- Neural Analytics, Inc., Los Angeles, CA, United States
The possibility of sex-related differences in mild traumatic brain injury (mTBI) severity and recovery remains a controversial subject. With some studies showing that female subjects suffer a longer period of symptom recovery, while others have failed to demonstrate differences. In this study, we explored the sex-related effects of mTBI on self-reported symptoms and transcranial Doppler ultrasound (TCD) measured features in an adolescent population. Fifty-eight subjects were assessed—at different points post-injury—after suffering an mTBI. Subjects answered a series of symptom questions before the velocity from the middle cerebral artery was measured. Subjects participated in breath-holding challenges to evaluate cerebrovascular reactivity. The Pulsatility Index (PI), the ratio of the first peaks (P2R), and the Breath-Hold Index (BHI), were computed. Linear mixed effects models were developed to explore the interactions between measured features, sex, and time since injury while accounting for within subject variation. Over the first 10 days post-injury, the female group had significant interactions between sex and time since injury that was not present in the TCD features. This is the first study to compare sex-related differences in self-reported symptoms and TCD measurements in adolescents suffering an mTBI. It illustrates the pitfalls clinicians face when relying on subjective measures alone during diagnosis and tracking of mTBI patients. In addition, it highlights the need for more focused research on sex-related differences in concussion pathophysiology.
Introduction
The presence of sex-related differences in mild traumatic brain injuries or concussions (mTBI), is a contentious subject. Several studies have found increased symptoms in females (1–3), as well as increased length of recovery (4–6). In the case of Preiss-Farzanegan et al. (7), a difference existed in adults (18 years-old or older), but not in minors (17 years-old and younger). Conversely, others have failed to demonstrate increases in female symptoms at all (8–12). In general, the current state of the literature suggests that the existence of gender dependence in concussion recovery and severity is still an open question (13, 14).
One commonality between these studies however, is that they all rely on neurocognitive evaluations, patient symptoms, or physical performance testing. Although these have been shown to provide insight into concussion severity and prognosis, they do not objectively measure physiological changes resulting from concussive injury. Here, we present a study comparing features of the cerebral blood flow velocity (CBFV) as measured with Transcranial Doppler (TCD) ultrasound in addition to self-reported symptoms to investigate sex-related differences in concussion.
With recent research demonstrating abnormalities in cerebral blood flow after a mTBI, it is clear that the microvasculature is affected (15–24). In Thibeault et al. (23), the cerebral hemodynamic changes in adolescents between 14 and 19 years old after suffering a clinically diagnosed mTBI were assessed using TCD. In that study two distinct phases of hemodynamic alterations after a concussive injury were identified. In the initial phase, beginning within an hour of injury and lasting through the first 48 h, Pulsatility Index (PI), and peak ratio (P2R), showed a significant difference from controls. After 48 h however, these differences in pulsatile features were no longer observable. At this point in their recovery the breath-holding index (BHI), a measure of the cerebral vascular reactivity (CVR), was significantly increased when compared to controls. This lasted through day seven. After which, the population level increase was no longer significant.
Although Thibeault et al. (23) was the first study to suggest the presence of multiple phases of hemodynamic dysfunction, there have been others demonstrating measurable alterations in mTBI subjects using TCD. Utilizing a hypercapnia challenge, Len et al. (15) found significant changes in a population of concussed subjects. A subsequent study found significant differences during hypocapnia (24). Similarly, the study from Albalawi et al. (25), found vasoreactivity was linearly related to both severe headaches and cognitive symptoms. Baily et al. (18), found lowered CVR in a population of subjects suffering from chronic symptoms. The present study, however, appears to be the first to explore sex specific abnormalities in mTBI subjects with both self-reported symptoms and an objective physiological measure.
Methods
Patient Population
Participants in this study consisted of adolescents between 14 and 19 years old from the Los Angeles, California metropolitan area. Subjects classified with an mTBI were diagnosed by independent physicians and were scanned at different times post-injury. For this analysis these longitudinal measurements were restricted to 13 days post-jury from 58 unique subjects. The population was comprised of 37 male and 21 female participants, with 81 and 57 total exams for each group, respectively. Within the male group, 17 subjects had more than one scan during the course of recovery and a median number of scans of 1.0 with an IQR of 2.0. In the female group, 13 subjects had more than one scan and there as an overall median 2.0 scans with an IQR 3.0. The control group consisted of 109 age-matched subjects, 89 male and 12 female, who had no reported head-injuries in the preceding 12-months. The control group was only scanned a once. The study was approved by Western Institutional Review Board (IRB #20141111). This data was previously used in Thibeault et al. (23).
