- 1School of Health, Medical and Applied Sciences, Central Queensland University, Rockhampton, QLD, Australia
- 2School of Exercise and Nutrition Sciences, Institute for Physical Activity and Nutrition, Deakin University, Melbourne, VIC, Australia
- 3Physical Education and Sports Science Academic Group, National Institute of Education, Nanyang Technological University, Singapore, Singapore
- 4School of Science, RMIT University, Melbourne, VIC, Australia
Parkinson's disease (PD) is a heterogeneous neurodegenerative disorder that affects an estimated 10 million sufferers worldwide. The two forms of PD include familial and sporadic, and while the etiology of PD is still largely unknown, the condition is likely to be multifactorial with genetic and environmental factors contributing to disease genesis. Diagnosis of the condition is attained through the observation of cardinal clinical manifestations including resting tremor, muscle rigidity, slowness or loss of movement, and postural instability. Unfortunately, by the time these features become apparent extensive neurological damage has already occurred. A cure for PD has not been identified and the current therapy options are pharmaceutical- and/or surgical-based interventions to treat condition symptoms. There is no specific test for PD and most diagnoses are confirmed by a combination of clinical symptoms and positive responses to dopaminergic drug therapies. The prevalence and incidence of PD vary worldwide influenced by several factors such as age, gender, ethnicity, genetic susceptibilities, and environmental exposures. Here, we will present environmental factors implicated in sporadic PD onset. By understanding the mechanisms in which environmental factors interact with, and affect the brain we can stride toward finding the underlying cause(s) of PD.
Introduction
Parkinson's disease (PD) is characterized as a progressive neurodegenerative disorder that results in death (1) and affects ~1–3% of the global population aged over 60 years (2, 3). There are two forms of PD, familial; genetically inherited in either an autosomal dominant or recessive manner (4), and sporadic (idiopathic); believed to develop from gene-environment interactions (5). Genetically linked PD accounts for ~10–15% of all PD cases (6) with the remainder classed as sporadic. Seven causal genes have been identified for familial PD; alpha-synuclein (SNCA), glucocerebrosidase (GBA), leucine-rich repeat Kinase 2 (LRRK2), vacuolar protein sorting-associated protein 35 (VPS35) parkin RBR E3 ubiquitin protein ligase (PARK2), phosphatase and tensing homolog-induced Kinase 1 (PINK1), and Parkinson protein 7 (PARK7) (6, 7). These genes, along with specific metabolites (8) and PD-associated biomarkers (9–11) have been used to research possible early detection methods for PD. Due to an estimated 70% of neuronal death before the onset of clinical symptoms (12) early diagnosis of PD is essential.
Gene-environment interactions are understood to be the underlying cause of idiopathic PD. Human genotypes are independently unique and individuals exposed to the same environmental factor are affected differently, resulting in diverse disease phenotypes (13). The combined effect of genetic and environmental factors may influence the onset of human disease (14) by structurally altering deoxyribonucleic acid (DNA) (15). For example, caffeine is an adenosine A2A (ADORA2A gene) receptor antagonist which increases dopamine neurotransmission (16) and polymorphisms of ADORA2A have been found to reduce the risk of PD (16, 17). In contrast, pesticides and heavy metals display a negative effect and increase PD by causing gene variations linked to familial PD (e.g., PARK1, LRRK2, PINK1) resulting in PD-associated mechanisms such as mitochondrial dysfunction, oxidative stress and protein degradation impairment (18, 19).
Demographic factors including age, gender and ethnicity may also impact PD susceptibility. Age is a major risk factor for PD (20). Not only has the prevalence of PD been observed with increasing age (21), but so too the severity of disease symptoms (22). Most cases develop between the ages of 60–65 years however, young on-set (<50 years) and juvenile cases (<21 years) have also been identified (23). Gender may also predispose an individual to PD. The incidence of PD amongst men is higher than women (14). Estrogen may act as a neuro-protective agent (24), and women who have had a high lifetime exposure from such things as lengthy fertility windows and multiple births show a reduced risk of developing PD (25, 26).
The prevalence of PD due to ethnicity is sparse. Recent research has highlighted predominantly white Western populations in having higher PD prevalence than Asian nations (27). Older studies have shown a correlation between Caucasians and Hispanic races having an increased risk of developing PD when compared to Asian and Black cultures (28, 29). One reason for this could be, commonly, individuals with darker skin tones produce more melanin than fair skinned people (30), which may suggest increased levels of neuromelanin in the substantia nigra may have a neuro-protective effect. Another explanation lies in the socioeconomic state of a nation. Industrialized countries have higher urbanization leading to environmental degradation and exposure to environmental toxins (31). Research has shown areas in which socioeconomic status is higher equates to an increased incidence of PD (32).
Due to the diverse nature of the disease, its symptomology differs from one individual to the next. Some cases may be influenced by demographical factors such as age, gender, and ethnicity while others may be attributed to environmental, occupational, or residential exposure to neurotoxins which may selectively target substantia nigra neurons (33) including, heavy metals, pesticides and illicit drugs. This mini-review will focus on environmental factors which have been implicated in PD.
Environmental Contributors
Numerous environmental toxins have been implicated in the onset of PD, however, data has been inconsistent. Some studies suggest PD incidence may be linked to occupational exposure to chemicals (34–36). Several occupations, some with increased risk have been investigated, for example, agriculture, and working with pesticides (37) and heavy metals (38). Others having a null effect, such as electrical vocations, and working with extremely low frequency-magnetic fields (39), diesel motor emissions, or solvents (40) have also been examined. Rural residency as a causative factor for idiopathic PD has been a long-disputed topic amongst PD research. Some studies have found no link between rural living and PD, citing the opposite that urban living may lead to an increased risk (28, 41). Studies conducted on highly populated urban areas have also found significant correlations between industrial airborne heavy metal pollution (42, 43) and ambient air pollution from traffic (44, 45), and an augmented chance of PD onset. Other studies have found no difference between geographical location and the inflation of PD incidence (46, 47). Still, other investigations have associated rural exposure as a heightened risk (34, 48, 49). Elevated incidences of PD in rural locations may be attributed to these locations having a proportionately higher number of aged population (50).
Heavy Metals
Neurotoxins in the brain can lead to oxidative stress and neurotransmission disruption with detrimental effects in the basal ganglia (51). The generation of reactive oxygen species occurs when hydroxyl radicals are produced from hydrogen peroxide under the Fenton-Haber-Weiss reaction (52). This reaction may lead to oxidative stress (53) and neurotoxicity (54) thus, causing damage to numerous aspects of the cell with preferential damage to the mitochondria (55). Hydroxyl radicals react to deoxyribonucleic acid (DNA), membrane lipids, and proteins of the cell leading to their eventual dysfunction (51). Within the neuron, the reduction and metabolism of dopamine results in the generation of hydroxyl radicals as by-products, thus resulting in a ready supply of hydroxyl radicals within the substantia nigra pars compacta (51).
