- 1Hotchkiss Brain Institute, Calgary, AB, Canada
- 2Department of Clinical Neurosciences, Cumming School of Medicine, University of Calgary, Calgary, AB, Canada
- 3Department of Radiology, Cumming School of Medicine, University of Calgary, Calgary, AB, Canada
Background: Approximately 25% of concussion patients experience persistent post-concussion symptoms (PPCS). Repetitive transcranial magnetic stimulation (rTMS) has been explored as a treatment, and functional near-infrared spectroscopy (fNIRS) may be a cost-effective method for assessing response.
Objectives: Evaluate rTMS for the treatment of PPCS and introduce fNIRS as a method of assessing treatment response.
Methods: Design: Two-patient case study. Setting: Calgary Brain Injury Program. Participants: 47 and 49 years. male, with PPCS for 1–2 years (headache, cognitive difficulties, nausea, visual difficulties, irritability, anxiety, poor mood, sleep, and fatigue). Intervention: 10 sessions of rTMS therapy to the left dorsolateral prefrontal cortex (DLPFC), at 10 Hz (600 pulses) and 70% of resting motor threshold amplitude. Participants completed an 8-week headache diary and a battery of clinical questionnaires prior to each fNIRS session. fNIRS: Hemodynamic changes were recorded over the frontoparietal cortex during rest, finger tapping, and a graded working memory test. fNIRS was completed pre-rTMS, following rTMS (day 14), and at 1-month post-rTMS (day 45). For comparison, two healthy, sex-matched controls were scanned with fNIRS once daily for five consecutive days.
Results: Clinical scores improved (headache severity, MoCA, HIT-6, PHQ-9, GAD-7, QOLIBRI, RPSQ, BCPSI) or remained stable (PCL-5, headache frequency) post-rTMS, for both participants. Participant 1 reported moderate symptom burden, and a fNIRS task-evoked hemodynamic response showing increased oxyhemoglobin was observed following a working memory task, as expected. Participant 2 exhibited a high symptom burden pre-treatment, with abnormal fNIRS hemodynamic response where oxyhemoglobin declined, in response to task. One month following rTMS treatment, participant 2 had a normal fNIRS hemodynamic response to task, corresponding to significant improvements in clinical outcomes.
Conclusion: This case study suggests fNIRS may be sensitive to physiological changes that accompany rTMS treatment. Further studies exploring fNIRS as a cost-effective technology for monitoring rTMS response in patients with PPCS are suggested.
Background
Annually, up to 280,000 people in Canada (1) and 42 million worldwide (2, 3) experience a mild traumatic brain injury (mTBI). In patients with mTBI, symptoms experienced following injury usually resolve within 3 months. However, up to 25% of patients will experience persistent post-concussion symptoms (PPCS), which can continue up to 1 year following injury (4). Common symptoms include headaches, dizziness, fatigue, irritability, depression, anxiety, emotional lability, concentration or memory difficulties, insomnia, and reduced alcohol tolerance (ICD-10 post-concussion syndrome diagnostic criteria) (5–8).
Repetitive transcranial magnetic stimulation (rTMS) is a non-invasive neurostimulation treatment whereby a rapidly alternating magnetic field applied to the scalp induces an electrical stimulus in a targeted region of the brain (9). This may lead to neuronal depolarization and either excitation or inhibition, depending on the neurons stimulated. Clinically, TMS has been approved by the FDA for treatment-resistant depression (rTMS) (10, 11) and migraine with aura (single pulse TMS) (12) Recent preliminary studies have explored rTMS as a treatment option for PPCS (13–15), although the physiological changes associated with rTMS intervention remain relatively unknown.
Functional near infrared spectroscopy (fNIRS) is a neuroimaging technology that non-invasively measures changes in cerebral tissue oxygenation coupled to neuronal activity. Continuous traces of cerebral tissue oxygenation are recorded and changes in the optical absorption properties of the brain tissue are measured (16). These changes can then be used to map local changes in brain activity, similar to how the blood-oxygenation level-dependent (BOLD) signal is used to measure brain activity in functional MRI studies (17). In comparison to functional MRI, fNIRS is advantageous for clinical application because it is small, portable, and useful in a variety of environments where neuroimaging is not feasible. We propose functional near-infrared spectroscopy (fNIRS) as a cost-effective method for studying rTMS treatment response. In this case study, we utilized fNIRS to explore the relationship between physiological changes in brain function and clinical markers of recovery associated with rTMS treatment in two patients with PPCS.
Objectives
To evaluate the effectiveness of rTMS for the treatment of PPCS and to assess whether fNIRS could be a biomarker of rTMS treatment response.
Patient Histories
Participant #1
A 47-year-old male accountant was seen in the Calgary Brain Injury Program (CBIP) at the Division of Physical Medicine and Rehabilitation Department, Foothills Medical Centre, Calgary, AB, Canada. He was originally referred with a history of persistent symptoms (headache, dizziness, vision, neck pain, nausea, poor sleep, and mood) following mild traumatic brain injury while playing soccer. He had a past medical history of migraine with aura, basal cell carcinoma (removed), and gastroesophageal reflux disorder (GERD).
The patient was hit in the head by a high-speed ball while playing in a soccer game. He did not lose consciousness nor report post traumatic amnesia. He finished playing the game and was later diagnosed with a sport-related concussion (SRC) by a sports medicine physician based on the Consensus statement on Concussion in sport−5th International Conference (18). Initial symptoms included feeling “off and foggy” for the first 3 days, with subsequent development of persisting headaches/head pressure sensations, vision difficulties, fatigue, slowed processing speed and dizziness. Approximately 2–3 weeks after the head injury, he had a CT scan of his head, which was normal. In regard to treatment, he had trialed craniosacral therapy, physiotherapy, vestibular and vision therapy with only mild improvement in his symptoms. As a result, he was on short term disability.
Physical exam at the initial assessment demonstrated evidence of saccades when looking to the left and left eye nystagmus when looking to the right. He had evidence of convergence insufficiency on exam. The remainder of his neurologic exam was normal. Investigations, including neuro-ophthalmology evaluation, CT head, and neuroendocrine testing (CBC, electrolytes, glucose, TSH, free T4, a.m. cortisol) were all within normal limits.
