- 1Department of Neurology, Leiden University Medical Center, Leiden, Netherlands
- 2Department of Human Movement Sciences, Faculty of Behavioural and Movement Sciences, Vrije Universiteit Amsterdam, Amsterdam Movement Sciences, Amsterdam, Netherlands
Introduction: In people with Parkinson's disease (PD) many aspects of walking ability deteriorate with advancing disease. Clinical tests typically evaluate single aspects of walking and to a lesser extent assess more complex walking tasks involving a combination of the three key aspects of walking ability (i.e., generating stepping, maintaining postural equilibrium, adapting walking). The Interactive Walkway allows for assessing more complex walking tasks to address features that are relevant for daily life walking of patients, including adaptive walking and dual-task walking.
Methods: To evaluate the expected added value of Interactive Walkway assessments in people with PD, we first evaluated its known-groups validity for outcome measures of unconstrained walking, adaptive walking and dual-task walking. Subsequently, these outcome measures were related to commonly used clinical test scores. Finally, we evaluated the expected added value of these outcomes over clinical tests scores in discriminating people with PD with and without freezing of gait.
Results: Interactive Walkway outcome measures showed significant differences between freezers, non-freezers and healthy controls, in expected directions. Most Interactive Walkway outcome measures were not or at best moderately correlated with clinical test scores. Finally, Interactive Walkway outcome measures of adaptive walking slightly better discriminated freezers from non-freezers than clinical tests scores.
Conclusion: We confirmed the added value of Interactive Walkway assessments, which provides a comprehensive evaluation of walking ability incorporating features of its three key aspects. Future studies are warranted to examine the potential of the Interactive Walkway for the assessment of fall risk and informing on tailored falls prevention programs in people with PD and in other populations with impaired walking ability.
Introduction
Walking ability is a multifaceted construct which includes the ability to generate stepping, to maintain postural equilibrium, and to adjust walking to meet behavioral goals and environmental demands (1). In Parkinson's disease (PD) these walking ability aspects all deteriorate to some extent with advancing disease. This is evidenced by an inability to generate effective stepping (e.g., freezing of gait [FOG]), a reduced ability to adapt walking to environmental circumstances, and a limited ability to combine walking with secondary tasks (2–5). Such impairments in walking ability may contribute to an increased fall risk. This is clearly demonstrated in PD, where most falls are due to FOG, impaired adaptive walking resulting in trips, and limitations in dual-task walking (6, 7). Clinical tests to evaluate gait and balance disturbances in PD typically evaluate single aspects of walking ability (i.e., the ability to generate stepping or to maintain postural equilibrium) and to a lesser extent assess more complex walking tasks (i.e., adaptive walking and dual-task walking) involving a combination of the three key aspects of walking (stepping, equilibrium and adaptation). The Interactive Walkway (IWW; Figure 1) allows for assessing more complex walking tasks to address features that are relevant for daily life walking of patients, which could guide the management of clinical care.
This study aimed to evaluate the expected added value of IWW assessments in people with PD, which includes an assessment of more complex walking tasks. The IWW utilizes multiple external sensors for a validated quick markerless 3D full-body motion registration of unconstrained walking (8). Moreover, the IWW can be used to assess adaptive walking by augmenting the walkway with visual context, such as suddenly appearing obstacles (9), whose location and timing can be controlled based on real-time processed full-body kinematics. Finally, the IWW may be used to assess the ability to combine walking tasks with a secondary task by quantifying dual-task costs of walking and adaptive walking (10). In this study, we first examined the known-groups validity of IWW outcome measures of unconstrained walking, adaptive walking, and dual-task walking to detect differences between people with PD with FOG, people with PD without FOG and healthy controls. Secondly, we compared IWW outcome measures to commonly used clinical tests of gait and balance impairment to identify redundancy and complementarity among and between tests. Thirdly, we examined the expected added value of the IWW over clinical tests in discriminating people with PD with and without FOG.
Methods
Subjects
Walking ability was assessed in 30 people with PD and 30 age- and sex-matched healthy controls (Table 1). People with PD and controls were recruited from the outpatient clinic of the Leiden University Medical Center and via advertisement, respectively. People with PD had to meet the UK Parkinson's Disease Society Brain Bank clinical diagnostic criteria (11) and have a Hoehn and Yahr stage of 1–4 (12). In addition, subjects had to be 18 years or older, have command of the Dutch language, be able to stand unsupported for more than 20 s and walk independently. People with PD were evaluated using the Movement Disorder Society version of the Unified Parkinson's Disease Rating Scale motor score (13). The New Freezing of Gait Questionnaire (14) was used to classify people with PD with and without FOG (i.e., based on a score greater than or equal to zero, respectively), leading to the classification of 14 freezers and 16 non-freezers. The Scales for Outcomes in Parkinson's disease—Cognition (15) was administered to assess cognitive abilities, since this scale is sensitive to PD-specific cognitive deficits. People with PD were measured in the ON state. Controls did not suffer from neurological or orthopedic diseases interfering with gait, had normal cognitive function [Montreal Cognitive Assessment score ≥ 23; (16)] and (corrected to) normal vision. All subjects gave written informed consent, and the study was approved by the local medical ethics committee (P15.232).