Numerous studies deny any relationship between PD etiology and heavy metals (56–60), while others have claimed an association (61–63). Iron is one heavy metal implicated due to the role it plays in the generation of oxidative stress (via the Fenton-Haber-Weiss reaction, Figure 1) leading to neuronal death (52), as well as its involvement in alpha-synuclein toxicity (51). A meta-analysis of five studies including 126, 507 PD individuals found no significant link between dietary iron and an increased risk of PD [moderate iron intake; relative risk [RR] 1.08, 95% CI 0.16–1.93, p = 0.787 and high iron intake; RR 1.03. 95% CI 0.83 – 1.80, p = 0.766] (56). This research indicates dietary intake of iron does not proliferate PD risk (56) giving more credibility to environmental pathways as vectors of susceptibility. The meta-analysis was robust in its design with stringent selection criteria. A limitation was differing methodology between the studies. Four studies used recent recall techniques whereas, the fifth used a past-history approach which may have resulted in recall bias.
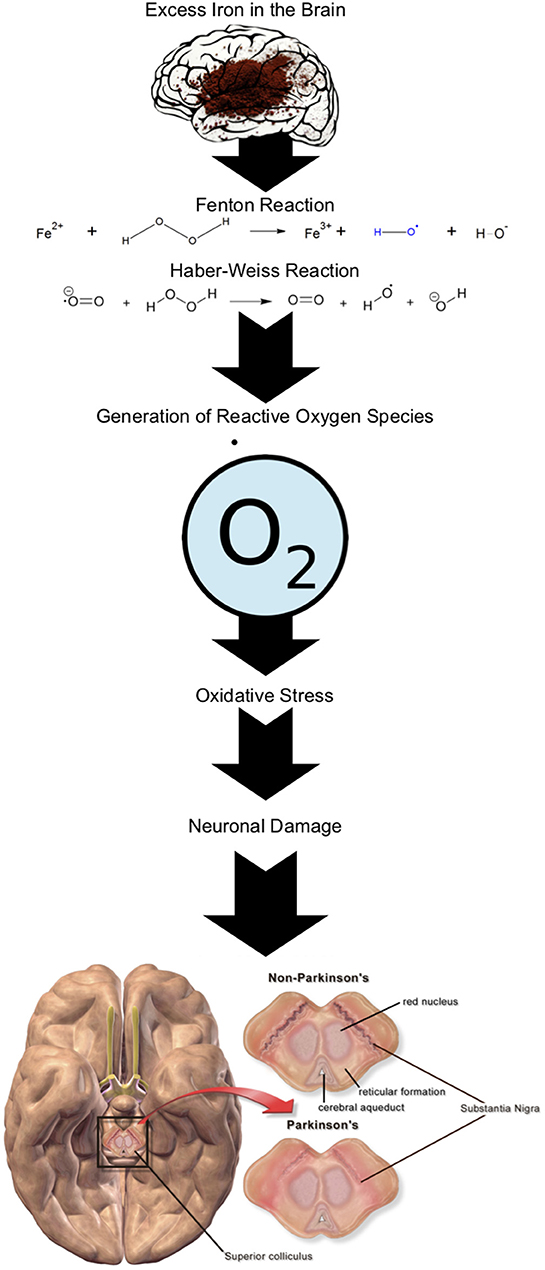
Figure 1. Fenton-Haber-Weiss reaction in the presence of Fe in the brain generating reactive oxygen species leading to neuronal damage ( superoxide anion radical).
Similar to iron, copper contributes to oxidative stress however copper has two modes of action; the Fenton-Haber-Weiss reaction (55) and 6-OHDA (6-hydroxydopamine) redox cycle (62). Accumulation of copper in the brain has seen the reduction of dopamine (64), alpha-synuclein aggregation (61) and the reduction of the protective factor for neuronal survival; superoxide dismutase 1 (64). Research shows that occupations which expose an individual to copper intensifies a PD risk (65). Gorell et al. conducted a 20-year investigation into the link between occupational heavy metal exposure and PD and found an individual with chronic copper exposure had a two-and-a-half-fold increased risk of PD [Odds Ratio [OR] 2.49, 95% CI 1.06–5.89] (65). To measure occupational exposure this study used an extensive “risk factor questionnaire.” Self-reporting questionnaires run the risk of recall bias. To minimize this, the investigators employed an experienced industrial hygienist blinded to a participant's status to assess job description concentrations. One flaw in the study design is, the researchers did not measure heavy metals concentrations within participating individuals which could have further supported their claims.
Manganese may amplify PD risk through manganese toxicity resulting in impaired motor function and damage to the substantia nigra and other basal ganglia nuclei (66). Exposure can occur through diet, occupation and environmental pathways (51, 67). Chronic manganese intoxication can clinically resemble idiopathic PD (68). Manganese-induced Parkinsonism may be identified due to its predilection to accumulate in and damage the palladium and striatum as opposed to the substantia nigra pars compacta in PD (69).
Lead exposure may see a two-to-three-fold increased risk of PD. Coon et al. (70) and Weisskopf et al. (71) used K-X-ray fluorescence on tibia bone (PD patients vs. controls) and both found an increased risk of PD in individuals with chronic lifetime exposure to lead; OR 2.27, 95% CI 1.13–4.55, p = 0.021 and OR 3.21, 95% CI 1.11–5.93, p = 0.03, respectively (70, 71). Bone measurement of Pb is a reliable tool as it is able to account for more than 90% of cumulative Pb burden in adults (72) when compared to blood measurement which can only give a result for recent exposure (4–6 weeks) (73). Additionally, the Coon et al. study strengthened their data by combining occupational exposure (assessed by an industrial hygienist) and blood Pb levels with their Pb bone results. Lead is able to enter the brain by mimicking calcium via calcium channels (70). Monnet-Tschudi et al. (74) exposure to lead can cause several neurological deficits including severe swelling and loss of neurons in the central nervous system (CNS) and peripheral nervous system, manifesting as motor-based dysfunction in the form of loss of voluntary muscle movement (51). Occupational exposure to lead, especially in the long-term, may increase concentration levels of lead within subjects, heightening PD risk (70, 71).
