- 1Neuropsychology Unit, Neurology Department, University Hospital of Geneva, Geneva, Switzerland
- 2Neurology and Cognitive Imaging Laboratory, Faculty of Medicine, University of Geneva, Geneva, Switzerland
- 3Psychology Department, University of Montréal, Montréal, QC, Canada
- 4CNRS, LNSC, Aix-Marseille University, Marseille, France
- 5SCALab, CNRS, Lille University, Lille, France
Vertical representation is central to posture control, as well as to spatial perception and navigation. This representation has been studied for a long time in patients with vestibular disorders and more recently in patients with hemispheric damage, in particular in those with right lesions causing spatial or postural deficits. The aim of the study was to determine the brain areas involved in the visual perception of the vertical. Sixteen right-handed healthy participants were evaluated using fMRI while they were judging the verticality of lines or, in a control task, the color of the same lines. The brain bases of the vertical perception proved to involve a bilateral temporo-occipital and parieto-occipital cortical network, with a right dominance tendency, associated with cerebellar and brainstem areas. Consistent with the outcomes of neuroanatomical studies in stroke patients, The data of this original fMRI study in healthy subjects provides new insights into brain networks associated with vertical perception which is typically impaired in both vestibular and spatial neglect patients. Interestingly, these networks include not only brain areas associated with postural control but also areas implied in body representation.
Introduction
The transition to bipedalism in man had many implications on orientation and navigation skills. The vertical position freed the hands, modified the perception of the environment (with a largest horizon) and of the body, and drastically changed social interaction (1). Based on multisensory integration of visual, vestibular and somesthetic origin (2–4), the representation of the vertical makes it possible to reference the positions and displacements of our body as well as surrounding objects with respect to gravity. There is clinical evidence of deficits in verticality perception after peripheral vestibular loss (5–7) or central lesions (8–10). Neuroimagery performed in brain damaged patients suggested that several cortical regions could participate in a cortical network of vertical perception. Indeed, impaired vertical judgments were reported in patients with damage of the posterior parietal and temporal cortices (9, 10) or of the posterior insula (11, 12). Though the studies carried out in brain damaged patients certainly provided precious data, the issue remains poorly explored in healthy participants and the precise functional neuroanatomy of vertical perception is still uncertain. To our knowledge, only one study using high-density electrical neuroimaging showed an early potential map specific to the visual judgement of the vertical in the right temporal-occipital cortex, followed by a bilateral map in the temporal-occipital and parietal-occipital cortices (13).
In the present study, we use functional magnetic resonance imaging (fMRI) in order to describe, with a better spatial precision and in healthy participants, the brain areas involved in the visual judgment of the vertical. For this purpose, a special set of stimuli was designed that could be used in a main verticality judgment task and in a control color judgment task as well.
Methods
Subjects
Sixteen healthy volunteers (mean age: 25.7 ± 5.8 yr; 6 males and 10 females) were recruited from the general population. All participants signed an informed consent according to the ethics rules of the University Hospital of Geneva. Exclusion criteria were: past history of cerebral disease, epilepsy, head trauma, vestibular disorders or major psychiatric illness; visual acuity below 20/40; left handedness; pregnancy; claustrophobia or contraindication to magnetic field exposure (pacemaker, metallic prosthesis, dental apparatus, etc.); addiction or intake of any drug interfering with neuronal activity or cerebral blood flow.
Behavioral Design
The tasks were designed to assess the perception of the verticality. On each trial, a vertical line (height = 10°) was presented. The thickness of the line (1°) was sufficient to be clearly visible. The vertical line was presented 24 times straight (0°) and 36 times tilted by −30°, −25°, −20°, −15°, −10°, −5°, 5°, 10°, 15°, 20°, 25°, or 30° (3 times each). A circle and an irregular frame were also presented on the screen so as to avoid systematic strategies and frame effects (14).
The line was presented for 1,500 ms and followed by an inter-trial interval of 1,500–3,000 ms (pseudo-randomly jittered). All visual stimuli were projected on a screen in the MRI scanner and seen through a mirror mounted on the head coil.
All stimuli configurations were shown in two tasks requiring a similar binary response (yes / no) indicated by a key-press: a line verticality (LV) task, in which the participants judged whether the line was aligned with the true vertical and a line color (LC) task, in which they judged whether the line was red or green (control task).
