- 1Department of Psychiatry, First Hospital/First Clinical Medical College of Shanxi Medical University, Taiyuan, China
- 2MDT Center for Cognitive Impairment and Sleep Disorders, First Hospital of Shanxi Medical University, Taiyuan, China
- 3National Key Disciplines, Key Laboratory for Cellular Physiology of Ministry of Education, Department of Neurobiology, Shanxi Medical University, Taiyuan, China
- 4Department of Humanities and Social Science, Shanxi Medical University, Taiyuan, China
Electroconvulsive therapy (ECT) was established based on Meduna's hypothesis that there is an antagonism between schizophrenia and epilepsy, and that the induction of a seizure could alleviate the symptoms of schizophrenia. However, subsequent investigations of the mechanisms of ECT have largely ignored this originally established relationship between these two disorders. With the development of functional magnetic resonance imaging (fMRI), brain-network studies have demonstrated that schizophrenia and epilepsy share common dysfunctions in the default-mode network (DMN), saliency network (SN), dorsal-attention network (DAN), and central-executive network (CEN). Additionally, fMRI-defined brain networks have also been shown to be useful in the evaluation of the treatment efficacy of ECT. Here, we compared the ECT-induced changes in the pathological conditions between schizophrenia and epilepsy in order to offer further insight as to whether the mechanisms of ECT are truly based on antagonistic and/or affinitive relationships between these two disorders.
Introduction
Electroconvulsive therapy (ECT) is one of the oldest therapeutic modalities in psychiatric clinical practice. The therapeutic effects of ECT putatively depend on the ECT-induced manifestation of seizure-like states, which was first proposed by the Hungarian neuropsychiatrist Ladislas Meduna in 1934 (1). This hypothesis arose from conspicuously opposite pathological results in epileptic vs. schizophrenic patients; Meduna observed an excess of glial cells in the brain tissue of epileptic patients (2), while his colleague found a reduction of glial cells in the brain tissue of schizophrenic patients (3). This findings convinced Meduna that there was an antagonism between schizophrenia and epilepsy, and gave birth to the idea that induction of seizure might help to alleviate the symptoms of schizophrenia. Subsequently, Meduna conducted the first human experiment by using camphor intramuscular injections (4). Finally, camphor was replaced by electricity to achieve more stable therapeutic effects by Ugo Cerletti and Lucio Bini, from which ECT was born (5).
Although Meduna' hypothesis has since been refuted by the finding that different glial subtypes have different pathological features and do not exhibit a homogeneous opposing relationship between epilepsy and schizophrenia (6, 7), the relationship between these two disorders is still an interesting topic and has been debated for many years (8, 9). Schizophrenia is a serious psychiatric disorder that is always characterized by positive symptoms (hallucinations and delusions), negative symptoms (emotional disorders and impaired motivations), and cognitive impairment (10). Temporal lobe epilepsy (TLE) is the most widely studied and specific subtype of epilepsy (11, 12). Since these two neuropsychiatric disorders always shared some common symptoms, such as psychosis (13), emotion recognition disorders (14), and cognitive impairment (15), TLE showed a tight relation with schizophrenia in clinical diagnosis. The primary point of controversy is whether these two disorders have biological antagonism or affinity with one another. Some recent neuropathological studies still supported the antagonistic hypothesis for decreased astrocyte numbers in schizophrenia (16, 17) and increased astrocyte numbers and size in epilepsy (7). However, increasing sources of data also indicate that these two disorders may share some similarity. One study reported that there were some overlapping etiological factors between epilepsy and schizophrenia based on a population-based family study (18). Another study reported that schizophrenia and epilepsy share common features at the genetic level (19). As such, the relationship between epilepsy and schizophrenia is still an open question and requires further exploration for its elucidation.
Originating from the putative relationship between epilepsy and schizophrenia, ECT is now widely used for depression, acute manic episodes, catatonia, and treatment-resistant schizophrenia (20). Over the past 80 years, numerous psychological, psychoanalytical, and biological theories have been built to posit the potential therapeutic mechanisms of ECT (5). The present putative mechanisms of ECT have been primarily focused on structural, functional, and compositional changes of the brain after ECT treatment (21). Among these phenomena, the roles of cerebral blood flow (22), the blood-brain barrier (23), neurotransmitters (24), and the immune system (25) during ECT have been investigated. However, the potential mechanisms of ECT based on the correlation of epilepsy and schizophrenia remain largely unknown.
With the development of structural magnetic resonance imaging (sMRI) and functional MRI (fMRI), both structural and functional neuroimaging studies have provided more information to help better understand the relationship between schizophrenia and epilepsy (11, 26). Based on neuroimaging data, brain networks have been defined as correlational networks between several related brain regions in resting or task conditions. Disrupted brain networks—including the default-mode network (DMN), dorsal-attention network (DAN), central-executive network (CEN), and saliency network (SN)—have been detected in both schizophrenic (27, 28) and epileptic patients (29–31). These shared brain networks may help to better understand the relationship between schizophrenia and epilepsy. These disease-disrupted brain networks can also be used as valuable biomarkers for assaying the therapeutic effects of ECT (32–34), which have shown apparent changes after ECT treatment at both structural and functional levels. Thus, comparison of the ECT-induced changes in the pathological conditions of schizophrenia and epilepsy may offer further insight as to whether the mechanisms of ECT are truly based on antagonistic and/or affinitive relationships between these two disorders.
This review will focus on brain-network changes in schizophrenia and epilepsy to discuss the affinity and/or antagonism between these two disorders. Our view was synthesized based on brain networks including the DMN, DAN, CEN, and SN, and a more large scale assessment of networks from graph theory. Additionally, changes in brain networks after ECT treatment will be compared under these two pathological conditions to help better understand the principles of ECT. All of the cited articles included in this review were written and published in English and were published before January 2019. The search engine, PubMed, was used with MESH terms.