Mercury is a known neurotoxin which can cause neuronal death (75). Research is still conflicted regarding its participation in PD pathogenesis, however, mercury is present in organic (elemental mercury and methylmercury), and inorganic forms (51, 76) and can contaminate water sources including rain, ground, and sea. In the aquatic environment, inorganic mercury is the most toxic form when it is microbially transformed into methylmercury. The transformation also makes mercury prone to bioaccumulation and biomagnification leading to a potential food web transfer causing mercury-laced meat, vegetables, and fish (77). It has been reported mercury can reduce the number of neurons present in the brain (75) and cause movement disorders including tremors and loss of voluntary muscle movement (51).
Pesticides
In the 1980's interest in the action of 1-methyl-4-phenyl-1,2,3,6-tetrahydropyridine (MPTP) arose due to its ability to selectively target the substantia nigra. Patients who had been exposed to MPTP presented with textbook cases of advanced PD. The research discovered MPTP was metabolized by astrocytes in the brain to 1-methly-4-phenylpyridium (MPP+) which had a distinctively similar composition to a popular pesticide; paraquat thus, inciting the hypothesis of pesticides and their role in PD pathogenesis (78). The current evidence suggests there is an association between some pesticides and PD (Table 1).
Pesticides (including insecticides and herbicides) are used globally in public health to control disease vectors and in the agricultural industry to control pests and their subsequent diseases (83, 84). They have been consistently investigated due to their ability to affect neurological changes in the brain leading to the destruction of dopamine producing neurons (85). Insecticide classes commonly investigated include organochlorines and organophosphates. A well-known organochlorine pesticide associated with increasing the risk of PD is dieldrin which affects the CNS causing neurotoxic damage to the dopaminergic system (86). Two recent studies on mice models have confirmed preferential targeting of the dopaminergic system by dieldrin (87, 88) and both found the severity of the impact of dieldrin was dose-dependent. Rotenone is the major organophosphate constituent for raising PD risk and its mode of action is the acceleration of alpha-synuclein aggregation resulting in the death of dopaminergic neurons (89). Like dieldrin, its effects appear to be dose-dependent (90, 91). The question remains, are non-human models able to predict human health effects with enough efficacy? Correlation between animal models and human disease is only an estimated 60% (92).
PD associated risk and herbicide exposure are still unclear. Studies have found some correlation between PD risk and the use of a combination of herbicides (93) and occupational exposure (80) however, individual herbicide action has not been identified as causative. Tanner et al. conducted an occupational study and reported pesticide use, rather than occupation was a risk factor for PD (93). 2,4-Dichlorophenoxyacetic acid (2,4-D) a primary constituent in Agent Orange was identified as being statistically significant risk for PD (OR 2.59, 95% CI 1.03–6.48, p = 0.04). Also noted was the significant association between PD and exposure to any 1 of 8 specific pesticides (2,4-D, paraquat, permethrin, dieldrin, diquat, maneb, mancozeb, and rotenone) OR 2.20, 95% CI 1.02–4.75, p = 0.04. Hancock et al. also found a positive correlation for 2,4-D however, the association was not statistically significant (94). Other studies concluded no association between herbicides and increased PD risk (95, 96).
Many pesticide studies have looked at human exposures through population-based studies which can suffer from bias. These biases may occur in the forms of (a) recall bias with over- or under-estimation of exposure amount or time, (b) social bias where public approval may influence responses on sensitive topics (e.g., illicit drug use underestimation), (c) measurement error bias including systematic and random error, and (d) confirmation bias where a patient's preconceptions or beliefs can influence their response (97).
Illicit Substances
A history of illicit drug use has been found to cause abnormal morphology in the substantia nigra (98), such that, substantia nigra hyperechogenicity is a major risk factor for the development of PD (99). The abuse of illegal stimulants can elevate reactive oxygen species levels causing oxidative stress leading to dopamine neuron toxicity and death (100). Illicit drug residues have been found in river basins, surface waters, and wastewaters, with inadequately-treated municipal wastewater discharge, cited as the main vector of contamination (101). Water epidemiology studies have been used in recent times to estimate community drug use by measuring drug residues in waste-water (102–104). These studies have succeeded in the detection and estimation of drug residue concentrations in wastewater. Analysis of drinking and surface waters has found extrication rates of illicit drugs are inadequate, with results demonstrating detectable levels after treatment (101, 105–107). Due to the high production volume and use of illicit drugs, their residues may persist in the environment, and leach into other environmental media including sediments and biota (107, 108).
The three common psychostimulants implicated in PD are amphetamine, methamphetamine and cocaine (109–111). High doses of the stimulant drug amphetamine may cause damage to dopaminergic neurons and axon terminals within the human brain (112). Animal studies on non-human primates and rodents have found acute amphetamine exposure is toxic to dopamine terminals (113). Garwood et al. (109) through a telephone survey found the prolonged use of amphetamine may be a statistically significant risk factor for PD (OR 4.46, 95%CI 1.0–19.8, p = 0.049). This study had some limitations such as possible recall bias due to the human factor of the survey, a small sample size (n = 76 PD), and it did not distinguish between prescribed and non-prescribed amphetamine use. This is an important differentiation to be assessed as amphetamines are also used to treat PD (109).
In addition, methamphetamine, a highly water-soluble psychostimulant drug plays a role in decreasing the integrity of dopamine neuron terminals in the basal ganglia, reducing levels of dopamine, and dopamine transporters (114, 115). These effects can be long-term, causing damage to the dopamine terminal and death of dopamine neurons (114, 116, 117). A cohort study using hospital admission records found methamphetamine/amphetamine exposure increased PD risk nearly three-fold when compared to unexposed controls (Hazard Ratio 2.8, 95%CI 1.6–4.8, p = 0.01) (111). Although the study had a good sample size (PD n = 4935, control n = 24,675), due to no “gold standard” of PD diagnosis some members of the control group may have been undiagnosed. Additionally, researchers did not account for other medications or addictions (e.g., alcohol) which may cause PD-like symptoms.
Lastly, cocaine is a drug of abuse known to bind to dopamine transport proteins causing short-term inhibition of dopamine uptake (118, 119) however, no direct association has been found between an elevated risk of PD and cocaine use (110, 111). A study of 44 cocaine users vs. 44 controls medical records reported cocaine users showing excess iron accumulation in the Globus pallidus. This suggested cocaine addiction may lead to iron dysregulation (120) thus, possibly contributing further to oxidative stress. Although the effects of illicit drugs and their role in PD pathophysiology presently remain unclear, results so far have shown a strong argument for a possible role in neurodegeneration. Further continued research is warranted to fully understand these roles and associations.