Data Collection
The TCD signals were acquired from the middle cerebral arteries (MCA) transtemporally by ultrasonographers utilizing 2 MHz probes held by an adjustable headset. End-tidal CO2 was collected concurrently through a nasal cannula. The exam protocol, illustrated in Figure 1, began with a 5-min baseline period of normal breathing. This was followed by a series of 4 breath-holding challenges as an estimate of CVR. Each of these consisted of a 25-s period where the subject was instructed to hold their breath, followed by 35-s of normal breathing.
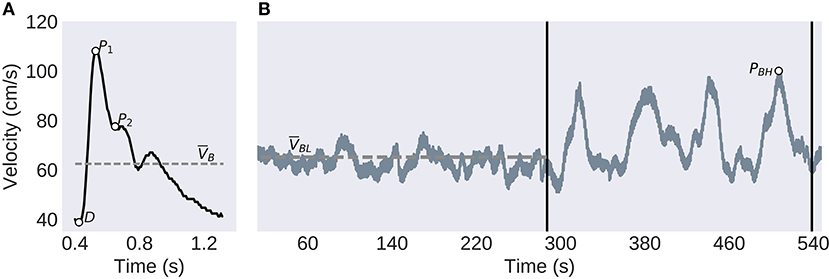
Figure 1. Experimental Protocol and TCD Analysis. (A) The individual pulses are extracted before the systolic peak (P1), diastolic trough (D), second peak (P2), and the mean velocity (VB) are identified. (B) The CVR protocol consists of a 5-min baseline, left of the first vertical bar, followed by the four breath-holding challenges, between the vertical bars. The low-frequency component of the global signal (solid gray line), is used to compute the baseline mean velocity (VBL, dashed line) and the largest peak velocity (PBH) used to calculate the BHI.
Symptom Reporting
Before each of the data collection session, subjects were asked to answer a number of questions similar to the graded symptom scale checklist. Table 1 presents the list of questions where subjects were asked to numerically rate their current symptom state. The ratings were used both individually and summed together as an estimate of severity.
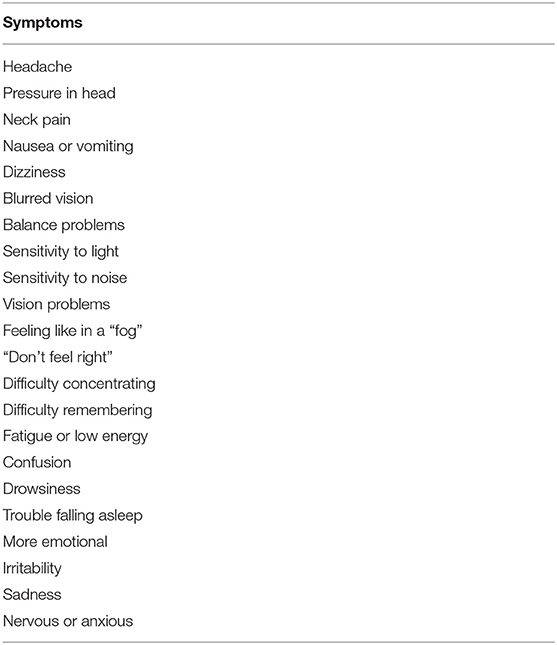
Table 1. Subjects were asked score themselves on the following symptoms based on how they feel now–None (0), Mild (1,2), Moderate (3,4), Severe (5,6).
Analysis
The TCD features found to correlate with mTBI in Thibeault et al. (23), were used to compare with the self-reported symptoms. The first pulse level feature, extracted from the baseline section, was the PI. This is generally believed to be related to distal resistance however, it appears to be more modulated by a number of physiological processes (26), PI is found by
Where P1, D, and VB are defined in Figure 1.
The second, P2R, is the ratio of P2, and P1, as illustrated in Figure 1. This has been hypothesized to be related to distal bed compliance (27). This is found by
These features were individually averaged across all the extracted pulses from the baseline section.
The CVR was estimated using the BHI. This was found by first finding the highest peak of the low-pass filtered CBFV waveform between the four breath-hold sections as illustrated in Figure 1. This is then related to the baseline mean velocity by
Statistical Modeling
Linear mixed-effect models were developed to explore the interactions between effects of time and sex on the measured variables while compensating for the unbalanced groupings and the potential individual subject variation. The models were developed in R using the lme4 package (28). Summary statistics and significance values—using the satterthwaite method of degrees of freedom and t-test—were computed with the lmertest package (29). Additional model analysis was completed with the Psycho library (30). Effects were considered significant if p < 0.05, and the reported beta was at least twice the standard error (SE).
