- 1Collaborative Innovation Center for Birth Defect Research and Transformation of Shandong Province, Jining Medical University, Jining, China
- 2Shandong Collaborative Innovation Center for Diagnosis, Treatment and Behavioral Interventions of Mental Disorders, Institute of Mental Health, Jining Medical University, Jining, China
- 3Shandong Key Laboratory of Behavioral Medicine, School of Mental Health, Jining Medical University, Jining, China
Stroke is one of the leading causes of death and disability worldwide. Age is associated with increased risk of stroke, while telomere length shortening plays a pivotal role in the process of aging. Moreover, telomere length shortening is associated with many risk factors of stroke in addition to age. Accumulated evidence shows that short leukocyte telomere length is not only associated with stroke occurrence but also associated with post-stroke recovery in the elderly population. In this review, we aimed to summarize the association between leukocyte telomere length and stroke, and discuss that telomere length might serve as a potential biomarker to predict the risk and prognosis of stroke.
Introduction
Stroke is an acute cerebrovascular disease caused by the disruption of blood supply. As the second leading cause of death and the third leading cause of disability worldwide (1), stroke brings heavy burden to both family and health care system. The incidence of stroke is increased with age and ~65% of stroke cases occur in individuals older than 65 years. In addition to age per se, various risk factors of stroke are age-related, including hypertension, atherosclerosis, atrial fibrillation, high cholesterol, and diabetes. Telomere length (TL) shortening is associated with age and many other risk factors of stroke. Consistently, a number of studies indicated that stroke occurrence is associated with short leukocyte telomere length (LTL) (2–4). Moreover, accumulated evidence indicates that short LTL is also associated with the post-stroke recovery. Therefore, we aimed to summarize the association between LTL and stroke, and discuss the potential of TL as a biomarker to predict the risk and prognosis of stroke.
TL Maintenance
Telomeres
Human telomeres are specialized structure of DNA-protein complex, capping the end of linear chromosomes. Human telomeric DNA consists of a variable number of tandem repeats of double-stranded TTAGGG and a 3′ G-rich single-stranded overhang, known as the G-tail (5). The length of double-stranded repeats ranges from 2 to 30 kb, while the length of 3′ overhang is around 150 nt. The invasion of 3′ G-tail into the double-stranded region forms a high order structure and a triple-stranded structure, called T-(telomere) loop and D-(displacement) loop, respectively (6) (Figure 1A). Shelterin complex, the protein components of telomere, includes six subunits, telomeric repeat binding factor 1 (TERF1), telomeric repeat binding factor 2 (TERF2), TERF2 interacting protein (TERF2IP), TERF1-interacting nuclear factor 2 (TINF2), tripeptidyl peptidase I (TPP1), and protection of telomeres 1 (POT1) (Figure 1B). TERF1/TERF2 and POT1 bind directly to double-stranded and single-stranded telomeric DNA, respectively (Figure 1B). TERF2IP, the partner of TERF2, interacts solely with TERF2, while TINF2 connects TERF1, and TERF2. POT1 is tethered to TERF1 and TERF2 via an interaction between TPP1 and TINF2 (7). Telomeric DNA along with the shelterin protein complex maintains the specialized telomere structure and protects the ends of chromosomes from degradation, fusion, and recombination for maintaining genomic integrity (8). Moreover, mammalian CTC1-STN1-TEN1 (CST) complex does localize to telomeres, which resembles the Cdc13-Stn1-Ten1 complex from Saccharomyces cerevisiae in that the STN1 and TEN1 subunits are conserved. CST complex binds to single strand DNA in a non-sequence specific manner and plays a key role in telomere replication (9–11). In addition, various DNA repair proteins contribute to telomere maintenance including TL maintenance via interacting with telomere proteins or modifying telomere proteins, including Polβ, FEN1, APE1, XPF-ERCC1 complex, and MRN complex which are involved in base excision repair pathway, nucleotide excision repair pathway and double strand break repair pathway, respectively (12–15).
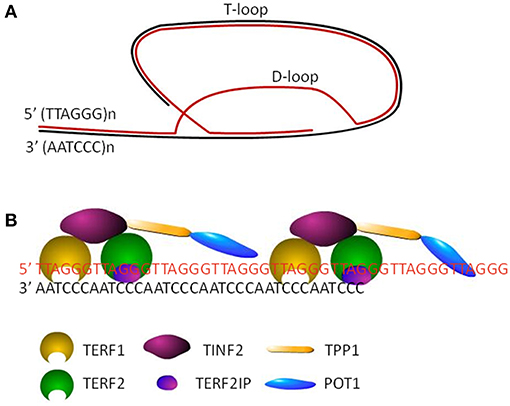
Figure 1. The structure of telomeres. (A) Cap structure of telomeres consists of a T-loop and a D-loop. (B) The shelterin complex, including TERF1, TERF2, TINF2, TERF2IP, TPP1, and POT1, maintains the stability of loop structures by binding to the telomeric DNA.
Telomerase
Inability of DNA polymerase complex to replicate the 3′-end of the lagging strand in linear chromosomes leads to telomere shortening by each cycle of DNA replication during cell division, so-called end replication problem. As the number of cell division increases, TL is gradually shortened. When TL is reduced to the critical length, cells stop dividing and may enter cellular senescence or apoptosis (16). To counter this issue, human telomerase is responsible for synthesizing telomeric DNA to compensate the erosion of TL during DNA replication. Telomerase is a unique ribonucleoprotein enzymatic complex consisting of a RNA component and a protein component, telomerase RNA component (TERC), and telomerase reverse transcriptase (TERT). TERC is a non-coding RNA which is essential for telomere synthesis by serving as a template for the elongation of telomere 3′ overhang of the telomeric G-rich strand, while TERT catalyzes the process by its reverse transcriptase activity (17). In mammalian, telomerase is active only in germ cells, some types of stem cells and cancer cells, while most human somatic cells lack telomerase activity. Moreover, the activity of telomerase is regulated by the shelterin complex, CST complex, and telomeric repeat containing RNA (TERRA) which is transcribed from telomeric DNA. For example, shelterin complex control telomerase-dependent telomere elongation through a TERF1-mediated negative regulatory pathway, i.e., the more TERF1 is binding to telomeres, the less telomerase accesses to the ends of telomeres leading to less telomere elongation (13, 18, 19). In addition, the phosphoryaltion and PARylation of TERF1 promotes the release of TERF1 from telomeres contributing to telomere lengthening (13, 20, 21). Recently, the structure of telomerase with telomeric DNA has been resolved, which will promote the understanding the function of telomerase (22).