Conclusion
In the 200 years since James Parkinson first described the “shaking palsy” phenomenon, significant research in roads toward diagnosis and causative associations with PD have been realized. Yet, science has still not been able to deduce the complete etiology of PD. There have, however, been many advances in the genetic inheritance of the disease. The role in which environmental factors interact to contribute to the pathophysiology of the disease remains elusive, however, as discussed, many demographical/environmental factors may be at play in the etiology of PD and may impact the severity of the disease. Age is a major risk factor for the condition, however, age alone may not be the only contributor to PD genesis. Gender, ethnicity, living circumstances, and occupation have been implicated in heightened PD risk nevertheless, data on this is still contradictory. Study design can also impact the reliability of data and reporting of causal links to PD. Using a mixed-method approach encompassing patient history as well as chemical analysis could improve study robustness especially in regards to population-based studies. Exposure to toxins in the environment has been linked to PD-associated neurodegeneration particularly heavy metals, pesticides, and illicit drugs. These avenues of exposure can be directly related to rural living, adding support to the argument that rural living may be a risk factor for PD itself. Each case of PD is specific to the individual and given the heterogeneity of the disease, one can hypothesize that individual susceptibility to environmental factors plays a large role in PD etiology. The complex nature of PD only adds to the difficulty in pinpointing its cause.
Author Contributions
Manuscript conceived by NB, W-PT, SC, and JC. Initial drafting completed by NB. Contributed and critically reviewed by NB, W-PT, SC, and JC. All authors read and approved the final manuscript.
Conflict of Interest Statement
The authors declare that the research was conducted in the absence of any commercial or financial relationships that could be construed as a potential conflict of interest.
Acknowledgments
This research higher degree candidature was supported under the Commonwealth Government's Research Training Program/Research Training Scheme. We gratefully acknowledge the financial support provided by the Australian Government. The work is a joint venture project between Central Queensland University, Deakin University, and RMIT University in Australia. The work acknowledges funding from Central Queensland University.
References
1. Scorza FA, do Carmo AC, Fiorini AC, Nejm MB, Scorza CA, Finsterer J, et al. Sudden unexpected death in Parkinson's disease (SUDPAR): a review of publications since the decade of the brain. Clinics. (2017) 72:649–51. doi: 10.6061/clinics/2017(11)01
2. Hirsch L, Jette N, Frolkis A, Steeves T, Pringsheim T. The Incidence of Parkinson's Disease: a systematic review and meta-analysis. Neuroepidemiology. (2016) 46:292–300. doi: 10.1159/000445751
3. Shalash AS, Hamid E, Elrassas HH, Bedair AS, Abushouk AI, Khamis M, et al. Non-motor symptoms as predictors of quality of life in egyptian patients with parkinson's disease: a cross-sectional study using a culturally adapted 39-item Parkinson's disease questionnaire. Front Neurol. (2018) 9:357. doi: 10.3389/fneur.2018.00357
4. Lill CM. Genetics of Parkinson's disease. Mol Cell Probes. (2016) 30:386–96. doi: 10.1016/j.mcp.2016.11.001
5. Gao H-M, Hong J-S. Gene-environment interactions: Key to unraveling the mystery of Parkinson's disease. Prog Neurobiol. (2011) 94:1–19. doi: 10.1016/j.pneurobio.2011.03.005
6. Verstraeten A, Theuns J, Van Broeckhoven C. Progress in unraveling the genetic etiology of Parkinson disease in a genomic era. Trends Genet. (2015) 31:140–9. doi: 10.1016/j.tig.2015.01.004
7. Kalinderi K, Bostantjopoulou S, Fidani L. The genetic background of Parkinson's disease: current progress and future prospects. Acta Neurol Scand. (2016) 134:314–26. doi: 10.1111/ane.12563
8. Zhao H, Wang C, Zhao N, Li W, Yang Z, Liu X, et al. Potential biomarkers of Parkinson's disease revealed by plasma metabolic profiling. J Chromatogr B Analyt Technol Biomed Life Sci. (2018) 1081–2:101–8. doi: 10.1016/j.jchromb.2018.01.025
9. Ma W, Qin L-X, Liu F-T, Gu Z, Wang J, Pan ZG, et al. Ubiquinone-quantum dot bioconjugates forin vitro and intracellular complex I sensing. Sci Rep. (2013) 3:1537. doi: 10.1038/srep01537
10. Ankireddy SR, Kim J. Selective detection of dopamine in the presence of ascorbic acid via fluorescence quenching of inp/zns quantum dots. Int J Nanomed. (2015) 10:113–9. doi: 10.2147/IJN.S88388
11. Kruse N, Schulz-Schaeffer WJ, Schlossmacher MG, Mollenhauer B. Development of electrochemiluminescence-based singleplex and multiplex assays for the quantification of α-synuclein and other proteins in cerebrospinal fluid. Methods. (2012) 56:514–8. doi: 10.1016/j.ymeth.2012.03.016
12. Jakubowski JL, Labrie V. Epigenetic biomarkers for Parkinson's disease: from diagnostics to therapeutics. J Park Dis. (2017) 7:1–12. doi: 10.3233/JPD-160914
13. Baye TM, Abebe T, Wilke RA. Genotype–environment interactions and their translational implications. Pers Med. (2011) 8:59–70. doi: 10.2217/pme.10.75
14. Lubomski M, Louise Rushworth R, Lee W, Bertram KL, Williams DR. Sex differences in Parkinson's disease. J Clin Neurosci. (2014) 21:1503–6. doi: 10.1016/j.jocn.2013.12.016
15. Bollati V, Baccarelli A. Environmental epigenetics. Heredity. (2010) 105:105–12. doi: 10.1038/hdy.2010.2
16. Chuang Y-H, Lill CM, Lee P-C, Hansen J, Lassen CF, Bertram L, et al. Gene-environment interaction in Parkinson's disease: coffee, ADORA2A, and CYP1A2. Neuroepidemiology. (2016) 47:192–200. doi: 10.1159/000450855