The models were developed for each of the three TCD features as well as the summed symptom scores, as dependent variables. For symptoms and BHI, the random-effects were explored by fitting different models with the maximum likelihood method and comparing with the likelihood ratio test—the models with significant improvement were selected. The fixed-effects and interactions were similarly compared, and the final models were then fit with the restricted maximum likelihood method. The models for PI and P2R failed to converge with the maximum likelihood method, however, the restricted method did reach convergence. Because of this, the resulting models both used a similar structure, with days-post injury, sex, and their interactions as fixed effects, and subject specific intercepts as random effects. For the sex category, a contrast encoding of [0.5, −0.5] with males as the reference was employed. Similarly, a dummy encoding with the controls as the reference group was used for the days-post category. These were grouped similar to Thibeault et al. (23). Correlations between features were evaluated using the Pearson correlation coefficient and the sex dependent interactions of the resulting regression lines were explored using the ANCOVA method with a set of linear models fit with the ordinary least squares method from lme4 (28). A one-way ANOVA was conducted to compare the effect of sex and condition (case or control), on age using the StatsModels package (31) in Python.
Results
Population
There was no significant interaction between the effects of sex and condition on age [F(1, 163) = 0.08, p = 0.78], or main effects of either sex [F(1, 163) = 1.86, p = 0.17] or condition [F(1, 163) = 0.004, p = 0.96]. All subjects identified as athletes, with the majority of males, 26 (74%) subjects from the mTBI group and 77 (87%) from the control group, playing football as their primary sport. The other subjects were split between rugby, soccer, basketball, baseball, lacrosse, ice hockey, and quidditch. Although within the female population soccer was the most popular, 10 (45%) from the mTBI group and 7 (75%) subjects from the control group, the overall spread was more diverse and included volleyball, dance, track, swimming, basketball, softball and cheer. Within the mTBI population there was a slight difference in the reported mechanism of injury. For the male population 33 (89%) subjects reported being injured playing a sport, while 4 (11%) did not provide a mechanism. Within the female population 16 (76%) identified their cause of injury from a sport, whereas 5 (24%) reported another mechanism or did not provide a cause.
Symptoms
The model for symptoms had an explanatory power (conditional R2) of 76.74%, in which the fixed effects explain 49.95% of the variance (marginal R2). Though there was no overall main effect of sex in the model (β = 2.91, SE = 3.94, 95% CI [−4.58, 10.51], t(215) = 0.74, p > 0.1), there were significant interactions between sex and days-post groups for the first 11 days, see Table 2. This is illustrated by the increased self-reported summed symptoms scores in the female population in Figure 2A. In addition, the individual symptom averages in Figure 3 illustrate that it was not a small subset of symptoms dominating the summed score for the female population. Additionally, there were large main effects for all days-post groupings, see Table 2.
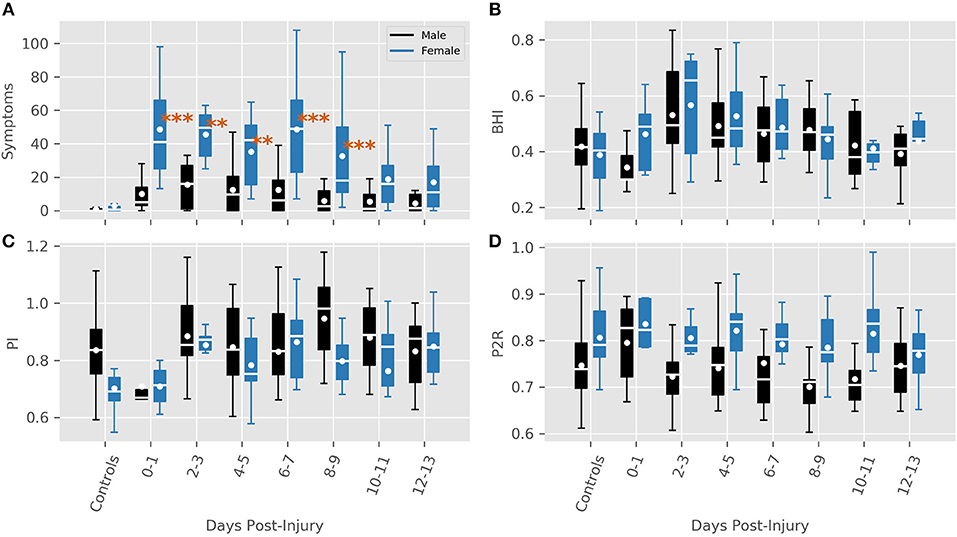
Figure 2. Features for each sex grouped by days post-injury. (A) Summed symptoms. (B) BHI. (C) PI (D) P2R. Significance values are indicated where a large interaction between sex and days-post was found (**P < 0.01, ***P < 0.001).
