- 1Department of Neurology, Samsung Medical Center, Sungkyunkwan University School of Medicine, Seoul, South Korea
- 2Department of Radiology, Severance Hospital Stroke Center, Yonsei University College of Medicine, Seoul, South Korea
- 3Department of Radiology, Samsung Medical Center, Sungkyunkwan University School of Medicine, Seoul, South Korea
Large vessel occlusion (LVO) due to intracranial atherosclerosis (ICAS) is a common cause of acute ischemic stroke (AIS) in Asians. Endovascular therapy (EVT) has been established as the mainstay of treatment in patients with AIS and LVO. However, only a few patients of Asian descent with ICAS-related LVO (ICAS-LVO) were included in recent randomized controlled trials of EVT for AIS. Therefore, the findings of these trials cannot be directly applied to Asian patients with ICAS-LVO. In embolic LVO due to thrombus from the heart or a more proximal vessel, rapid, and complete recanalization can be achieved in more than 70–80% of patients, and it is important to exclude patients with large cores. In contrast, patients with ICAS-LVO usually have favorable hemodynamic profiles (good collateral status, small core, and less severe perfusion deficit), but poor response to EVT (more rescue treatments and longer procedure times are required for successful recanalization due to higher rates of reocclusion). Patients with ICAS-LVO may have different anatomic (plaque, angioarchitecture), hemodynamic (collateral status), and pathophysiologic (thrombus composition) features on neuroimaging compared to patients with embolic LVO. In this review, we discuss these neuroimaging features, their clinical implications with respect to determination of EVT responses, and the need for development of specific EVT devices and procedures for patients with ICAS-LVO.
Introduction
Large vessel occlusion (LVO), thought to originate from intracranial atherosclerosis (ICAS), is a common cause of acute ischemic stroke (AIS) in Asians (1). Embolic LVO due to thrombus from the heart or a more proximal vessel and ICAS-related LVO (ICAS-LVO) both show similar luminal changes and are treated with endovascular therapy (EVT) in acute settings. However, recent clinical studies suggest that treatment responses may differ between these two types of LVO (2–10). Patients with ICAS-LVO may have different anatomic (plaque, angioarchitecture), hemodynamic (collateral status), and pathophysiologic (thrombus composition) features on neuroimaging compared to patients with embolic LVO.
In this review, we discuss these features, their clinical implications with respect to determination of EVT responses, and the need for development of specific EVT devices and procedures for patients with ICAS-LVO.
Search Strategy and Selection Criteria
We searched PubMed and ClinicalTrials.gov for articles published in English up to September 2018 using the following search terms: stroke, cerebrovascular disease, endovascular therapy, and intracranial stenosis. We also searched references from relevant articles and reviews. The final reference list was generated based on originality and relevance to this topic. We did not discuss individual imaging techniques or etiologies of non-atherosclerotic intracranial arterial disease in depth, since these topics are reviewed elsewhere (11–18).
ICAS-LVO in Recent Randomized Controlled Trials of EVT for AIS
Phase III, randomized controlled trials (RCTs) conducted in 2015 demonstrated overwhelming evidence of the benefit of early window EVT for treatment of AIS with small core and LVO (19–23). More recently, the results of phase III RCTs of EVT in extended time windows showed significant and remarkable functional recovery after EVT compared to medical treatment in carefully selected patients (24, 25). In individual patient data meta-analyses of RCTs, the benefits of EVT were consistent in all prespecified subgroups of age, sex, initial stroke severity score, site of vessel occlusion, presence of tandem occlusion, extent of initial early ischemic changes on computed tomography (CT), intravenous tissue plasminogen activation (tPA), and onset-to-randomization time (26, 27). However, the type of LVO was not considered in the RCTs, and the number of patients with ICAS-LVO was small considering that only few Asian patients were enrolled in the 2 RCTs (20, 22).
The results of EVT in patients with ICAS-LVO are shown in Table 1. Recanalization failure, residual stenosis, and reocclusion were more frequently observed than embolic occlusion and rescue therapy with permanent stent placement or adjuvant antithrombotics are often required after EVT in ICAS-LVO patients (2–4, 9). Consequently, longer procedure times were required and higher complication rates and poorer long-term outcomes were reported after EVT in patients with ICAS-LVO than in those with embolic occlusion (5, 6, 8). Therefore, the results of the phase III RCTs of EVT cannot be directly applied to patients with ICAS-LVO.
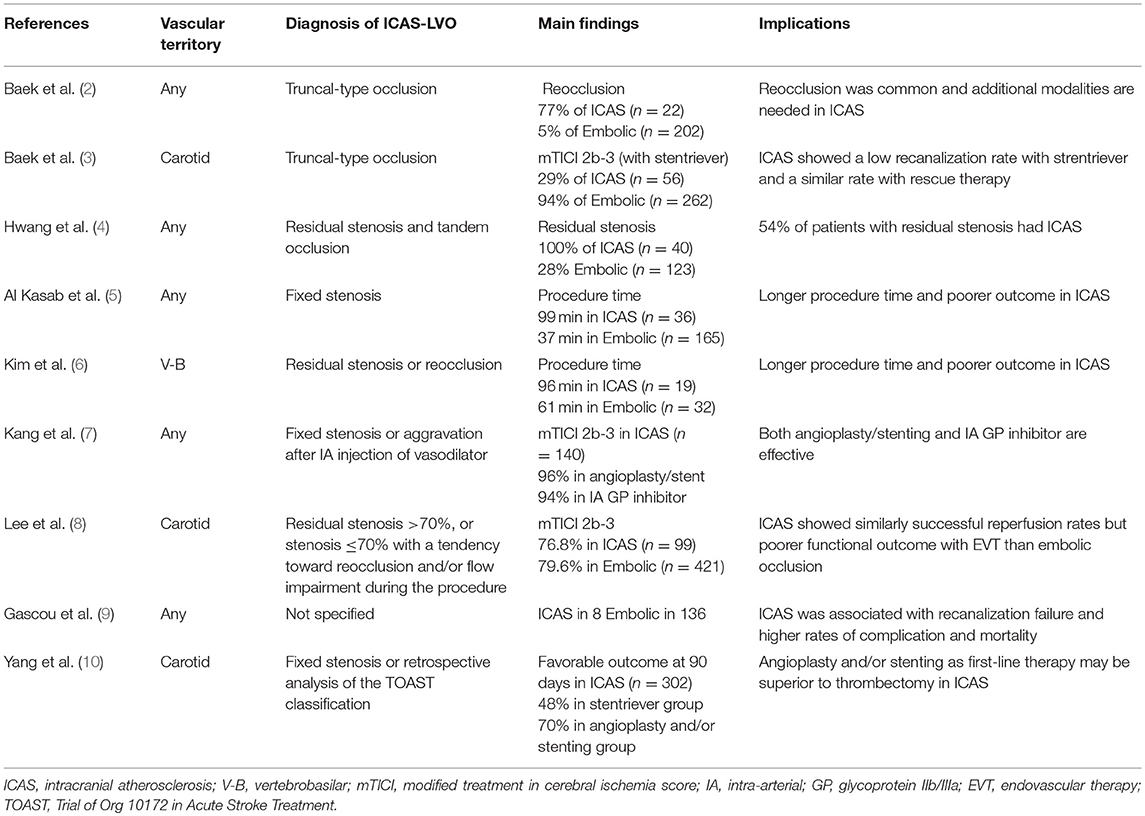
Table 1. Summary of literature on angiographic features suggesting large vessel occlusion of suspected intracranial atherosclerotic origin and outcomes after endovascular therapy.
Diagnosis of Suspected ICAS-LVO
Differentiation of ICAS-LVO from embolic LVO is often challenging, especially in cases without known ICAS and in the setting of EVT for AIS when workups for potential sources of cardioembolism cannot be performed (Figure 1). Several clinical features may be helpful for differentiating ICAS-LVO from embolic LVO (28). Although advanced magnetic resonance imaging (MRI) techniques may provide information on the ischemic zone, thrombus, blood-brain barrier, and vessel wall pathology (29), only non-contrast brain CT and conventional angiographic techniques are available to differentiate these two types of LVOs in most centers.