Default-Mode Network
Initial fMRI studies mainly focused on task-induced increases in regional brain activities during goal-directed behaviors. Subsequently, it was found that there are also consistent and task-independent decreases in regional brain activities, which is a phenomenon that has been defined as the baseline or default mode of brain function (35). Since then, the default mode of brain function has generated far more interest, discussion, and controversy, and it has called more attention to the importance of intrinsic brain activities (36). Combined with blood-oxygen-level-dependent (BOLD) signals and diffusion-tensor imaging (DTI) data, these intrinsic brain activities have been shown to depend on networks across several brain regions, which collectively has been termed the DMN and consists of the following: the posterior cingulate cortex (PCC); adjacent precuneus (PCUN); medial prefrontal cortex (mPFC); mesial and inferior temporal lobes (mTL/iTL); and the inferior parietal lobe (iPL) (37) (Figure 1A). Functionally, these intrinsic brain activities of the DMN are activated at rest, become deactivated at the initiation of a task, and play an important role in cognitive functions and emotional processing (38). In the N-back working-memory task, when cognition load increased, the functional connectivity within the DMN concomitantly decreased (39). Additionally, during long-term stabilization of memory, the intra-network synchrony of the DMN was positively corelated to individual performance (40). In emotional processing, less decreased DMN activity was highly related to poor emotionality (41). These physiological signatures of the DMN have attracted more and more attention in terms of their promising clinical applications in assessing and treating neuropsychiatric disorders.
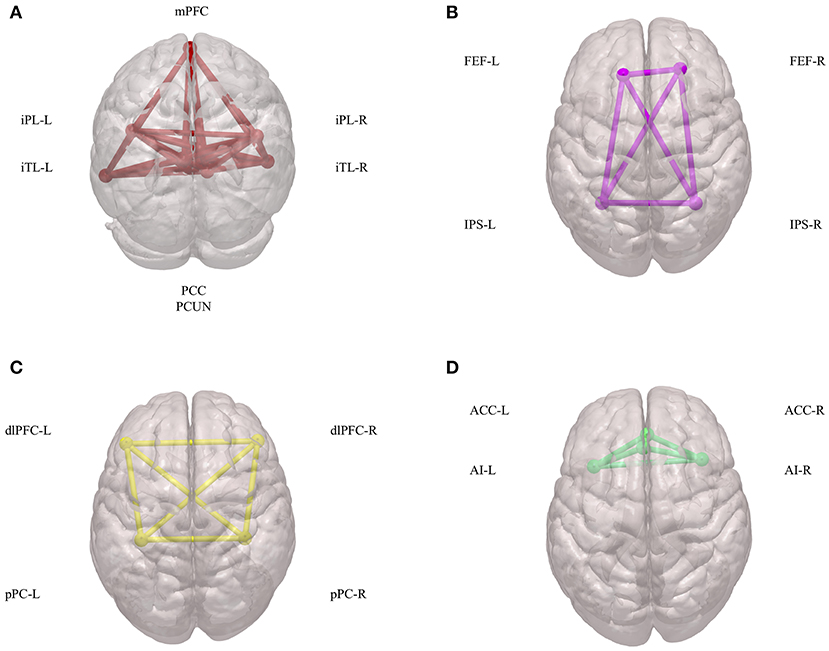
Figure 1. Components of DMN, DAN, CEN, and SN. (A) Spatial components of DMN. (B) Spatial components of DAN. (C) Spatial components of CEN. (D) Spatial components of SN. DMN, default mode network; DAN, dorsal attention network; CEN, central executive network; SN, salience network; mPFC, medial prefrontal cortex; iPL, inferior parietal lobe; iTL, inferior temporal lobes; PCC, posterior cingulate cortex; PCUN, adjacent precuneus; FEF, frontal eye fields; IPS, intraparietal sulcus; dlPFC, dorsal lateral prefrontal cortex; pPC, posterior parietal cortex; ACC, anterior cingulate cortex; AI, anterior insula.
Brain network studies have demonstrated increasing evidence of correlations between the DMN and some symptoms of schizophrenia (42). Auditory verbal hallucinations represent the most common positive symptom of schizophrenia and induce alterations of functional connectivity within the DMN (43). Additionally, patients with delusions also exhibit reduced regional deactivation of the DMN (44). Besides typical symptoms, positive symptoms scored by Positive and Negative Syndrome Scale (PANSS) have also been shown to be positively correlated with increased deactivation of brain regions, including the medial frontal, temporal, and cingulate gyri (45). For negative symptoms, emotional disorders of patients were found to be positively related to the magnitude of deactivation of rAC and mPFC regions (46). Additionally, negative symptoms scored by SANS have been shown to have a linear correlation to the functional connectivity within the DMN (47). These results indicate that changes of deactivation and functional connectivity within the DMN may represent a valuable means for assessing positive and negative schizophrenic symptoms; however, further evidence is required before this potential utility is sufficiently verified. Since the DMN is an important brain network that participates in cognitive processes, the relationship between the DMN and cognitive impairment of schizophrenia has also been widely studied. In a working memory task, the mPFC showed less deactivation (48) and greater activation (49). When the task load changed, DMN functions were over-recruited during a low-task load, and hyper-deactivated during a high-task load (50). Other brain regions, such as the left-superior temporal gyrus, have also been shown to be positively correlated to cognitive impairment (51). In addition to BOLD-based functional studies, DTI anatomical data have also revealed that altered frontal structural connectivity is corelated to cognitive ability as well as schizophrenic symptoms (52).
Studies of the DMN in TLE have indicated altered task-related deactivations compared with those of healthy controls (53) and reduced functional connectivity (54). However, it may be difficult to confirm the affinity or antagonistic relationship of psychiatric manifestations between schizophrenia and TLE based on the present DMN results. More well-designed investigations are needed in terms of elucidating the short-term and long-term effects of seizure on the DMN, and for the comparison of the symptoms of these two disorders. Similar to schizophrenia, the role of the DMN in TLE-induced cognitive impairment has also been comprehensively studied. In the N-back working-memory task, the ACC showed greater deactivation in TLE patients (55). Additionally, there was decreased functional connectivity between the mPFC and mTL/iTL (54), as well as the mPFC and hippocampus (56). Interestingly, comparison of the cognitive-related DMN changes between TLE and schizophrenia suggests an affinity between these two disorders. This relationship may be due to similar pathological changes in terms of the hippocampus exhibiting overlapping pathological features between the two disorders (57).