17. Popat RA, Eeden SKVD, Tanner CM, Kamel F, Umbach DM, Marder K, et al. Coffee, ADORA2A, and CYP1A2: the caffeine connection in Parkinson's disease. Eur J Neurol. (2011) 18:756–65. doi: 10.1111/j.1468-1331.2011.03353.x
18. Cannon JR, Greenamyre JT. Gene-environment interactions in Parkinson's disease: specific evidence in humans and mammalian models. Neurobiol Dis. (2013) 57:38–46. doi: 10.1016/j.nbd.2012.06.025
19. Fleming SM. Mechanisms of gene-environment interactions in Parkinson's disease. Curr Environ Health Rep. (2017) 4:192–9. doi: 10.1007/s40572-017-0143-2
20. Collier TJ, Kanaan NM, Kordower JH. Ageing as a primary risk factor for Parkinson's disease: evidence from studies of non-human primates. Nat Rev Neurosci. (2011) 12:359–66. doi: 10.1038/nrn3039
21. Wong SL, Gilmour H, Ramage-Morin PL. Parkinson's disease: prevalence, diagnosis and impact. Health Rep. (2014) 25:10–4. Available online at: https://www150.statcan.gc.ca/n1/en/pub/82-003-x/2014011/article/14112-eng.pdf?st=v1CPZHp7
22. Szewczyk-Krolikowski K, Tomlinson P, Nithi K, Wade-Martins R, Talbot K, Ben-Shlomo Y, et al. The influence of age and gender on motor and non-motor features of early Parkinson's disease: initial findings from the Oxford Parkinson Disease Center (OPDC) discovery cohort. Parkinsonism Relat Disord. (2014) 20:99–105. doi: 10.1016/j.parkreldis.2013.09.025
23. Paviour DC, Surtees RAH, Lees AJ. Diagnostic considerations in juvenile parkinsonism. Mov Disord. (2004) 19:123–35. doi: 10.1002/mds.10644
24. Zárate S, Stevnsner T, Gredilla R. Role of estrogen and other sex hormones in brain aging. Neuroprotection and DNA repair. Front Aging Neurosci. (2017) 9:430. doi: 10.3389/fnagi.2017.00430
25. Smith KM, Dahodwala N. Sex differences in Parkinson's disease and other movement disorders. Exp Neurol. (2014) 259:44–56. doi: 10.1016/j.expneurol.2014.03.010
26. Frentzel D, Judanin G, Borozdina O, Klucken J, Winkler J, Schlachetzki JCM. Increase of reproductive life span delays age of onset of Parkinson's disease. Front Neurol. (2017) 8:397. doi: 10.3389/fneur.2017.00397
27. Pringsheim T, Jette N, Frolkis A, Steeves TDL. The prevalence of Parkinson's disease: a systematic review and meta-analysis. Mov Disord. (2014) 29:1583–90. doi: 10.1002/mds.25945
28. Wright Willis A, Evanoff BA, Lian M, Criswell SR, Racette BA. Geographic and ethnic variation in Parkinson disease: a population-based study of US medicare beneficiaries. Neuroepidemiology. (2010) 34:143–51. doi: 10.1159/000275491
29. Van Den Eeden KS, Tanner CM, Bernstein AL, Fross RD, Leimpeter A, Bloch DA, et al. Incidence of Parkinson's disease: variation by age, gender, and race/ethnicity. Am J Epidemiol. (2003) 157:1015–22. doi: 10.1093/aje/kwg068
30. Pan T, Li X, Jankovic J. The association between Parkinson's disease and melanoma. Int J Cancer. (2011) 128:2251–60. doi: 10.1002/ijc.25912
31. Chinta SJ, Lieu CA, DeMaria M, Laberge R-M, Campisi J, Andersen JK. Environmental stress, ageing and glial cell senescence: a novel mechanistic link to Parkinson's disease?J Intern Med. (2013) 273:429–36. doi: 10.1111/joim.12029
32. Yang F, Johansson ALV, Pedersen NL, Fang F, Gatz M, Wirdefeldt K. Socioeconomic status in relation to Parkinson's disease risk and mortality. Medicine. (2016) 95:e4337. doi: 10.1097/MD.0000000000004337
33. Di Monte DA. The environment and Parkinson's disease: is the nigrostriatal system preferentially targeted by neurotoxins?Lancet Neurol. (2003) 2:531–8. doi: 10.1016/S1474-4422(03)00501-5
34. Pezzoli G, Cereda E. Exposure to pesticides or solvents and risk of Parkinson disease. Neurology. (2013) 80:2035. doi: 10.1212/WNL.0b013e318294b3c8
35. Gamache P-L, Roux-Dubois N, Provencher P, Lebouthiller J, Gan-Or Z, Dupre N. Professional exposure to pesticides and heavy metals hastens Parkinson Disease onset (P6.008). Neurology. (2017) 88:P6.008. Available online at: https://n.neurology.org/content/88/16_Supplement/P6.008
36. Elbaz A, Clavel J, Rathouz PJ, Moisan F, Galanaud J-P, Delemotte B, et al. Professional exposure to pesticides and Parkinson disease. Ann Neurol. (2009) 66:494–504. doi: 10.1002/ana.21717
37. Pouchieu C, Piel C, Carles C, Gruber A, Helmer C, Tual S, et al. Pesticide use in agriculture and Parkinson's disease in the AGRICAN cohort study. Int J Epidemiol. (2018) 47:299–310. doi: 10.1093/ije/dyx225
38. Castillo S, Muñoz P, Behrens MI, Diaz-Grez F, Segura-Aguilar J. On the role of mining exposure in epigenetic effects in Parkinson's disease. Neurotox Res. (2017) 32:172–4. doi: 10.1007/s12640-017-9736-7
39. Gunnarsson L-G, Bodin L. Parkinson's disease and occupational exposures: a systematic literature review and meta-analyses. Scand J Work Environ Health. (2017) 43:197–209. doi: 10.5271/sjweh.3641
40. Brouwer M, Koeman T, van den Brandt PA, Kromhout H, Schouten LJ, Peters S, et al. Occupational exposures and Parkinson's disease mortality in a prospective Dutch cohort. Occup Environ Med. (2015) 72:448–55. doi: 10.1136/oemed-2014-102209
41. Chen CC, Chen TF, Hwang YC, Wen YR, Chiu YH, Wu CY, et al. Different prevalence rates of Parkinson's Disease in Urban and Rural Areas: a population-based study in Taiwan. Neuroepidemiology. (2009) 33:350–7. doi: 10.1159/000254572
42. Willis AW, Evanoff BA, Lian M, Galarza A, Wegrzyn A, Schootman M, et al. Metal emissions and urban incident Parkinson disease: a community health study of medicare beneficiaries by using geographic information systems. Am J Epidemiol. (2010) 172:1357–63. doi: 10.1093/aje/kwq303
43. Palacios N. Air pollution and Parkinson's disease – evidence and future directions. Rev Environ Health. (2017) 32:303–13. doi: 10.1515/reveh-2017-0009