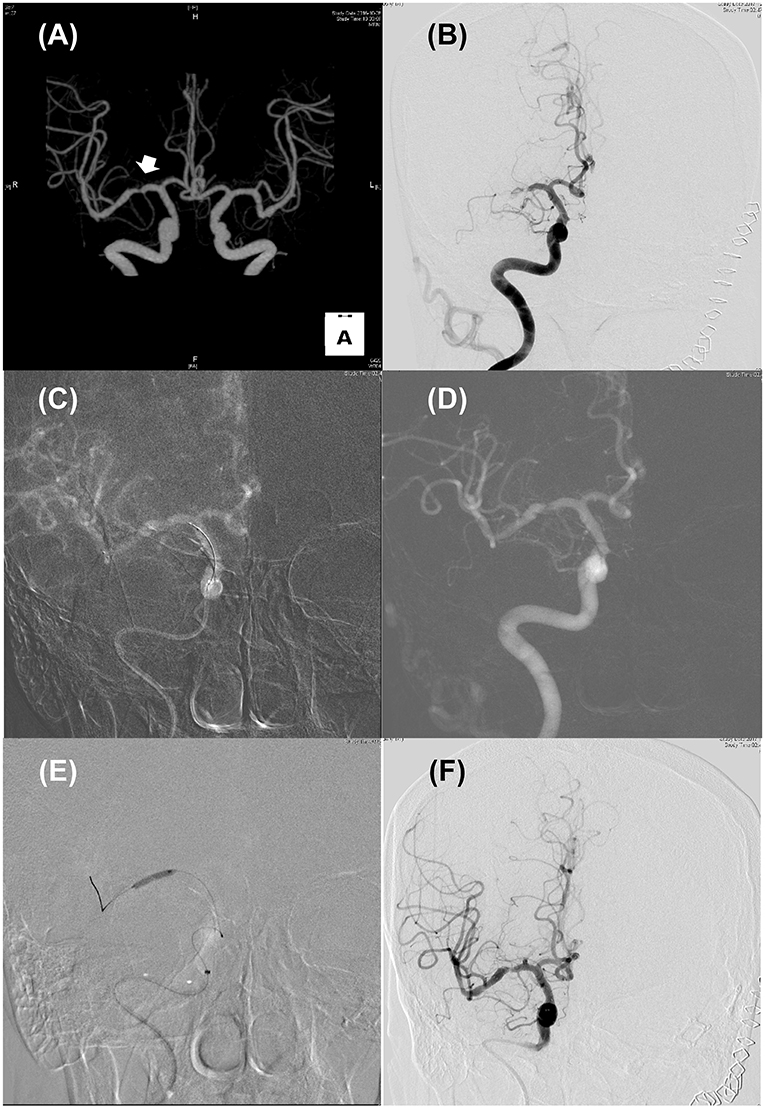
Figure 1. Illustrated case for the management of acute stroke due to intracranial atherosclerosis. (A) CT angiography performed 2 years ago revealed focal stenosis on right mid-MCA (Arrow). (B) Initial internal carotid angiography showed truncal-type occlusive lesion on right mid-MCA with minimal blood flow across the occlusive lesion. (C,D) Roadmap images during solitaire stent (4 × 20 mm) placement (C) and after retrieval (D). Pre-existing stenotic lesion still be seen. (E) Balloon angioplasty using Gateway TPA balloon (2 × 15 mm; Boston scientific) was performed. (F) Delayed carotid angiography 30 min after permanent solitaire stent placement. Despite residual stenosis, improved distal flow can be seen.
As shown in Table 1, most investigators used angiographic features for the diagnosis of ICAS-LVO. Baek et al. defined ICAS-LVO as truncal-type occlusion when all major branches and their bifurcation sites are clearly visible beyond the occlusion segment (2, 3). Other investigators considered angiographic findings of residual or fixed stenosis to be ICAS-LVO (4–8).
The prevalence of ICAS-LVO was reported to range from 5.5 to 25%. The prevalence of ICAS in EVT candidates varied depending on the diagnostic methods for ICAS-LVO and race or ethnicity (1, 9).
Features of ICAS-LVO
ICAS-LVO has more differentiating features than embolic LVO, which are discussed below (Table 2).
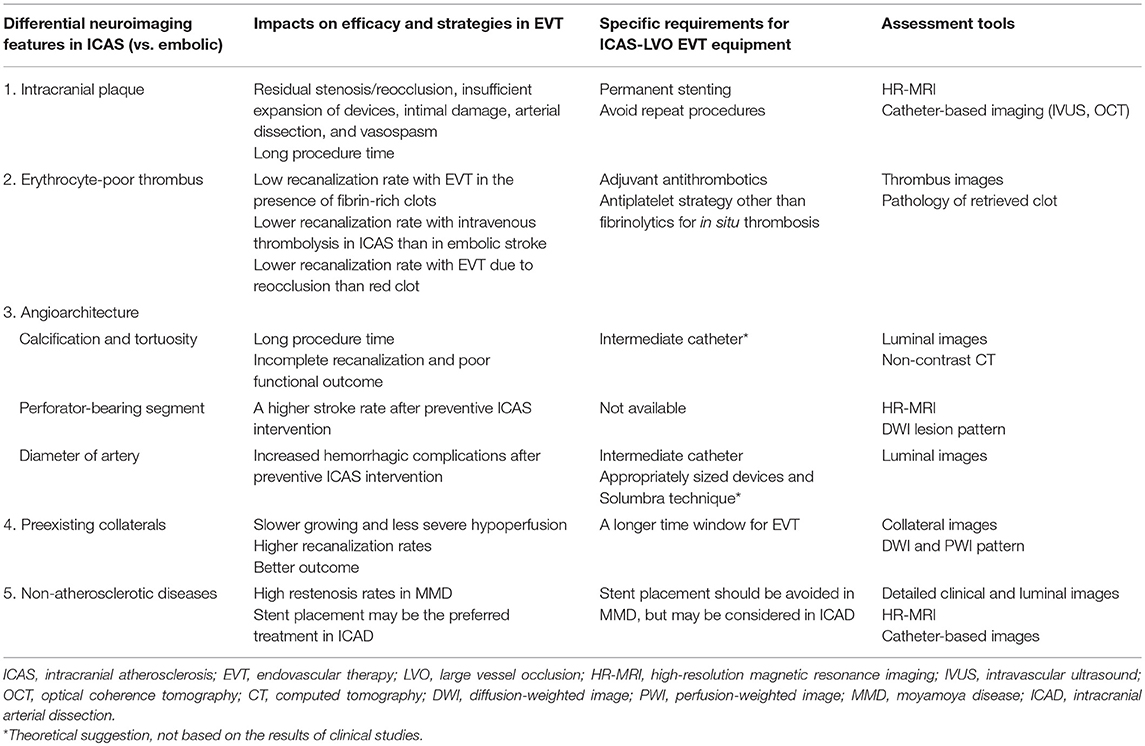
Table 2. Neuroimaging features and specific considerations in endovascular therapy for large vessel occlusions of intracranial atherosclerotic origin.
Intracranial Plaque
The presence of intracranial plaques can influence endovascular procedures and affect outcome. EVT for ICAS-LVO is associated with residual stenosis or reocclusion, insufficient expansion of devices, inadvertent detachment, arterial dissection, and vasospasm (4, 5, 30). Therefore, repeat procedures and long procedure times are often necessary for successful reperfusion. They are also associated with poor clinical outcomes (2, 6). Repeated stent retrieval attempts, especially in the presence of a plaque at the LVO site, can further damage the fibrous cap and lead to aggravation of in situ thrombosis. In western trials, ICAS was less prevalent and early reocclusion after successful reperfusion with EVT was rare (31).