Procedure
Before acquiring fMRI data, the perceived vertical was compared in supine (like in the MRI device) and in sitting positions. In both postural conditions, the percentage of correct responses and the response times were analyzed for LV and LC tasks. Here, the aim was to determine whether, in the present experimental conditions, the body position (supine or sitting) differentially affected the performance in the two tasks.
During fMRI acquisition, the two tasks were administered in a blocked design to maximize signal-to-noise ratio and to minimize attentional demand. Each block lasted 24 s and included 6 stimuli. In a given fMRI run, five blocks of each task were presented in a pseudo-random order, with brief resting periods (total duration 5 min). Two fMRI runs were obtained in each participant (duration 2 × 5min), separated by a brief pause (Figure 1). The positions and tilts of the stimuli were equally distributed between the tasks.
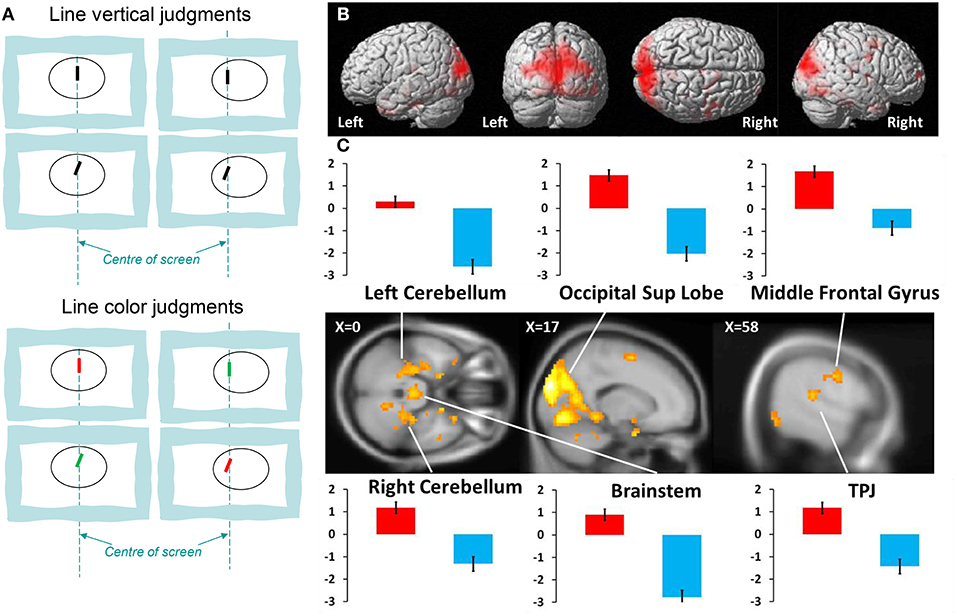
Figure 1. (A) Examples of stimuli for Line Verticality (LV) judgment and Line Color (LC) judgment; (B) activation in the whole group (n = 16) during LV vs. LC control tasks (P < 0.001 uncorrected; cluster size > 10); (C) fMRI analyses for vertical task. Activated brain regions are projected on a standard anatomical template. Parameter estimates of activity (beta value, in arbitrary units, averaged across responsive voxels in each cluster) are shown for main peaks in each task condition. Red bars, vertical task; blue bars, control task; TPJ, temporo-parietal junction.
Acquisition of fMRI Data
MRI data were acquired in the Brain and Behavior Laboratory at the University Medical Center, using a 3-T whole-body TRIO system (Siemens) with the standard head-coil configuration. Functional T2*-weighted images were obtained using echoplanar imaging (EPI) with axial slices (TR/TE/Flip = 2,200 ms/30 ms/85°, FOV = 235 mm, matrix = 128 × 128). Each functional volume was comprised of 32 contiguous 3.5 mm-thick slices, parallel to the inferior surface of occipital and temporal lobes. For each patient, a high-resolution anatomical image was also acquired after the functional scans, using a 3D-GRE T1-weighted sequence (FOV = 250 mm, TR/TE/Flip = 15 ms/5.0 ms/30°, matrix = 256 × 256, slice-thickness = 1.25 mm). This anatomical image was used for co-registration with functional images and subsequent normalization procedure.
Analysis of Behavioral Data
In the first part (before fMRI), behavioral data (percentage of correct responses and response time) were analyzed using a two-way repeated-mesures analysis of variance (ANOVA, Statistica software) with the task (LV, LC) and body position (sitting, supine) as within-subjects factors. In the second part (during the fMRI), a one-way ANOVA was performed on the task (LV, LC).