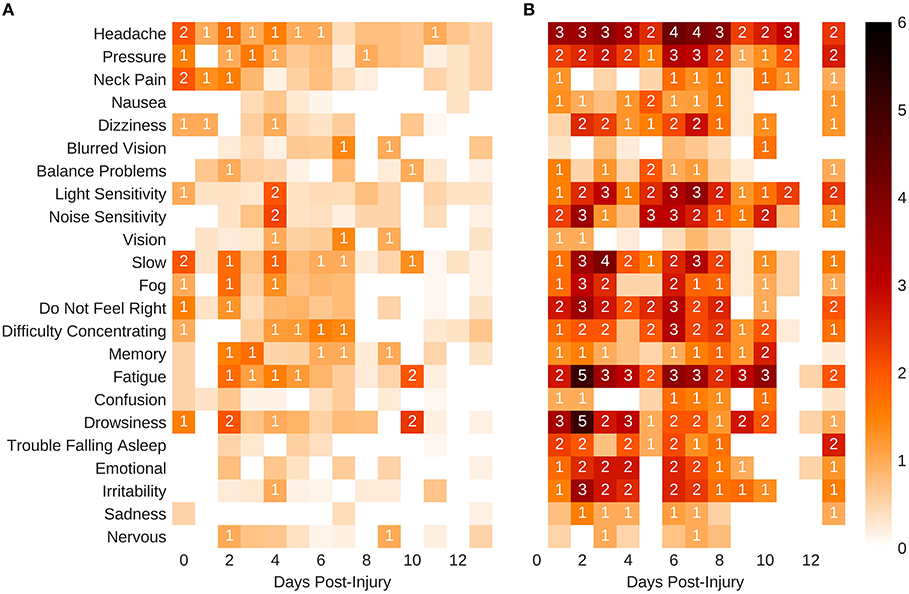
Figure 3. Self-reported mean symptom scores. (A) Males. (B) Females. The shaded squares without a value are below 1.
TCD Features
BHI
The BHI model had a total a total explanatory power (conditional R2) of 45.16%, in which the fixed effects explain 13.83% of the variance (marginal R2). There was no main effect of sex found in the model, (β = −0.03, SE = 0.03, 95% CI [−0.09, 0.04], t(215) = −0.73, p > 0.1), but there was one large interaction between sex and days-post at the 0–1 days grouping (β = 0.15, SE = 0.07, 95% CI [0.01, 0.29], t(215) = 1.98, p < 0.05), Table 3. However, within this grouping, all of the male subjects were collected on the day of their injury, while the female subjects were all collected the day after their injury occurred. In this instance, it seems more feasible that the interaction is a product of the female subjects being collected closer to the period of hyperreactivity found in Thibeault et al. (23), as opposed to a sex-related disparity.
The overall longitudinal profile found in Thibeault et al. (23) was also predicted here by main effects for days-post 2–3 (β = 0.14, SE = 0.03, 95% CI [0.08, 0.21], t(224) = 4.44, p < 0.001), 4–5 (β = 0.09, SE = 0.03, 95% CI [0.04, 0.15], t(222) = 3.17, p < 0.01), and 6–7 (β = 0.06, SE = 0.03, 95% CI [0.01, 0.12], t(231) = 2.32, p < 0.05), see Figure 1B.
PI
The model for PI had total explanatory power (conditional R2) of 72.02%, in which the fixed effects explain 8.35% of the variance (marginal R2). Here, a main effect of sex was predicted (β = −0.13, SE = 0.04, 95% CI [−0.21, −0.04], t(195) = −2.96, p < 0.01). Exploring the population results in Figure 2C suggest that this effect may be a product of an inherent difference between males and females in the control population, as opposed to a sex-related difference. This is supported by the lack of significant interactions between sex and days-post injury grouping, Table 4. There was a significant main effect found at days-post 8–9 (β = 0.07, SE = 0.03, 95% CI [0.01, 0.13], t(227) = 2.14, p < 0.05), that cannot be fully explained.
P2R
The model predicting P2R had a total explanatory power (conditional R2) of 74.42%, in which the fixed effects explain 10.32% of the variance (marginal R2). A main effect of sex was present (β = 0.05, SE = 0.02, 95% CI [0.01, 0.10], t(196) = 2.21, p < 0.05). However, similar to PI, Figure 2D illustrates a difference between control groups. There were no significant interactions between sex and days-post found, see Table 5.
Correlations
The correlations between features provides additional information about the sex-related differences in this population, Figures 4A,B. Both sexes had significant negative correlations between PI and P2R (rmale = −0.8, p < 0.001; rfemale = −0.67, p < 0.001). For the male population there were significant correlations between BHI and PI (r = 0.27, p < 0.001), as well as BHI and P2R (−0.18, p = 0.02), that were no present in the female population, Table 6. Conversely, the female population had significant correlations between symptoms and BHI (r = 0.28, p < 0.01), as well as symptoms and PI (r = 0.28, p < 0.01), that were not found in the male population, Table 6.