TL and Stroke
TL maintenance is critical for human health as telomere shortening is associated with aging and various age-related diseases. Leukocyte telomere length (LTL) is served as a marker in a number of association studies of aging and age-related diseases (3, 23–26). The alteration of LTL has been observed in patients with stroke, which is one of the most common age-related diseases leading to death and disability. The positive association between short LTL and the risk of stroke was reported in the case-control studies from China, while the short LTL was also associated with post-stroke death (2, 25, 27, 28). In a 10-year prospective study from the United States, the positive association between short LTL and increased risk of stroke was only existed in the population ranging from 65 to 73 years old, but not in the population beyond 73 years old (29). A study from Europe showed that short LTL was associated with cerebrovascular accidents in the hypertensive patients with left ventricular hypertrophy aged 55–80 years (30). Moreover, short LTL was associated with higher mortality rate in patients with cardiovascular diseases aged 60 years or older (31). However, two nested case-control studies from the United States have not found the positive association between LTL and stroke (32, 33). In addition, no association between short LTL and stroke was found in a 29-year cohort study from Denmark (34). It has to be noted that the participants aged 30–55, 40–84, and 30–60 years, respectively, in the three studies when the blood samples were collected. It highly suggested that the positive association between short LTL and the risk of stroke or post-stroke death might only exist in the aged population although further validation is necessary. Recently, two meta-analysis have been performed to investigate the association between TL and stroke. First, a meta-analysis including 11 studies showed that shortened TL is significantly associated with stroke, even though subgroup analysis showed equivocal results for both prospective (n = 7, p = 0.051) and retrospective (n = 4, p = 0.067) studies (35). The high heterogeneity, particularly age difference, and reduced sample size might contribute the equivocal results of the subgroup analysis. Another meta-analysis based on causal inference approaches using TL-related SNPs as instrumental variables did not support the causal effect of shorter TL on ischemic stroke (36). It has to be noted that age, one of the most important covariates, was not considered in this study. As previous studies do indicate that the positive association between short LTL and the risk of stroke or post-stroke death might only exist in the aged population, the effect of age might need to be taken into consideration in future studies. Currently, although mechanisms of the positive association between short LTL and stroke in the elderly remain elusive, multiple signaling pathways may contribute the positive association, e.g., apelin-apelin receptor mediated signaling pathway, histone deacetylases mediated epigenetic regulation (37, 38).
Risk Factors of Stroke and TL
Age
Age is one of the major risk factors of stroke (39). The incidence of stroke is increased with age, and the mean age at first-ever stroke was more than 68 years (40). TL is considered as a marker of the biological aging process because it shortens with each cell division. The estimated shortening rate is about 25–27 base pairs per year with individual difference (23, 41, 42). However, the actual LTL shortening rate is about 31–72 base pairs per year (23, 43). It suggests that many factors may contribute to LTL shortening in addition to end replication problem. For example, oxidative stress-induced oxidative DNA damage could lead to telomere uncapping and subsequent telomere shortening, while telomere shortening further enhances oxidative stress forming a vicious cycle (44–47). Age-related increase of oxidative damage is observed in human (48, 49). Moreover, increased evidence suggests that reduction of mitochondria function contributes to the aging process (50). Dysfunction of mitochondria contributes to age-related increase of oxidative stress, which in turn leads to TL shortening (51). Furthermore, multiple molecules (e.g., apelin, HDAC4, RCAN1, α-synuclein), altered in stroke are implicated in aging process, premature disorders (e.g., Down syndrome, Werner syndrome) and age-related disorders (e.g., Alzheimer's disease, Parkinson's disease, Huntington's disease) (37, 38, 52–66). Thus, age-related physiological or pathological alterations may also contribute to TL shortening.
Genetic Factors
Growing evidence indicates that genetic factors play a major role in stroke and family history of stroke is associated with increased stroke risk (67–69). Genomewide association study (GWAS) showed that the heritability for all ischemic stroke was 37.9%, while the heritability varied markedly in different subtypes of stroke, 32.6% for cardioembolic stroke, 40.3% for large-vessel stroke and 16.1% for small-vessel stroke (70). It was also reported that genetic factors appeared to be more important in large-vessel and small-vessel strokes than in cardioembolic stroke (71). Moreover, various genetic variants associated with short telomeres also contribute to the risk of stroke. For example, genetic variants of ACYP2 and TSPYL6 were associated with shorter telomere, which did contribute to the increased risk of stroke (72, 73).
Obesity
Obesity is associated with elevated risk of stroke. A number of studies showed that obesity is consistently associated with increased inflammation and oxidative stress, which promotes telomere shortening (74–80). Valdes et al. found that LTL of obese women was 240 bp shorter than that of age-matched lean women (41). A couple of cross-sectional studies showed a negative correlation between LTL and obesity (81, 82), whereas others did not show significant associations between LTL and obesity (83, 84). A recent meta-analysis showed there is a tendency toward demonstrating negative correlation between obesity and TL. Although 39 of 63 studies showed either weak or moderate correlation between obesity and TL, heterogeneity among the selected studies made the relationship of obesity and TL still open (85).
Smoking and Alcohol Intake
Smoking is a well-established risk factor for all forms of stroke (86–88). Even passive smoking significantly increases the risk of stroke (89–91). Meta-analysis including 30 studies showed that shorter LTL was among ever smokers compared to never smokers, while the dosage of smoking was negatively associated with LTL (92). Moreover, Valdes et al. showed that each pack-year smoked caused an additional loss of 5 base pairs of LTL per year (41). Babizhayev et al. reported that telomere attrition can serve as a biomarker of the oxidative stress and inflammation induced by tobacco smoking (93). In addition, heavy alcohol consumption was strongly associated with a reduced LTL in participants aged ≥65 years (94).
Psychological Stress and Depression
Numerous studies demonstrated that psychological stress caused by negative life event, work stress, illness etc. has significant effect on the risk of stroke (95–98). Accelerated LTL shortening was associated with psychological stress, which might be caused by psychological stress-induced higher oxidative stress and lower telomerase activity (99, 100). In addition, depression is a strong risk factor for stroke, while long-term stress could lead to depression (101, 102). Although the association between LTL and depression was inconsistent, two meta-analyses showed that short LTL was associated with depression (103, 104).
Age-Related Diseases
Hypertension
Hypertension is one of the most important modifiable risk factors for stroke. The strong, direct, linear, and continuous association between increased blood pressure and stroke risk was observed, while intensive blood pressure lowering appears to be beneficial for the reduction of stroke incidence (105–109). Two studies showed that LTL was negatively associated with pulse pressure and hypertension (110, 111). Importantly, meta-analyses from 3,097 participants showed that LTL of hypertensive patients was significantly shorter than that of controls (112).
Diabetes
Diabetes is an independent risk factor for stroke with a 2-fold increased risk in stroke for diabetic patients (113). Compared with control individuals, TL is significantly shorter in patients with type 1 diabetes or type 2 diabetes (114–121). For example, LTL was significantly shorter in type 2 diabetes cases compared with controls over a wide age range in a retrospective case-control study comprising 4,016 subjects (121). Moreover, the escalated telomere attrition was associated with insulin resistance in young adults aged from 21.0 to 43.5 years, which might be caused by increased oxidative stress, metabolic and inflammatory factors (122). In addition, the inverse association between LTL and body mass index (BMI)was observed (120).