The alpha risk was fixed at p < 0.05.
Analysis of fMRI Data
All fMRI data were processed and analyzed using the general linear model for event-related designs in SPM8 (Wellcome Department of Imaging Neuroscience, London, UK; http://www.fil.ion.ucl.ac.uk/spm). Functional images were realigned, corrected for slice, normalized to an EPItemplate (re-sampled at a voxel-size of 3 mm), spatially smoothed (8 mm FWHM), and high-pass filtered (cutoff: 180 s). Statistical analyses were performed on a voxelwise basis across the whole-brain, using a mixed blocked and event-related design (15).
Individual visual events were modeled by a standard synthetic haemodynamic response function (HRF). This HRF was estimated at each voxel by a General Linear Model (GLM) using a least-square fit to the data, for each condition, and each individual participant. Statistical maps (SPM[t]) generated from comparisons between conditions in individual subjects were then included in a second-stage random-effect analysis, using one-sample t-tests (16). The resulting maps SPM[t] were thresholded at conventional statistical values (voxel threshold at P < 0.001 and cluster threshold of P < 0.05), using standard parameters similar to previous imaging studies in our group (17). Main comparison was performed between vertical and control tasks. Thus this analyse enabled us to identify the neural networks that are selectively responsible for vertical coding.
Results
Behavioral Data
The first ANOVA carried out on the data obtained before fMRI runs showed no main effect of task on the rate of correct responses (p = 0.72), no main effect of body position (p = 0.82) and no interaction (LV: supine = 95 ± 2%, sitting = 96 ± 3%, LC: supine = 97 ± 2%, sitting = 98 ± 1%,). Similarly, the second ANOVA performed on the response times showed no effect of task (p = 0.77), no effect of body position (p = 0.75) and no interaction (LV: supine = 669 ± 52 ms, sitting = 717 ± 81 ms; LC: supine = 602 ± 79 ms, sitting = 580 ± 54 ms). The body position (supine or sitting) did not differentially affected the performance in the two tasks.
Behavioral data obtained during fMRI scanning did not show any effect of the task (p = 0.79 neither for the rate of correct responses (LV: 96 ± 3%; LC = 98 ± 1%) nor for response times (LV: 698 ± 47 ms; LC: 550 ± 65 ms).
Neuroimagery Data
The data from brain imaging are shown in Figure 1 and listed in Table 1. The brain activations during verticality judgment relative to the control task were localized principally in both temporo-occipital cortices, with a right dominance tendency. We then directly compared the two tasks against each other. The contrast LV > LC showed strong bilateral activations within the superior occipital gyrus, the parietal lobe, the middle and superior temporal gyrus and the supplementary motor areas. Specific activations occurred in the right hemisphere for the inferior parietal lobe, the thalamus and the anterior part of the cerebellum (dentate, nodulus peduncles) and the midbrain. In the left hemisphere, specific activations were located in the parahippocampal gyrus and the brainstem. We then directly compared the two spatial tasks against each other. The contrast LC > LV showed selective activity in the left inferior temporal gyrus (xyz = −60 −16 −26, Z = 3.47, P < 0.001).
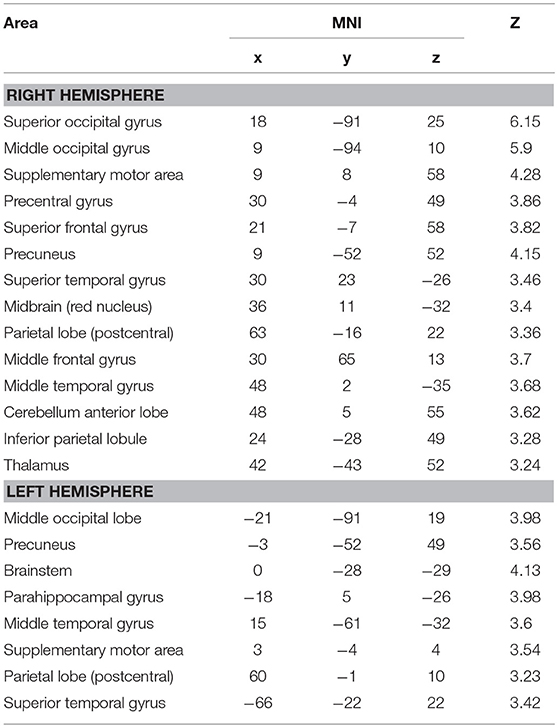
Table 1. Activation peaks (Montreal Neurological Institute coordinates) obtained for Line Vertical judgment > Line Color judgment (P < 0.001 uncorrected; cluster size > 15).