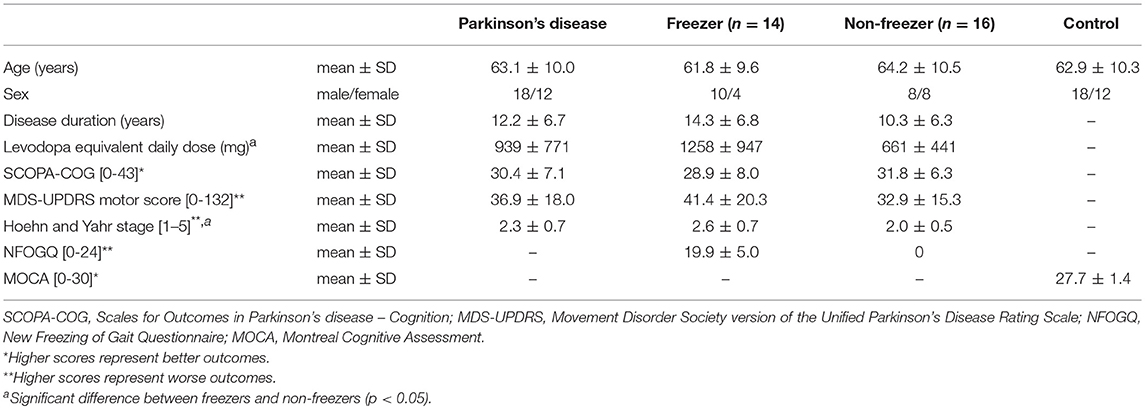
Table 1. Group characteristics of people with Parkinson's disease (all, freezers and non-freezers) and healthy controls.
Experimental Set-Up and Procedure
We used clinical tests of gait and balance impairment that have previously been suggested or recommended for use in people with PD (17). Two tests assessed mobility: the Timed-Up-and-Go test and the 10-m walking test at comfortable and maximum walking speed. Longer completion times indicate poorer mobility. The Tinetti Balance Assessment has two sections that evaluate gait and balance performance of which the combined score was used in this study (higher scores indicate a better performance). Two other balance tests were administered: the 7-item Berg Balance Scale, to measure static and dynamic balance, and the Functional Reach Test, to determine the maximal reaching distance (higher scores indicating a better balance). The order of these clinical tests was randomized.
The IWW was used to assess unconstrained walking, adaptive walking and dual-task walking (cf. Figure 2; see Supplementary Video and Table 2 for more details). Full-body kinematics was obtained using four spatially and temporally integrated Kinect v2 sensors, which allows for a quick markerless assessment of walking. The sensor set-up was based on a validated IWW set-up (8, 9), with improved inter-sensor distances following recommendations of Geerse et al. (18) (Figure 1). The sensors were positioned at a height of 0.95 m alongside a walkway of 8 by 0.75 m. The first three sensors were placed frontoparallel (i.e., with an angle of 70° relative to the walkway direction) with a distance of 1.2 m from the left border of the walkway. The last sensor was positioned frontally at the end of the walkway, since this will minimize orientation-based biases. The first sensor was positioned at 3 m from the start and the other sensors were placed at inter-sensor distances of 2.1 m (Figure 1). The IWW was equipped with a projector (EPSON EB-585W, ultra-short-throw 3LCD projector) to augment the entire walkway with visual context. The coordinate systems of the sensors and the projector were spatially aligned using a spatial calibration grid. IWW data were sampled at 30 Hz using custom-written software utilizing the Kinect-for-Windows Software Development Kit (SDK 2.0). Unconstrained walking was assessed with an 8-m walking test. Adaptive walking was assessed with obstacle avoidance, sudden stops-and-starts, goal-directed stepping (symmetric and irregular stepping stones), narrow walkway (entire walkway and sudden narrowing), speed adjustments (speeding up and slowing down), slalom and turning (half and full turns). Dual-task walking was assessed in plain and augmented walking environments by adding an auditory Stroop task in which the words high and low were pronounced at a high or low pitch (i.e., congruent and incongruent stimuli) to the 8-m walking test and obstacle-avoidance task, respectively. Subjects had to respond with the pitch of the spoken word. The IWW assessment contained 36 trials (Table 2). Subjects were instructed to complete each trial at a self-selected walking speed, while also responding to the Stroop stimuli in case of dual-task walking. Figure 2 presents a schematic representation of the IWW assessment.