Perforator
In preventive intervention for ICAS, the incidence of symptomatic complications was high after intracranial stenting for perforator-bearing segments or in patients with branch occlusive disease (BOD) with subcortical infarcts caused by occluding the perforator orifice (32–34). The involved segment was more diffuse and positive remodeling was less frequently observed in BOD-type ICAS than in non-BOD-type ICAS (35, 36). The complication rates of EVT may also be increased in patients with AIS and LVO in the perforator-bearing segments, especially when permanent stent placement is required. Therefore, increased complication rate with the permanent placement of stent in the perforator bearing segment should be considered, especially in the setting of EVT for LVO when appropriate antiplatelet premedication before the procedure is not possible. Further studies are needed because a higher peri-procedural ischemic stroke rate was reported in the treatment of perforator-bearing arteries, and there was no difference between angioplasty alone and balloon mounted/self-expandable stenting (33).
Arterial Diameter
ICAS-LVO often involves smaller-sized vessels than clots that originated from the heart (such as red clots in atrial fibrillation occluding the distal internal carotid artery). Moreover, the ring finger protein 213 (RNF213) gene variant, the most susceptible gene for moyamoya in Asians, was found in 1 in 4 Japanese and Korean patients with non-moyamoya intracranial stenosis (37, 38). Hongo et al. reported that patients with ICAS and RNF213 variants had middle cerebral arteries with relatively smaller outer diameter (2.09 ± 0.32 mm) (39). The results of the RCT of the Stenting and Aggressive Medical Management for Preventing Recurrent Stroke in Intracranial Arterial Stenosis showed that treating very small vessels (<2.5–2.75 mm diameter) was associated with higher complication rates, because small vessels are more likely to have restenosis or acute thrombosis and they may also be more prone to injury with stenting (32).
Calcification And Tortuosity
Patients with ICAS may have stiff, calcified, and tortuous vessels. In these patients, a longer time may be required to reach the target site and incomplete recanalization and poor functional outcomes were reported (40). A post-hoc analysis of a RCT showed that the type of intracranial arterial calcification determined the effect of EVT for AIS (41).
Thrombus
Blood flow affects thrombus composition, with “red clots” or erythrocyte-rich thrombi found in low-pressure systems (heart or venous system), and “white clots” or platelet-rich thrombi found in high pressure systems (e.g., arteries) (42). The composition and burden of clot correlate with revascularization rate in EVT. Fibrin-rich thrombi have higher coefficients of static friction with the vessel walls, and larger thrombi have larger surface areas of thrombus-vessel interaction (43). Treatment response to medical treatment (such as tPA and glycoprotein IIb/IIIa inhibitors) and EVT may vary for ICAS-LVO and embolic occlusion. The thrombus size is usually smaller in ICAS-LVO than in embolic LVO, but the recanalization rates with EVT or tPA were lower in the former than in the latter (43–46). A histopathologic analysis of retrieved thrombi showed that atheromatous gruel (cholesterol clefts, form cells, or fibrous caps) was associated with failed recanalization, and erythrocyte-rich thrombi were associated with successful recanalization (45). In ICAS-LVO cases, adjuvant glycoprotein IIb/IIIa inhibitors for in situ thrombosis or angioplasty with/without permanent stent placement may be helpful (2, 3). However, beside stroke subtypes, other factors also influence the characteristics of thrombi, such as collaterals and angioarchitecture (44, 47). In addition, in patients with coronary atherosclerotic plaques, growing thrombi consist of both platelet-rich and erythrocyte-rich clots, and thrombus stability also determines the response to revascularization therapy (48).
Collaterals
The importance of collateral status has been reported in preventive RCTs of ICAS patients and in acute interventional RCTs (49–52). Although the individual patient data meta-analysis of RCTs of LVO for AIS showed that early treatment with EVT was associated with improved outcomes (53), a recent meta-analysis showed that good collateral status is associated with better clinical responses to EVT even in later time windows, suggesting that collateral status can extend the time window for EVT (54). A retrospective multicenter study of 720 patients showed that while the probability of good outcomes in patients with embolic occlusion declined as onset-to-puncture time increased, the probability of good outcomes in patients with ICAS-LVO did not decline but tended to increase with increase in onset-to-puncture time (8). The incidence of slow progressors may be <30% of patients with anterior circulation LVO in large referral centers (55), but may be higher in ICAS-LVO because collateral circulation in patients with ICAS was better than in those with other stroke subtypes (56).
Non-atherosclerotic Origin
In addition to ICAS, non-atherosclerotic intracranial arterial diseases, such as moyamoya disease or intracranial arterial dissection, may also cause LVO. Careful evaluation of clinical and luminal studies (such as healthy risk factor profiles and no tandem stenosis or calcification in intracranial arterial dissection, and the presence of family history and basal collaterals in moyamoya disease) may provide clues for the diagnosis of these non-atherosclerotic diseases. However, it is often difficult to differentiate them in clinical practice. Prospective observational high-resolution MRI (HR-MRI) studies of non-stroke subjects (57), young stroke patients (58), and acute stroke patients (59) showed that non-atherosclerotic intracranial large artery disease is prevalent across a wide range of atherosclerosis risk groups. Therapeutic strategies used in intracranial atherosclerosis may not be helpful or may even be detrimental in some patients with non-atherosclerotic LVO (18). For example, stent placement should be avoided in moyamoya disease (60–62), but stent placement (especially, closed cell-type stent) may be considered in intracranial arterial dissection. A recent study showed that endovascular thrombectomy is an effective in selected patients with acute ischemic stroke associated with cervical artery dissection (63), but further studies are needed in patients with acute infarcts due to intracranial non-atherosclerotic occlusion.
Specific Diagnostic and Therapeutic Considerations in EVT for ICAS-LVO
Assessment Tools for Underlying Features of ICAS
Plaque Images
HR-MRI may provide information on arterial wall pathology, such as plaque characteristics and arterial remodeling. Recently, the imaging findings of intracranial plaques were verified with histopathology (64, 65). A HR-MRI study showed that EVT causes post-recanalization changes of affected arterial segments, which correlated with thrombectomy procedural factors such as number of procedures and type of device used, and was associated with poor outcomes (66, 67). HR-MRI studies conducted after various modes of EVT demonstrated vessel damage related to stentriever process and may be useful for the development of optimal endovascular therapeutic strategies or devices with minimal intimal injury (66, 67). HR-MRI can also provide information on angioarchitecture. Data on the presence and location of perforators in relation to the plaque, in patients with ICAS-LVO, can be useful when considering stent placement in perforator-bearing segments. Lastly, HR-MRI can be used to differentiate non-atherosclerotic intracranial large vessel disease from ICAS in patients. Although concerted efforts have been made to increase signal-to-noise and contrast-to-noise characteristics and to shorten the scanning time, routine use of HR-MRI is not feasible in clinical practice. Like in coronary heart disease, catheter-based imaging can be an alternative modality for use in EVT settings. Intravascular ultrasound (IVUS) and optical coherence tomography (OCT, the light analog of IVUS) are intravascular imaging techniques used in interventional cardiology (68). A meta-analysis of RCTs comparing IVUS- and angiographic-guided percutaneous coronary interventions showed that IVUS guidance was associated with significantly lower rates of angiographic restenosis, repeat revascularization, and overall occurrence of major cardiac events (69). The results of several case reports suggest that these techniques may provide useful information for the selection of patients with ICAS who may benefit from stent placement therapy (16). In addition, IVUS can be used during the EVT procedure to differentiate ICAS-LVO from embolic LVO by visualization of calcified plaque in ICAS. IVUS may help differentiation of intracranial arterial dissection from ICAS and identification of the most distal and proximal extent of arterial dissection, so that the entire length of the dissection could be covered with stent (70). These techniques can also provide virtual histology to characterize plaques in large intracranial vessels. An in vitro study of intracranial arterial segments with atherosclerotic plaques demonstrated a strong correlation between virtual histology using IVUS and 7T MRI and histopathologic analysis (71). Gounis et al. recently introduced the high-frequency OCT device for the highly tortuous cerebrovasculature that provides good quality imaging of vessel wall layers, the ostium of small branches/perforators, and the relationship between neurovascular devices and vessel wall (17).