The patient was seen seven times at the CBIP over the course of 16 months with ongoing treatment for headache, neck pain/hyperalgesia, vision, mood and return to work counseling. Despite further treatment with oral pharmacologic medications (trazadone, amitriptyline, desvenlafaxine), topicals, greater occipital nerve blocks, cranial botox injections (PREEMPT protocol) (19), prism glasses, and exercise, he continued to experience PPCS.
Participant #2
A 49-year-old male elementary school teacher was originally referred to the CBIP with a history of PPCS (vision changes, headaches, confusion, slowed thinking, difficulty with multitasking, poor balance, postural dizziness, nausea, emotional lability, fatigue) following a motor vehicle accident. He had a past medical history of a mild TBI (with loss of consciousness) at 19 years old, as well as remote history of lower extremity orthopedic injuries.
The patient was involved in a motor vehicle accident, which occurred at ~50 km/h. The air-bags deployed and his car started on fire. He was unable to remove himself from the vehicle. He did not report amnesia or loss of consciousness, but did experience a sensation of nausea, vision changes, headache, dizziness, and fatigue following the event. He was diagnosed with a concussion by his family doctor in accordance with the World Health Organization Criteria (20), based on alteration in mental state and neurological deficits following the event. He tried to go back to work 3 days after the injury, however reported word-finding difficulties, problems concentrating, poor memory, and confusion. Treatment prior to assessment in the CBIP included acetaminophen, ibuprofen, physiotherapy, massage, and hyperbaric oxygen chamber therapy without significant benefit. The patient was on short-term disability from work as a Grade 5 teacher. He was previously active in Iron Man Triathlons but was unable to train since his accident.
Initial physical exam demonstrated convergence insufficiency. He became symptomatic with eye movement. The rest of his neurologic exam was normal. Investigations, including an MRI of the brain and neuroendocrine testing (CBC, electrolytes, glucose, TSH, free T4, IGF-1, a.m. cortisol, and urine electrolytes) were within normal limits.
He was seen five times at CBIP over the course of 8 months with ongoing treatment for vision, headaches, dizziness, mood, return to exercise, and general rehabilitation (occupational and physical therapy, social work, psychology, and productivity consultant). He was treated with oral medications (sertraline, rizatriptan), prism glasses, vestibular physiotherapy, vision therapy, and personal exercise training with ongoing post-concussion symptoms.
Methods
Design
Two-patient case study.
Setting
Calgary Brain Injury Program, Calgary, Alberta, Canada.
Included Participants
The two included participants, described above, consented to participate in a case study using rTMS and fNIRS for possible treatment of their persistent post traumatic brain injury symptoms. Two male control subjects (19 and 28 years of age, respectively) with no history of concussion or mild TBI underwent five identical fNIRS scans across five consecutive measurement days, in an effort to demonstrate reproducibility of baseline fNIRS measurement. Participant 1 was maintained on oral pharmacologic management with amitriptyline throughout the trial, while participant 2 did not take any oral medications.
Intervention
Ten sessions of rTMS therapy using an air-cooled 70-mm coil (Airfilm; Magstim, Whitland, UK) to the left dorsolateral prefrontal cortex (DLPFC), over 2 weeks, at 10 Hz (600 pulses) and 70% of resting motor threshold amplitude. Participants clinical MRI scans were loaded onto the neuronavigation software platform (Brainsight2, Rogue, Montreal).
Assessments
Clinical questionnaires included the headache impact test-6 (HIT-6), Montreal cognitive assessment (MoCA), patient health questionnaire-9 (PHQ-9), generalized anxiety disorder scale-7 (GAD-7), post-traumatic stress disorder checklist for DSM-5 (PCL-5), quality of life after brain injury questionnaire (QOLIBRI), Rivermead PPCS questionnaire (RPSQ-3, RPSQ-13), and British Columbia post-concussion symptom inventory (BCPSI). fNIRS recordings and clinical questionnaires were completed at baseline, immediately following rTMS (day 14), and at one month (day 45) post-rTMS. An 8-week headache diary documenting frequency and severity was also completed (2 weeks at baseline, during treatment, post-treatment, and 1-month post treatment).
fNIRS
Functional near infrared spectroscopy (fNIRS) scans were recorded at baseline, immediately following rTMS (day 14), and at one month (day 45) post-rTMS to investigate changes in brain physiology associated with rTMS treatment. fNIRS data were recorded over the frontoparietal cortex at a sampling rate of 3.91 Hz, using the NIRScout fNIRS system (NIRx Medical Technologies, Berlin, Germany; Figure 1). Each recording consisted of a 5 min rest period, followed by a finger tapping exercise, and a graded working memory task, previously described by Hocke et al. (21). The fNIRS data was processed and analyzed for task-evoked activation using an ordinary least squares method of general linear modeling, as implemented in the NIRS Brain AnalyzIR Toolbox (22). See Huppert et al. for a detailed discussion of fNIRS principles, acquisition, and analysis (23).
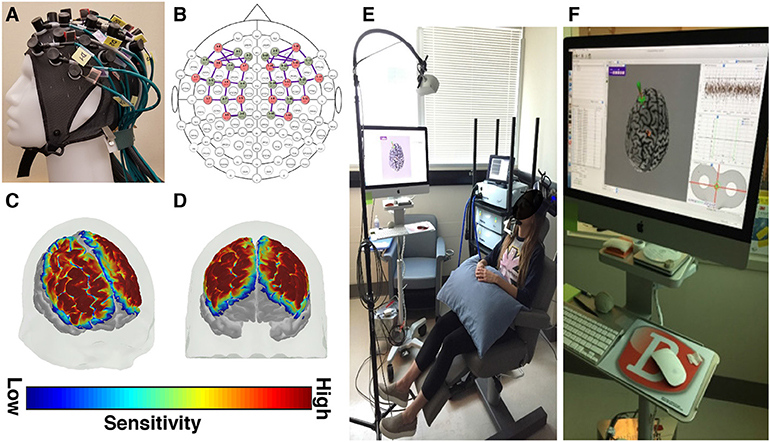
Figure 1. fNIRS and rTMS equipment descriptions. (A) A custom fNIRS headcap and (B) optode configuration was used. The fNIRS headcap was designed to measure tissue oxygenation over fronto-parietal brain areas, including the dorsolateral prefrontal cortex and the primary motor cortex. (C,D) The sensitivity maps (shown here at 2 different angles) depict the areas of the cerebral cortex where tissue oxygenation was recorded, based on the custom optode configuration. These sensitivity maps are created by projecting the simulated photon paths for each fNIRS channel onto a 3D model of the brain. (E) rTMS equipment configuration and (F) rTMS neuronavigational system.
