and tau proteins in patients with vascular cognitive impairment. Neuromol Med. (2018) 20:498–503. doi: 10.1007/s12017-018-8513-y
17. Nasreddine ZS, Phillips NA, Bédirian V, Charbonneau S, Whitehead V, Collin I, et al. The Montreal Cognitive Assessment, MoCA: a brief screening tool for mild cognitive impairment. J Am Geriatr Soc. (2005) 53:695–9. doi: 10.1111/j.1532-5415.2005.53221.x
18. Carson N, Leach L, Murphy KJ. A re-examination of Montreal Cognitive Assessment (MoCA) cutoff scores. Int J Geriatr Psychiatry. (2018) 33:379–88. doi: 10.1002/gps.4756
19. Fazekas F, Chawluk JB, Alavi A, Hurtig HI, Zimmerman RA. Mr Signal Abnormalities At 1.5-T in Alzheimer Dementia and Normal Aging. Am J Roentgenol. (1987) 149:351–6. doi: 10.2214/ajr.149.2.351
20. Adams HP Jr, Bendixen BH, Kappelle LJ, Biller J, Love BB, Gordon DL, et al. Classification of subtype of acute ischemic stroke. Definitions for use in a multicenter clinical trial. TOAST. Trial of Org 10172 in Acute Stroke Treatment. Stroke. (1993) 24:35–41.
21. Yang C-C, Yang S-Y, Chieh J-J, Horng H-E, Hong C-Y, Yang H-C, et al. Biofunctionalized magnetic nanoparticles for specifically detecting biomarkers of Alzheimer's disease in vitro. ACS Chem Neurosci. (2011) 2:500–5. doi: 10.1021/cn200028j
22. Chiu MJ, Yang SY, Horng HE, Yang CC, Chen TF, Chieh JJ, et al. Combined plasma biomarkers for diagnosing mild cognition impairment and Alzheimer's disease. ACS Chem Neurosci. (2013) 4:1530–6. doi: 10.1021/cn400129p
23. Jacquin A, Binquet C, Rouaud O, Graule-Petot A, Daubail B, Osseby G-V, et al. Post-stroke cognitive impairment: high prevalence and determining factors in a cohort of mild stroke. J Alzheimers Dis. (2014) 40:1029–38. doi: 10.3233/JAD-131580
24. Srikanth VK, Thrift AG, Saling MM, Anderson JFI, Dewey HM, Macdonell RAL, et al. Increased risk of cognitive impairment 3 months after mild to moderate first-ever stroke: a community-based prospective study of nonaphasic english-speaking survivors. Stroke. (2003) 34:1136–43. doi: 10.1161/01.STR.0000069161.35736.39
25. Lisabeth LD, Sánchez BN, Baek J, Skolarus LE, Smith MA, Garcia N, et al. Neurological, functional, and cognitive stroke outcomes in Mexican Americans. Stroke. (2014) 45:1096–101. doi: 10.1161/STROKEAHA.113.003912
26. Yu KH, Cho SJ, Oh MS, Jung S, Lee JH, Shin JH, et al. Cognitive impairment evaluated with vascular cognitive impairment harmonization standards in a multicenter prospective stroke cohort in Korea. Stroke. (2013) 44:786–8. doi: 10.1161/STROKEAHA.112.668343
27. Tang WK, Chan SSM, Chiu HFK, Ungvari GS, Wong KS, Kwok TCY, et al. Frequency and clinical determinants of poststroke cognitive impairment in nondemented stroke patients. J Geriatr Psychiatry Neurol. (2006) 19:65–71. doi: 10.1177/0891988706286230
28. Zhou DHD, Wang JYJ, Li J, Deng J, Gao C, Chen M. Frequency and risk factors of vascular cognitive impairment three months after ischemic stroke in china: the Chongqing stroke study. Neuroepidemiology. (2005) 24:87–95. doi: 10.1159/000081055
29. Tu Q, Ding B, Yang X, Bai S, Tu J, Liu X, et al. The current situation on vascular cognitive impairment after ischemic stroke in Changsha. Arch Gerontol Geriatr. (2014) 58:236–47. doi: 10.1016/j.archger.2013.09.006
30. Douiri A, Rudd AG, Wolfe CDA. Prevalence of poststroke cognitive impairment: South London stroke register 1995-2010. Stroke. (2013) 44:138–45. doi: 10.1161/STROKEAHA.112.670844