Thrombus Images
Identifying the characteristics of a thrombus in AIS may provide vital information for the determination of the optimal strategy for revascularization therapy and for the choice of antithrombotics for the secondary prevention of stroke. The characteristics of a thrombus (size and composition) may determine the recanalization rate, time required for re-opening, and the response to acute and preventive treatment in patients with AIS. Therefore, it is extremely useful to know the thrombus characteristics before initiating recanalization therapy. A thrombus can be detected on a non-contrast CT image as a hyperdense artery sign or a blooming artifact on T2*-weighted gradient-recalled image. Details on the methods to measure thrombus size and burden are presented elsewhere (14). Thrombus size determines the response to revascularization. Although thrombus length is strongly associated with successful recanalization with intravenous tPA therapy, the predictive power of thrombus size in determining successful reperfusion in EVT appears to be diminished (14). The results of recent RCTs showed that there was no correlation between the clot burden score (using clot volume and length) and the effects of EVT (72, 73). Thrombus composition and its associated pathogenesis can be visualized by CT or MRI. The density on CT may reflect the thrombus composition. Erythrocytes in thrombi increase attenuation on CT, and the hyperdense artery sign is more commonly seen in erythrocyte-dominant thrombi than in fibrin-rich thrombi. For example, thrombus permeability, as measured by thrombus density on thin-slice non-contrast CT imaging, was found to correlate with the histological components of retrieved thrombi and permeable thrombi were associated with cardioembolic occlusion in patients with AIS (74). However, a recent systematic analysis showed a lack of association between a CT-based clot image (e.g., Hounsfield units) and histopathology of thrombi or stroke etiology (75). Similarly, an erythrocyte component in thrombi induces ferromagnetic field distortion, which results in a blooming artifact on gradient-recalled echo or susceptibility-weighted imaging. The presence of a blooming artifact on MRI is associated with cardioembolic stroke (76, 77). Pathological studies of thrombi retrieved via EVT showed that the presence and absence of blooming artifacts were found to be due to erythrocyte- and fibrin-predominant occlusive thrombi, respectively, and erythrocyte-rich thrombi were associated with successful recanalization of EVT and cardioembolic stroke (78, 79). Lastly, direct thrombus imaging targeting fibrinogen can determine the initial burden and location of thrombi and may also help visualize residual thrombi or distal thromboembolism. Kim et al. investigated hyperacute direct thrombus imaging techniques and monitored the therapeutic efficacy of thrombolysis using fibrin-targeted gold nanoparticles and CT imaging (80). Various MRI probes, such as fibrin-binding gadolinium-labeled peptides, have been used for the evaluation of acute thrombosis after plaque rupture in animal models (81–83).
Collateral Images
Conventional angiographic evaluation is the gold standard for collateral assessment (84). However, more time is needed to include the venous phase and contralateral or vertebrobasilar views. In using EVT in clinical settings, most interventionalists perform angiography of the affected territory and open the occluded vessel without performing angiography of unaffected territories in a bid to shorten the puncture-to-reperfusion time. Both multiphase CT angiography and perfusion MRI-based collateral maps can be performed in acute settings (85–87), and they showed a good correlation with conventional angiography for leptomeningeal collateral grading in AIS (87–89). These non-invasive collateral assessments are particularly important in ICAS-LVO, because pre-procedure CT or MRI data can be used for selecting slower infarct progressors presented at a later time. CT angiography shows the anatomical configuration of collateral vessels and its use is becoming more routine. However, there is no consensus on the best method for evaluating and grading collaterals and various CT angiography techniques and grading systems are used (90–92). Other imaging techniques, such as CT perfusion and arterial spin labeling MRI, may also provide information on collateral status (15).
EVT Devices and Techniques for ICAS-LVO
Stentrievers were the main devices described for use in EVT in the RCTs, and the current guidelines recommend mechanical thrombectomy with a strentriever in conjunction with intravenous tPA as the standard of care in anterior circulation stroke caused by LVOs (93). Owing to the aforementioned characteristics of ICAS-LVO, better tools and techniques are needed for smaller and/or tortuous arteries, the minimization of vessel damage, and the facilitation of rescue therapies. For these purposes, detachable stents with radio-opaque markers for visualizing residual stenosis are required. Stents with radiopaque design can provide better visualization of stent-thrombus interaction during stentrieval process, and also provide additional information on the nature of thrombus as atherosclerotic lesion may appear as an area of strut compression or waist. Permanent stent placement may be required in case of residual stenosis or re-occlusion. In this situation, radiopaque stent strip is informative in stent placement and detachability is essential. However, no radiopaque detachable stentriever is available until now; radiopaque trevo stent is not detachable while solitaire AB is not radiopaque.
In cases in which the relevant artery is tortuous, a large bore balloon guide catheter is preferred, and the stenotic segment of the intracranial artery is crossed with microwire as distally as possible to ensure maximal support while allowing tracking of the balloon guide catheter. To overcome vascular tortuosity, coaxial double-guiding catheter technique, or double-wire technique could be considered (94–96).
In addition, distal access catheters (such as intermediate catheter) provide support and stability for microcatheters and are also suitable for aspiration. The ability to deliver intermediate catheters to the vicinity of the thrombus ensures the generation of greater effective retrieval force by the device especially in cases with significant vessel tortuosity. It also provides a strong enough suction force to remove soft thrombi without using a stent retriever (ADAPT, a direct aspiration first pass technique) (43). Theoretically, this approach is ideal as it results in lesser damage to vessels and underlying plaques, and it may prevent the distal migration of clots to a greater extent than possible with stentrievers. However, the contact aspiration technique requires optimal contact between the aspiration catheter tip and the thrombus, which depends on the location of the thrombus and the tortuosity of the vessel (97). In some cases, the contact aspiration technique may not be effective due to imprecise positioning of the aspiration catheter tip relative to the thrombus. The results of a recent RCT showed no significant differences in the primary outcome of final successful recanalization rates between ADAPT and stentrievers (98). Stentrievers can also be used in conjunction with direct aspiration at the face of a thrombus during thrombectomy (Solumbra technique) (99, 100).
Rescue treatments, including balloon angioplasty, rescue stenting, and intra-arterial glycoprotein IIb/IIIa inhibitor infusion, can be considered for ICAS-LVO refractory to stentriever (3). On the contrary, Yang and the ACUAL investigators studied 302 patients with ICAS-LVO and reported that patients who received angioplasty and/or stenting as first-line therapy showed favorable outcome and lower rate of intracranial bleeding than those received stentriever (10). Further studies are needed in patients with ICAS-LVO to determine the first-line device and technique for thrombectomy (stentriever, ADAPT, or Solumbra), pharmacological adjunct (intravenous tPA or intra-arterial antithrombotics), and cessation time for procedures in cases of repetitive reocclusion.
Conclusions
Despite the recent success of EVT, there are still numerous challenges with respect to management of ICAS-LVO. Studies discussed herein suggest that there are more diverse neuroimaging features in ICAS-LVO than in embolic occlusion. While recent RCTs of EVT showed that appropriate selection is important in AIS, selection of appropriate EVT procedures may be more important in patients with ICAS-LVO. Patients with ICAS-LVO usually have favorable hemodynamic profiles but demonstrate poor response to EVT. Though ICAS-LVO requires more complex and technically demanding recanalization strategies than embolic occlusion, good outcomes are attainable with the application of appropriate therapeutic strategies.