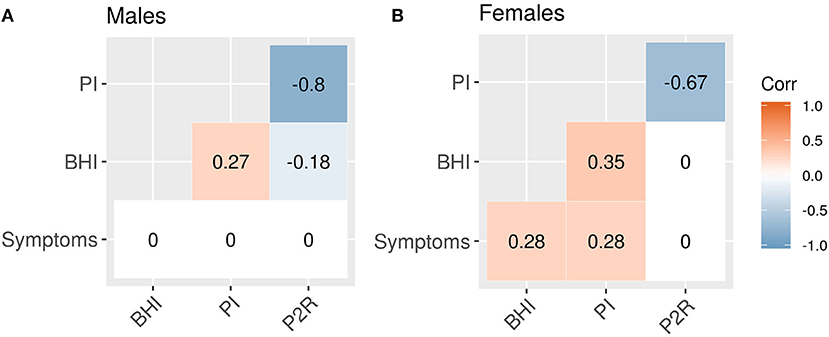
Figure 4. Feature correlations. (A) Male correlations. (B) Female correlations. Correlations that are not significant at the 0.05 level are set to zero.
Further exploring the sex-related correlation structure by an ANCOVA analysis reveals a significant difference in the slopes of the regression lines for symptoms and BHI between sexes (β = 58.55, SE = 19.07, 95% CI [20.98, 96.12], t = 3.07, p < 0.01). A difference in slopes was also found comparing symptoms and PI (β = 69.08, SE = 17.58, 95% CI [34.45, 103.70], t = 3.93, p < 0.001). For symptoms and P2R however, there was no significant difference in slope (β = −56.21, SE = 31.79, 95% CI [−118.82, 6.40], t = −1.77, p = 0.08), instead a difference in y-intercepts was found (β = 69.45, SE = 25.11, 95% CI [20.00, 118.91], t = 2.77, p < 0.01).
Discussion
Symptoms
Several studies have found a similar increase in self-reported symptoms for female subjects (1, 2). In the study from Baker et al. (4), the increased symptoms in the acute stage may have influenced recovery time—explaining the prolonged recovery for females. Although the difference between sexes here does appear more pronounced, comparing that difference to those other studies is not possible given the heterogeneity of the symptom collection.
The mechanism of injury presents a potentially confounding factor. In this study the majority of male subjects played helmeted sports (68%). The protection afforded by these helmets could have contributed to the overall lowered symptoms. However, in the study from Broshek et al. (1), female subjects were more than twice as likely to experience cognitive impairments than males in unhelmeted sports—illustrating that a difference existed even when accounting for helmets. The sex differences in reported symptoms observed in the current study were not accompanied by evidence for corresponding concussion-related differences in the TCD features. Moreover, the main effects of time observed for BHI suggest the progression of vascular injury to be similar for both sexes. A more compelling explanation would be an inherent reporting bias in the female group. Other studies have shown that female athletes tend to report more symptoms than males (7, 32). In addition, females are generally more focused on, and aware of, their health (33), suggesting that there is more of a motivation to ensure a complete recovery. Conversely, male athletes have a number of societal and cultural motivations to perceptually diminish the magnitude of their injury and return to sport as soon as possible (34). Similarly, it was shown in Kerr et al. (35), that male athletes were significantly more likely to hide a concussive injury.
There have been several studies exploring the physiological differences between sexes that contribute to the susceptibility and recovery from concussion, many of which center on the possible role of estrogen. In more severe traumatic brain injuries, estrogen has been shown to have a neuroprotective effect in male rats, but a deleterious one in females (36). In humans, the study from Gallagher et al. (5), found that female subjects suffering from a sport-related concussion who used hormonal contraceptives reported lower symptom severity than those who did not, suggesting that hormonal contraceptives may play a role in modulating the collapsing neurometabolic cascade that is a hallmark of concussive injuries (16). Another consistent theme in mTBI gender differences is decreased neck strength in women (37, 38), which has been shown to be inversely related to concussion susceptibility. A similar confound of this study is the role physical maturity plays in how someone responds to an mTBI. The study from Krix et al. (39) found that male subjects in early stages of puberty had increased odds of a prolonged recovery from a concussive injury. Although puberty clearly affects the adolescent brain (40), it is still unclear how that would contribute to the results of this work.
TCD Features
It is important to note that in this context BHI is not meant an exact measure of reactivity. Breath-holding can introduce other autonomic and sympathetic responses that can confound its use for directly quantifying reactivity. However, as illustrated in Thibeault et al. (23), and confirmed by the main effects of days-post here, BHI as measured in this population, is a robust biomarker of mTBI. The difference in slopes of the regression lines between symptoms and BHI illustrate the vulnerability of relying on subjective measures alone.
The overall sex-related main effect for both PI and P2R is most surprising aspect of this analysis. For both features that effect did not appear to be based on the injury, but rather an inherent sex-related difference in this population. Previously, when sex was ignored both were altered immediately following an mTBI (23). The alterations of these features here, as illustrated in Figure 2, appear to only be present in the male population. PI is a complex metric that is influenced by the combinations of cerebral perfusion pressure, cerebrovascular resistance, arterial bed compliance, heart rate, and the pulse amplitude (26). Similarly, It has proposed that P2R is associated with distal bed compliance dynamics (41), however there is no established physiological correlation. Why either of these features would have a sex-related dependence is unclear and will need to be explored further in the future. Regardless, these results illustrate that that dependence is not due to the injury.