Discussion
This study in healthy participants shows that the neuroanatomical substrates of the judgment of the visual vertical involves a wide cortical network distributed bilaterally. This network includes mainly the occipital cortex, with the cuneus and the lingual gyri, the precuneus, the cerebellum and the brainstem. That these regions played a role in the judgment of verticality is in agreement with the results of previous studies which showed that the lingual gyrus and the cuneus are involved in orientation discrimination tasks (18). Recently, these brain regions have been shown to be involved in the treatment of vestibular information (19). In this fMRI study, the regions specifically activated during galvanic vestibular stimulation were the vestibular cortex, the inferior parietal lobe, the superior temporal gyrus and the cerebellum.
The role of these areas specifically activated during vertical judgment has also been mentioned in studies of vertical perception in stroke patients (9, 10, 20). The posterior temporo-parietal areas closely corresponded to those found by Lopez et al. (13). Their EEG study in normal subjects revealed a bilateral activity in the temporo-occipital and parieto-occipital regions during a vertical estimation task. Moreover, the present data are compatible with monkey studies showing that neural populations in the ventral and dorsal streams respond to orientation discrimination (21, 22). In addition, they are in agreement with the perceptual data reported in brain-damaged patients as deviation of vertical have been reported after a damage of the temporo-parietal junction, including the superior temporal gyrus and inferior parietal lobe (9, 10). Moreover, a recent study in patients suffering from unilateral vestibular neuritis who underwent resting state F-FDG PET showed, in the acute phase, a deviation of the vertical that was associated with a metabolic response in main cortical vestibular areas similar to those we evidenced here (23). In this study, the authors also found a metabolic response in the cerebellum for patients with left neuritis. In support of these data, a lesion of the inferior peduncle has been shown to bias the subjective visual vertical (24). In our study, the cerebellar activation appeared restricted to its anterior part. Though no strong activation of the vermis was expected in healthy participants lying on their back, our data are compatible with an involvement of the caudal and rostral parts of the vermis, where the lower part of the body is represented. This would suggest that, even when lying, the body remains a reference for verticality judgments. However, the activation of this anterior region and of midbrain could also sign an activity in cognitive/visuospatial loops including the ventral dentate nucleus (25, 26).
It is noteworthy and of the greatest clinical relevance that the brain areas involved in vertical visual perception in the healthy subjects largely overlap those reported in the studies of verticality disorders. More specifically, the current study identified clusters in the cerebellum, the brainstem, right inferior parietal lobe that overlapped with lesioned sites typically associated to pathological tilt of vertical (9, 20, 27, 28). Keeping in mind that the present study is grounded on a paradigm that was firstly shown to yield similar behavioral performance in supine and in sitting positions, we have to consider that perception of verticality is not influenced by the body position. In fact, in supine position, the participants could refer the visual stimulus to a bodily horizontal axis, or project their main body axis in the vertical plane to judge the verticality of the stimulus (29, 30). Finally, one can note that brain and vestibular damaged patients with an altered perception of verticality are usually older than the participants tested here.
To conclude, the present fMRI study indicates that during vertical judgments activation spreads to the temporo-occipital and parieto-occipital cortices, and also to the cerebellum and the brainstem. Recently, these regions have been claimed to be also implicated in the body representation (17), the balance control (31) and the spatial navigation (32). All in all, the data obtained here from healthy participants clarify the neural substrate of these functions that all require a continuously updated representation of the vertical.
Author Contributions
AS: study concept and design, analysis and interpretation of data, and drafting the manuscript; LB: interpretation of data, revision of the manuscript for intellectual content; JH: interpretation of data, revision of the manuscript for intellectual content.
Funding
AS is supported by Geneva Academic Society (Foremane Fund). LB was supported by grants from CNRS, Ministère de l'Enseignement Supérieur et de la Recherche (UMR 7260).
Conflict of Interest Statement
The authors declare that the research was conducted in the absence of any commercial or financial relationships that could be construed as a potential conflict of interest.