Results
Clinical Scores
Participant 1 reported moderate overall symptom burden at baseline based on symptom scores, with minor improvements in most clinical scores following rTMS (Table 1). Participant 2 had greater symptom burden at baseline, and experienced improvements in all clinical scores, with clinically significant improvements in headache frequency, functional impact, and depression post-rTMS treatment, which persisted at 1-month post-rTMS (day 45; Table 1).
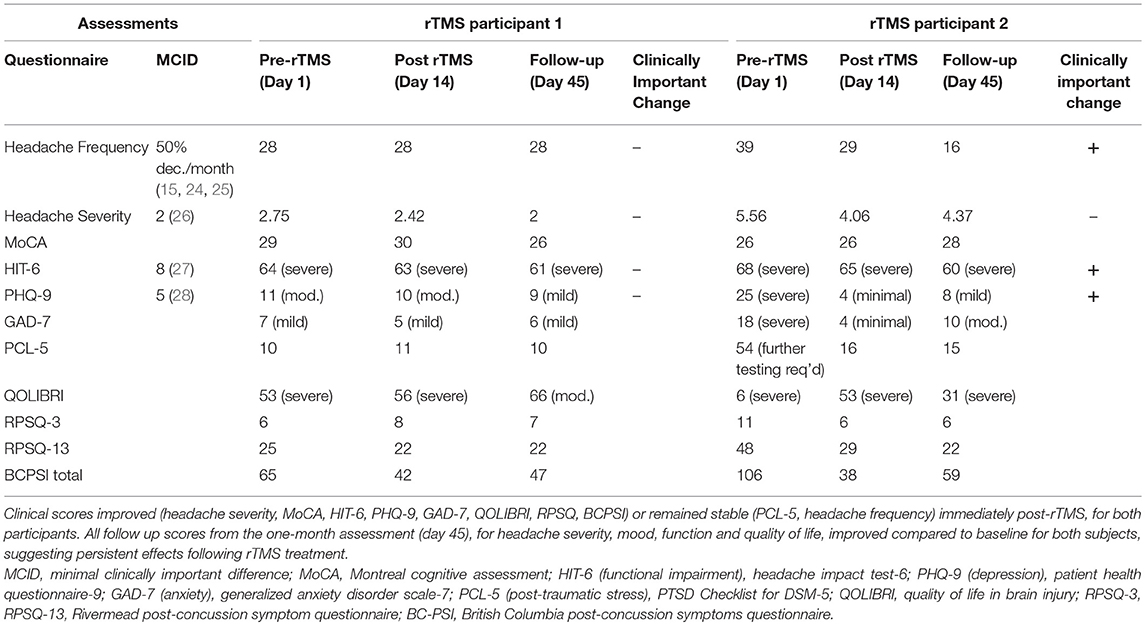
Table 1. Clinical questionnaire outcome measures before treatment (day 1), immediately following rTMS (day 14), and at one-month post treatment (day 45).
Both participants reported decreased headache severity immediately following rTMS and the effect persisted at the 1 month follow up. Headache frequency did not change in participant 1, however, there was a gradual reduction in headache frequency in participant 2. Clinical questionnaire outcomes, including the MoCA, HIT-6, PHQ-9, GAD-7, and QOLIBRI, RPSQ-3, RPSQ-13, and BCPSI all either improved or stayed the same immediately following rTMS treatment for both participants (day 14). Further, follow up scores from the 1-month assessment (day 45) improved compared to baseline for both subjects, suggesting persistent effects on headache severity, function, mood, and quality of life (Table 1). Participant 1 maintained part time work following rTMS and participant 2 was able to return to work at the completion of treatment.
fNIRS
It has been shown previously that working memory tasks evoke a robust hemodynamic response in the DLPFC, characterized by an increase in oxygenated hemoglobin (29, 30). This expected hemodynamic response was observed across 5 measurement days in the controls, highlighting the reproducibility of the activation pattern (Figures 2C,D). In patients with PPCS, participant 1 demonstrated the expected task-evoked hemodynamic response to the working memory task at baseline and both post-rTMS time points (Figure 2A). Interestingly, participant 2 exhibited an abnormal fNIRS hemodynamic response to the working memory test (Figures 2B, 3) whereby oxygenated hemoglobin in the left DLPFC was decreased at the baseline time-point. This response appeared to normalize by the 45-day follow-up.