The study from Esposito et al. (42) showed that women had higher Cerebral Blood Flow (CBF) compared to males. Although, in the population here there was no significant trend in mean velocity during injury recovery, that may be because TCD cannot measure CBF directly, only the velocity. In addition, a post-concussive change in mean velocity has not been demonstrated with TCD (23).
Conclusions
This is the first study to compare sex-related differences between clinical symptoms and TCD measurements in adolescent mTBI subjects. The objective measures highlight the need to mitigate patient heterogeneity when assessing concussion recovery and the discrepancy in clinical symptoms illustrates how difficult this can be for clinicians. In the case of males the possibility of under-reporting may need to be considered. A physiological measurement such as TCD may eventually help remove ambiguity and provide clinicians with an objective physiological measure of mTBI recovery.
Data Availability
The raw data supporting the conclusions of this manuscript will be made available by the authors, without undue reservation, to any qualified researcher.
Ethics Statement
This study was carried out in accordance with the recommendations of Western Institutional Review Board (IRB #20141111), with written informed consent from all subjects. All subjects gave written informed consent in accordance with the Declaration of Helsinki. The protocol was approved by the Western Institutional Review Board.
Author Contributions
CT had full access to all the data in the study and takes responsibility for the integrity of the data and the accuracy of the data analysis. RH: study concept and design. CT and ST: analysis. CT, ST, and RH: interpretation of data. CT and ST: drafting of the manuscript. RH, SW, and ST: critical revision of the manuscript for important intellectual content. CT and ST: statistical analysis. NC and SW: technical or material support. RH and CT: study supervision.
Funding
This work was supported by the National Institute Of Neurological Disorders And Stroke of the National Institutes of Health under award numbers 1R43NS092209-01 and 2R44NS092209-02.
The NIH had no role in the design and conduct of the study; collection, management, analysis, and interpretation of the data; preparation, review, or approval of the manuscript; and decision to submit the manuscript for publication. The content is solely the responsibility of the authors and does not necessarily represent the official views of the National Institutes of Health.
Conflict of Interest Statement
At the time that this research was conducted, CT, ST, NC, SW, and RH, were employees of, and either hold stock or stock options in, Neural Analytics, Inc.
References
1. Broshek DK, Kaushik T, Freeman JR, Erlanger D, Webbe F, Barth JT. Sex differences in outcome following sports-related concussion. J Neurosurg. (2005) 102:856–63. doi: 10.3171/jns.2005.102.5.0856
2. Henry LC, Elbin RJ, Collins MW, Marchetti G, Kontos AP. Examining recovery trajectories after sport-related concussion with a multimodal clinical assessment approach. Neurosurgery. (2016) 78:232–41. doi: 10.1227/NEU.0000000000001041
3. Colvin AC, Mullen J, Lovell MR, West RV, Collins MW, Groh M. The role of concussion history and gender in recovery from soccer-related concussion. Am J Sports Med. (2009) 37:1699–704. doi: 10.1177/0363546509332497
4. Baker JG, Leddy JJ, Darling SR, Shucard J, Makdissi M, Willer BS. Gender differences in recovery from sports-related concussion in adolescents. Clin Pediatr. (2016) 55:771–5. doi: 10.1177/0009922815606417
5. Gallagher V, Kramer N, Abbott K, Alexander J, Breiter H, Herrold A, et al. The effects of sex differences and hormonal contraception on outcomes after collegiate sports-related concussion. J Neurotrauma. (2018) 35:1242–7. doi: 10.1089/neu.2017.5453
6. Thomas DJ, Coxe K, Li H, Pommering TL, Young JA, Smith GA, et al. Length of recovery from sports-related concussions in pediatric patients treated at concussion clinics. Clin J Sport Med. (2018) 28:56–63. doi: 10.1097/JSM.0000000000000413
7. Preiss-Farzanegan SJ, Chapman B, Wong TM, Wu J, Bazarian JJ. The relationship between gender and postconcussion symptoms after sport-related mild traumatic brain injury. PMR. (2009) 1:245–53. doi: 10.1016/j.pmrj.2009.01.011
8. Covassin T, Schatz P, Swanik CB. Sex differences in neuropsychological function and post-concussion symptoms of concussed collegiate athletes. Neurosurgery. (2007) 61:345–50; discussion 350–1. doi: 10.1227/01.NEU.0000279972.95060.CB
9. Chan RC. Base rate of post-concussion symptoms among normal people and its neuropsychological correlates. Clin Rehabil. (2001) 15:266–73. doi: 10.1191/026921501675253420