Clinical practices have demonstrated that ECT can effectively ameliorate the positive symptoms of schizophrenia (58) and significantly reduce PANSS scores (59). Additionally, a brain network study revealed that increased mTL connectivity and PCC volume were accompanied by clinical improvement (33). These results may suggest that the therapeutic effects of ECT are achieved by an opposite regulation of the DMN in schizophrenia. However, this hypothesis still requires additional evidence from large-sample investigations. Additionally, whether this opposing change of the DMN can be detected in TLE patients requires further investigation. By exploring seizure-induced changes in TLE patients, we may better elucidate the relationship between schizophrenia and TLE and any therapeutic outcomes of ECT. Although ECT is effective, the side effects of ECT still harbor great concerns (60). Also, although similar cognitive impairments between schizophrenia and TLE have been documented, further studies are required to determine whether the side effects of ECT are related to seizure-induced hippocampal dysfunction.
Dorsal Attention Network
The DAN has been defined as a network that is comprised of the intraparietal sulcus (IPS) and the frontal eye fields (FEF) (61) (Figure 1B). Both IPS and FEF within the DAN play an important role in the maintenance of spatial attention, saccade planning, and visual working memory (62). The DAN is also activated during feature-based attention and provides a spatial coding in multiple reference frames (63). Furthermore, there is an interaction between the DAN and the DMN to carry out brain functions (64).
The DAN has been found to be altered in schizophrenic patients while carrying out several tasks that are mainly related to cognitive impairment. In visual attention and motor learning tasks, patients had reduced activation in the dorsal neocortical visual attention network (65). In a visual oddball task, connectivity between the right IPS (intraparietal sulcus) and right anterior insula (AI, a component of the ventral network) was significantly decreased in schizophrenic patients (66). In an N-back task, patients with schizophrenia had decreased inhibitory self-connections within the DAN regions, particularly in the left FEF and the left SPL (67). The interacting changes between the DAN and other networks have also been found. In schizophrenics during a working memory task, the DMN connectivity with the DAN was decreased (68, 69). In contrast to the default mode, patients demonstrated less connectivity in the executive control and dorsal attention networks (70).
Patients with TLE presented decreased functional connectivity in almost all of the regions within the DAN (31, 71). For example, the FC values of the bilateral frontal eye field (FEF) and left intraparietal sulcus (IPS) were decreased (72). Thus, the DAN can also be regarded as a biomarker to explain the common cognition pathological mechanisms between schizophrenia and TLE, for which neuroimaging studies have revealed similar connectivity changes. ECT has also been shown to influence the DAN in depressed patients (73). However, the influence of the DAN in schizophrenia requires further investigation.
Central-Executive Networks
The CEN is a brain network that links the dorsal lateral prefrontal cortex (dlPFC) and posterior parietal cortex (pPC) (74) (Figure 1C). The CEN is frequently activated during typical fMRI executive tasks and its activity is often contrary to that of DMN activity (75). Brain imaging studies have shown that intelligence differences are positively correlated to functional interactions within the CEN, in both children and adults (76). Additionally, another important role of the CEN is to inhibit the DMN functions under certain conditions (77). This coordination between the CEN and the DMN is important in many neuropsychiatric disorders.
From recent literature, most results have reported that the CEN participates in a triple network including the DMN, the CEN, and the salience network (SN) rather than serving an isolated role in schizophrenia (78). Thus, we will be discuss this topic after introducing the SN.
Salience Network
The SN is defined as a brain network comprised of the anterior insula (AI) and anterior cingulate cortex (ACC) (79) (Figure 1D). Physically, there is a strong functional connectivity within the SN, which is important for sensory perception and the coordination of behavioral responses (80, 81). Additionally, during many forms of emotional processing, the brain regions within the SN exhibit increased activity (82). The SN can also interact with the DMN and CEN to form a triple network, which participates in many mental process and disorders (83–85).
Schizophrenic patients show consistent abnormalities of insular signatures in both structural and functional neuroimaging studies, which indicates that the SN is involved in pathological processes (86). The SN has a direct correlation to schizophrenia symptoms, in which both auditory verbal hallucinations and delusions were detected to induce aberrant SN functional connectivity (87, 88). However, the strengths of these functional connectivities are not always homogeneous, because some studies have found a reduction in such connectivities (86), whereas other studies have reported a mixed pattern of increased and decreased connections (89). The interaction of the SN and DMN showed delayed communication that was directly correlated to positive and negative symptoms of schizophrenia (90). Additionally, a disrupted SN-CEN circuit also accounted for these schizophrenic symptoms (91). The triple network formed by SN-CEN-DMN nodes has shown dysregulated connections in schizophrenia (92) and mainly contributes to positive symptoms (93).
In TLE patients, one study reported that there was decreased connectivity to insula and ACC, suggesting a reduced SN (31). However, this result still needs to be further confirmed by more neuroimaging studies. When compared to schizophrenia, TLE showed a similar changes of the SN activity. However, it is difficult to confirm this hypothesis since there is still not enough neuroimaging evidence to properly assess this phenomenon. After ECT treatment, enhanced inter-network connectivity between the SN and the DMN has been found (73). However, whether this enhanced connectivity would be found in TLE patients requires further investigation.
The Emerging Role of Graph Theory
An increasing number of studies has supported that schizophrenia and TLE are disorders involving abnormal brain networks rather than several abnormal discrete brain regions (29, 70). It may be difficult to reach a unifying result by analysis of regional activation/deactivation or connectivity abnormalities. Therefore, graph theory, which is a mathematical framework that allows for the quantitative modeling and analysis of networks, has been applied with increasing success to neuroimaging data (94). In graph theory, the brain can be represented as a graph, and the set of nodes may be composed of brain regions or voxels (on a macroscopic level) or individual neurons (on a cellular level). Thus, edges will represent the connections between these brain regions/voxels or individual neurons depending on the conditions (95). Then, this information can be encoded in a mathematical data structure called a connectivity matrix. Based on the connectivity matrix, graph theory provides a more large-scale assessment of the human brain and can provide more integrative information of various diseases (96).
The results of graph theoretical analysis on schizophrenia are still inconsistent, but there has been some convergence around the concept of topological randomization (97, 98). Previous studies have shown that the functional brain networks of schizophrenia are relatively shifted toward the randomness of small-world topology (99). Another study also confirmed that schizophrenics demonstrate significant randomization of global network metrics (100). Additionally, the parameters of the global-network topology of schizophrenia have been found to be decreased in both functional and anatomical networks (96, 101).