44. Ritz B, Lee P-C, Hansen J, Lassen CF, Ketzel M, Sørensen M, et al. Traffic-related air pollution and Parkinson's disease in Denmark: a case–control study. Environ Health Perspect. (2016) 124:351–6. doi: 10.1289/ehp.1409313
45. Finkelstein MM, Jerrett M. A study of the relationships between Parkinson's disease and markers of traffic-derived and environmental manganese air pollution in two Canadian cities. Environ Res. (2007) 104:420–32. doi: 10.1016/j.envres.2007.03.002
46. Walker RW, Hand A, Jones C, Wood BH, Gray WK. The prevalence of Parkinson's disease in a rural area of North-East England. Parkinsonism Relat Disord. (2010) 16:572–5. doi: 10.1016/j.parkreldis.2010.07.002
47. de Rijk MC, Tzourio C, Breteler MM, Dartigues JF, Amaducci L, Lopez-Pousa S, et al. Prevalence of parkinsonism and Parkinson's disease in Europe: the EUROPARKINSON Collaborative Study. European community concerted action on the epidemiology of Parkinson's disease. J Neurol Neurosurg Psychiatry. (1997) 62:10–5. doi: 10.1136/jnnp.62.1.10
48. Peters CM, Gartner CE, Silburn PA, Mellick GD. Prevalence of Parkinson's disease in metropolitan and rural Queensland: a general practice survey. J Clin Neurosci. (2006) 13:343–8. doi: 10.1016/j.jocn.2005.04.015
49. Osaki Y, Morita Y, Kuwahara T, Miyano I, Doi Y. Prevalence of Parkinson's disease and atypical parkinsonian syndromes in a rural Japanese district. Acta Neurol Scand. (2011) 124:182–7. doi: 10.1111/j.1600-0404.2010.01442.x
51. Caudle WM, Guillot TS, Lazo CR, Miller GW. Industrial toxicants and Parkinson's disease. Neuro Toxicol. (2012) 33:178–88. doi: 10.1016/j.neuro.2012.01.010
52. Hellman NE, Gitlin JD. Ceruloplasmin metabolism and function. Annu Rev Nutr. (2002) 22:439–58. doi: 10.1146/annurev.nutr.22.012502.114457
53. Scheiber IF, Mercer JFB, Dringen R. Metabolism and functions of copper in brain. Prog Neurobiol. (2014) 116:33–57. doi: 10.1016/j.pneurobio.2014.01.002
54. Bhat AH, Dar KB, Anees S, Zargar MA, Masood A, Sofi MA, et al. Oxidative stress, mitochondrial dysfunction and neurodegenerative diseases; a mechanistic insight. Biomed Pharmacother. (2015) 74:101–10. doi: 10.1016/j.biopha.2015.07.025
55. Paris I, Perez-Pastene C, Couve E, Caviedes P, Ledoux S, Segura-Aguilar J. Copper dopamine complex induces mitochondrial autophagy preceding caspase-independent apoptotic cell death. J Biol Chem. (2009) 284:13306–15. doi: 10.1074/jbc.M900323200
56. Cheng P, Yu J, Huang W, Bai S, Zhu X, Qi Z, et al. Dietary intake of iron, zinc, copper, and risk of Parkinson's disease: a meta-analysis. Neurol Sci. (2015) 36:2269–75. doi: 10.1007/s10072-015-2349-0
57. Jiménez-Jiménez FJ, Fernández-Calle P, Martínez-Vanaclocha M, Herrero E, Molina JA, Vázquez A, et al. Serum levels of zinc and copper in patients with Parkinson's disease. J Neurol Sci. (1992) 112:30–3.
58. Meamar R, Nikyar H, Dehghani L, Basiri K, Ghazvini MRA. Assessing of plasma levels of iron, zinc and copper in Iranian Parkinson's disease. Adv Biomed Res. (2016) 5:31. doi: 10.4103/2277-9175.178788
59. Mariani S, Ventriglia M, Simonelli I, Donno S, Bucossi S, Vernieri F, et al. Fe and Cu do not differ in Parkinson's disease: a replication study plus meta-analysis. Neurobiol Aging. (2013) 34:632–3. doi: 10.1016/j.neurobiolaging.2012.05.015
60. Semchuk KM, Love EJ, Lee RG. Parkinson's disease: a test of the multifactorial etiologic hypothesis. Neurology. (1993) 43:1173–80.
61. Rose F, Hodak M, Bernholc J. Mechanism of copper(II)-induced misfolding of Parkinson's disease protein. Sci Rep. (2011) 1:11. doi: 10.1038/srep00011
62. Cruces-Sande A, Méndez-Álvarez E, Soto-Otero R. Copper increases the ability of 6-hydroxydopamine to generate oxidative stress and the ability of ascorbate and glutathione to potentiate this effect: potential implications in Parkinson's disease. J Neurochem. (2017) 141:738–49. doi: 10.1111/jnc.14019
63. Fukushima T, Tan X, Luo Y, Kanda H. Serum vitamins and heavy metals in blood and urine, and the correlations among them in Parkinson's disease patients in China. Neuroepidemiology. (2011) 36:240–4. doi: 10.1159/000328253
64. Yu W-R, Jiang H, Wang J, Xie J-X. Copper (Cu2+) induces degeneration of dopaminergic neurons in the nigrostriatal system of rats. Neurosci Bull. (2008) 24:73–8. doi: 10.1007/s12264-008-0073-y
65. Gorell JM, Johnson CC, Rybicki BA, Peterson EL, Kortsha GX, Brown GG, et al. Occupational exposures to metals as risk factors for Parkinson's disease. Neurology. (1997) 48:650–8. doi: 10.1212/WNL.48.3.650
66. Aschner M, Nass R. Colloquium C012: manganese in CNS neurotoxicity and idiopathic Parkinson's disease. J Neurochem. (2006) 96:89–90. doi: 10.1111/j.1471-4159.2006.tuesdaysession6.x
67. Kwakye GF, Paoliello MMB, Mukhopadhyay S, Bowman AB, Aschner M. Manganese-induced parkinsonism and Parkinson's disease: Shared and distinguishable features. Int J Environ Res Public Health. (2015) 12:7519. doi: 10.3390/ijerph120707519
68. Guilarte TR, Gonzales KK. Manganese-induced parkinsonism is not idiopathic Parkinson's disease: Environmental and genetic evidence. Toxicol Sci. (2015) 146:204–12. doi: 10.1093/toxsci/kfv099
69. Olanow CW. Manganese-induced parkinsonism and Parkinson's disease. Ann N Y Acad Sci. (2004) 1012:209–23. doi: 10.1196/annals.1306.018
70. Coon S, Stark A, Peterson E, Gloi A, Kortsha G, Pounds J, et al. Whole-body lifetime occupational lead exposure and risk of Parkinson's disease. Environ Health Perspect. (2006) 114:1872. doi: 10.1289/ehp.9102
71. Weisskopf MG, Weuve J, Nie H, Saint-Hilaire M-H, Sudarsky L, Simon DK, et al. Association of cumulative lead exposure with Parkinson's disease. Environ Health Perspect. (2010) 118:1609–13. doi: 10.1289/ehp.1002339