10. Kontos AP, Covassin T, Elbin RJ, Parker T. Depression and neurocognitive performance after concussion among male and female high school and collegiate athletes. Arch Phys Med Rehabil. (2012) 93:1751–6. doi: 10.1016/j.apmr.2012.03.032
11. Frommer LJ, Gurka KK, Cross KM, Ingersoll CD, Dawn Comstock R, Saliba SA. Sex differences in concussion symptoms of high school Athletes. J Athl Train. (2011) 46:76–84. doi: 10.4085/1062-6050-46.1.76
12. Zuckerman SL, Solomon GS, Forbes JA, Haase RF, Sills AK, Lovell MR. Response to acute concussive injury in soccer players: is gender a modifying factor? J Neurosurg Pediatr. (2012) 10:504–10. doi: 10.3171/2012.8.PEDS12139
13. McCrory P, Meeuwisse W, Johnston K, Dvorak J, Aubry M, Molloy M, et al. Consensus statement on concussion in sport – the 3rd International conference on concussion in sport held in Zurich, november 2008. PMR. (2009) 1:406–20. doi: 10.1016/j.pmrj.2009.03.010
14. Makdissi M, Davis G, Jordan B, Patricios J, Purcell L, Putukian M. Revisiting the modifiers: how should the evaluation and management of acute concussions differ in specific groups? Br J Sports Med. (2013) 47:314–20. doi: 10.1136/bjsports-2013-092256
15. Len TK, Neary JP, Asmundson GJG, Goodman DG, Bjornson B, Bhambhani YN. Cerebrovascular reactivity impairment after sport-induced concussion. Med Sci Sports Exerc. (2011) 43:2241–8. doi: 10.1249/MSS.0b013e3182249539
16. Giza CC, Hovda DA. The new neurometabolic cascade of concussion. Neurosurgery. (2014) 75(Suppl. 4):S24–33. doi: 10.1227/NEU.0000000000000505
17. Meier TB, Bellgowan PSF, Singh R, Kuplicki R, Polanski DW, Mayer AR. Recovery of cerebral blood flow following sports-related concussion. JAMA Neurol. (2015) 72:530–8. doi: 10.1001/jamaneurol.2014.4778
18. Bailey DM, Jones DW, Sinnott A, Brugniaux JV, New KJ, Hodson D, et al. Impaired cerebral haemodynamic function associated with chronic traumatic brain injury in professional boxers. Clin Sci. (2013) 124:177–89. doi: 10.1042/CS20120259
19. Militana AR, Donahue MJ, Sills AK, Solomon GS, Gregory AJ, Strother MK, et al. Alterations in default-mode network connectivity may be influenced by cerebrovascular changes within 1 week of sports related concussion in college varsity athletes: a pilot study. Brain Imaging Behav. (2016) 10:559–68. doi: 10.1007/s11682-015-9407-3
20. Barkhoudarian G, Hovda DA, Giza CC. The molecular pathophysiology of concussive brain injury – an update. Phys Med Rehabil Clin N Am. (2016) 27:373–93. doi: 10.1016/j.pmr.2016.01.003
21. Maugans TA, Farley C, Altaye M, Leach J, Cecil KM. Pediatric sports-related concussion produces cerebral blood flow alterations. Pediatrics. (2012) 129:28–37. doi: 10.1542/peds.2011-2083
22. Mutch WAC, Ellis MJ, Ryner LN, McDonald PJ, Morissette MP, Pries P, et al. Patient-specific alterations in CO2 cerebrovascular responsiveness in acute and sub-acute sports-related concussion. Front Neurol. (2018) 9:23. doi: 10.3389/fneur.2018.00023
23. Thibeault CM, Thorpe S, O'Brien MJ, Canac N, Ranjbaran M, Patanam I, et al. A cross-sectional study on cerebral hemodynamics after mild traumatic brain injury in a pediatric population. Front Neurol. (2018) 9:200. doi: 10.3389/fneur.2018.00200
24. Len TK, Neary JP, Asmundson GJG, Candow DG, Goodman DG, Bjornson B, et al. Serial monitoring of CO2 reactivity following sport concussion using hypocapnia and hypercapnia. Brain Inj. (2013) 27:346–53. doi: 10.3109/02699052.2012.743185
25. Albalawi T, Hamner JW, Lapointe M, Meehan WP, Tan CO. The relationship between cerebral vasoreactivity and post-concussive symptom severity. J. Neurotrauma. (2017) 34:2700–5. doi: 10.1089/neu.2017.5060
26. de Riva N, Budohoski KP, Smielewski P, Kasprowicz M, Zweifel C, Steiner LA, et al. Transcranial doppler pulsatility index: what it is and what it isn't. Neurocrit Care. (2012) 17:58–66. doi: 10.1007/s12028-012-9672-6
27. Hamilton R, Baldwin K, Fuller J, Vespa P, Hu X, Bergsneider M. Intracranial pressure pulse waveform correlates with aqueductal cerebrospinal fluid stroke volume. J Appl Physiol. (2012) 113:1560–6. doi: 10.1152/japplphysiol.00357.2012
28. Bates D, Mächler M, Bolker B, Walker S. Fitting linear mixed-effects models using lme4. J Statist Softw. (2015) 2015:67. doi: 10.18637/jss.v067.i01
29. Kuznetsova A, Brockhoff PB, Christensen RHB. lmertest package: tests in linear mixed effects models. J Statist Softw. (2017) 2017:82. doi: 10.18637/jss.v082.i13
30. Makowski D. The psycho package: an efficient and publishing-oriented workflow for psychological science. J Open Sour Softw. (2018) 3:470. doi: 10.21105/joss.00470
31. Seabold S, Perktold J. Statsmodels: econometric and statistical modeling with python. In: Proceedings of the 9th Python in Science Conference (Austin, TX) (2010).