On the contrary, graph theoretical analyses of networks have provided evidence generally suggesting a shift toward a more regularized topology in TLE patients (102). Within the range of small-world topologies, a more regularized network topology is present in TLE patients (103). The increased path length and clustering in TLE patients supports this more regularized arrangement (104). Thus, interestingly, topological characteristics have revealed an antagonistic feature between schizophrenia and epilepsy, although these studies of schizophrenia and epilepsy were independently conducted.
However, there are limited reports regarding the effects of ECT on brain-network dynamics in schizophrenia. Whether ECT treatment reverses randomized brain networks to a more regularized pattern will require further investigations. As for the side effects of ECT, graph analysis of TLE patients showed that individuals with poor seizure control experienced more severe memory impairment (105). These results may indicate that a well-organized ECT practice may reduce side effects, but further neuroimaging evidence is still needed to validate or refute this hypothesis.
Conclusions
Functional brain networks, such as the DMN, the SN and the DAN, have provided a new perspective to understand the relationship between schizophrenia and epilepsy. At this level, these two diseases show similar connectivity changes and suggest that they have more of an affinity-type relationship due to their similar pathological features. At larger scale, graph theoretical analysis has indicated an antagonistic relationship between these two diseases, although more evidence is needed to determine the validity of these findings. In addition, ECT treatment has been shown to modify the dynamics of these brain networks. If future studies verify that ECT treatment can reverse randomized brain networks in schizophrenia to more regularized patterns, the original premise for the creation of ECT may be further corroborated and better understood.
Author Contributions
YX designed and supervised the study. QL and SL drafted the manuscript. MG and C-XY collected some literature.
Funding
This work was supported by the National Key Research and Development Program of China (2016YFC1307004), the National Natural Science Foundation of China (81571319 and 81701326), the Multidisciplinary Team for Cognitive Impairment of Shanxi Science and Technology Innovation Training Team (201705D131027), the Support Program of the Youth Sanjin Scholars, and the 136 Medical Rejuvenation Project of Shanxi Province.
Conflict of Interest Statement
The authors declare that the research was conducted in the absence of any commercial or financial relationships that could be construed as a potential conflict of interest.
Acknowledgments
We thank LetPub (www.letpub.com) for its linguistic assistance during the preparation of this manuscript.
References
1. Fink M. Meduna and the origins of convulsive therapy. Am J Psychiatry. (1984) 141:1034–41. doi: 10.1176/ajp.141.9.1034
2. Meduna Lv. Beiträge zur Histopathologie der Mikroglia. Arch Psychiatr Nervenkrankheiten. (1928) 82, 123–193. doi: 10.1007/bf01828859
3. Hechst B. Zur Histopathologie der Schizophrenie mit besonderer Berücksichtigung der Ausbreitung des Prozessses. Z Gesamte Neurol Psychiatr. (1931) 134:163–267. doi: 10.1007/bf02896997
4. Gazdag G, Bitter I, Ungvari GS, Baran B, Fink M. Laszlo Meduna's pilot studies with camphor inductions of seizures: the first 11 patients. J ECT. (2009) 25:3–11. doi: 10.1097/YCT.0b013e31819359fc
5. Gazdag G, Ungvari GS. Electroconvulsive therapy: 80 years old and still going strong. World J Psychiatry. (2019) 9:1–6. doi: 10.5498/wjp.v9.i1.1
6. Bernstein HG, Steiner J, Guest PC, Dobrowolny H, Bogerts B. Glial cells as key players in schizophrenia pathology: recent insights and concepts of therapy. Schizophr Res. (2015) 161:4–18. doi: 10.1016/j.schres.2014.03.035
7. Farrell JS, Wolff MD, Teskey GC. Neurodegeneration and pathology in epilepsy: clinical and basic perspectives. Adv Neurobiol. (2017) 15:317–34. doi: 10.1007/978-3-319-57193-5_12
8. Reynolds EH. Epilepsy and schizophrenia: relationship and biochemistry. Lancet. (1968) 1:398–401.
9. Cascella NG, Schretlen DJ, Sawa A. Schizophrenia and epilepsy: is there a shared susceptibility? Neurosci Res. (2009) 63:227–35. doi: 10.1016/j.neures.2009.01.002
10. Owen MJ, Sawa A, Mortensen PB. Schizophrenia. Lancet. (2016) 388:86–97. doi: 10.1016/s0140-6736(15)01121-6
11. Butler T, Weisholtz D, Isenberg N, Harding E, Epstein J, Stern E, et al. Neuroimaging of frontal-limbic dysfunction in schizophrenia and epilepsy-related psychosis: toward a convergent neurobiology. Epilepsy Behav. (2012) 23:113–22. doi: 10.1016/j.yebeh.2011.11.004
12. Kandratavicius L, Hallak JE, Leite JP. What are the similarities and differences between schizophrenia and schizophrenia-like psychosis of epilepsy? A neuropathological approach to the understanding of schizophrenia spectrum and epilepsy. Epilepsy Behav. (2014) 38:143–7. doi: 10.1016/j.yebeh.2014.01.005
13. Hilger E, Zimprich F, Pataraia E, Aull-Watschinger S, Jung R, Baumgartner C, et al. Psychoses in epilepsy: a comparison of postictal and interictal psychoses. Epilepsy Behav. (2016) 60:58–62. doi: 10.1016/j.yebeh.2016.04.005