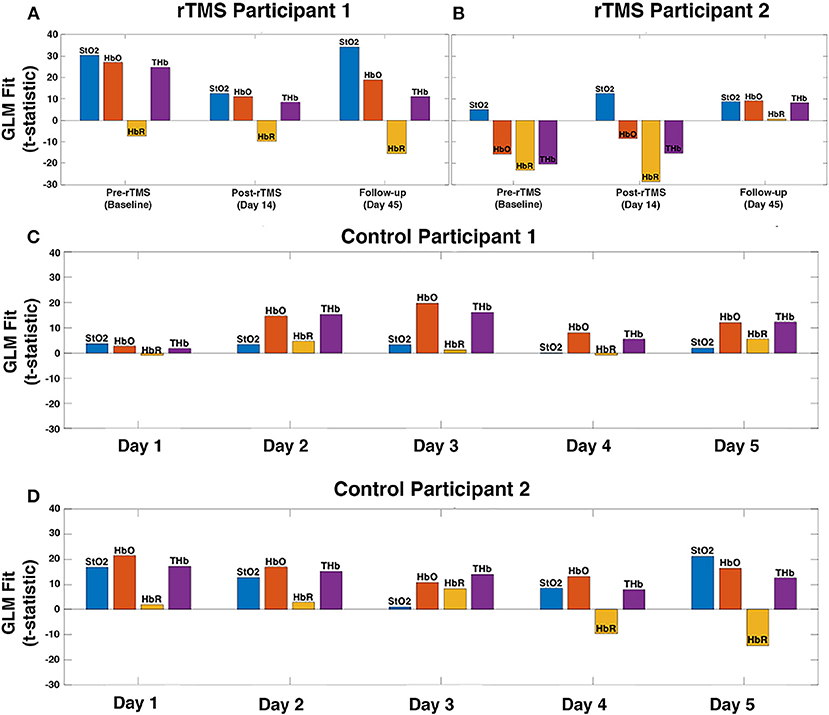
Figure 2. fNIRS measured hemodynamic response to a working memory task in the left DLPFC of patients with persistent post-concussion symptoms (PPCS) who received repetitive transcranial magnetic stimulation (rTMS) treatment (A,B). As an example of the reproducibility of the hemodynamic response pattern to this task, data from two healthy sex-matched controls across five measurement days is presented (C,D). A general linear model (GLM) was used to calculate the location and magnitude of task-evoked changes in oxygen saturation (StO2), oxyhemoglobin (HbO), deoxyhemoglobin (HbR), and total hemoglobin (THb) for each participant. (A) rTMS participant 1 exhibits normal task-evoked hemodynamic response (characterized by a large increase in HbO) at baseline, as well as post-rTMS (day 14 and follow-up day 45). (B) rTMS participant 2 exhibits abnormal task-evoked hemodynamic response (HbO decreased during working memory task execution) at baseline and post-rTMS day 14, however, is normalized 1 month after rTMS treatment (day 45). (C,D) As expected, a consistent hemodynamic response of increased oxygenation was observed in the control subjects across all five measurement days.
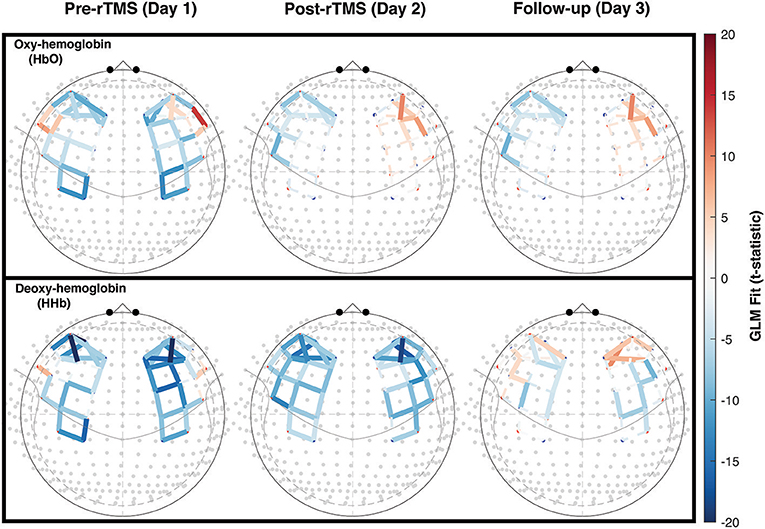
Figure 3. Working memory task-activation color maps for rTMS participant 2, at each time point. Abnormal hemodynamic response (significant deactivation) is detected in the left DLPFC at pre- and post-rTMS timepoints (day 1 and 14) and appears to be normalized (significant activation) by follow-up (day 45). Statistical t-values from the GLM are mapped as red (positive or activation) and blue (negative or deactivation) for each channel pair. Colors increase in intensity as the absolute t-value increases. Solid colored lines represent significance at the level of p < 0.05, after correction for false discovery rate. Broken lines represent non-significant changes.
Discussion
This paper is a preliminary investigation of the feasibility and applicability of using fNIRS to quantitatively assess functional responses to rTMS treatment. Prefrontal cortex rTMS has shown promise as an effective treatment for multiple disorders, particularly depression (10, 11) and headache (12, 31–33) which are both prominent post-concussion symptoms. Consequently, rTMS has been proposed as a treatment for PPCS. Several studies report that rTMS in patients with PPCS may significantly reduce symptoms of headache, depression, dizziness, and improve quality of life (13–15, 34, 35), however these studies are limited by small sample sizes, weak study design, and/or a lack of objective tools to assess treatment response. Furthermore, little is known about the physiological mechanism of how rTMS influences symptoms and function in this population. Therefore, we propose using fNIRS technology to assess possible functional changes in PPCS patients following rTMS intervention.
Treatment of PPCS With rTMS
In this case study, two participants with PPCS who received 10 sessions of rTMS to the left DLPFC reported positive outcomes following treatment, with participant 2 reporting greater than the minimal clinically important difference (MCID) on several questionnaires. There were many similarities between the two participants with regards to the presence of symptoms that were reported at baseline (i.e., headaches, dizziness, vision changes, neck pain, fatigue, and mood difficulties), although, participant 2 reported overall more severe symptom burden prior to rTMS treatment, which may have contributed to their difference in post-rTMS treatment response.
In particular, at baseline, participant 2 had markedly higher headache frequency (39 vs. 28; participant 2 vs. participant 1, respectively), headache severity (5.56 vs. 2.75), mood symptoms (PHQ-9 depression score: 25 vs. 11; GAD-7 anxiety score: 18 vs. 7; PCL-5 post-traumatic stress score: 54 vs. 10), lower quality of life (QOLIBRI: 6 vs. 53), and more severe post-concussion symptom scores (RPSQ-3: 11 vs. 6; RPSQ-13: 48 vs. 25; BCPSI: 106 vs. 65), in comparison to participant 1 (Table 1). At follow-up (45 days post-rTMS intervention) participant 2 reported clinically significant improvements in headache frequency (59% decrease), functional impact of headaches (HIT-6: 8-point reduction), and depression (PHQ-9: 17-point reduction), as well as a large reduction in global post-concussion symptoms and improved quality of life. On the other hand, participant 1 reported improvement on most clinical questionnaire outcomes following rTMS, however changes were below the MCID (Table 1). This case study, although a limited sample, suggests that rTMS treatment response in PPCS patients is variable, and may relate to baseline function.