Future studies should focus on investigating reliable imaging predictors related to response to EVT in ICAS-LVO patients, and on developing and evaluating thrombectomy approaches to overcome the characteristic drawback of reocclusion in ICAS-LVO. Advanced neuroimaging of plaques, thrombi, and collaterals could not be performed in the EVT setting. However, post-EVT analysis may be useful for the characterization of patients with ICAS-LVO, clearer understanding of the pathophysiology of ICAS-LVO, and future guidance for optimal therapeutic strategies for ICAS-LVO. For clinical use of advanced neuroimaging techniques for patients with AIS, fast, and safe assessment tools that can visualize individual features of ICAS, automated software that allows fast post-processing is mandatory, and is increasingly being used in clinical trials (17, 29). In addition, optimal tools and techniques for ICAS-LVO are not settled yet. Most of the studies presented here were retrospective studies conducted in East Asian countries. It is necessary to conduct RCTs of acute interventions for ICAS-LVO in diversified populations to reach recommendations.
Author Contributions
OB study concept and design, acquisition of data, analysis and interpretation of data, drafting/revising the manuscript for content. BK, W-KS, and PJ drafting/revising the manuscript for content.
Funding
This study was supported by a grant from the Ministry of Science and Information and Communication Technology (2018R1A2B2003489).
Conflict of Interest Statement
The authors declare that the research was conducted in the absence of any commercial or financial relationships that could be construed as a potential conflict of interest.
References
1. Gorelick PB, Wong KS, Bae HJ, Pandey DK. Large artery intracranial occlusive disease: a large worldwide burden but a relatively neglected frontier. Stroke. (2008) 39:2396–9. doi: 10.1161/STROKEAHA.107.505776
2. Baek JH, Kim BM, Kim DJ, Heo JH, Nam HS, Song D, et al. Importance of truncal-type occlusion in stentriever-based thrombectomy for acute stroke. Neurology. (2016) 87:1542–50. doi: 10.1212/WNL.0000000000003202
3. Baek JH, Kim BM, Heo JH, Kim DJ, Nam HS, Kim YD. Outcomes of endovascular treatment for acute intracranial atherosclerosis-related large vessel occlusion. Stroke. (2018) 49:2699–705. doi: 10.1161/STROKEAHA.118.022327
4. Hwang YH, Kim YW, Kang DH, Kim YS, Liebeskind DS. Impact of target arterial residual stenosis on outcome after endovascular revascularization. Stroke. (2016) 47:1850–7. doi: 10.1161/STROKEAHA.116.013046
5. Al Kasab S, Almadidy Z, Spiotta AM, Turk AS, Chaudry MI, Hungerford JP, et al. Endovascular treatment for AIS with underlying ICAD. J Neurointerv Surg. (2017) 9:948–51. doi: 10.1136/neurintsurg-2016-012529
6. Kim YW, Hong JM, Park DG, Choi JW, Kang DH, Kim YS, et al. Effect of intracranial atherosclerotic disease on endovascular treatment for patients with acute vertebrobasilar occlusion. AJNR Am J Neuroradiol. (2016) 37:2072–8. doi: 10.3174/ajnr.A4844
7. Kang DH, Yoon W, Kim SK, Baek BH, Lee YY, Kim YW, et al. Endovascular treatment for emergent large vessel occlusion due to severe intracranial atherosclerotic stenosis. J Neurosurg. (2018). doi: 10.3171/2018.1.JNS172350. [Epub ahead of print].
8. Lee JS, Lee SJ, Yoo JS, Hong JH, Kim CH, Kim YW, et al. Prognosis of acute intracranial atherosclerosis-related occlusion after endovascular treatment. J Stroke. (2018) 20:394–403. doi: 10.5853/jos.2018.01627
9. Gascou G, Lobotesis K, Machi P, Maldonado I, Vendrell JF, Riquelme C, et al. Stent retrievers in acute ischemic stroke: complications and failures during the perioperative period. AJNR Am J Neuroradiol. (2014) 35:734–40. doi: 10.3174/ajnr.A3746
10. Yang D, Lin M, Wang S, Wang H, Hao Y, Zi W, et al. Primary angioplasty and stenting may be superior to thrombectomy for acute atherosclerotic large-artery occlusion. Interv Neuroradiol. (2018) 24:412–20. doi: 10.1177/1591019918763380
11. Bodle JD, Feldmann E, Swartz RH, Rumboldt Z, Brown T, Turan TN. High-resolution magnetic resonance imaging: an emerging tool for evaluating intracranial arterial disease. Stroke. (2013) 44:287–92. doi: 10.1161/STROKEAHA.112.664680
12. Alexander MD, Yuan C, Rutman A, Tirschwell DL, Palagallo G, Gandhi D, et al. High-resolution intracranial vessel wall imaging: imaging beyond the lumen. J Neurol Neurosurg Psychiatry. (2016) 87:589–97. doi: 10.1136/jnnp-2015-312020
13. Kim J, Park JE, Nahrendorf M, Kim DE. Direct thrombus imaging in stroke. J Stroke. (2016) 18:286–96. doi: 10.5853/jos.2016.00906
14. Heo JH, Kim K, Yoo J, Kim YD, Nam HS, Kim EY. Computed tomography-based thrombus imaging for the prediction of recanalization after reperfusion therapy in stroke. J Stroke. (2017) 19:40–9. doi: 10.5853/jos.2016.01522
15. Bang OY, Goyal M, Liebeskind DS. Collateral circulation in ischemic stroke: assessment tools and therapeutic strategies. Stroke. (2015) 46:3302–9. doi: 10.1161/STROKEAHA.115.010508
16. Pavlin-Premrl D, Sharma R, Campbell BCV, Mocco J, Opie NL, Oxley TJ. Advanced imaging of intracranial atherosclerosis: lessons from interventional cardiology. Front Neurol. (2017) 8:387. doi: 10.3389/fneur.2017.00387
17. Gounis MJ, Ughi GJ, Marosfoi M, Lopes DK, Fiorella D, Bezerra HG, et al. Intravascular optical coherence tomography for neurointerventional surgery. Stroke. (2018). doi: 10.1161/STROKEAHA.118.022315. [Epub ahead of print].