14. Monti G, Meletti S. Emotion recognition in temporal lobe epilepsy: a systematic review. Neurosci Biobehav Rev. (2015) 55:280–93. doi: 10.1016/j.neubiorev.2015.05.009
15. Allone C, Lo Buono V, Corallo F, Pisani LR, Pollicino P, Bramanti P, et al. Neuroimaging and cognitive functions in temporal lobe epilepsy: a review of the literature. J Neurol Sci. (2017) 381:7–15. doi: 10.1016/j.jns.2017.08.007
16. Steffek AE, McCullumsmith RE, Haroutunian V, Meador-Woodruff JH. Cortical expression of glial fibrillary acidic protein and glutamine synthetase is decreased in schizophrenia. Schizophr Res. (2008) 103:71–82. doi: 10.1016/j.schres.2008.04.032
17. Williams MR, Hampton T, Pearce RK, Hirsch SR, Ansorge O, Thom M, et al. Astrocyte decrease in the subgenual cingulate and callosal genu in schizophrenia. Eur Arch Psychiatry Clin Neurosci. (2013) 263:41–52. doi: 10.1007/s00406-012-0328-5
18. Clarke MC, Tanskanen A, Huttunen MO, Clancy M, Cotter DR, Cannon M. Evidence for shared susceptibility to epilepsy and psychosis: a population-based family study. Biol Psychiatry. (2012) 71:836–9. doi: 10.1016/j.biopsych.2012.01.011
19. Vonberg FW, Bigdeli TB. Genetic correlation between schizophrenia and epilepsy. JAMA Neurol. (2016) 73:125–6. doi: 10.1001/jamaneurol.2015.3480
20. Silvia HC, Mezzasalma MAU, Nardi AE. Pivotal role of tissue plasminogen activator in the mechanism of action of electroconvulsive therapy. J Psychopharmacol. (2014) 28:99–105. doi: 10.1177/0269881113507639
21. Singh A, Kar SK. How electroconvulsive therapy works?: understanding the neurobiological mechanisms. Clin Psychopharmacol Neurosci. (2017) 15:210–21. doi: 10.9758/cpn.2017.15.3.210
22. Takano H, Motohashi N, Uema T, Ogawa K, Ohnishi T, Nishikawa M, et al. Changes in regional cerebral blood flow during acute electroconvulsive therapy in patients with depression: positron emission tomographic study. Br J Psychiatry. (2007) 190:63–8. doi: 10.1192/bjp.bp.106.023036
23. Andrade C, Bolwig TG. Electroconvulsive therapy, hypertensive surge, blood-brain barrier breach, and amnesia: exploring the evidence for a connection. J ECT. (2014) 30:160–4. doi: 10.1097/yct.0000000000000133
24. Baldinger P, Lotan A, Frey R, Kasper S, Lerer B, Lanzenberger R. Neurotransmitters and electroconvulsive therapy. J ECT. (2014) 30:116–21. doi: 10.1097/yct.0000000000000138
25. Yrondi A, Sporer M, Peran P, Schmitt L, Arbus C, Sauvaget A. Electroconvulsive therapy, depression, the immune system and inflammation: a systematic review. Brain Stimul. (2018) 11:29–51. doi: 10.1016/j.brs.2017.10.013
26. Marsh L, Sullivan EV, Morrell M, Lim KO, Pfefferbaum A. Structural brain abnormalities in patients with schizophrenia, epilepsy, and epilepsy with chronic interictal psychosis. Psychiatry Res. (2001) 108:1–15. doi: 10.1016/s0925-4927(01)00115-9
27. Lee WH, Doucet GE, Leibu E, Frangou S. Resting-state network connectivity and metastability predict clinical symptoms in schizophrenia. Schizophr Res. (2018) 201:208–16. doi: 10.1016/j.schres.2018.04.029
28. Lefort-Besnard J, Bassett DS, Smallwood J, Margulies DS, Derntl B, Gruber O, et al. Different shades of default mode disturbance in schizophrenia: subnodal covariance estimation in structure and function. Hum Brain Mapp. (2018) 39:644–61. doi: 10.1002/hbm.23870
29. Cataldi M, Avoli M, de Villers-Sidani E. Resting state networks in temporal lobe epilepsy. Epilepsia. (2013) 54:2048–59. doi: 10.1111/epi.12400
30. Wei H, An J, Shen H, Zeng LL, Qiu S, Hu D. Altered effective connectivity among core neurocognitive networks in idiopathic generalized epilepsy: an fMRI evidence. Front Hum Neurosci. (2016) 10:447. doi: 10.3389/fnhum.2016.00447
31. Burianova H, Faizo NL, Gray M, Hocking J, Galloway G, Reutens D. Altered functional connectivity in mesial temporal lobe epilepsy. Epilepsy Res. (2017) 137:45–52. doi: 10.1016/j.eplepsyres.2017.09.001
32. Mulders PC, van Eijndhoven PF, Pluijmen J, Schene AH, Tendolkar I, Beckmann CF. Default mode network coherence in treatment-resistant major depressive disorder during electroconvulsive therapy. J Affect Disord. (2016) 205:130–7. doi: 10.1016/j.jad.2016.06.059
33. Wolf RC, Nolte HM, Hirjak D, Hofer S, Seidl U, Depping MS, et al. Structural network changes in patients with major depression and schizophrenia treated with electroconvulsive therapy. Eur Neuropsychopharmacol. (2016) 26:1465–74. doi: 10.1016/j.euroneuro.2016.06.008
34. Chen GD, Ji F, Li GY, Lyu BX, Hu W, Zhuo CJ. Antidepressant effects of electroconvulsive therapy unrelated to the brain's functional network connectivity alterations at an individual level. Chin Med J. (2017) 130:414–9. doi: 10.4103/0366-6999.199845
35. Raichle ME, MacLeod AM, Snyder AZ, Powers WJ, Gusnard DA, Shulman GL. A default mode of brain function. Proc Natl Acad Sci USA. (2001) 98:676–82. doi: 10.1073/pnas.98.2.676
36. Raichle ME, Snyder AZ. A default mode of brain function: a brief history of an evolving idea. Neuroimage. (2007) 37:1083–90; discussion 1097–89. doi: 10.1016/j.neuroimage.2007.02.041
37. Greicius MD, Supekar K, Menon V, Dougherty RF. Resting-state functional connectivity reflects structural connectivity in the default mode network. Cereb Cortex. (2009) 19:72–8. doi: 10.1093/cercor/bhn059
38. Mohan A, Roberto AJ, Mohan A, Lorenzo A, Jones K, Carney MJ, et al. The significance of the default mode network (DMN) in neurological and neuropsychiatric disorders: a review. Yale J Biol Med. (2016) 89:49–57.