Despite persistence of lengthy post-concussion symptoms in both participants, there was an immediate positive effect on self-reported symptoms following rTMS treatment (headache severity, depression, anxiety, cognition, functional impact, quality of life). To date, few studies have investigated rTMS for the treatment of PPCS. Koski et al. performed rTMS to the DLPFC in patients with PPCS>3 months following injury (13). Although there were differences in rTMS stimulation protocol compared to our study (20 sessions, 10 Hz, 110% RMT, 600 pulses), they too found a decline in post-concussion symptom scores post-rTMS, which correlated with increased fMRI task-related activation peaks in the DLPFC.
fNIRS as a Tool to Explore rTMS Treatment Response
A number of studies report that chronic headache, depression, and mTBI are associated with alterations in prefrontal brain areas, characterized by reduced gray matter volume and/or cortical thickness, changes in frontal white matter microstructure, as well as altered function in studies of task-response and functional connectivity (36–44). Interestingly, many studies report functional and behavioral improvements following prefrontal rTMS treatment in patients with these disorders (14, 15, 34, 35, 45–49). This has led to the hypothesis that rTMS to the prefrontal cortex can alter its function and microstructure, and result in improved outcomes in patients with PPCS. As a cost-effective neuroimaging tool, functional near infrared spectroscopy (fNIRS) is well suited for assessing functional changes following rTMS treatment and has the potential to add to our understanding of the mechanism by which these changes occur.
fNIRS is a functional imaging technology that measures changes in cortical tissue oxygenation which correspond to local changes in brain metabolism, allowing for portable and non-invasive measurement of functional brain changes (16, 17). Our group has previously shown that fNIRS has the potential to detect alterations in brain function associated with concussion injury (21, 50). In addition to our findings, two other studies suggest that fNIRS is sensitive to altered function in the prefrontal cortex of patients with post-concussion symptoms, particularly while performing cognitive tasks such as visual attention or working memory (21, 51, 52). In this case study, we used fNIRS measures of functional activation in the left DLPFC, in response to a working memory task, as a method to assess baseline function and rTMS treatment response. A normal hemodynamic response recorded by fNIRS during a working memory task is characterized by a robust increase in oxygenated hemoglobin and a far less robust decrease (or no change at all) in deoxygenated hemoglobin, in the DLPFC (29, 30). This normal or expected response of a robust increase in oxygenated hemoglobin is evident in the two control subjects across five measurement time-points, highlighting the reproducibility of this measurement (Figures 2C,D). In addition, total hemoglobin (oxygenated + deoxygenated hemoglobin) and oxygen saturation (oxygenated/total hemoglobin) can also be calculated and are both expected to increase with task-activation.
Pre-treatment, participant 2 (high pre-treatment symptom burden) had a decrease in the oxyhemoglobin response to the working memory task, which is an abnormal task-evoked fNIRS hemodynamic response (29, 30). Forty-five days following the start of the rTMS treatment, participant 2's fNIRS response appeared more comparable to the normal response observed in the controls (Figures 2C,D), which paralleled the significant improvement in clinical scores. In participant 1, who had less severe symptom burden at baseline, we observed an expected task-evoked fNIRS hemodynamic response at baseline (similar to control subjects), as well as post-rTMS treatment (Figure 2A).
The exact mechanism by which rTMS treatment improves brain function is still a matter of debate, although one proposed mechanism that has gained traction is the hypothesis that rTMS helps to re-establish connections in areas of the brain that exhibit dysfunctional activity (53, 54). This hypothesis aligns with our preliminary findings in participant two who had a positive treatment response, after exhibiting high symptom burden and altered fNIRS response prior to treatment. Further, the idea that baseline physiological features may, in part, determine rTMS treatment response was recently explored in a cohort of individuals with depression. The authors of this study report that a baseline decrease in the ratio of blood flow in the dorsolateral prefrontal cortex (DLPFC) relative to the ventromedial prefrontal cortex (VMPFC) was predictive of treatment response (55), suggesting that those who experience the greatest treatment-response have decreased DLPFC cerebral blood flow at baseline. Abnormal vascular coupling or network activation may help to explain the unusual reduction in oxyhemoglobin we observed in participant 2.
Considering this is a case study of two participants, we cannot accurately determine the timeline or trajectory of recovery of fNIRS measured brain function, nor can we conclude with confidence that pre-injury function on fNIRS measures relate to treatment response. These preliminary findings do however suggest that fNIRS could be utilized in future studies as a means to better understand the acute and long term effects of rTMS treatment, and support the hypothesis that pre-treatment baseline function may play a role in who responds best to rTMS intervention. Taken together, this two-patient case study highlights a potential role for fNIRS imaging technology to be used as a tool for assessing rTMS treatment response in patients with PPCS.
Limitations
Potential limitations identified in our study include differences in the mechanism of injury and length of time between concussion to rTMS treatment, for the two participants. Participant 1 received rTMS treatment ~3 years following a sport-related concussion, whereas participant 2 received rTMS treatment 8 months following a motor vehicle accident-related concussion. Pre-injury risk factors for developing post-concussion symptoms, including migraine, depression, life stressors, and personality characteristics may have played a role in persistence of symptoms in the two participants (56). In addition, a limitation of fNIRS is that it is only sensitive to hemodynamic changes in the cerebral cortex, limiting the ability to study deeper brain structures. Further, this study was completed on a small sample size with only one sex (male) represented, and control participants were not age matched to rTMS subjects. Although age-related effects on the magnitude of response to working memory have been observed previously, the direction of the task-effect (increase in oxygenation) does not differ between young and old healthy adults, suggesting the response we observed in participant 2 is unrelated to aging (57). Time since injury and mechanism of injury may factor into rTMS treatment efficacy and should be considered in future trials, along with a larger sample of mTBI patients and including age- and sex-matched controls.
Conclusion
To our knowledge, this is the first study to utilize functional near infrared spectroscopy (fNIRS) to explore rTMS treatment response in participants with persistent post-concussion symptoms. In this pilot trial, both participants reported symptom amelioration and improved quality of life after rTMS. Interestingly, participant 2, who had more severe symptomatology also showed abnormal oxyhemoglobin (hemodynamic) response to a working memory task as quantified with fNIRS. As participant 2's symptoms improved, the fNIRS hemodynamic response also changed to a more typical or expected pattern (increased oxyhemoglobin) during task response.