Cardiovascular Diseases
Short LTL are associated with various cardiovascular diseases (CVD) including atherosclerosis, myocardial infarction, coronary artery disease (CAD) and atrial fibrillation (AF) (123–129). For example, short TL was associated with severe CAD adjusting for age and sex (123). A recent systematic review and meta-analysis from 43,725 participants and 8,400 patients suggested that LTL was inversely associated with the risk of coronary heart disease independent of conventional vascular risk factors (26). LTL was shorter in patients with myocardial infarction than that in age-matched control individuals (124). In a prospective study, Chen et al. examined LTL at baseline to predict the incidence and progression of carotid atherosclerosis (130). Compared to participants in the highest LTL tertile, those in the lowest tertile had significantly elevated risk for both incident plaque and plaque progression (130). A recent study indicated that telomere shortening may constitute a novel factor for cardioembolic stroke in patients with AF (129).
Although the underlying mechanism of the relationship between short LTL and CVD remains elusive, booming studies have provided a number of hypotheses (131–133). First, genomic evidence showed that inheritance of shorter telomere might act as a cause of CVD. Genome-wide association studies (GWAS) identified a small set of loci, including SNPs within TERT and TERC, but all with very small R2 values (<0.5%) (72, 134). Moreover, it has been reported that cardiovascular risk-related systemic oxidative stress and inflammation might accelerate telomere attrition (135). In addition, the attrition-rate hypothesis implies that variation in adult telomere attrition rates might be more important than TL inheritance (136), indicating that telomere attrition rate could serve as a clinical atherosclerosis CVD biomarker. However, a smaller longitudinal study did not show and so on an association between leukocyte telomere attrition rate and atherosclerosis (137).
Conclusions
Short LTL is positively associated with stroke in the elderly population. In addition, multiple risk factors of stroke are associated with shortened LTL, e.g., age, smoking, alcohol intake, psychological stress, while the possibility of short LTL as an independent risk indicator of stroke still remains. It indicates that LTL might serve as a potential biomarker to evaluate the risk of stroke in the elderly. Moreover, short LTL was also positively associated with the mortality of stroke and cardiovascular diseases, indicating that LTL might be a potential biomarker to predict the prognosis of stroke.
Author Contributions
YT, SW, FJ, QK, and CL wrote the manuscript. YW formulated and revised the manuscript.
Funding
This work was supported by grants from National Natural Science Foundation of China (81771147), Natural Science Foundation of Shandong Province of China (ZR2016HM30, ZR2016HM55), Medical and Health Science and Technology Planning Project of Shandong Province of China (2018WS463), Supporting Fund for Teachers' Research of Jining Medical University (JY2017KJ039, JY2017JS006, JY2017JS003), the Startup Fund from Jining Medical University (to FJ).
Conflict of Interest Statement
The authors declare that the research was conducted in the absence of any commercial or financial relationships that could be construed as a potential conflict of interest.
References
1. Donnan GA. International journal of stroke. Editorial. Int J Stroke. (2008) 3:157. doi: 10.1111/j.1747-4949.2008.00208.x
2. Ding H, Chen C, Shaffer JR, Liu L, Xu Y, Wang X, et al. Telomere length and risk of stroke in Chinese. Stroke. (2012) 43:658–63. doi: 10.1161/STROKEAHA.111.637207
3. D'mello MJ, Ross SA, Briel M, Anand SS, Gerstein H, Pare G. Association between shortened leukocyte telomere length and cardiometabolic outcomes: systematic review and meta-analysis. Circ Cardiovasc Genet. (2015) 8:82–90. doi: 10.1161/CIRCGENETICS.113.000485
4. Hamad R, Walter S, Rehkopf DH. Telomere length and health outcomes: a two-sample genetic instrumental variables analysis. Exp Gerontol. (2016) 82:88–94. doi: 10.1016/j.exger.2016.06.005
5. Blackburn EH, Epel ES, Lin J. Human telomere biology: a contributory and interactive factor in aging, disease risks, and protection. Science. (2015) 350:1193–8. doi: 10.1126/science.aab3389
6. Griffith JD, Comeau L, Rosenfield S, Stansel RM, Bianchi A, Moss H, et al. Mammalian telomeres end in a large duplex loop. Cell. (1999) 97:503–14. doi: 10.1016/S0092-8674(00)80760-6
8. Lu W, Zhang Y, Liu D, Songyang Z, Wan M. Telomeres-structure, function, and regulation. Exp Cell Res. (2013) 319:133–41. doi: 10.1016/j.yexcr.2012.09.005
9. Miyake Y, Nakamura M, Nabetani A, Shimamura S, Tamura M, Yonehara S, et al. RPA-like mammalian Ctc1-Stn1-Ten1 complex binds to single-stranded DNA and protects telomeres independently of the Pot1 pathway. Mol Cell. (2009) 36:193–206. doi: 10.1016/j.molcel.2009.08.009
10. Surovtseva YV, Churikov D, Boltz KA, Song X, Lamb JC, Warrington R, et al. Conserved telomere maintenance component 1 interacts with STN1 and maintains chromosome ends in higher eukaryotes. Mol Cell. (2009) 36:207–18. doi: 10.1016/j.molcel.2009.09.017
11. Stewart JA, Wang F, Chaiken MF, Kasbek C, Chastain PD, Wright WE, et al. Human CST promotes telomere duplex replication and general replication restart after fork stalling. EMBO J. (2012) 31:3537–49. doi: 10.1038/emboj.2012.215
12. Muftuoglu M, Wong HK, Imam SZ, Wilson DM III, Bohr VA, Opresko PL. Telomere repeat binding factor 2 interacts with base excision repair proteins and stimulates DNA synthesis by DNA polymerase beta. Cancer Res. (2006) 66:113–24. doi: 10.1158/0008-5472.CAN-05-2742