72. Barry PS. A comparison of concentrations of lead in human tissues. Br J Ind Med. (1975) 32:119–39.
73. Brito JAA, McNeill FE, Webber CE, Chettle DR. Grid search: an innovative method for the estimation of the rates of lead exchange between body compartments. J Environ Monit. (2005) 7:241–7. doi: 10.1039/B416054A
74. Monnet-Tschudi F, Zurich M-G, Boschat C, Corbaz A, Honegger P. Involvement of environmental mercury and lead in the etiology of neurodegenerative diseases. Rev Environ Health. (2006) 21:105–17. doi: 10.1515/REVEH.2006.21.2.105
75. Fernandes Azevedo B, Barros Furieri L, Peçanha FM, Wiggers GA, Frizera Vassallo P, Ronacher Simões M, et al. Toxic effects of mercury on the cardiovascular and central nervous systems. J Biomed Biotechnol. (2012) 2012:949048. doi: 10.1155/2012/949048
76. Rooney JPK. The retention time of inorganic mercury in the brain — A systematic review of the evidence. Toxicol Appl Pharmacol. (2014) 274:425–35. doi: 10.1016/j.taap.2013.12.011
77. Zahir F, Rizwi SJ, Haq SK, Khan RH. Low dose mercury toxicity and human health. Environ Toxicol Pharmacol. (2005) 20:351–60. doi: 10.1016/j.etap.2005.03.007
79. Tanner CM, Kamel F, Ross GW, Hoppin JA, Goldman SM, Korell M, et al. Rotenone, paraquat, and Parkinson's disease. Environ Health Perspect. (2011) 119:866. doi: 10.1289/ehp.1002839
80. Wang A, Costello S, Cockburn M, Zhang X, Bronstein J, Ritz B. Parkinson's disease risk from ambient exposure to pesticides. Eur J Epidemiol. (2011) 26:547–55. doi: 10.1007/s10654-011-9574-5
81. Dick FD, De Palma G, Ahmadi A, Scott NW, Prescott GJ, Bennett J, et al. Environmental risk factors for Parkinson's disease and parkinsonism: the Geoparkinson study. Occup Environ Med. (2007) 64:666–72. doi: 10.1136/oem.2006.027003
83. García-García CR, Parrón T, Requena M, Alarcón R, Tsatsakis AM, Hernández AF. Occupational pesticide exposure and adverse health effects at the clinical, hematological and biochemical level. Life Sci. (2016) 145:274–83. doi: 10.1016/j.lfs.2015.10.013
84. World Health Organisation. Pesticides. World Health Organisation (2017) Available online at: http://www.who.int/topics/pesticides/en/ (Accessed August 8, 2018).
85. Elbaz A, Moisan F. The scientific bases to consider Parkinson's disease an occupational disease in agriculture professionals exposed to pesticides in France. J Epidemiol Community Health Lond. (2016) 70:319. doi: 10.1136/jech-2015-205455
86. Kanthasamy AG, Kitazawa M, Kanthasamy A, Anantharam V. Dieldrin-induced neurotoxicity: Relevance to Parkinson's disease pathogenesis. NeuroToxicol. (2004)701–19. doi: 10.1016/j.neuro.2004.07.010
87. Richardson JR, Caudle WM, Wang M, Dean ED, Pennell KD, Miller GW. Developmental exposure to the pesticide dieldrin alters the dopamine system and increases neurotoxicity in an animal model of Parkinson's disease. FASEB J. (2006) 20:1695–7. doi: 10.1096/fj.06-5864fje
88. Hatcher JM, Richardson JR, Guillot TS, McCormack AL, Di Monte DA, Jones DP, et al. Dieldrin exposure induces oxidative damage in the mouse nigrostriatal dopamine system. Exp Neurol. (2007) 204:619–30. doi: 10.1016/j.expneurol.2006.12.020
89. Dawson TM, Dawson VL. Molecular pathways of neurodegeneration in Parkinson's disease. Science. (2003) 302:819–22. doi: 10.1126/science.1087753
90. Uversky VN, Li J, Bower K, Fink AL. Synergistic effects of pesticides and metals on the fibrillation of alpha-synuclein: implications for Parkinson's disease. Neurotoxicology. (2002) 23:527–36. doi: 10.1016/S0161-813X(02)00067-0
91. Pan-Montojo F, Anichtchik O, Dening Y, Knels L, Pursche S, Jung R, et al. Progression of Parkinson's disease pathology is reproduced by intragastric administration of rotenone in mice. PLoS ONE. (2010) 5:e8762. doi: 10.1371/journal.pone.0008762
92. Hartung T. Thoughts on limitations of animal models. Parkinsonism Relat Disord. (2008) 14:S81–3. doi: 10.1016/j.parkreldis.2008.04.003
93. Tanner CM, Ross GW, Jewell SA, Hauser RA, Jankovic J, Factor SA, et al. Occupation and risk of parkinsonism: a multicenter case-control study. Arch Neurol. (2009) 66:1106–13. doi: 10.1001/archneurol.2009.195
94. Hancock DB, Martin ER, Mayhew GM, Stajich JM, Jewett R, Stacy MA, et al. Pesticide exposure and risk of Parkinson's disease: A family-based case-control study. BMC Neurol. (2008) 8:6. doi: 10.1186/1471-2377-8-6
95. Rugbjerg K, Harris MA, Shen H, Marion SA, Tsui JKC, Teschke K. Pesticide exposure and risk of Parkinson's disease — a population-based case—control study evaluating the potential for recall bias. Scand J Work Environ Health. (2011) 37:427–36. doi: 10.5271/sjweh.3142
96. Wan N, Lin G. Parkinson's disease and pesticides exposure: new findings from a comprehensive study in Nebraska, USA. J Rural Health Off J Am Rural Health Assoc Natl Rural Health Care Assoc. (2016) 32:303–13. doi: 10.1111/jrh.12154
97. Althubaiti A. Information bias in health research: definition, pitfalls, and adjustment methods. J Multidiscip Healthc. (2016) 9:211–7. doi: 10.2147/JMDH.S104807
98. Todd G, Pearson-Dennett V, Wilcox RA, Chau MT, Thoirs K, Thewlis D, et al. Adults with a history of illicit amphetamine use exhibit abnormal substantia nigra morphology and parkinsonism. Parkinsonism Relat Disord. (2016) 25:27–32. doi: 10.1016/j.parkreldis.2016.02.019
99. Todd G, Noyes C, Flavel SC, Della Vedova CB, Spyropoulos P, Chatterton B, et al. Illicit stimulant use is associated with abnormal substantia nigra morphology in humans. PLoS ONE. (2013) 8:e56438. doi: 10.1371/journal.pone.0056438