This study demonstrates the feasibility of examining rTMS treatment response with fNIRS and suggests the possibility of a measurable relationship between the two technologies. fNIRS may be a sensitive tool to predict response to rTMS in patients with PPCS, providing a first step toward utilizing fNIRS as an objective assessment tool in future rTMS trials. To further evaluate rTMS treatment efficacy and gain a greater understanding of the physiological changes underlying rTMS intervention for PPCS, large longitudinal clinical trials with objective assessments at multiple time points are needed. As a cost-effective portable neuroimaging device, fNIRS is well-suited for this role.
Ethics Statement
This was a case study of two patients. We received written consent from these two patients in accordance with the University of Calgary Research Ethics board. However, as there was not a protocol, ethics was not approved for the protocol.
Author Contributions
Conception and study design were completed by CCD, JMS, CTD, and JFD. Data collection was done by CCD, IO, JMS, and EP. Data analysis and interpretation was performed by CCD, JFD, JMS, and CTD. Drafting the article was done by JMS and CCD. Critical revision of the article was completed by all authors. Final approval of the version to be published was done by JMS, CCD, and CTD.
Funding
Canadian Institutes of Health Research project grant (CIHR-CPG-140174) CHRP Grant-Natural Sciences and Engineering Research Council of Canada (NSERC-CHRP/478476-2015). No grant number for Branch Out (Studentship).
Conflict of Interest Statement
The authors declare that the research was conducted in the absence of any commercial or financial relationships that could be construed as a potential conflict of interest.
Acknowledgments
We would like to thank Trevor Low for assistance with rTMS administration.
References
1. Taylor CA, Bell JM, Breiding MJ, Xu L. Traumatic brain injury-related emergency department visits, hospitalizations, and deaths - United States, 2007 and 2013. Morbidity Mortality Wkly Rep Surv Summ. (2017) 66:1–16. doi: 10.15585/mmwr.ss6609a1
2. Gardner RC, Yaffe K. Epidemiology of mild traumatic brain injury and neurodegenerative disease. Mol Cell Neurosci. (2015) 66:75–80. doi: 10.1016/j.mcn.2015.03.001
3. Cassidy JD, Carroll L, Peloso P, Borg J, Von Holst H, Holm L, et al. Incidence, risk factors and prevention of mild traumatic brain injury: results of the WHO Collaborating Centre Task Force on Mild Traumatic Brain Injury. J Rehab Med. (2004) 43:28–60. doi: 10.1080/16501960410023732
4. McMahon P, Hricik A, Yue JK, Puccio AM, Inoue T, Lingsma HF, et al. Symptomatology and functional outcome in mild traumatic brain injury: results from the prospective track-TBI study. J Neurotrauma. (2014) 31:26–33. doi: 10.1089/neu.2013.2984
5. Kashluba S, Casey JE, Paniak C. Evaluating the utility of ICD-10 diagnostic criteria for postconcussion syndrome following mild traumatic brain injury. J Int. Neuropsychol Soc. (2006) 12:111–8. doi: 10.1017/S1355617706060036
6. World Health Organization. The ICD-10 Classification of Mental and Behavioural Disorders: Clinical Descriptions and Diagnostic Guidelines, Section F07.2. Geneva: World Health Organization (1992).
7. Boake C, McCauley SR, Levin HS, Pedroza C, Contant CF, Song JX, et al. Diagnostic criteria for postconcussional syndrome after mild to moderate traumatic brain injury. J Neuropsychiatry Clin Neurosci. (2005) 17:350–6. doi: 10.1176/jnp.17.3.350
8. Mayer AR, Quinn DK, Master CL. The spectrum of mild traumatic brain injury: a review. Neurology. (2017) 89:623–32. doi: 10.1212/WNL.0000000000004214
9. Hallett M Transcranial magnetic stimulation and the human brain. Nature. (2000) 406:147–50. doi: 10.1038/35018000
10. O'Reardon JP, Solvason HB, Janicak PG, Sampson S, Isenberg KE, Nahas Z, et al. Efficacy and safety of transcranial magnetic stimulation in the acute treatment of major depression: a multisite randomized controlled trial. Biol Psychiatry. (2007) 62:1208–16. doi: 10.1016/j.biopsych.2007.01.018
11. Brunoni AR, Chaimani A, Moffa AH, Razza LB, Gattaz WF, Daskalakis ZJ, et al. Repetitive transcranial magnetic stimulation for the acute treatment of major depressive episodes: a systematic review with network meta-analysis. JAMA Psychiatry. (2017) 74:143–52. doi: 10.1001/jamapsychiatry.2016.3644
12. Lipton RB, Dodick DW, Silberstein SD, Saper JR, Aurora SK, Pearlman SH, et al. Single-pulse transcranial magnetic stimulation for acute treatment of migraine with aura: a randomised, double-blind, parallel-group, sham-controlled trial. Lancet Neurol. (2010) 9:373–80. doi: 10.1016/S1474-4422(10)70054-5
13. Koski L, Kolivakis T, Yu C, Chen JK, Delaney S, Ptito A. Noninvasive brain stimulation for persistent postconcussion symptoms in mild traumatic brain injury. J Neurotrauma. (2015) 32:38–44. doi: 10.1089/neu.2014.3449
14. Leung A, Metzger-Smith V, He Y, Cordero J, Ehlert B, Song D, et al. Left dorsolateral prefrontal cortex rTMS in alleviating MTBI related headaches and depressive symptoms. Neuromodulation. (2017) 21:390–401. doi: 10.1111/ner.12615
15. Leung A, Shukla S, Fallah A, Song D, Lin L, Golshan S, et al. Repetitive transcranial magnetic stimulation in managing mild traumatic brain injury-related headaches. Neuromodulation. (2016) 19:133–41. doi: 10.1111/ner.12364
16. Scholkmann F, Kleiser S, Metz AJ, Zimmermann R, Mata Pavia J, Wolf U, et al. A review on continuous wave functional near-infrared spectroscopy and imaging instrumentation and methodology. Neuroimage. (2014) 85(Pt 1):6–27. doi: 10.1016/j.neuroimage.2013.05.004
17. Kleinschmidt A, Obrig H, Requardt M, Merboldt KD, Dirnagl U, Villringer A, et al. Simultaneous recording of cerebral blood oxygenation changes during human brain activation by magnetic resonance imaging and near-infrared spectroscopy. J Cereb Blood Flow Metab. (1996) 16:817–26. doi: 10.1097/00004647-199609000-00006
18. McCrory P, Meeuwisse W, Dvorak J, Aubry M, Bailes J, Broglio S, et al. Consensus statement on concussion in sport-the 5(th) international conference on concussion in sport held in Berlin, October 2016. Br J Sports Med. (2017) 51:838–47. doi: 10.1136/bjsports-2017-097699
19. Dodick DW, Turkel CC, DeGryse RE, Aurora SK, Silberstein SD, Lipton RB, et al. OnabotulinumtoxinA for treatment of chronic migraine: pooled results from the double-blind, randomized, placebo-controlled phases of the PREEMPT clinical program. Headache. (2010) 50:921–36. doi: 10.1111/j.1526-4610.2010.01678.x
20. Ruff RM, Iverson GL, Barth JT, Bush SS, Broshek DK. Methodological issues and research recommendations for mild traumatic brain injury: the WHO Collaborating Centre Task Force on Mild Traumatic Brain Injury. J Rehabil Med. (2004) 43:113–25.