39. Liang X, Zou Q, He Y, Yang Y. Topologically reorganized connectivity architecture of default-mode, executive-control, and salience networks across working memory task loads. Cereb Cortex. (2016) 26:1501–11. doi: 10.1093/cercor/bhu316
40. Sneve MH, Grydeland H, Amlien IK, Langnes E, Walhovd KB, Fjell AM. Decoupling of large-scale brain networks supports the consolidation of durable episodic memories. Neuroimage. (2017) 153:336–45. doi: 10.1016/j.neuroimage.2016.05.048
41. Ho TC, Connolly CG, Henje Blom E, LeWinn KZ, Strigo IA, Paulus MP, et al. Emotion-dependent functional connectivity of the default mode network in adolescent depression. Biol Psychiatry. (2015) 78:635–46. doi: 10.1016/j.biopsych.2014.09.002
42. Hu ML, Zong XF, Mann JJ, Zheng JJ, Liao YH, Li ZC, et al. A review of the functional and anatomical default mode network in schizophrenia. Neurosci Bull. (2017) 33:73–84. doi: 10.1007/s12264-016-0090-1
43. Alonso-Solis A, Vives-Gilabert Y, Grasa E, Portella MJ, Rabella M, Sauras RB, et al. Resting-state functional connectivity alterations in the default network of schizophrenia patients with persistent auditory verbal hallucinations. Schizophr Res. (2015) 161:261–8. doi: 10.1016/j.schres.2014.10.047
44. Lavigne KM, Menon M, Woodward TS. Functional brain networks underlying evidence integration and delusions in schizophrenia. Schizophr Bull. (2019). doi: 10.1093/schbul/sbz032. [Epub ahead of print].
45. Garrity AG, Pearlson GD, McKiernan K, Lloyd D, Kiehl KA, Calhoun VD. Aberrant “default mode” functional connectivity in schizophrenia. Am J Psychiatry. (2007) 164:450–7. doi: 10.1176/ajp.2007.164.3.450
46. Harrison BJ, Yucel M, Pujol J, Pantelis C. Task-induced deactivation of midline cortical regions in schizophrenia assessed with fMRI. Schizophr Res. (2007) 91:82–6. doi: 10.1016/j.schres.2006.12.027
47. Bluhm RL, Miller J, Lanius RA, Osuch EA, Boksman K, Neufeld RW, et al. Spontaneous low-frequency fluctuations in the BOLD signal in schizophrenic patients: anomalies in the default network. Schizophr Bull. (2007) 33:1004–12. doi: 10.1093/schbul/sbm052
48. Pomarol-Clotet E, Salvador R, Sarro S, Gomar J, Vila F, Martinez A, et al. Failure to deactivate in the prefrontal cortex in schizophrenia: dysfunction of the default mode network? Psychol Med. (2008) 38:1185–93. doi: 10.1017/s0033291708003565
49. Whitfield-Gabrieli S, Thermenos HW, Milanovic S, Tsuang MT, Faraone SV, McCarley RW, et al. Hyperactivity and hyperconnectivity of the default network in schizophrenia and in first-degree relatives of persons with schizophrenia. Proc Natl Acad Sci USA. (2009) 106:1279–84. doi: 10.1073/pnas.0809141106
50. Hahn B, Harvey AN, Gold JM, Ross TJ, Stein EA. Load-dependent hyperdeactivation of the default mode network in people with schizophrenia. Schizophr Res. (2017) 185:190–6. doi: 10.1016/j.schres.2017.01.001
51. Wang S, Zhan Y, Zhang Y, Lyu L, Lyu H, Wang G, et al. Abnormal long- and short-range functional connectivity in adolescent-onset schizophrenia patients: a resting-state fMRI study. Prog Neuropsychopharmacol Biol Psychiatry. (2018) 81:445–51. doi: 10.1016/j.pnpbp.2017.08.012
52. Camchong J, MacDonald AW III, Bell C, Mueller BA, Lim KO. Altered functional and anatomical connectivity in schizophrenia. Schizophr Bull. (2011) 37:640–50. doi: 10.1093/schbul/sbp131
53. Stretton J, Winston G, Sidhu M, Centeno M, Vollmar C, Bonelli S, et al. Neural correlates of working memory in Temporal Lobe Epilepsy–an fMRI study. Neuroimage. (2012) 60:1696–703. doi: 10.1016/j.neuroimage.2012.01.126
54. Zhang Z, Lu G, Zhong Y, Tan Q, Liao W, Wang Z, et al. Altered spontaneous neuronal activity of the default-mode network in mesial temporal lobe epilepsy. Brain Res. (2010) 1323:152–60. doi: 10.1016/j.brainres.2010.01.042
55. Stretton J, Pope RA, Winston GP, Sidhu MK, Symms M, Duncan JS, et al. Temporal lobe epilepsy and affective disorders: the role of the subgenual anterior cingulate cortex. J Neurol Neurosurg Psychiatry. (2015) 86:144–51. doi: 10.1136/jnnp-2013-306966
56. Pittau F, Grova C, Moeller F, Dubeau F, Gotman J. Patterns of altered functional connectivity in mesial temporal lobe epilepsy. Epilepsia. (2012) 53:1013–23. doi: 10.1111/j.1528-1167.2012.03464.x
57. Nakahara S, Adachi M, Ito H, Matsumoto M, Tajinda K, van Erp TGM. Hippocampal pathophysiology: commonality shared by temporal lobe epilepsy and psychiatric disorders. Neurosci J. (2018) 2018:4852359. doi: 10.1155/2018/4852359
58. Wang J, Tang Y, Curtin A, Xia M, Tang X, Zhao Y, et al. ECT-induced brain plasticity correlates with positive symptom improvement in schizophrenia by voxel-based morphometry analysis of grey matter. Brain Stimul. (2019) 12:319–28. doi: 10.1016/j.brs.2018.11.006
59. Kim HS, Kim SH, Lee NY, Youn T, Lee JH, Chung S, et al. Effectiveness of electroconvulsive therapy augmentation on clozapine-resistant schizophrenia. Psychiatry Investig. (2017) 14:58–62. doi: 10.4306/pi.2017.14.1.58
60. Sanghani SN, Petrides G, Kellner CH. Electroconvulsive therapy (ECT) in schizophrenia: a review of recent literature. Curr Opin Psychiatry. (2018) 31:213–22. doi: 10.1097/yco.0000000000000418
61. Vossel S, Geng JJ, Fink GR. Dorsal and ventral attention systems: distinct neural circuits but collaborative roles. Neuroscientist. (2014) 20:150–9. doi: 10.1177/1073858413494269