31. Das S, Paul N, Hazra A, Ghosal M, Ray BK, Banerjee TK, et al. Cognitive dysfunction in stroke survivors: a community-based prospective study from Kolkata, India. J Stroke Cerebrovasc Dis. (2013) 22:1233–42. doi: 10.1016/j.jstrokecerebrovasdis.2012.03.008
32. Rasquin SMC, Verhey FRJ, VanOostenbrugge RJ, Lousberg R, Lodder J. Demographic and CT scan features related to cognitive impairment in the first year after stroke. J Neurol Neurosurg Psychiatry. (2004) 75:1562–7. doi: 10.1136/jnnp.2003.024190
33. Nijsse B, Visser-Meily JMA, VanMierlo ML, Post MWM, DeKort PLM, VanHeugten CM. Temporal evolution of poststroke cognitive impairment using the montreal cognitive assessment. Stroke. (2017) 48:98–104. doi: 10.1161/STROKEAHA.116.014168
34. Hassing LB, Johansson B, Nilsson SE, Berg S, Pedersen NL, Gatz M, et al. Diabetes mellitus is a risk factor for vascular dementia, but not for Alzheimer's disease: a population-based study of the oldest old. Int Psychogeriatr. (2002) 14:239–48.
35. Koike MA, Green KN, Blurton-Jones M, Laferla FM. Oligemic hypoperfusion differentially affects tau and amyloid-{beta}. Am J Pathol. (2010) 177:300–10. doi: 10.2353/ajpath.2010.090750
36. Yamada M, Ihara M, Okamoto Y, Maki T, Washida K, Kitamura A, et al. The influence of chronic cerebral hypoperfusion on cognitive function and amyloid β metabolism in app overexpressing mice. PLoS ONE. (2011) 6:doi: 10.1371/journal.pone.0016567
37. Liu W, Wong A, Law ACK, Mok VCT. Cerebrovascular disease, amyloid plaques, and dementia. Stroke. (2015) 46:1402–7. doi: 10.1161/STROKEAHA.114.006571
38. Chi N-F, Chien L-N, Ku H-LH-L, Hu C-JC-J, Chiou H-YH-Y. Alzheimer disease and risk of stroke: a population-based cohort study. Neurology. (2013) 80:705–11. doi: 10.1212/WNL.0b013e31828250af
39. Pase MP, Beiser AS, Himali JJ, Satizabal CL, Aparicio HJ, DeCarli C, et al. Assessment of plasma total tau level as a predictive biomarker for dementia and related endophenotypes. JAMA Neurol. (2019) 76:598–606. doi: 10.1001/jamaneurol.2018.4666.
40. Chiu MJ, Chen YF, Chen TF, Yang SY, Yang FPG, Tseng TW, et al. Plasma tau as a window to the brain-negative associations with brain volume and memory function in mild cognitive impairment and early alzheimer's disease. Hum Brain Mapp. (2014) 35:3132–42. doi: 10.1002/hbm.22390
Keywords: tau, Amyloid (A) 42, post-stroke cognitive impairment, vascular cognition impairment, Montreal Cognitive Assessment (MoCA)
Citation: Chi N-F, Chao S-P, Huang L-K, Chan L, Chen Y-R, Chiou H-Y and Hu C-J (2019) Plasma Amyloid Beta and Tau Levels Are Predictors of Post-stroke Cognitive Impairment: A Longitudinal Study. Front. Neurol. 10:715. doi: 10.3389/fneur.2019.00715
Received: 11 February 2019; Accepted: 17 June 2019;
Published: 02 July 2019.
Edited by:
Hamid R. Sohrabi, Edith Cowan University, AustraliaReviewed by:
Peng Lei, Sichuan University, ChinaPratishtha Chatterjee, Macquarie University, Australia
Copyright © 2019 Chi, Chao, Huang, Chan, Chen, Chiou and Hu. This is an open-access article distributed under the terms of the Creative Commons Attribution License (CC BY). The use, distribution or reproduction in other forums is permitted, provided the original author(s) and the copyright owner(s) are credited and that the original publication in this journal is cited, in accordance with accepted academic practice. No use, distribution or reproduction is permitted which does not comply with these terms.
*Correspondence: Chaur-Jong Hu, chaurjongh@tmu.edu.tw