References
1. Washburn SL. Behaviour and the origin of man. In: Proceedings of the Royal Anthropological Institute of Great Britain and Ireland (1967). p. 21–7.
2. Lackner JR, DiZio P. Vestibular, proprioceptive, and haptic contributions to spatial orientation. Annu Rev Psychol. (2005) 56:115–47. doi: 10.1146/annurev.psych.55.090902.142023
3. Borel L, Lopez C, Péruch P, Lacour M. Vestibular syndrome: a change in internal spatial representation. Neurophysiol Clin. (2008) 38:375–89. doi: 10.1016/j.neucli.2008.09.002
4. Lopez C. A neuroscientific account of how vestibular disorders impair bodily self-consciousness. Front Integr Neurosci. (2013) 7:91. doi: 10.3389/fnint.2013.00091
5. Friedmann G. The judgement of the visual vertical and horizontal with peripheral and central vestibular lesions. Brain (1970) 93:313–28. doi: 10.1093/brain/93.2.313
6. Lopez C, Lacour M, El Ahmadi AS, Magnan J, Borel L. Changes of visual vertical perception: a long-term sign of unilateral and bilateral vestibular loss. Neuropsychologia (2007) 45:2025–37. doi: 10.1016/j.neuropsychologia.2007.02.004
7. Müller JA, Bockisch CJ, Tarnutzer AA. Spatial orientation in patients with chronic unilateral vestibular hypofunction is ipsilesionally distorted. Clin. Neurophysiol. (2016) 127:3243–51. doi: 10.1016/j.clinph.2016.07.010
8. Baier B, Suchan J, Karnath HO, Dieterich M. Neural correlates of disturbed perception of verticality. Neurology (2012) 78:728–35. doi: 10.1212/WNL.0b013e318248e544
9. Rousseaux M, Honoré J, Vuilleumier P, Saj A. Neuroanatomy of space, body, and posture perception in patients with right hemisphere stroke. Neurology (2013) 49:2607–15. doi: 10.1212/WNL.0b013e3182a823a7
10. Rousseaux M, Braem B, Honoré J, Saj A. An anatomical and psychophysical comparison of subjective verticals in patients with right brain damage. Cortex (2015) 69:60–7. doi: 10.1016/j.cortex.2015.04.004
11. Brandt T, Dieterich M. Vestibular syndromes in the roll plane: topographic diagnosis from brainstem to cortex. Ann Neurol. (1994) 36:337–47. doi: 10.1002/ana.410360304
12. Maffei V, Mazzarella E, Piras F, Spalletta G, Caltagirone C, Lacquaniti F, Daprati E. Processing of visual gravitational motion in the peri-sylvian cortex: evidence from brain-damaged patients. Cortex (2016) 78:55–69. doi: 10.1016/j.cortex.2016.02.004
13. Lopez C, Mercier MR, Halje P, Blanke O. Spatiotemporal dynamics of visual vertical judgments: early and late brain mechanisms as revealed by high-density electrical neuroimaging. Neuroscience (2011) 181:134–49. doi: 10.1016/j.neuroscience.2011.02.009
14. Bonan I, Derighetti F, Gellez-Leman MC, Bradaï N, Yelnik A. Visual dependence after recent stroke. Ann Readapt Med Phys. (2006) 49:166–71. doi: 10.1016/j.annrmp.2006.01.010
15. Mechelli A, Henson RN, Price CJ, Friston KJ. Comparing event-related and epoch analysis in blocked design fMRI. Neuroimage (2003) 18:806–10. doi: 10.1016/S1053-8119(02)00027-7
16. Friston KJ, Fletcher P, Josephs O, Holmes A, Rugg MD, Turner R. Event-related fMRI: characterizing differential responses. Neuroimage (1998) 7:30–40. doi: 10.1006/nimg.1997.0306
17. Saj A, Cojan Y, Musel B, Honoré J, Borel L, Vuilleumier P. Functional neuro-anatomy of egocentric versus allocentric space representation. Neurophysiol Clin. (2014) 44:33–40. doi: 10.1016/j.neucli.2013.10.135
18. Vandenberghe R, Dupont P, De Bruyn B, Bormans G, Michiels J, Mortelmans L, Orban GA. The influence of stimulus location on the brain activation pattern in detection and orientation discrimination. A PET study of visual attention. Brain (1996) 119(Pt 4):1263–76.