62. Jerde TA, Merriam EP, Riggall AC, Hedges JH, Curtis CE. Prioritized maps of space in human frontoparietal cortex. J Neurosci. (2012) 32:17382–90. doi: 10.1523/jneurosci.3810-12.2012
63. Ptak R. The frontoparietal attention network of the human brain: action, saliency, and a priority map of the environment. Neuroscientist. (2012) 18:502–15. doi: 10.1177/1073858411409051
64. Dixon ML, Andrews-Hanna JR, Spreng RN, Irving ZC, Mills C, Girn M, et al. Interactions between the default network and dorsal attention network vary across default subsystems, time, and cognitive states. Neuroimage. (2017) 147:632–49. doi: 10.1016/j.neuroimage.2016.12.073
65. Keedy SK, Reilly JL, Bishop JR, Weiden PJ, Sweeney JA. Impact of antipsychotic treatment on attention and motor learning systems in first-episode schizophrenia. Schizophr Bull. (2015) 41:355–65. doi: 10.1093/schbul/sbu071
66. Jimenez AM, Lee J, Green MF, Wynn JK. Functional connectivity when detecting rare visual targets in schizophrenia. Psychiatry Res Neuroimaging. (2017) 261:35–43. doi: 10.1016/j.pscychresns.2017.01.007
67. Zhou Y, Zeidman P, Wu S, Razi A, Chen C, Yang L, et al. Altered intrinsic and extrinsic connectivity in schizophrenia. Neuroimage Clin. (2018) 17:704–16. doi: 10.1016/j.nicl.2017.12.006
68. Woodward TS, Leong K, Sanford N, Tipper CM, Lavigne KM. Altered balance of functional brain networks in Schizophrenia. Psychiatry Res Neuroimaging. (2016) 248:94–104. doi: 10.1016/j.pscychresns.2016.01.003
69. Godwin D, Ji A, Kandala S, Mamah D. Functional connectivity of cognitive brain networks in schizophrenia during a working memory task. Front Psychiatry. (2017) 8:294. doi: 10.3389/fpsyt.2017.00294
70. Woodward ND, Rogers B, Heckers S. Functional resting-state networks are differentially affected in schizophrenia. Schizophr Res. (2011) 130:86–93. doi: 10.1016/j.schres.2011.03.010
71. Zhang Z, Lu G, Zhong Y, Tan Q, Yang Z, Liao W, et al. Impaired attention network in temporal lobe epilepsy: a resting FMRI study. Neurosci Lett. (2009) 458:97–101. doi: 10.1016/j.neulet.2009.04.040
72. Jiang LW, Qian RB, Fu XM, Zhang D, Peng N, Niu CS, et al. Altered attention networks and DMN in refractory epilepsy: a resting-state functional and causal connectivity study. Epilepsy Behav. (2018) 88:81–6. doi: 10.1016/j.yebeh.2018.06.045
73. Wang J, Wei Q, Wang L, Zhang H, Bai T, Cheng L, et al. Functional reorganization of intra- and internetwork connectivity in major depressive disorder after electroconvulsive therapy. Hum Brain Mapp. (2018) 39:1403–11. doi: 10.1002/hbm.23928
74. Seeley WW, Menon V, Schatzberg AF, Keller J, Glover GH, Kenna H, et al. Dissociable intrinsic connectivity networks for salience processing and executive control. J Neurosci. (2007) 27:2349–56. doi: 10.1523/jneurosci.5587-06.2007
75. Sherman LE, Rudie JD, Pfeifer JH, Masten CL, McNealy K, Dapretto M. Development of the default mode and central executive networks across early adolescence: a longitudinal study. Dev Cogn Neurosci. (2014) 10:148–59. doi: 10.1016/j.dcn.2014.08.002
76. Langeslag SJ, Schmidt M, Ghassabian A, Jaddoe VW, Hofman A, van der Lugt A, et al. Functional connectivity between parietal and frontal brain regions and intelligence in young children: the Generation R study. Hum Brain Mapp. (2013) 34:3299–307. doi: 10.1002/hbm.22143
77. Chen AC, Oathes DJ, Chang C, Bradley T, Zhou ZW, Williams LM, et al. Causal interactions between fronto-parietal central executive and default-mode networks in humans. Proc Natl Acad Sci USA. (2013) 110:19944–9. doi: 10.1073/pnas.1311772110
78. Kang Y, Huang K, Cai S, Wang H, Liu J, Wang Y, et al. Association between function and structure of the triple network and catechol-O-methyltransferase val(158)met polymorphism in the first episode schizophrenia. Neurosci Lett. (2018) 687:65–70. doi: 10.1016/j.neulet.2018.09.033
79. Palaniyappan L, White TP, Liddle PF. The concept of salience network dysfunction in schizophrenia: from neuroimaging observations to therapeutic opportunities. Curr Top Med Chem. (2012) 12:2324–38. doi: 10.2174/156802612805289881
80. Lamichhane B, Dhamala M. The salience network and its functional architecture in a perceptual decision: an effective connectivity study. Brain Connect. (2015) 5:362–70. doi: 10.1089/brain.2014.0282
81. Lamichhane B, Adhikari BM, Dhamala M. Salience network activity in perceptual decisions. Brain Connect. (2016) 6:558–71. doi: 10.1089/brain.2015.0392
82. Barrett LF, Satpute AB. Large-scale brain networks in affective and social neuroscience: towards an integrative functional architecture of the brain. Curr Opin Neurobiol. (2013) 23:361–72. doi: 10.1016/j.conb.2012.12.012
83. Chand GB, Dhamala M. Interactions among the brain default-mode, salience, and central-executive networks during perceptual decision-making of moving dots. Brain Connect. (2016) 6:249–54. doi: 10.1089/brain.2015.0379
84. Fan J, Zhong M, Gan J, Liu W, Niu C, Liao H, et al. Altered connectivity within and between the default mode, central executive, and salience networks in obsessive-compulsive disorder. J Affect Disord. (2017) 223:106–14. doi: 10.1016/j.jad.2017.07.041
85. Liu Y, Li L, Li B, Feng N, Li L, Zhang X, et al. Decreased triple network connectivity in patients with recent onset post-traumatic stress disorder after a single prolonged trauma exposure. Sci Rep. (2017) 7:12625. doi: 10.1038/s41598-017-12964-6