18. Bang OY, Toyoda K, Arenillas JF, Liu L, Kim JS. Intracranial large artery disease of non-atherosclerotic origin: recent progress and clinical implications. J Stroke. (2018) 20:208–17. doi: 10.5853/jos.2018.00150
19. Berkhemer OA, Fransen PS, Beumer D, van den Berg LA, Lingsma HF, Yoo AJ, et al. A randomized trial of intraarterial treatment for acute ischemic stroke. N Engl J Med. (2015) 372:11–20. doi: 10.1056/NEJMoa1411587
20. Goyal M, Demchuk AM, Menon BK, Eesa M, Rempel JL, Thornton J, et al. Randomized assessment of rapid endovascular treatment of ischemic stroke. N Engl J Med. (2015) 372:1019–30. doi: 10.1056/NEJMoa1414905
21. Campbell BC, Mitchell PJ, Kleinig TJ, Dewey HM, Churilov L, Yassi N, et al. Endovascular therapy for ischemic stroke with perfusion-imaging selection. N Engl J Med. (2015) 372:1009–18. doi: 10.1056/NEJMoa1414792
22. Saver JL, Goyal M, Bonafe A, Diener HC, Levy EI, Pereira VM, et al. Stent-retriever thrombectomy after intravenous t-PA vs. t-PA alone in stroke. N Engl J Med. (2015) 372:2285–95. doi: 10.1056/NEJMoa1415061
23. Jovin TG, Chamorro A, Cobo E, de Miquel MA, Molina CA, Rovira A, et al. Thrombectomy within 8 hours after symptom onset in ischemic stroke. N Engl J Med. (2015) 372:2296–306. doi: 10.1056/NEJMoa1503780
24. Nogueira RG, Jadhav AP, Haussen DC, Bonafe A, Budzik RF, Bhuva P, et al. Thrombectomy 6 to 24 hours after stroke with a mismatch between deficit and infarct. N Engl J Med. (2018) 378:11–21. doi: 10.1056/NEJMoa1706442
25. Albers GW, Marks MP, Kemp S, Christensen S, Tsai JP, Ortega-Gutierrez S, et al. Thrombectomy for stroke at 6 to 16 hours with selection by perfusion imaging. N Engl J Med. (2018) 378:708–18. doi: 10.1056/NEJMoa1713973
26. Goyal M, Menon BK, van Zwam WH, Dippel DW, Mitchell PJ, Demchuk AM, et al. Endovascular thrombectomy after large-vessel ischaemic stroke: a meta-analysis of individual patient data from five randomised trials. Lancet. (2016) 387:1723–31. doi: 10.1016/S0140-6736(16)00163-X
27. Campbell BC, Hill MD, Rubiera M, Menon BK, Demchuk A, Donnan GA, et al. Safety and efficacy of solitaire stent thrombectomy: individual patient data meta-analysis of randomized trials. Stroke. (2016) 47:798–806. doi: 10.1161/STROKEAHA.115.012360
28. Lee JS, Hong JM, Lee KS, Suh HI, Demchuk AM, Hwang YH, et al. Endovascular therapy of cerebral arterial occlusions: intracranial atherosclerosis versus embolism. J Stroke Cerebrovasc Dis. (2015) 24:2074–80. doi: 10.1016/j.jstrokecerebrovasdis.2015.05.003
29. Bang OY, Chung JW, Son JP, Ryu WS, Kim DE, Seo WK, et al. Multimodal MRI-based triage for acute stroke therapy: challenges and progress. Front Neurol. (2018) 9:586. doi: 10.3389/fneur.2018.00586
30. Toyoda K, Koga M, Hayakawa M, Yamagami H. Acute reperfusion therapy and stroke care in Asia after successful endovascular trials. Stroke. (2015) 46:1474–81. doi: 10.1161/STROKEAHA.115.008781
31. Mosimann PJ, Kaesmacher J, Gautschi D, Bellwald S, Panos L, Piechowiak E, et al. Predictors of unexpected early reocclusion after successful mechanical thrombectomy in acute ischemic stroke patients. Stroke. (2018) 49:2643–51. doi: 10.1161/STROKEAHA.118.021685
32. Abou-Chebl A, Steinmetz H. Critique of “Stenting versus aggressive medical therapy for intracranial arterial stenosis” by Chimowitz et al in the new England Journal of Medicine. Stroke. (2012) 43:616–20. doi: 10.1161/STROKEAHA.111.641563
33. Nordmeyer H, Chapot R, Aycil A, Stracke CP, Wallocha M, Hadisurya MJ, et al. Angioplasty and stenting of intracranial arterial stenosis in perforator-bearing segments: a comparison between the anterior and the posterior circulation. Front Neurol. (2018) 9:533. doi: 10.3389/fneur.2018.00533
34. Park KY, Kim BM, Kim DJ, Kim DI, Heo JH, Nam HS, et al. Incidence and risk factors for diffusion-weighted imaging (+) lesions after intracranial stenting and its relationship with symptomatic ischemic complications. Stroke. (2014) 45:3298–303. doi: 10.1161/STROKEAHA.114.006182
35. Ryoo S, Park JH, Kim SJ, Kim GM, Chung CS, Lee KH, et al. Branch occlusive disease: clinical and magnetic resonance angiography findings. Neurology. (2012) 78:888–96. doi: 10.1212/WNL.0b013e31824c4699
36. Ryoo S, Lee MJ, Cha J, Jeon P, Bang OY. Differential vascular pathophysiologic types of intracranial atherosclerotic stroke: a high-resolution wall magnetic resonance imaging study. Stroke. (2015) 46:2815–21. doi: 10.1161/STROKEAHA.115.010894
37. Miyawaki S, Imai H, Shimizu M, Yagi S, Ono H, Mukasa A, et al. Genetic variant RNF213 c.14576G>A in various phenotypes of intracranial major artery stenosis/occlusion. Stroke. (2013) 44:2894–7. doi: 10.1161/STROKEAHA.113.002477
38. Bang OY, Chung JW, Cha J, Lee MJ, Yeon JY, Ki CS, et al. A polymorphism in RNF213 is a susceptibility gene for intracranial atherosclerosis. PLoS ONE. (2016) 11:e0156607. doi: 10.1371/journal.pone.0156607
39. Hongo H, Miyawaki S, Imai H, Shinya Y, Ono H, Mori H, et al. Smaller outer diameter of atherosclerotic middle cerebral artery associated with RNF213 c.14576G>A Variant (rs112735431). Surg Neurol Int. (2017) 8:104. doi: 10.4103/sni.sni_59_17
40. Hernandez-Perez M, Bos D, Dorado L, Pellikaan K, Vernooij MW, Lopez-Cancio E, et al. Intracranial carotid artery calcification relates to recanalization and clinical outcome after mechanical thrombectomy. Stroke. (2017) 48:342–7. doi: 10.1161/STROKEAHA.116.015166
41. Compagne KCJ, Clephas PRD, Majoie C, Roos Y, Berkhemer OA, van Oostenbrugge RJ, et al. Intracranial carotid artery calcification and effect of endovascular stroke treatment. Stroke. (2018) 49:2961–8. doi: 10.1161/STROKEAHA.118.022400
42. Mackman N. Triggers, targets and treatments for thrombosis. Nature. (2008) 451:914–8. doi: 10.1038/nature06797
43. Yoo AJ, Andersson T. Thrombectomy in acute ischemic stroke: challenges to procedural success. J Stroke. (2017) 19:121–30. doi: 10.5853/jos.2017.00752
44. Qazi EM, Sohn SI, Mishra S, Almekhlafi MA, Eesa M, d'Esterre CD, et al. Thrombus characteristics are related to collaterals and angioarchitecture in acute stroke. Can J Neurol Sci. (2015) 42:381–8. doi: 10.1017/cjn.2015.291
45. Hashimoto T, Hayakawa M, Funatsu N, Yamagami H, Satow T, Takahashi JC, et al. Histopathologic analysis of retrieved thrombi associated with successful reperfusion after acute stroke thrombectomy. Stroke. (2016) 47:3035–7. doi: 10.1161/STROKEAHA.116.015228
46. Choi MH, Park GH, Lee JS, Lee SE, Lee SJ, Kim JH, et al. Erythrocyte fraction within retrieved thrombi contributes to thrombolytic response in acute ischemic stroke. Stroke. (2018) 49:652–9. doi: 10.1161/STROKEAHA.117.019138
47. Alves HC, Treurniet KM, Dutra BG, Jansen IGH, Boers AMM, Santos EMM, et al. Associations between collateral status and thrombus characteristics and their impact in anterior circulation stroke. Stroke. (2018) 49:391–6. doi: 10.1161/STROKEAHA.117.019509