19. Della-Justina HM, Gamba HR, Lukasova K, Nucci-da-Silva MP, Winkler AM, Amaro E, et al. Interaction of brain areas of visual and vestibular simultaneous activity with fMRI. Exp Brain Res. (2015) 233:237–52. doi: 10.1007/s00221-014-4107-6
20. Choi K-D, Lee H, Kim J-S. Vertigo in brainstem and cerebellar strokes. Curr Opin. (2013) 26:90–5. doi: 10.1097/WCO.0b013e32835c5edd
21. Faillenot I, Decety J, Jeannerod M. Human brain activity related to the perception of spatial features of objects. Neuroimage (1999) 10:114–24. doi: 10.1006/nimg.1999.0449
22. Becker-Bense S, Buchholz HG, zu Eulenburg P, Best C, Bartenstein P, Schreckenberger M, Dieterich M. Ventral and dorsal streams processing visual motion perception (FDG-PET study). BMC Neurosci. (2012) 13:81. doi: 10.1186/1471-2202-13-81
23. Becker-Bense S, Dieterich M, Buchholz HG, Bartenstein P, Schreckenberger M, Brandt T. The differential effects of acute right- vs. left-sided vestibular failure on brain metabolism. Brain Struct Funct. (2014) 219:1355–67. doi: 10.1007/s00429-013-0573-z
24. Choi JH, Seo JD, Choi YR, Kim MJ, Kim HJ, Kim JS, et al. Inferior cerebellar peduncular lesion causes a distinct vestibular syndrome. Eur J Neurol. (2015) 22:1062–7. doi: 10.1111/ene.12705
25. Kirsch V, Keeser D, Hergenroeder T, Erat O, Ertl-Wagner B, Brandt T, et al. Structural and functional connectivity mapping of the vestibular circuitry from human brainstem to cortex. Brain Struct Funct. (2016) 221:1291–308. doi: 10.1007/s00429-014-0971-x
26. Bostan AC, Dum RP, Strick PL. Cerebellar networks with the cerebral cortex and basal ganglia. TICS (2013) 17:241–54. doi: 10.1016/j.tics.2013.03.003
27. Yang TH, Oh SY, Kwak K, Lee JM, Shin BS, Jeong SK. Topology of brainstem lesions associated with subjective visual vertical tilt. Neurology (2014) 82:1968–75. doi: 10.1212/WNL.0000000000000480
28. Baier B, Bense S, Dieterich M. Are signs of ocular tilt reaction in patients with cerebellar lesions mediated by the dentate nucleus? Brain (2008) 131:1445–54. doi: 10.1093/brain/awn086
29. Luyat M, Ohlmann T, Barraud PA. Subjective vertical and postural activity. Acta Psychol. (1997) 95:181–93. doi: 10.1016/S0001-6918(96)00015-7
30. Lopez C, Lacour M, Léonard J, Magnan J, Borel L. How body position changes visual vertical perception after unilateral vestibular loss. Neuropsychologia (2008) 46:2435–40. doi: 10.1016/j.neuropsychologia.2008.03.017
31. Karim HT, Sparto PJ, Aizenstein HJ, Furman JM, Huppert TJ, Erickson KI, et al. Functional MR imaging of a simulated balance task. Brain Res. (2014) 1555:20–7. doi: 10.1016/j.brainres.2014.01.033
Keywords: vertical perception, posture, fMRI, visual orientation, vestibular system
Citation: Saj A, Borel L and Honoré J (2019) Functional Neuroanatomy of Vertical Visual Perception in Humans. Front. Neurol. 10:142. doi: 10.3389/fneur.2019.00142
Received: 28 August 2018; Accepted: 04 February 2019;
Published: 25 February 2019.
Edited by:
Christian Van Nechel, Clinique des Vertiges, BelgiumReviewed by:
Alexander A. Tarnutzer, University of Zurich, SwitzerlandAndrés Soto-Varela, Complejo Hospitalario Universitario de Santiago, Spain
Copyright © 2019 Saj, Borel and Honoré. This is an open-access article distributed under the terms of the Creative Commons Attribution License (CC BY). The use, distribution or reproduction in other forums is permitted, provided the original author(s) and the copyright owner(s) are credited and that the original publication in this journal is cited, in accordance with accepted academic practice. No use, distribution or reproduction is permitted which does not comply with these terms.
*Correspondence: Arnaud Saj, arnaud.saj@umontreal.ca