32. Farace E, Alves WM. Do women fare worse: a metaanalysis of gender differences in traumatic brain injury outcome. J Neurosurg. (2000) 93:539–45. doi: 10.3171/jns.2000.93.4.0539
33. Niemeier JP, Perrin PB, Holcomb MG, Rolston CD, Artman LK, Lu J, et al. Gender differences in awareness and outcomes during acute traumatic brain injury recovery. J Womens Health. (2014) 23:573–80. doi: 10.1089/jwh.2013.4535
34. Yard EE, Dawn Comstock R. Compliance with return to play guidelines following concussion in US high school athletes, 2005–2008. Brain Inj. (2009) 23:888–98. doi: 10.1080/02699050903283171
35. Kerr ZY, Register-Mihalik JK, Kroshus E, Baugh CM, Marshall SW. Motivations Associated With Nondisclosure of Self-Reported Concussions in Former Collegiate Athletes. Am J Sports Med. (2016) 44:220–5. doi: 10.1177/0363546515612082
36. Emerson CS, Headrick JP, Vink R. Estrogen improves biochemical and neurologic outcome following traumatic brain injury in male rats, but not in females. Brain Res. (1993) 608:95–100. doi: 10.1016/0006-8993(93)90778-L
37. Collins CL, Fletcher EN, Fields SK, Kluchurosky L, Rohrkemper MK, Comstock RD, et al. Neck strength: a protective factor reducing risk for concussion in high school sports. J Prim Prev. (2014) 35:309–19. doi: 10.1007/s10935-014-0355-2
38. Tierney RT, Sitler MR, Swanik CB, Swanik KA, Higgins M, Torg J. Gender differences in head-neck segment dynamic stabilization during head acceleration. Med Sci Sports Exerc. (2005) 37:272–9. doi: 10.1249/01.MSS.0000152734.47516.AA
39. Kriz PK, Stein C, Kent J, Ruggieri D, Dolan E, O'Brien M, et al. Physical maturity and concussion symptom duration among adolescent ice hockey players. J Pediatr. (2016) 171:234–9.e1–2. doi: 10.1016/j.jpeds.2015.12.006
40. Blakemore S-J, Burnett S, Dahl RE. The role of puberty in the developing adolescent brain. Hum Brain Mapp. (2010) 31:926–33. doi: 10.1002/hbm.21052
41. Kim S, Hamilton R, Pineles S, Bergsneider M, Hu X. Noninvasive intracranial hypertension detection utilizing semisupervised learning. IEEE Trans Biomed Eng. (2013) 60:1126–33. doi: 10.1109/TBME.2012.2227477
Keywords: sex differences, traumatic brain injury, vascular reactivity, CBF autoregulation, blood flow
Citation: Thibeault CM, Thorpe S, Canac N, Wilk SJ and Hamilton RB (2019) Sex-Based Differences in Transcranial Doppler Ultrasound and Self-Reported Symptoms After Mild Traumatic Brain Injury. Front. Neurol. 10:590. doi: 10.3389/fneur.2019.00590
Received: 21 February 2019; Accepted: 20 May 2019;
Published: 11 June 2019.
Edited by:
Jack Tsao, University of Tennessee Health Science Center (UTHSC), United StatesReviewed by:
Can Ozan Tan, Harvard Medical School, United StatesRamona E. Von Leden, University of Texas at Austin, United States
Copyright © 2019 Thibeault, Thorpe, Canac, Wilk and Hamilton. This is an open-access article distributed under the terms of the Creative Commons Attribution License (CC BY). The use, distribution or reproduction in other forums is permitted, provided the original author(s) and the copyright owner(s) are credited and that the original publication in this journal is cited, in accordance with accepted academic practice. No use, distribution or reproduction is permitted which does not comply with these terms.
*Correspondence: Corey M. Thibeault, corey@neuralanalytics.com
†These authors have contributed equally to this work