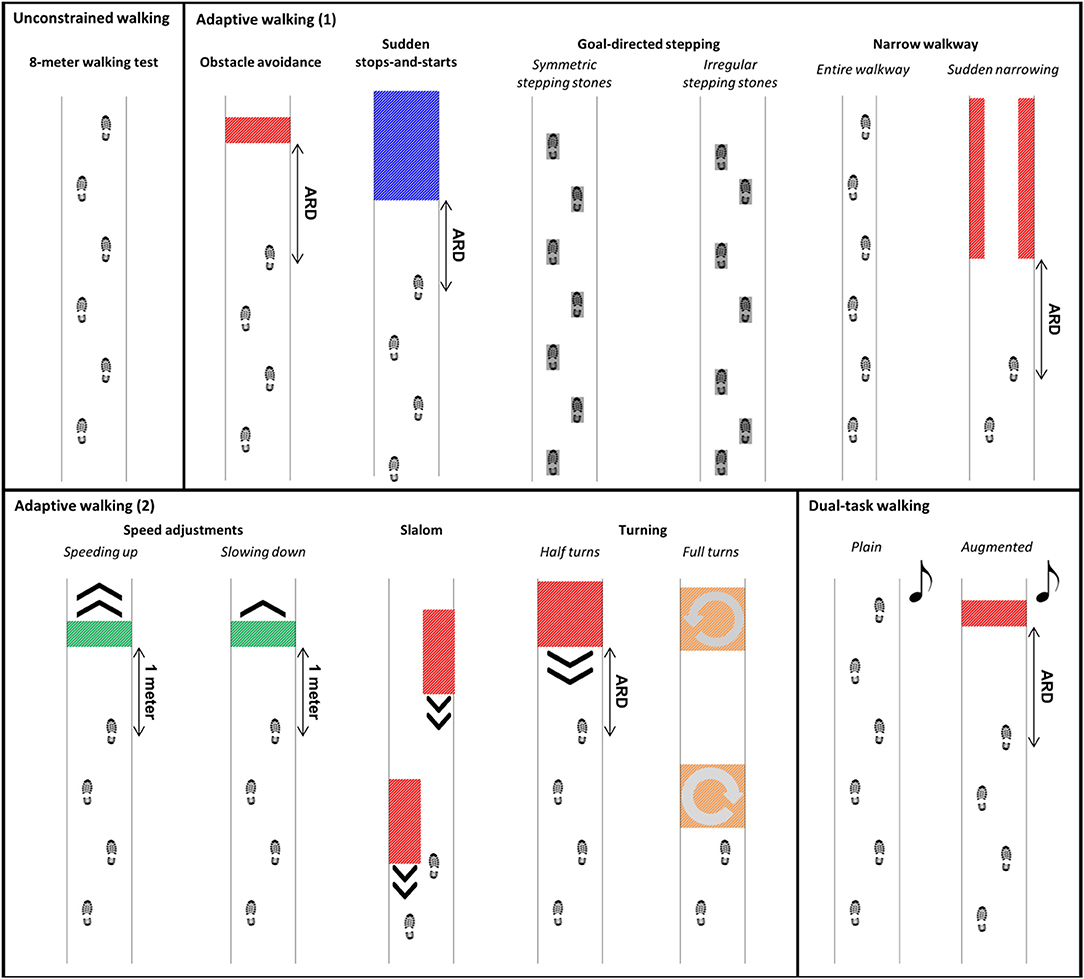
Figure 2. Schematic representation of the Interactive Walkway assessment, including unconstrained walking, adaptive walking, and dual-task walking. The available response distance (ARD) of the suddenly appearing obstacles and cues was patient-tailored to yield a similar response time.