13. Wu Y, Xiao S, Zhu XD. MRE11-RAD50-NBS1 and ATM function as co-mediators of TRF1 in telomere length control. Nat Struct Mol Biol. (2007) 14:832–40. doi: 10.1038/nsmb1286
14. Wu Y, Mitchell TR, Zhu XD. Human XPF controls TRF2 and telomere length maintenance through distinctive mechanisms. Mech Ageing Dev. (2008) 129:602–10. doi: 10.1016/j.mad.2008.08.004
15. Miller AS, Balakrishnan L, Buncher NA, Opresko PL, Bambara RA. Telomere proteins POT1, TRF1 and TRF2 augment long-patch base excision repair in vitro. Cell Cycle. (2012) 11:998–1007. doi: 10.4161/cc.11.5.19483
16. Collado M, Blasco MA, Serrano M. Cellular senescence in cancer and aging. Cell. (2007) 130:223–33. doi: 10.1016/j.cell.2007.07.003
17. Greider CW, Blackburn EH. A telomeric sequence in the RNA of Tetrahymena telomerase required for telomere repeat synthesis. Nature. (1989) 337:331–7. doi: 10.1038/337331a0
18. Van Steensel B, De Lange T. Control of telomere length by the human telomeric protein TRF1. Nature. (1997) 385:740–3. doi: 10.1038/385740a0
19. Ancelin K, Brunori M, Bauwens S, Koering CE, Brun C, Ricoul M, et al. Targeting assay to study the cis functions of human telomeric proteins: evidence for inhibition of telomerase by TRF1 and for activation of telomere degradation by TRF2. Mol Cell Biol. (2002) 22:3474–87. doi: 10.1128/MCB.22.10.3474-3487.2002
20. Ye JZ, De Lange T. TIN2 is a tankyrase 1 PARP modulator in the TRF1 telomere length control complex. Nat Genet. (2004) 36:618–23. doi: 10.1038/ng1360
21. Li B, Qiao R, Wang Z, Zhou W, Li X, Xu W, et al. Crystal structure of a tankyrase 1-telomere repeat factor 1 complex. Acta Crystallogr F Struct Biol Commun. (2016) 72:320–7. doi: 10.1107/S2053230X16004131
22. Jiang J, Wang Y, Susac L, Chan H, Basu R, Zhou ZH, et al. Structure of Telomerase with Telomeric DNA. Cell. (2018) 173:1179–90 e1113. doi: 10.1016/j.cell.2018.04.038
23. Muezzinler A, Zaineddin AK, Brenner H. A systematic review of leukocyte telomere length and age in adults. Ageing Res Rev. (2013) 12:509–19. doi: 10.1016/j.arr.2013.01.003
24. Sanders JL, Newman AB. Telomere length in epidemiology: a biomarker of aging, age-related disease, both, or neither? Epidemiol Rev. (2013) 35:112–31. doi: 10.1093/epirev/mxs008
25. Zhang W, Chen Y, Wang Y, Liu P, Zhang M, Zhang C, et al. Short telomere length in blood leucocytes contributes to the presence of atherothrombotic stroke and haemorrhagic stroke and risk of post-stroke death. Clin Sci. (2013) 125:27–36. doi: 10.1042/CS20120691
26. Haycock PC, Heydon EE, Kaptoge S, Butterworth AS, Thompson A, Willeit P. Leucocyte telomere length and risk of cardiovascular disease: systematic review and meta-analysis. BMJ. (2014) 349:g4227. doi: 10.1136/bmj.g4227
27. Jiang X, Dong M, Cheng J, Huang S, He Y, Ma K, et al. Decreased leukocyte telomere length (LTL) is associated with stroke but unlikely to be causative. PLoS ONE. (2013) 8:e68254. doi: 10.1371/journal.pone.0068254
28. Li J, Feng C, Li L, Yang S, Chen Y, Hui R, et al. The association of telomere attrition with first-onset stroke in Southern Chinese: a case-control study and meta-analysis. Sci Rep. (2018) 8:2290. doi: 10.1038/s41598-018-20434-w
29. Fitzpatrick AL, Kronmal RA, Gardner JP, Psaty BM, Jenny NS, Tracy RP, et al. Leukocyte telomere length and cardiovascular disease in the cardiovascular health study. Am J Epidemiol. (2007) 165:14–21. doi: 10.1093/aje/kwj346
30. Fyhrquist F, Silventoinen K, Saijonmaa O, Kontula K, Devereux RB, De Faire U, et al. Telomere length and cardiovascular risk in hypertensive patients with left ventricular hypertrophy: the LIFE study. J Hum Hypertens. (2011) 25:711–8. doi: 10.1038/jhh.2011.57
31. Cawthon RM, Smith KR, O'brien E, Sivatchenko A, Kerber RA. Association between telomere length in blood and mortality in people aged 60 years or older. Lancet. (2003) 361:393–5. doi: 10.1016/S0140-6736(03)12384-7
32. Zee RY, Castonguay AJ, Barton NS, Ridker PM. Relative leukocyte telomere length and risk of incident ischemic stroke in men: a prospective, nested case-control approach. Rejuvenation Res. (2010b) 13:411–4. doi: 10.1089/rej.2009.0975
33. Schurks M, Prescott J, Dushkes R, De Vivo I, Rexrode KM. Telomere length and ischaemic stroke in women: a nested case-control study. Eur J Neurol. (2013) 20:1068–74. doi: 10.1111/ene.12135
34. Ellehoj H, Bendix L, Osler M. Leucocyte telomere length and risk of cardiovascular disease in a cohort of 1,397 danish men and women. Cardiology. (2016) 133:173–7. doi: 10.1159/000441819
35. Jin X, Pan B, Dang X, Wu H, Xu D. Relationship between short telomere length and stroke: a meta-analysis. Medicine. (2018) 97:e12489. doi: 10.1097/MD.0000000000012489
36. Cao W, Li X, Zhang X, Zhang J, Sun Q, Xu X, et al. No causal effect of telomere length on ischemic stroke and its subtypes: a mendelian randomization study. Cells. (2019) 8:159. doi: 10.3390/cells8020159
37. Wu Y, Wang X, Zhou X, Cheng B, Li G, Bai B. Temporal expression of apelin/apelin receptor in ischemic stroke and its therapeutic potential. Front Mol Neurosci/. (2017) 10:1. doi: 10.3389/fnmol.2017.00001
38. Kong Q, Hao Y, Li X, Wang X, Ji B, Wu Y. HDAC4 in ischemic stroke: mechanisms and therapeutic potential. Clin Epigenet. (2018) 10:117. doi: 10.1186/s13148-018-0549-1
39. Benjamin EJ, Virani SS, Callaway CW, Chamberlain AM, Chang AR, Cheng S, et al. Heart disease and stroke statistics-2018 update: a report from the american heart association. Circulation. (2018) 137, e67–e492. doi: 10.1161/CIR.0000000000000558