100. Mursaleen LR, Stamford JA. Drugs of abuse and Parkinson's disease. Prog Neuropsychopharmacol Biol Psychiatry. (2016) 64:209–17. doi: 10.1016/j.pnpbp.2015.03.013
101. Kasprzyk-Hordern B, Dinsdale RM, Guwy AJ. The removal of pharmaceuticals, personal care products, endocrine disruptors and illicit drugs during wastewater treatment and its impact on the quality of receiving waters. Water Res. (2009) 43:363–80. doi: 10.1016/j.watres.2008.10.047
102. Karolak S, Nefau T, Bailly E, Solgadi A, Levi Y. Estimation of illicit drugs consumption by wastewater analysis in Paris area (France). Forensic Sci Int. (2010) 200:153–60. doi: 10.1016/j.forsciint.2010.04.007
103. Lai FY, Thai PK, O'Brien J, Gartner C, Bruno R, Kele B, et al. Using quantitative wastewater analysis to measure daily usage of conventional and emerging illicit drugs at an annual music festival. Drug Alcohol Rev. (2013) 32:594–602. doi: 10.1111/dar.12061
104. Lai FY, Bruno R, Hall W, Gartner C, Ort C, Kirkbride P, et al. Profiles of illicit drug use during annual key holiday and control periods in Australia: wastewater analysis in an urban, a semi-rural and a vacation area. Addiction. (2013) 108:556–65. doi: 10.1111/add.12006
105. Huerta-Fontela M, Galceran MT, Ventura F. Stimulatory drugs of abuse in surface waters and their removal in a conventional drinking water treatment plant. Environ Sci Technol. (2008) 42:6809–16. doi: 10.1021/es800768h
106. Boleda MR, Galceran MT, Ventura F. Monitoring of opiates, cannabinoids and their metabolites in wastewater, surface water and finished water in Catalonia, Spain. Water Res. (2009) 43:1126–36. doi: 10.1016/j.watres.2008.11.056
107. Postigo C, López de Alda MJ, Barceló D. Drugs of abuse and their metabolites in the Ebro River basin: Occurrence in sewage and surface water, sewage treatment plants removal efficiency, and collective drug usage estimation. Environ Int. (2010) 36:75–84. doi: 10.1016/j.envint.2009.10.004
108. Hunter DJ. Gene–environment interactions in human diseases. Nat Rev Genet. (2005) 6:287–98. doi: 10.1038/nrg1578
109. Garwood ER, Bekele W, McCulloch CE, Christine CW. Amphetamine exposure is elevated in Parkinson's disease. NeuroToxicology. (2006) 27:1003–6. doi: 10.1016/j.neuro.2006.03.015
110. Callaghan RC, Cunningham JK, Sykes J, Kish SJ. Increased risk of Parkinson's disease in individuals hospitalized with conditions related to the use of methamphetamine or other amphetamine-type drugs. Drug Alcohol Depend. (2012) 120:35–40. doi: 10.1016/j.drugalcdep.2011.06.013
111. Curtin K, Fleckenstein AE, Robison RJ, Crookston MJ, Smith KR, Hanson GR. Methamphetamine/amphetamine abuse and risk of Parkinson's disease in Utah: a population-based assessment. Drug Alcohol Depend. (2015) 146:30–8. doi: 10.1016/j.drugalcdep.2014.10.027
112. Berman S, O'Neill J, Fears S, Bartzokis G, London ED. Abuse of amphetamines and structural abnormalities in brain. Ann N Y Acad Sci. (2008) 1141:195–220. doi: 10.1196/annals.1441.031
113. Yamamoto BK, Moszczynska A, Gudelsky GA. Amphetamine toxicities classical and emerging mechanisms. Ann N Y Acad Sci. (2010) 1187:101–21. doi: 10.1111/j.1749-6632.2009.05141.x
114. Granado N, Ares-Santos S, Moratalla R. Methamphetamine and Parkinson's disease. Park Dis. (2013) 2013:e308052. doi: 10.1155/2013/308052
115. Guilarte TR. Is methamphetamine abuse a risk factor in parkinsonism?NeuroToxicol. (2001) 22:725–1. doi: 10.1016/S0161-813X(01)00046-8
116. Ares-Santos S, Granado N, Moratalla R. The role of dopamine receptors in the neurotoxicity of methamphetamine. J Intern Med. (2013) 273:437–53. doi: 10.1111/joim.12049
117. Morrow BA, Roth RH, Redmond DE, Elsworth JD. Impact of methamphetamine on dopamine neurons in primates is dependent on age: implications for development of Parkinson's disease. Neuroscience. (2011) 189:277–85. doi: 10.1016/j.neuroscience.2011.05.046
118. Scheel-Krüger J, Braestrup C, Nielson M, Golembiowska K, Mogilnicka E. Cocaine: discussion on the role of dopamine in the biochemical mechanism of action. In:Cocaine and Other Stimulants Advances in Behavioral Biology. Ellinwood EH Jr, and Kilbey MM, editors. Boston, MA: Springer (1977). p. 373–407. doi: 10.1007/978-1-4684-3087-5_19
119. Hummel M, Unterwald EM. D1 dopamine receptor: a putative neurochemical and behavioral link to cocaine action. J Cell Physiol. (2002) 191:17–27. doi: 10.1002/jcp.10078
Keywords: Parkinson's disease, demographical, environmental, pesticides, heavy metals, illicit drugs
Citation: Ball N, Teo W-P, Chandra S and Chapman J (2019) Parkinson's Disease and the Environment. Front. Neurol. 10:218. doi: 10.3389/fneur.2019.00218
Received: 29 August 2018; Accepted: 20 February 2019;
Published: 19 March 2019.
Edited by:
Steven Jacobson, National Institute of Neurological Disorders and Stroke (NINDS), United StatesReviewed by:
Judith Walters, National Institute of Neurological Disorders and Stroke (NINDS), United StatesSonja W. Scholz, National Institutes of Health (NIH), United States
Anelyssa D'Abreu, Brown University, United States
Copyright © 2019 Ball, Teo, Chandra and Chapman. This is an open-access article distributed under the terms of the Creative Commons Attribution License (CC BY). The use, distribution or reproduction in other forums is permitted, provided the original author(s) and the copyright owner(s) are credited and that the original publication in this journal is cited, in accordance with accepted academic practice. No use, distribution or reproduction is permitted which does not comply with these terms.
*Correspondence: Shaneel Chandra, s.chandra@cqu.edu.au
James Chapman, james.chapman@rmit.edu.au