48. Gorog DA, Fayad ZA, Fuster V. Arterial thrombus stability: does it matter and can we detect it? J Am Coll Cardiol. (2017) 70:2036–47. doi: 10.1016/j.jacc.2017.08.065
49. Liebeskind DS, Cotsonis GA, Saver JL, Lynn MJ, Turan TN, Cloft HJ, et al. Collaterals dramatically alter stroke risk in intracranial atherosclerosis. Ann Neurol. (2011) 69:963–74. doi: 10.1002/ana.22354
50. Liebeskind DS, Tomsick TA, Foster LD, Yeatts SD, Carrozzella J, Demchuk AM, et al. Collaterals at angiography and outcomes in the Interventional Management of Stroke (IMS) III trial. Stroke. (2014) 45:759–64. doi: 10.1161/STROKEAHA.113.004072
51. Liebeskind DS, Jahan R, Nogueira RG, Zaidat OO, Saver JL, SWIFT Investigators. Impact of collaterals on successful revascularization in Solitaire FR with the intention for thrombectomy. Stroke. (2014) 45:2036–40. doi: 10.1161/STROKEAHA.114.004781
52. Berkhemer OA, Jansen IG, Beumer D, Fransen PS, van den Berg LA, Yoo AJ, et al. Collateral status on baseline computed tomographic angiography and intra-arterial treatment effect in patients with proximal anterior circulation stroke. Stroke. (2016) 47:768–76. doi: 10.1161/STROKEAHA.115.011788
53. Saver JL, Goyal M, van der Lugt A, Menon BK, Majoie CB, Dippel DW, et al. Time to treatment with endovascular thrombectomy and outcomes from ischemic stroke: a meta-analysis. JAMA. (2016) 316:1279–88. doi: 10.1001/jama.2016.13647
54. Leng X, Fang H, Leung TW, Mao C, Miao Z, Liu L, et al. Impact of collaterals on the efficacy and safety of endovascular treatment in acute ischaemic stroke: a systematic review and meta-analysis. J Neurol Neurosurg Psychiatry. (2016) 87:537–44. doi: 10.1136/jnnp-2015-310965
55. Copen WA, Rezai Gharai L, Barak ER, Schwamm LH, Wu O, Kamalian S, et al. Existence of the diffusion-perfusion mismatch within 24 hours after onset of acute stroke: dependence on proximal arterial occlusion. Radiology. (2009) 250:878–86. doi: 10.1148/radiol.2503080811
56. Kim SJ, Seok JM, Bang OY, Kim GM, Kim KH, Jeon P, et al. MR mismatch profiles in patients with intracranial atherosclerotic stroke: a comprehensive approach comparing stroke subtypes. J Cereb Blood Flow Metab. (2009) 29:1138–45. doi: 10.1038/jcbfm.2009.38
57. Ahn SH, Lee J, Kim YJ, Kwon SU, Lee D, Jung SC, et al. Isolated MCA disease in patients without significant atherosclerotic risk factors: a high-resolution magnetic resonance imaging study. Stroke. (2015) 46:697–703. doi: 10.1161/STROKEAHA.114.008181
58. Kim YJ, Lee JK, Ahn SH, Kim BJ, Kang DW, Kim JS, et al. Nonatheroscleotic isolated middle cerebral artery disease may be early manifestation of Moyamoya disease. Stroke. (2016) 47:2229–35. doi: 10.1161/STROKEAHA.116.012751
59. Park MS, Cha J, Chung JW, Seo WK, Kim GM, Bang OY. Arterial dissection as a cause of intracranial stenosis in East Asians. J Am Coll Cardiol. (2017) 70:2205–6. doi: 10.1016/j.jacc.2017.08.048
60. Natarajan SK, Karmon Y, Tawk RG, Hauck EF, Hopkins LN, Siddiqui AH, et al. Endovascular treatment of patients with intracranial stenosis with moyamoya-type collaterals. J Neurointerv Surg. (2011) 3:369–74. doi: 10.1136/jnis.2011.004754
61. Drazin D, Calayag M, Gifford E, Dalfino J, Yamamoto J, Boulos AS. Endovascular treatment for moyamoya disease in a Caucasian twin with angioplasty and Wingspan stent. Clin Neurol Neurosurg. (2009) 111:913–7. doi: 10.1016/j.clineuro.2009.08.012
62. Khan N, Dodd R, Marks MP, Bell-Stephens T, Vavao J, Steinberg GK. Failure of primary percutaneous angioplasty and stenting in the prevention of ischemia in Moyamoya angiopathy. Cerebrovasc Dis. (2011) 31:147–53. doi: 10.1159/000320253
63. Li S, Zi W, Chen J, Zhang S, Bai Y, Guo Y, et al. Feasibility of thrombectomy in treating acute ischemic stroke because of cervical artery dissection. Stroke. (2018) 49:3075–7. doi: 10.1161/STROKEAHA.118.023186
64. van der Kolk AG, Zwanenburg JJ, Brundel M, Biessels GJ, Visser F, Luijten PR, et al. Intracranial vessel wall imaging at 7.0-T MRI. Stroke. (2011) 42:2478–84. doi: 10.1161/STROKEAHA.111.620443
65. Harteveld AA, Denswil NP, Siero JC, Zwanenburg JJ, Vink A, Pouran B, et al. Quantitative intracranial atherosclerotic plaque characterization at 7T MRI: an ex vivo study with histologic validation. AJNR Am J Neuroradiol. (2016) 37:802–10. doi: 10.3174/ajnr.A4628
66. Seo WK, Oh K, Suh SI, Seol HY. Clinical significance of wall changes after recanalization therapy in acute stroke: high-resolution vessel wall imaging. Stroke. (2017) 48:1077–80. doi: 10.1161/STROKEAHA.116.015429
67. Renu A, Laredo C, Lopez-Rueda A, Llull L, Tudela R, San-Roman L, et al. Vessel wall enhancement and blood-cerebrospinal fluid barrier disruption after mechanical thrombectomy in acute ischemic stroke. Stroke. (2017) 48:651–7. doi: 10.1161/STROKEAHA.116.015648
68. Sanidas E, Dangas G. Evolution of intravascular assessment of coronary anatomy and physiology: from ultrasound imaging to optical and flow assessment. Eur J Clin Invest. (2013) 43:996–1008. doi: 10.1111/eci.12119
69. Parise H, Maehara A, Stone GW, Leon MB, Mintz GS. Meta-analysis of randomized studies comparing intravascular ultrasound versus angiographic guidance of percutaneous coronary intervention in pre-drug-eluting stent era. Am J Cardiol. (2011) 107:374–82. doi: 10.1016/j.amjcard.2010.09.030
70. Wehman JC, Holmes DR Jr, Hanel RA, Levy EI, Hopkins LN. Intravascular ultrasound for intracranial angioplasty and stent placement: technical case report. Neurosurgery. (2006) 59:ONSE481-3; discussion ONSE3. doi: 10.1227/01.NEU.0000222825.92929.0C
71. Majidi S, Sein J, Watanabe M, Hassan AE, Van de Moortele PF, Suri MF, et al. Intracranial-derived atherosclerosis assessment: an in vitro comparison between virtual histology by intravascular ultrasonography, 7T MRI, and histopathologic findings. AJNR Am J Neuroradiol. (2013) 34:2259–64. doi: 10.3174/ajnr.A3631
72. Mokin M, Levy EI, Siddiqui AH, Goyal M, Nogueira RG, Yavagal DR, et al. Association of clot burden score with radiographic and clinical outcomes following Solitaire stent retriever thrombectomy: analysis of the SWIFT PRIME trial. J Neurointerv Surg. (2017) 9:929–32. doi: 10.1136/neurintsurg-2016-012631
73. Treurniet KM, Yoo AJ, Berkhemer OA, Lingsma HF, Boers AM, Fransen PS, et al. Clot burden score on baseline computerized tomographic angiography and intra-arterial treatment effect in acute ischemic stroke. Stroke. (2016) 47:2972–8. doi: 10.1161/STROKEAHA.116.014565
74. Berndt M, Friedrich B, Maegerlein C, Moench S, Hedderich D, Lehm M, et al. Thrombus permeability in admission computed tomographic imaging indicates stroke pathogenesis based on thrombus histology. Stroke. (2018) 49:2674–82. doi: 10.1161/STROKEAHA.118.021873
75. Brinjikji W, Duffy S, Burrows A, Hacke W, Liebeskind D, Majoie CB, et al. Correlation of imaging and histopathology of thrombi in acute ischemic stroke with etiology and outcome: a systematic review. J Neurointerv Surg. (2016) doi: 10.1136/neurintsurg-2016-012589.53