21. Hocke LM, Duszynski CC, Debert CT, Dleikan D, Dunn JF. Reduced functional connectivity in adults with persistent post-concussion symptoms: a functional near-infrared spectroscopy study. J Neurotrauma. (2018) 35:1224–32. doi: 10.1089/neu.2017.5365
22. Santosa H, Zhai X, Fishburn F, Huppert T. The NIRS brain AnalyzIR toolbox. Algorithms. (2018) 11:73. doi: 10.3390/a11050073
23. Huppert TJ, Diamond SG, Franceschini MA, Boas DA. HomER: a review of time-series analysis methods for near-infrared spectroscopy of the brain. Appl Optics. (2009) 48:D280–8. doi: 10.1364/AO.48.00D280
24. Kalita J, Laskar S, Bhoi SK, Misra UK. Efficacy of single versus three sessions of high rate repetitive transcranial magnetic stimulation in chronic migraine and tension-type headache. J Neurol. (2016) 263:2238–46. doi: 10.1007/s00415-016-8257-2
25. Schoenen J. Guidelines for trials of drug treatments in tension-type headache. First edition: International Headache Society Committee on Clinical Trials. Cephalalgia. (1995) 15:165–79. doi: 10.1046/j.1468-2982.1995.015003165.x
26. Farrar JT, Young JP Jr, LaMoreaux L, Werth JL, Poole RM. Clinical importance of changes in chronic pain intensity measured on an 11-point numerical pain rating scale. Pain. (2001) 94:149–58. doi: 10.1016/S0304-3959(01)00349-9
27. Castien RF, Blankenstein AH, Windt DA, Dekker J. Minimal clinically important change on the Headache Impact Test-6 questionnaire in patients with chronic tension-type headache. Cephalalgia. (2012) 32:710–4. doi: 10.1177/0333102412449933
28. Lowe B, Schenkel I, Carney-Doebbeling C, Gobel C. Responsiveness of the PHQ-9 to psychopharmacological depression treatment. Psychosomatics. (2006) 47:62–7. doi: 10.1176/appi.psy.47.1.62
29. Leon-Dominguez U, Martin-Rodriguez JF, Leon-Carrion J. Executive n-back tasks for the neuropsychological assessment of working memory. Behav Brain Res. (2015) 292:167–73. doi: 10.1016/j.bbr.2015.06.002
30. Owen AM, McMillan KM, Laird AR, Bullmore E. N-back working memory paradigm: a meta-analysis of normative functional neuroimaging studies. Hum Brain Mapp. (2005) 25:46–59. doi: 10.1002/hbm.20131
31. Brighina F, Piazza A, Vitello G, Aloisio A, Palermo A, Daniele O, et al. rTMS of the prefrontal cortex in the treatment of chronic migraine: a pilot study. J Neurol Sci. (2004) 227:67–71. doi: 10.1016/j.jns.2004.08.008
32. Misra UK, Kalita J, Bhoi SK. High-rate repetitive transcranial magnetic stimulation in migraine prophylaxis: a randomized, placebo-controlled study. J Neurol. (2013) 260:2793–801. doi: 10.1007/s00415-013-7072-2
33. Stilling JM, Monchi O, Amoozegar F, Debert CT. Transcranial magnetic and direct current stimulation (TMS/tDCS) for the treatment of headache: a systematic review. Headache. (2019) 59:339–57. doi: 10.1111/head.13479
34. Leung A, Fallah A, Shukla S, Lin L, Tsia A, Song D, et al. rTMS in alleviating mild TBI related headaches–a case series. Pain Phys. (2016) 19:E347–54.