40. Appelros P, Stegmayr B, Terent A. Sex differences in stroke epidemiology: a systematic review. Stroke. (2009) 40:1082–90. doi: 10.1161/STROKEAHA.108.540781
41. Valdes AM, Andrew T, Gardner JP, Kimura M, Oelsner E, Cherkas LF, et al. Obesity, cigarette smoking, and telomere length in women. Lancet. (2005) 366:662–4. doi: 10.1016/S0140-6736(05)66630-5
42. Zhu H, Belcher M, Van Der Harst P. Healthy aging and disease: role for telomere biology? Clin Sci. (2011) 120:427–40. doi: 10.1042/CS20100385
43. Vera E, Bernardes De Jesus B, Foronda M, Flores JM, Blasco MA. The rate of increase of short telomeres predicts longevity in mammals. Cell Rep. (2012) 2:732–7. doi: 10.1016/j.celrep.2012.08.023
44. Demissie S, Levy D, Benjamin EJ, Cupples LA, Gardner JP, Herbert A, et al. Insulin resistance, oxidative stress, hypertension, and leukocyte telomere length in men from the Framingham Heart Study. Aging Cell. (2006) 5:325–30. doi: 10.1111/j.1474-9726.2006.00224.x
45. Sampson MJ, Winterbone MS, Hughes JC, Dozio N, Hughes DA. Monocyte telomere shortening and oxidative DNA damage in type 2 diabetes. Diabetes Care. (2006) 29:283–9. doi: 10.2337/diacare.29.02.06.dc05-1715
46. Mourkioti F, Kustan J, Kraft P, Day JW, Zhao MM, Kost-Alimova M, et al. Role of telomere dysfunction in cardiac failure in Duchenne muscular dystrophy. Nat Cell Biol. (2013) 15:895–904. doi: 10.1038/ncb2790
47. Sharifi-Sanjani M, Oyster NM, Tichy ED, Bedi KCR, Harel O, Margulies KB, et al. Cardiomyocyte-specific telomere shortening is a distinct signature of heart failure in humans. J Am Heart Assoc. (2017) 6:e005086. doi: 10.1161/JAHA.116.005086
48. Smith CD, Carney JM, Starke-Reed PE, Oliver CN, Stadtman ER, Floyd RA, et al. Excess brain protein oxidation and enzyme dysfunction in normal aging and in Alzheimer disease. Proc Natl Acad Sci USA. (1991) 88:10540–3. doi: 10.1073/pnas.88.23.10540
49. Abdul HM, Sultana R St. Clair DK, Markesbery WR, Butterfield DA. Oxidative damage in brain from human mutant APP/PS-1 double knock-in mice as a function of age. Free Radic Biol Med. (2008) 45:1420–5. doi: 10.1016/j.freeradbiomed.2008.08.012
50. Wei YH, Wu SB, Ma YS, Lee HC. Respiratory function decline and DNA mutation in mitochondria, oxidative stress and altered gene expression during aging. Chang Gung Med J. (2009) 32:113–32.
51. Dzitoyeva S, Chen H, Manev H. Effect of aging on 5-hydroxymethylcytosine in brain mitochondria. Neurobiol Aging. (2012) 33:2881–91. doi: 10.1016/j.neurobiolaging.2012.02.006
52. Chang KT, Min KT. Drosophila melanogaster homolog of Down syndrome critical region 1 is critical for mitochondrial function. Nat Neurosci. (2005) 8:1577–85. doi: 10.1038/nn1564
53. Cho KO, Kim YS, Cho YJ, Kim SY. Upregulation of DSCR1 (RCAN1 or Adapt78) in the peri-infarct cortex after experimental stroke. Exp Neurol. (2008) 212:85–92. doi: 10.1016/j.expneurol.2008.03.017
54. Sun X, Wu Y, Chen B, Zhang Z, Zhou W, Tong Y, et al. Regulator of calcineurin 1 (RCAN1) facilitates neuronal apoptosis through caspase-3 activation. J Biol Chem. (2011) 286:9049–62. doi: 10.1074/jbc.M110.177519
55. Sobrado M, Ramirez BG, Neria F, Lizasoain I, Arbones ML, Minami T, et al. Regulator of calcineurin 1 (Rcan1) has a protective role in brain ischemia/reperfusion injury. J Neuroinflamm. (2012) 9:48. doi: 10.1186/1742-2094-9-48
56. Wu Y, Song W. Regulation of RCAN1 translation and its role in oxidative stress-induced apoptosis. FASEB J. (2013) 27:208–21. doi: 10.1096/fj.12-213124
57. Sun X, Wu Y, Herculano B, Song W. RCAN1 overexpression exacerbates calcium overloading-induced neuronal apoptosis. PLoS ONE. (2014) 9:e95471. doi: 10.1371/journal.pone.0095471
58. Wu Y, Ly PT, Song W. Aberrant expression of RCAN1 in Alzheimer's pathogenesis: a new molecular mechanism and a novel drug target. Mol Neurobiol. (2014) 50:1085–97. doi: 10.1007/s12035-014-8704-y
59. Wu Y, Deng Y, Zhang S, Luo Y, Cai F, Zhang Z, et al. Amyloid-beta precursor protein facilitates the regulator of calcineurin 1-mediated apoptosis by downregulating proteasome subunit alpha type-5 and proteasome subunit beta type-7. Neurobiol Aging. (2015) 36:169–77. doi: 10.1016/j.neurobiolaging.2014.07.029
60. Wu Y, Hou F, Wang X, Kong Q, Han X, Bai B. Aberrant expression of histone deacetylases 4 in cognitive disorders: molecular mechanisms and a potential target. Front Mol Neurosci. (2016) 9:114. doi: 10.3389/fnmol.2016.00114
61. Christoffersen M, Frikke-Schmidt R, Nordestgaard BG, Tybjaerg-Hansen A. Genetic variation in WRN and ischemic stroke: general population studies and meta-analyses. Exp Gerontol. (2017) 89:69–77. doi: 10.1016/j.exger.2017.01.005
62. Marto JP, Dias M, Alves JN, Montes V, Beato-Coelho J, Marques-Matos C, et al. Stroke in adults with Down syndrome. J Neurol Sci. (2017) 375:279–80. doi: 10.1016/j.jns.2017.02.014
63. Qiu J, Wang X, Wu F, Wan L, Cheng B, Wu Y, et al. Low dose of apelin-36 attenuates ER stress-associated apoptosis in rats with ischemic stroke. Front Neurol. (2017) 8:556. doi: 10.3389/fneur.2017.00556
64. Fu Q, Wu Y. RCAN1 in the inverse association between Alzheimer's disease and cancer. Oncotarget. (2018) 9:54–66. doi: 10.18632/oncotarget.23094
65. Wu F, Qiu J, Fan Y, Zhang Q, Cheng B, Wu Y, et al. Apelin-13 attenuates ER stress-mediated neuronal apoptosis by activating Galphai/Galphaq-CK2 signaling in ischemic stroke. Exp Neurol. (2018) 302:136–44. doi: 10.1016/j.expneurol.2018.01.006
66. Zhang S, Cai F, Wu Y, Bozorgmehr T, Wang Z, Huang D, et al. A presenilin-1 mutation causes Alzheimer disease without affecting Notch signaling. Mol Psychiatry. (2018) doi: 10.1038/s41380-018-0101-x. [Epub ahead of print].