76. Kang DW, Jeong HG, Kim DY, Yang W, Lee SH. Prediction of stroke subtype and recanalization using susceptibility vessel sign on susceptibility-weighted magnetic resonance imaging. Stroke. (2017) 48:1554–9. doi: 10.1161/STROKEAHA.116.016217
77. Zhang R, Zhou Y, Liu C, Zhang M, Yan S, Liebeskind DS, et al. Overestimation of susceptibility vessel sign: a predictive marker of stroke cause. Stroke. (2017) 48:1993–6. doi: 10.1161/STROKEAHA.117.016727
78. Liebeskind DS, Sanossian N, Yong WH, Starkman S, Tsang MP, Moya AL, et al. CT and MRI early vessel signs reflect clot composition in acute stroke. Stroke. (2011) 42:1237–43. doi: 10.1161/STROKEAHA.110.605576
79. Shin JW, Jeong HS, Kwon HJ, Song KS, Kim J. High red blood cell composition in clots is associated with successful recanalization during intra-arterial thrombectomy. PLoS ONE. (2018) 13:e0197492. doi: 10.1371/journal.pone.0197492
80. Kim DE, Kim JY, Sun IC, Schellingerhout D, Lee SK, Ahn CH, et al. Hyperacute direct thrombus imaging using computed tomography and gold nanoparticles. Ann Neurol. (2013) 73:617–25. doi: 10.1002/ana.23849
81. Botnar RM, Perez AS, Witte S, Wiethoff AJ, Laredo J, Hamilton J, et al. In vivo molecular imaging of acute and subacute thrombosis using a fibrin-binding magnetic resonance imaging contrast agent. Circulation. (2004) 109:2023–9. doi: 10.1161/01.CIR.0000127034.50006.C0
82. Sirol M, Fuster V, Badimon JJ, Fallon JT, Moreno PR, Toussaint JF, et al. Chronic thrombus detection with in vivo magnetic resonance imaging and a fibrin-targeted contrast agent. Circulation. (2005) 112:1594–600. doi: 10.1161/CIRCULATIONAHA.104.522110
83. Uppal R, Ay I, Dai G, Kim YR, Sorensen AG, Caravan P. Molecular MRI of intracranial thrombus in a rat ischemic stroke model. Stroke. (2010) 41:1271–7. doi: 10.1161/STROKEAHA.109.575662
84. Zaidat OO, Yoo AJ, Khatri P, Tomsick TA, von Kummer R, Saver JL, et al. Recommendations on angiographic revascularization grading standards for acute ischemic stroke: a consensus statement. Stroke. (2013) 44:2650–63. doi: 10.1161/STROKEAHA.113.001972
85. Menon BK, d'Esterre CD, Qazi EM, Almekhlafi M, Hahn L, Demchuk AM, et al. Multiphase CT angiography: a new tool for the imaging triage of patients with acute ischemic stroke. Radiology. (2015) 275:510–20. doi: 10.1148/radiol.15142256
86. Campbell BC, Christensen S, Tress BM, Churilov L, Desmond PM, Parsons MW, et al. Failure of collateral blood flow is associated with infarct growth in ischemic stroke. J Cereb Blood Flow Metab. (2013) 33:1168–72. doi: 10.1038/jcbfm.2013.77
87. Kim SJ, Son JP, Ryoo S, Lee MJ, Cha J, Kim KH, et al. A novel magnetic resonance imaging approach to collateral flow imaging in ischemic stroke. Ann Neurol. (2014) 76:356–69. doi: 10.1002/ana.24211
88. Lee KH, Cho SJ, Byun HS, Na DG, Choi NC, Lee SJ, et al. Triphasic perfusion computed tomography in acute middle cerebral artery stroke: a correlation with angiographic findings. Arch Neurol. (2000) 57:990–9. doi: 10.1001/archneur.57.7.990
89. Maier IL, Scalzo F, Leyhe JR, Schregel K, Behme D, Tsogkas I, et al. Validation of collateral scoring on flat-detector multiphase CT angiography in patients with acute ischemic stroke. PLoS ONE. (2018) 13:e0202592. doi: 10.1371/journal.pone.0202592
90. Shuaib A, Butcher K, Mohammad AA, Saqqur M, Liebeskind DS. Collateral blood vessels in acute ischaemic stroke: a potential therapeutic target. Lancet Neurol. (2011) 10:909–21. doi: 10.1016/S1474-4422(11)70195-8
91. van den Wijngaard IR, Holswilder G, Wermer MJ, Boiten J, Algra A, Dippel DW, et al. Assessment of collateral status by dynamic CT angiography in acute MCA stroke: timing of acquisition and relationship with final infarct volume. AJNR Am J Neuroradiol. (2016) 37:1231–6. doi: 10.3174/ajnr.A4746
92. Yeo LL, Paliwal P, Teoh HL, Seet RC, Chan BP, Ting E, et al. Assessment of intracranial collaterals on CT angiography in anterior circulation acute ischemic stroke. AJNR Am J Neuroradiol. (2015) 36:289–94. doi: 10.3174/ajnr.A4117
93. Powers WJ, Rabinstein AA, Ackerson T, Adeoye OM, Bambakidis NC, Becker K, et al. 2018 guidelines for the early management of patients with acute ischemic stroke: a guideline for healthcare professionals from the American heart association/American stroke association. Stroke. (2018) 49:e46–110. doi: 10.1161/STR.0000000000000158
94. Kim BM. Causes and solutions of endovascular treatment failure. J Stroke. (2017) 19:131–42. doi: 10.5853/jos.2017.00283
95. Nakahara T, Sakamoto S, Hamasaki O, Sakoda K. Double wire technique for intracranial stent navigation. J Vasc Interv Radiol. (2003) 14:667–8. doi: 10.1097/01.RVI.0000071092.76348.2E
96. Lee TH, Choi CH, Park KP, Sung SM, Lee SW, Lee BH, et al. Techniques for intracranial stent navigation in patients with tortuous vessels. AJNR Am J Neuroradiol. (2005) 26:1375–80.
97. Kim YW, Son S, Kang DH, Hwang YH, Kim YS. Endovascular thrombectomy for M2 occlusions: comparison between forced arterial suction thrombectomy and stent retriever thrombectomy. J Neurointerv Surg. (2017) 9:626–30. doi: 10.1136/neurintsurg-2016-012466
98. Lapergue B, Blanc R, Gory B, Labreuche J, Duhamel A, Marnat G, et al. Effect of endovascular contact aspiration vs stent retriever on revascularization in patients with acute ischemic stroke and large vessel occlusion: the ASTER randomized clinical trial. JAMA. (2017) 318:443–52. doi: 10.1001/jama.2017.9644
99. Lee JS, Hong JM, Lee SJ, Joo IS, Lim YC, Kim SY. The combined use of mechanical thrombectomy devices is feasible for treating acute carotid terminus occlusion. Acta Neurochir. (2013) 155:635–41. doi: 10.1007/s00701-013-1649-5
Keywords: atherosclerosis, neuroimage, endovascular therapy, acute ischemic stroke, intracranial
Citation: Bang OY, Kim BM, Seo W-K and Jeon P (2019) Endovascular Therapy for Acute Ischemic Stroke of Intracranial Atherosclerotic Origin—Neuroimaging Perspectives. Front. Neurol. 10:269. doi: 10.3389/fneur.2019.00269
Received: 22 November 2018; Accepted: 28 February 2019;
Published: 20 March 2019.
Edited by:
Osama O. Zaidat, St. Vincent Mercy Medical Center, United StatesReviewed by:
Syed Daniyal Asad, University of Connecticut, United StatesMahmoud Helmy Hafez Mohammaden, University of Illinois at Chicago, United States
Chris Southwood, Medical College of Wisconsin, United States
Shankar Balakrishnan, University Hospital Zürich, Switzerland
Copyright © 2019 Bang, Kim, Seo and Jeon. This is an open-access article distributed under the terms of the Creative Commons Attribution License (CC BY). The use, distribution or reproduction in other forums is permitted, provided the original author(s) and the copyright owner(s) are credited and that the original publication in this journal is cited, in accordance with accepted academic practice. No use, distribution or reproduction is permitted which does not comply with these terms.
*Correspondence: Oh Young Bang, b2h5b3VuZy5iYW5nQHNhbXN1bmcuY29t