86. Palaniyappan L, Liddle PF. Does the salience network play a cardinal role in psychosis? An emerging hypothesis of insular dysfunction. J Psychiatry Neurosci. (2012) 37:17–27. doi: 10.1503/jpn.100176
87. Orliac F, Naveau M, Joliot M, Delcroix N, Razafimandimby A, Brazo P, et al. Links among resting-state default-mode network, salience network, and symptomatology in schizophrenia. Schizophr Res. (2013) 148:74–80. doi: 10.1016/j.schres.2013.05.007
88. Mallikarjun PK, Lalousis PA, Dunne TF, Heinze K, Reniers RL, Broome MR, et al. Aberrant salience network functional connectivity in auditory verbal hallucinations: a first episode psychosis sample. Transl Psychiatry. (2018) 8:69. doi: 10.1038/s41398-018-0118-6
89. White TP, Gilleen J, Shergill SS. Dysregulated but not decreased salience network activity in schizophrenia. Front Hum Neurosci. (2013) 7:65. doi: 10.3389/fnhum.2013.00065
90. Hare SM, Ford JM, Mathalon DH, Damaraju E, Bustillo J, Belger A, et al. Salience-default mode functional network connectivity linked to positive and negative symptoms of schizophrenia. Schizophr Bull. (2018) 45:892–901. doi: 10.1093/schbul/sby112
91. Chen Q, Chen X, He X, Wang L, Wang K, Qiu B. Aberrant structural and functional connectivity in the salience network and central executive network circuit in schizophrenia. Neurosci Lett. (2016) 627:178–84. doi: 10.1016/j.neulet.2016.05.035
92. Jiang Y, Duan M, Chen X, Chang X, He H, Li Y, et al. Common and distinct dysfunctional patterns contribute to triple network model in schizophrenia and depression: a preliminary study. Prog Neuropsychopharmacol Biol Psychiatry. (2017) 79(Pt B):302–10. doi: 10.1016/j.pnpbp.2017.07.007
93. Supekar K, Cai W, Krishnadas R, Palaniyappan L, Menon V. Dysregulated brain dynamics in a triple-network saliency model of schizophrenia and its relation to psychosis. Biol Psychiatry. (2019) 85:60–9. doi: 10.1016/j.biopsych.2018.07.020
94. Bullmore E, Sporns O. Complex brain networks: graph theoretical analysis of structural and functional systems. Nat Rev Neurosci. (2009) 10:186–98. doi: 10.1038/nrn2575
95. Chiang S, Haneef Z. Graph theory findings in the pathophysiology of temporal lobe epilepsy. Clin Neurophysiol. (2014) 125:1295–305. doi: 10.1016/j.clinph.2014.04.004
96. Nelson BG, Bassett DS, Camchong J, Bullmore ET, Lim KO. Comparison of large-scale human brain functional and anatomical networks in schizophrenia. Neuroimage Clin. (2017) 15:439–48. doi: 10.1016/j.nicl.2017.05.007
97. Stam CJ, Reijneveld JC. Graph theoretical analysis of complex networks in the brain. Nonlinear Biomed Phys. (2007) 1:3. doi: 10.1186/1753-4631-1-3
98. Rubinov M, Knock SA, Stam CJ, Micheloyannis S, Harris AW, Williams LM, et al. Small-world properties of nonlinear brain activity in schizophrenia. Hum Brain Mapp. (2009) 30:403–16. doi: 10.1002/hbm.20517
99. Liu Y, Liang M, Zhou Y, He Y, Hao Y, Song M, et al. Disrupted small-world networks in schizophrenia. Brain. (2008) 131(Pt 4):945–61. doi: 10.1093/brain/awn018
100. Lo CY, Su TW, Huang CC, Hung CC, Chen WL, Lan TH, et al. Randomization and resilience of brain functional networks as systems-level endophenotypes of schizophrenia. Proc Natl Acad Sci USA. (2015) 112:9123–8. doi: 10.1073/pnas.1502052112
101. Erdeniz B, Serin E, Ibadi Y, Tas C. Decreased functional connectivity in schizophrenia: the relationship between social functioning, social cognition and graph theoretical network measures. Psychiatry Res Neuroimaging. (2017) 270:22–31. doi: 10.1016/j.pscychresns.2017.09.011
102. Bernhardt BC, Chen Z, He Y, Evans AC, Bernasconi N. Graph-theoretical analysis reveals disrupted small-world organization of cortical thickness correlation networks in temporal lobe epilepsy. Cereb Cortex. (2011) 21:2147–57. doi: 10.1093/cercor/bhq291
103. Bernhardt BC, Bonilha L, Gross DW. Network analysis for a network disorder: the emerging role of graph theory in the study of epilepsy. Epilepsy Behav. (2015) 50:162–70. doi: 10.1016/j.yebeh.2015.06.005
104. Bernhardt BC, Bernasconi N, Hong SJ, Dery S, Bernasconi A. Subregional mesiotemporal network topology is altered in temporal lobe epilepsy. Cereb Cortex. (2016) 26:3237–48. doi: 10.1093/cercor/bhv166
Keywords: electroconvulsive therapy (ECT), schizophrenia, temporal lobe epilepsy (TLE), brain networks, graph theory
Citation: Li Q, Liu S, Guo M, Yang C-X and Xu Y (2019) The Principles of Electroconvulsive Therapy Based on Correlations of Schizophrenia and Epilepsy: A View From Brain Networks. Front. Neurol. 10:688. doi: 10.3389/fneur.2019.00688
Received: 15 March 2019; Accepted: 13 June 2019;
Published: 27 June 2019.
Edited by:
Nicoletta Cera, University of Porto, PortugalReviewed by:
Christopher C. Abbott, University of New Mexico Health Science Center, United StatesZhi-De Deng, National Institute of Mental Health (NIMH), United States
Copyright © 2019 Li, Liu, Guo, Yang and Xu. This is an open-access article distributed under the terms of the Creative Commons Attribution License (CC BY). The use, distribution or reproduction in other forums is permitted, provided the original author(s) and the copyright owner(s) are credited and that the original publication in this journal is cited, in accordance with accepted academic practice. No use, distribution or reproduction is permitted which does not comply with these terms.
*Correspondence: Yong Xu, eHV5b25nc211JiN4MDAwNDA7dmlwLjE2My5jb20=
†These authors have contributed equally to this work