67. Flossmann E, Schulz UG, Rothwell PM. Systematic review of methods and results of studies of the genetic epidemiology of ischemic stroke. Stroke. (2004) 35:212–27. doi: 10.1161/01.STR.0000107187.84390.AA
68. Kumar P, Misra S, Kumar A, Faruq M, Shakya S, Vardhan G, et al. Transforming growth factor-beta1 (C509T, G800A, and T869C) gene polymorphisms and risk of ischemic stroke in North Indian population: a hospital-based case-control study. Ann Indian Acad Neurol. (2017) 20:5–12. doi: 10.4103/0972-2327.199910
69. Tian T, Jin G, Yu C, Lv J, Guo Y, Bian Z, et al. Family history and stroke risk in china: evidence from a large cohort study. J Stroke. (2017) 19:188–95. doi: 10.5853/jos.2016.01270
70. Bevan S, Traylor M, Adib-Samii P, Malik R, Paul NL, Jackson C, et al. Genetic heritability of ischemic stroke and the contribution of previously reported candidate gene and genomewide associations. Stroke. (2012) 43:3161–7. doi: 10.1161/STROKEAHA.112.665760
71. Schulz UG, Flossmann E, Rothwell PM. Heritability of ischemic stroke in relation to age, vascular risk factors, and subtypes of incident stroke in population-based studies. Stroke. (2004) 35:819–24. doi: 10.1161/01.STR.0000121646.23955.0f
72. Codd V, Nelson CP, Albrecht E, Mangino M, Deelen J, Buxton JL, et al. Identification of seven loci affecting mean telomere length and their association with disease. Nat Genet. (2013) 45:422–427; 427e421–422. doi: 10.1038/ng.2528
73. Liang Y, Zhang R, Zhang S, Ji G, Shi P, Yang T, et al. Association of ACYP2 and TSPYL6 genetic polymorphisms with risk of ischemic stroke in han chinese population. Mol Neurobiol. (2017) 54:5988–95. doi: 10.1007/s12035-016-0086-x
74. Von Zglinicki T, Saretzki G, Docke W, Lotze C. Mild hyperoxia shortens telomeres and inhibits proliferation of fibroblasts: a model for senescence? Exp Cell Res. (1995) 220:186–93. doi: 10.1006/excr.1995.1305
75. Halvorsen TL, Beattie GM, Lopez AD, Hayek A, Levine F. Accelerated telomere shortening and senescence in human pancreatic islet cells stimulated to divide in vitro. J Endocrinol. (2000) 166:103–9. doi: 10.1677/joe.0.1660103
76. Suzuki K, Ito Y, Ochiai J, Kusuhara Y, Hashimoto S, Tokudome S, et al. Relationship between obesity and serum markers of oxidative stress and inflammation in Japanese. Asian Pac J Cancer Prev. (2003) 4:259–66.
77. Kurz DJ, Decary S, Hong Y, Trivier E, Akhmedov A, Erusalimsky JD. Chronic oxidative stress compromises telomere integrity and accelerates the onset of senescence in human endothelial cells. J Cell Sci. (2004) 117:2417–26. doi: 10.1242/jcs.01097
78. Rankin JW, Andreae MC, Oliver Chen CY, O'keefe SF. Effect of raisin consumption on oxidative stress and inflammation in obesity. Diabetes Obes Metab. (2008) 10:1086–96. doi: 10.1111/j.1463-1326.2008.00867.x
79. Sohet FM, Neyrinck AM, Pachikian BD, De Backer FC, Bindels LB, Niklowitz P, et al. Coenzyme Q10 supplementation lowers hepatic oxidative stress and inflammation associated with diet-induced obesity in mice. Biochem Pharmacol. (2009) 78:1391–400. doi: 10.1016/j.bcp.2009.07.008
80. Njajou OT, Cawthon RM, Blackburn EH, Harris TB, Li R, Sanders JL, et al. Shorter telomeres are associated with obesity and weight gain in the elderly. Int J Obes. (2012) 36:1176–9. doi: 10.1038/ijo.2011.196
81. Zannolli R, Mohn A, Buoni S, Pietrobelli A, Messina M, Chiarelli F, et al. Telomere length and obesity. Acta Paediatr. (2008) 97:952–4. doi: 10.1111/j.1651-2227.2008.00783.x
82. Al-Attas OS, Al-Daghri NM, Alokail MS, Alfadda A, Bamakhramah A, Sabico S, et al. Adiposity and insulin resistance correlate with telomere length in middle-aged Arabs: the influence of circulating adiponectin. Eur J Endocrinol. (2010) 163:601–7. doi: 10.1530/EJE-10-0241
83. Diaz VA, Mainous AG, Player MS, Everett CJ. Telomere length and adiposity in a racially diverse sample. Int J Obes. (2010) 34:261–5. doi: 10.1038/ijo.2009.198
84. Maceneaney OJ, Kushner EJ, Westby CM, Cech JN, Greiner JJ, Stauffer BL, et al. Endothelial progenitor cell function, apoptosis, and telomere length in overweight/obese humans. Obesity. (2010) 18:1677–82. doi: 10.1038/oby.2009.494
85. Mundstock E, Sarria EE, Zatti H, Mattos Louzada F, Kich Grun L, Herbert Jones M, et al. Effect of obesity on telomere length: systematic review and meta-analysis. Obesity. (2015) 23:2165–74. doi: 10.1002/oby.21183
86. Shah RS, Cole JW. Smoking and stroke: the more you smoke the more you stroke. Expert Rev Cardiovasc Ther. (2010) 8:917–32. doi: 10.1586/erc.10.56
87. Goldstein LB, Bushnell CD, Adams RJ, Appel LJ, Braun LT, Chaturvedi S, et al. Guidelines for the primary prevention of stroke: a guideline for healthcare professionals from the American Heart Association/American Stroke Association. Stroke. (2011) 42:517–84. doi: 10.1161/STR.0b013e3181fcb238
88. Meschia JF, Bushnell C, Boden-Albala B, Braun LT, Bravata DM, Chaturvedi S, et al. Guidelines for the primary prevention of stroke: a statement for healthcare professionals from the American Heart Association/American Stroke Association. Stroke. (2014) 45:3754–832. doi: 10.1161/STR.0000000000000046
89. Oono IP, Mackay DF, Pell JP. Meta-analysis of the association between secondhand smoke exposure and stroke. J Public Health. (2011) 33:496–502. doi: 10.1093/pubmed/fdr025
90. Malek AM, Cushman M, Lackland DT, Howard G, Mcclure LA. Secondhand smoke exposure and stroke: the reasons for geographic and racial differences in stroke (REGARDS) study. Am J Prev Med. (2015) 49:e89–97. doi: 10.1016/j.amepre.2015.04.014
91. Lin MP, Ovbiagele B, Markovic D, Towfighi A. Association of secondhand smoke with stroke outcomes. Stroke. (2016a) 47:2828–35. doi: 10.1161/STROKEAHA.116.014099
92. Astuti Y, Wardhana A, Watkins J, Wulaningsih W, Network PR. Cigarette smoking and telomere length: a systematic review of 84 studies and meta-analysis. Environ Res. (2017) 158:480–9. doi: 10.1016/j.envres.2017.06.038
93. Babizhayev MA, Savel'yeva EL, Moskvina SN, Yegorov YE. Telomere length is a biomarker of cumulative oxidative stress, biologic age, and an independent predictor of survival and therapeutic treatment requirement associated with smoking behavior. Am J Ther. (2011) 18:e209–26. doi: 10.1097/MJT.0b013e3181cf8ebb
94. Wang H, Kim H, Baik I. Associations of alcohol consumption and alcohol flush reaction with leukocyte telomere length in Korean adults. Nutr Res Pract. (2017) 11:334–9. doi: 10.4162/nrp.2017.11.4.334
95. O'donnell MJ, Xavier D, Liu L, Zhang H, Chin SL, Rao-Melacini P, et al. Risk factors for ischaemic and intracerebral haemorrhagic stroke in 22 countries (the INTERSTROKE study): a case-control study. Lancet. (2010) 376:112–23. doi: 10.1016/S0140-6736(10)60834-3
96. Henderson KM, Clark CJ, Lewis TT, Aggarwal NT, Beck T, Guo H, et al. Psychosocial distress and stroke risk in older adults. Stroke. (2013) 44:367–72. doi: 10.1161/STROKEAHA.112.679159
97. Booth J, Connelly L, Lawrence M, Chalmers C, Joice S, Becker C, et al. Evidence of perceived psychosocial stress as a risk factor for stroke in adults: a meta-analysis. BMC Neurol. (2015) 15:233. doi: 10.1186/s12883-015-0456-4
98. Ramirez-Moreno JM, Munoz Vega P, Espada S, Bartolome Alberca S, Aguirre J, Peral D. Association between self-perceived psychological stress and transitory ischaemic attack and minor stroke: a case-control study. Neurologia. (2017). doi: 10.1016/j.nrl.2017.09.012. [Epub ahead of print].