35. Paxman E, Stilling J, Mercier L, Debert CT. Repetitive transcranial magnetic stimulation (rTMS) as a treatment for chronic dizziness following mild traumatic brain injury. BMJ Case Rep. (2018) 2018:bcr-2018-226698. doi: 10.1136/bcr-2018-226698
36. Eierud C, Craddock RC, Fletcher S, Aulakh M, King-Casas B, Kuehl D, et al. Neuroimaging after mild traumatic brain injury: review and meta-analysis. Neuroimage Clin. (2014) 4:283–94. doi: 10.1016/j.nicl.2013.12.009
37. Rogers MA, Kasai K, Koji M, Fukuda R, Iwanami A, Nakagome K, et al. Executive and prefrontal dysfunction in unipolar depression: a review of neuropsychological and imaging evidence. Neurosci Res. (2004) 50:1–11. doi: 10.1016/j.neures.2004.05.003
38. Yang Q, Wang Z, Yang L, Xu Y, Chen LM. Cortical thickness and functional connectivity abnormality in chronic headache and low back pain patients. Hum Brain Mapp. (2017) 38:1815–32. doi: 10.1002/hbm.23484
39. Chen WT, Chou KH, Lee PL, Hsiao FJ, Niddam DM, Lai KL, et al. Comparison of gray matter volume between migraine and “strict-criteria” tension-type headache. J Headache Pain. (2018) 19:4. doi: 10.1186/s10194-018-0834-6
40. Ma M, Zhang J, Chen N, Guo J, Zhang Y, He L. Exploration of intrinsic brain activity in migraine with and without comorbid depression. J Headache Pain. (2018) 19:48. doi: 10.1186/s10194-018-0876-9
41. George MS, Ketter TA, Post RM. Prefrontal cortex dysfunction in clinical depression. Depression. (1994) 2:59–72. doi: 10.1002/depr.3050020202
42. Leung A, Yang E, Lim M, Metzger-Smith V, Theilmann R, Song D, et al. Pain-related white matter tract abnormalities in mild traumatic brain injury patients with persistent headache. Mol Pain. (2018) 14:1744806918810297. doi: 10.1177/1744806918810297
43. Sacchet MD, Gotlib IH. Myelination of the brain in major depressive disorder: an in vivo quantitative magnetic resonance imaging study. Sci Rep. (2017) 7:2200. doi: 10.1038/s41598-017-02062-y
44. Obermann M, Nebel K, Schumann C, Holle D, Gizewski ER, Maschke M, et al. Gray matter changes related to chronic posttraumatic headache. Neurology. (2009) 73:978–83. doi: 10.1212/WNL.0b013e3181b8791a
45. Blumberger DM, Vila-Rodriguez F, Thorpe KE, Feffer K, Noda Y, Giacobbe P, et al. Effectiveness of theta burst versus high-frequency repetitive transcranial magnetic stimulation in patients with depression (THREE-D): a randomised non-inferiority trial. Lancet. (2018) 391:1683–92. doi: 10.1016/S0140-6736(18)30295-2
46. Conforto AB, Amaro E, Gonçalves AL, Mercante JP, Guendler VZ, Ferreira JR, et al. Randomized, proof-of-principle clinical trial of active transcranial magnetic stimulation in chronic migraine. Cephalalgia. (2014) 34:464–72. doi: 10.1177/0333102413515340
47. Siddiqi SH, Trapp NT, Hacker CD, Laumann TO, Kandala S, Hong X, et al. Repetitive transcranial magnetic stimulation with resting state network targeting for treatment-resistant depression in traumatic brain injury: a randomized, controlled, double blinded pilot study. J Neurotrauma. (2018) 36:1361–74. doi: 10.1089/neu.2018.5889
48. Reti IM, Schwarz N, Bower A, Tibbs M, Rao V. Transcranial magnetic stimulation: a potential new treatment for depression associated with traumatic brain injury. Brain Inj. (2015) 29:789–97. doi: 10.3109/02699052.2015.1009168
49. Hsu JH, Daskalakis ZJ, Blumberger DM. An update on repetitive transcranial magnetic stimulation for the treatment of co-morbid pain and depressive symptoms. Curr Pain Headache Rep. (2018) 22:51. doi: 10.1007/s11916-018-0703-7
50. Urban KJ, Barlow KM, Jimenez JJ, Goodyear BG, Dunn JF. Functional near-infrared spectroscopy reveals reduced interhemispheric cortical communication after pediatric concussion. J Neurotrauma. (2015) 32:833–40. doi: 10.1089/neu.2014.3577
51. Kontos AP, Huppert TJ, Beluk NH, Elbin RJ, Henry LC, French J, et al. Brain activation during neurocognitive testing using functional near-infrared spectroscopy in patients following concussion compared to healthy controls. Brain Imag Behav. (2014) 8:621–34. doi: 10.1007/s11682-014-9289-9
52. Wu Z, Mazzola CA, Catania L, Owoeye O, Yaramothu C, Alvarez T, et al. Altered cortical activation and connectivity patterns for visual attention processing in young adults post-traumatic brain injury: a functional near infrared spectroscopy study. CNS Neurosci Therapeut. (2018) 24:539–48. doi: 10.1111/cns.12811
53. Anderson RJ, Hoy KE, Daskalakis ZJ, Fitzgerald PB. Repetitive transcranial magnetic stimulation for treatment resistant depression: Re-establishing connections. Clin Neurophysiol. (2016) 127:3394–405. doi: 10.1016/j.clinph.2016.08.015
54. Taïb S, Arbus C, Sauvaget A, Sporer M, Schmitt L, Yrondi A. How does repetitive transcranial magnetic stimulation influence the brain in depressive disorders?: A review of neuroimaging magnetic resonance imaging studies. J ECT. (2018) 34:79–86. doi: 10.1097/YCT.0000000000000477
55. Kito S, Hasegawa T, Koga Y. Cerebral blood flow ratio of the dorsolateral prefrontal cortex to the ventromedial prefrontal cortex as a potential predictor of treatment response to transcranial magnetic stimulation in depression. Brain Stimul. (2012) 5:547–53. doi: 10.1016/j.brs.2011.09.004
56. Wäljas M, Iverson GL, Lange RT, Hakulinen U, Dastidar P, Huhtala H, et al. A prospective biopsychosocial study of the persistent post-concussion symptoms following mild traumatic brain injury. J Neurotrauma. (2015) 32:534–47. doi: 10.1089/neu.2014.3339
Keywords: concussion, post-concussion symptom, transcranial magnetic stimulation (repetitive), functional Near Infrared Spectroscopy (fNIRS), rehabilitation
Citation: Stilling JM, Duszynski CC, Oni I, Paxman E, Dunn JF and Debert CT (2019) Using Functional Near-Infrared Spectroscopy to Study the Effect of Repetitive Transcranial Magnetic Stimulation in Concussion: A Two-Patient Case Study. Front. Neurol. 10:476. doi: 10.3389/fneur.2019.00476
Received: 15 February 2019; Accepted: 23 April 2019;
Published: 08 May 2019.
Edited by:
Karen M. Barlow, University of Queensland, AustraliaReviewed by:
Joukje Van Der Naalt, University Medical Center Groningen, NetherlandsPaolo Tonin, Sant'Anna Institute, Italy
Copyright © 2019 Stilling, Duszynski, Oni, Paxman, Dunn and Debert. This is an open-access article distributed under the terms of the Creative Commons Attribution License (CC BY). The use, distribution or reproduction in other forums is permitted, provided the original author(s) and the copyright owner(s) are credited and that the original publication in this journal is cited, in accordance with accepted academic practice. No use, distribution or reproduction is permitted which does not comply with these terms.
*Correspondence: Chantel T. Debert, Q2hhbnRlbC5EZWJlcnRAYWxiZXJ0YWhlYWx0aHNlcnZpY2VzLmNh
†These authors have contributed equally to this work