99. Epel ES, Blackburn EH, Lin J, Dhabhar FS, Adler NE, Morrow JD, et al. Accelerated telomere shortening in response to life stress. Proc Natl Acad Sci USA. (2004) 101:17312–5. doi: 10.1073/pnas.0407162101
100. Wolkowitz OM, Mellon SH, Epel ES, Lin J, Dhabhar FS, Su Y, et al. Leukocyte telomere length in major depression: correlations with chronicity, inflammation and oxidative stress–preliminary findings. PLoS ONE. (2011) 6:e17837. doi: 10.1371/journal.pone.0017837
101. Dong JY, Zhang YH, Tong J, Qin LQ. Depression and risk of stroke: a meta-analysis of prospective studies. Stroke. (2012) 43:32–7. doi: 10.1161/STROKEAHA.111.630871
102. Jackson CA, Mishra GD. Depression and risk of stroke in midaged women: a prospective longitudinal study. Stroke. (2013) 44:1555–60. doi: 10.1161/STROKEAHA.113.001147
103. Lin PY, Huang YC, Hung CF. Shortened telomere length in patients with depression: a meta-analytic study. J Psychiatr Res. (2016b) 76:84–93. doi: 10.1016/j.jpsychires.2016.01.015
104. Ridout KK, Ridout SJ, Price LH, Sen S, Tyrka AR. Depression and telomere length: a meta-analysis. J Affect Disord. (2016) 191:237–47. doi: 10.1016/j.jad.2015.11.052
105. Lewington S, Clarke R, Qizilbash N, Peto R, Collins R, Prospective Studies C. Age-specific relevance of usual blood pressure to vascular mortality: a meta-analysis of individual data for one million adults in 61 prospective studies. Lancet. (2002) 360:1903–13. doi: 10.1016/S0140-6736(02)11911-8
106. Chobanian AV, Bakris GL, Black HR, Cushman WC, Green LA, Izzo JL, et al. The seventh report of the joint national committee on prevention, detection, evaluation, and treatment of high blood pressure: the JNC 7 report. JAMA. (2003) 289:2560–72. doi: 10.1001/jama.289.19.2560
107. Ettehad D, Emdin CA, Kiran A, Anderson SG, Callender T, Emberson J, et al. Blood pressure lowering for prevention of cardiovascular disease and death: a systematic review and meta-analysis. Lancet. (2016) 387:957–67. doi: 10.1016/S0140-6736(15)01225-8
108. Thomopoulos C, Parati G, Zanchetti A. Effects of blood pressure lowering on outcome incidence in hypertension: 7. Effects of more vs less intensive blood pressure lowering and different achieved blood pressure levels - updated overview and meta-analyses of randomized trials. J Hypertens. (2016) 34:613–22. doi: 10.1097/HJH.0000000000000881
109. Xie X, Atkins E, Lv J, Bennett A, Neal B, Ninomiya T, et al. Effects of intensive blood pressure lowering on cardiovascular and renal outcomes: updated systematic review and meta-analysis. Lancet. (2016) 387:435–43. doi: 10.1016/S0140-6736(15)00805-3
110. Jeanclos E, Schork NJ, Kyvik KO, Kimura M, Skurnick JH, Aviv A. Telomere length inversely correlates with pulse pressure and is highly familial. Hypertension. (2000) 36:195–200. doi: 10.1161/01.HYP.36.2.195
111. Benetos A, Okuda K, Lajemi M, Kimura M, Thomas F, Skurnick J, et al. Telomere length as an indicator of biological aging: the gender effect and relation with pulse pressure and pulse wave velocity. Hypertension. (2001) 37:381–85. doi: 10.1161/01.HYP.37.2.381
112. Tellechea ML, Pirola CJ. The impact of hypertension on leukocyte telomere length: a systematic review and meta-analysis of human studies. J Hum Hypertens. (2017) 31:99–105. doi: 10.1038/jhh.2016.45
113. Boehme AK, Esenwa C, Elkind MS. Stroke risk factors, genetics, and prevention. Circ Res. (2017) 120:472–95. doi: 10.1161/CIRCRESAHA.116.308398
114. Jeanclos E, Krolewski A, Skurnick J, Kimura M, Aviv H, Warram JH, et al. Shortened telomere length in white blood cells of patients with IDDM. Diabetes. (1998) 47:482–6. doi: 10.2337/diabetes.47.3.482
115. Adaikalakoteswari A, Balasubramanyam M, Mohan V. Telomere shortening occurs in Asian Indian Type 2 diabetic patients. Diabet Med. (2005) 22:1151–6. doi: 10.1111/j.1464-5491.2005.01574.x
116. Adaikalakoteswari A, Balasubramanyam M, Ravikumar R, Deepa R, Mohan V. Association of telomere shortening with impaired glucose tolerance and diabetic macroangiopathy. Atherosclerosis. (2007) 195:83–9. doi: 10.1016/j.atherosclerosis.2006.12.003
117. Uziel O, Singer JA, Danicek V, Sahar G, Berkov E, Luchansky M, et al. Telomere dynamics in arteries and mononuclear cells of diabetic patients: effect of diabetes and of glycemic control. Exp Gerontol. (2007) 42:971–8. doi: 10.1016/j.exger.2007.07.005
118. Olivieri F, Lorenzi M, Antonicelli R, Testa R, Sirolla C, Cardelli M, et al. Leukocyte telomere shortening in elderly Type2DM patients with previous myocardial infarction. Atherosclerosis. (2009) 206:588–93. doi: 10.1016/j.atherosclerosis.2009.03.034
119. Salpea KD, Talmud PJ, Cooper JA, Maubaret CG, Stephens JW, Abelak K, et al. Association of telomere length with type 2 diabetes, oxidative stress and UCP2 gene variation. Atherosclerosis. (2010) 209:42–50. doi: 10.1016/j.atherosclerosis.2009.09.070
120. Zee RY, Castonguay AJ, Barton NS, Germer S, Martin M. Mean leukocyte telomere length shortening and type 2 diabetes mellitus: a case-control study. Transl Res. (2010a) 155:166–9. doi: 10.1016/j.trsl.2009.09.012
121. Shen Q, Zhao X, Yu L, Zhang Z, Zhou D, Kan M, et al. Association of leukocyte telomere length with type 2 diabetes in mainland Chinese populations. J Clin Endocrinol Metab. (2012) 97:1371–4. doi: 10.1210/jc.2011-1562
122. Gardner JP, Li S, Srinivasan SR, Chen W, Kimura M, Lu X, et al. Rise in insulin resistance is associated with escalated telomere attrition. Circulation. (2005) 111:2171–7. doi: 10.1161/01.CIR.0000163550.70487.0B
123. Samani NJ, Boultby R, Butler R, Thompson JR, Goodall AH. Telomere shortening in atherosclerosis. Lancet. (2001) 358:472–3. doi: 10.1016/S0140-6736(01)05633-1
124. Brouilette S, Singh RK, Thompson JR, Goodall AH, Samani NJ. White cell telomere length and risk of premature myocardial infarction. Arterioscler Thromb Vasc Biol. (2003) 23:842–6. doi: 10.1161/01.ATV.0000067426.96344.32
125. Benetos A, Gardner JP, Zureik M, Labat C, Xiaobin L, Adamopoulos C, et al. Short telomeres are associated with increased carotid atherosclerosis in hypertensive subjects. Hypertension. (2004) 43:182–5. doi: 10.1161/01.HYP.0000113081.42868.f4
126. Farzaneh-Far R, Cawthon RM, Na B, Browner WS, Schiller NB, Whooley MA. Prognostic value of leukocyte telomere length in patients with stable coronary artery disease: data from the Heart and Soul Study. Arterioscler Thromb Vasc Biol. (2008) 28:1379–84. doi: 10.1161/ATVBAHA.108.167049
127. Huzen J, Van Veldhuisen DJ, Van Gilst WH, Van Der Harst P. Telomeres and biological ageing in cardiovascular disease. Ned Tijdschr Geneeskd. (2008) 152:1265–70.
128. O'donnell CJ, Demissie S, Kimura M, Levy D, Gardner JP, White C, et al. Leukocyte telomere length and carotid artery intimal medial thickness: the Framingham Heart Study. Arterioscler Thromb Vasc Biol. 28:1165–71. doi: 10.1161/ATVBAHA.107.154849
129. Allende M, Molina E, Gonzalez-Porras JR, Toledo E, Lecumberri R, Hermida J. Short leukocyte telomere length is associated with cardioembolic stroke risk in patients with atrial fibrillation. Stroke. (2016) 47:863–5. doi: 10.1161/STROKEAHA.115.011837
130. Chen S, Lin J, Matsuguchi T, Blackburn E, Yeh F, Best LG, et al. Short leukocyte telomere length predicts incidence and progression of carotid atherosclerosis in American Indians: the Strong Heart Family Study. Aging. (2014) 6:414–27. doi: 10.18632/aging.100671
131. Rietzschel ER, Bekaert S, De Meyer T. Telomeres and atherosclerosis: the attrition of an attractive hypothesis. J Am Coll Cardiol. (2016) 67:2477–9. doi: 10.1016/j.jacc.2016.03.541
132. De Meyer T, De Buyzere ML. Telomeres and atherosclerosis: the intricate pursuit of mechanistic insight through epidemiology. Hypertension. (2017) 70:243–4. doi: 10.1161/HYPERTENSIONAHA.117.09454
133. De Meyer T, Nawrot T, Bekaert S, De Buyzere ML, Rietzschel ER, Andres V. Telomere length as cardiovascular aging biomarker: JACC review topic of the week. J Am Coll Cardiol. (2018) 72:805–13. doi: 10.1016/j.jacc.2018.06.014
134. Scheller Madrid A, Rode L, Nordestgaard BG, Bojesen SE. Short telomere length and ischemic heart disease: observational and genetic studies in 290 022 individuals. Clin Chem. (2016) 62:1140–9. doi: 10.1373/clinchem.2016.258566
135. Bekaert S, De MT, Rietzschel ER, De Buyzere ML, De BD, Langlois M, et al. Telomere length and cardiovascular risk factors in a middle-aged population free of overt cardiovascular disease. Aging Cell. (2007) 6:639–47. doi: 10.1111/j.1474-9726.2007.00321.x
136. De Meyer T, Rietzschel ER, De Buyzere ML, Langlois MR, De Bacquer D, Segers P, et al. Systemic telomere length and preclinical atherosclerosis: the Asklepios Study. Eur Heart J. (2009) 30:3074–81. doi: 10.1093/eurheartj/ehp324
Keywords: telomere, telomerase, telomere length, risk of stroke, prognosis of stroke
Citation: Tian Y, Wang S, Jiao F, Kong Q, Liu C and Wu Y (2019) Telomere Length: A Potential Biomarker for the Risk and Prognosis of Stroke. Front. Neurol. 10:624. doi: 10.3389/fneur.2019.00624
Received: 14 December 2018; Accepted: 28 May 2019;
Published: 13 June 2019.
Edited by:
Gregory Jaye Bix, University of Kentucky, United StatesReviewed by:
Marc De Buyzere, Ghent University, BelgiumRobert Y. Zee, Tufts Medical Center, United States
Copyright © 2019 Tian, Wang, Jiao, Kong, Liu and Wu. This is an open-access article distributed under the terms of the Creative Commons Attribution License (CC BY). The use, distribution or reproduction in other forums is permitted, provided the original author(s) and the copyright owner(s) are credited and that the original publication in this journal is cited, in accordance with accepted academic practice. No use, distribution or reproduction is permitted which does not comply with these terms.
*Correspondence: Yili Wu, eWlsaV93dTIwMDRAeWFob28uY2E=; d3V5aWxpQG1haWwuam5tYy5lZHUuY24=
†These authors have contributed equally to this work