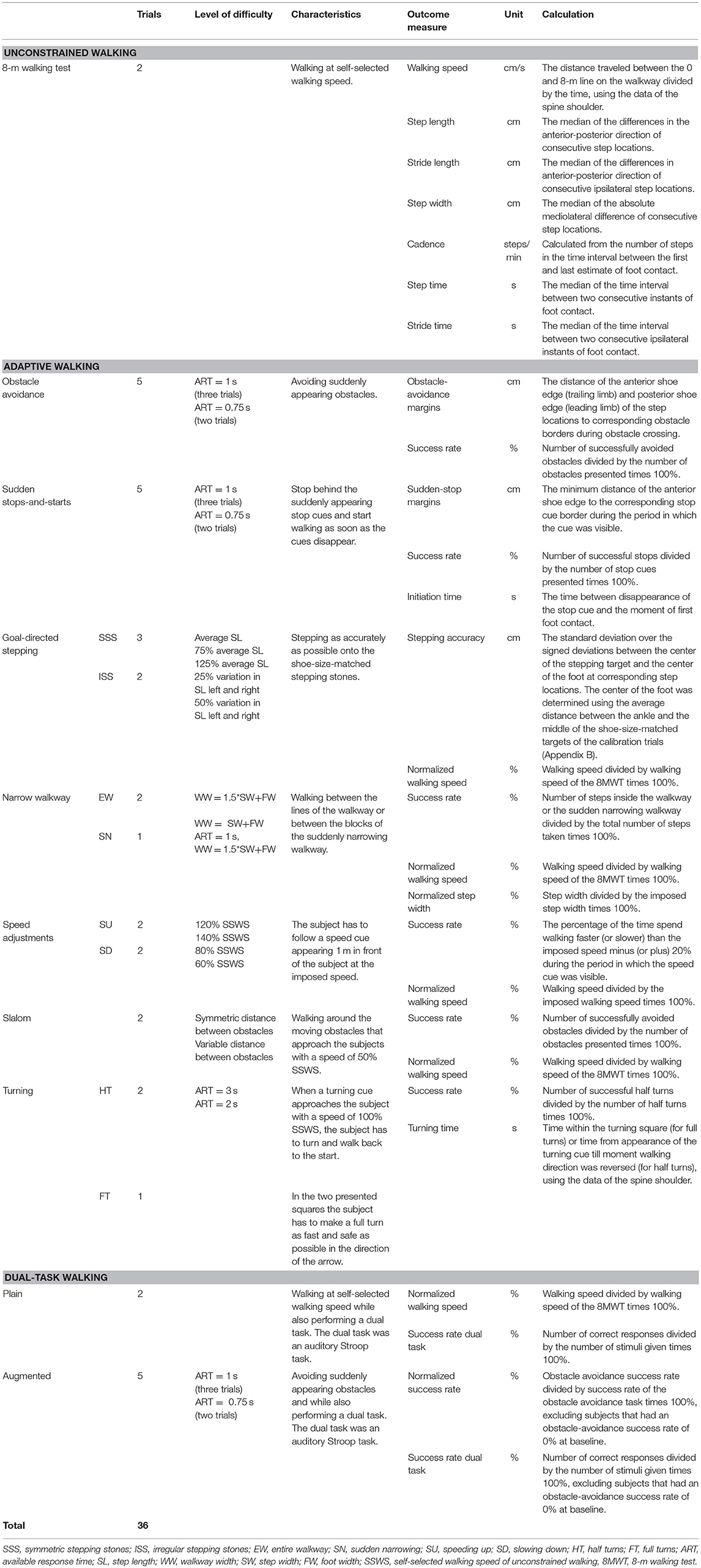
Table 2. Interactive Walkway tasks and outcome measures of unconstrained walking, adaptive walking, and dual-task walking.
Half of the subjects started with the block of clinical tests, the other half with the IWW assessment. With regard to the latter, subjects always started with the 8-m walking test, allowing us to adjust the settings of the adaptive walking tasks to one's own gait characteristics in an attempt to obtain a similar level of difficulty for each subject (see Table 2). For example, available response times for suddenly appearing obstacles were controlled by self-selected walking speed during the 8-m walking test and available response distance (ARD in Figure 2). Subsequently, plain dual-task walking was performed, preceded by a familiarization trial in which the dual task was practiced while sitting. The remaining IWW tasks were randomized in blocks (Table 2).
Data Pre-processing and Analysis
Data pre-processing followed Geerse et al. (8, 9), as detailed in the Supplementary Material. In total, 12 trials (1.1% of all trials) were excluded since subjects were not able to perform the tasks or trials were not recorded properly (i.e., incorrect recording or not all sensors were able to track the subject). These trials only concerned people with PD. The IWW outcome measures of unconstrained walking, adaptive walking and dual-task walking were calculated from specific body points' time series, estimates of foot contact and foot off, and step locations, as detailed in Table 2 and the Supplementary Material. The average over trials per IWW task per subject was calculated for all outcome measures (Table 2).
Statistical Analysis
IBM SPSS Statistics for Windows, version 24 (IBM Corp., Armonk, N.Y., USA) was used to perform the statistical analyses. With regard to the known-groups validity we examined the effect of group (i.e., freezer, non-freezer or control) on clinical test scores and IWW outcome measures of unconstrained walking, adaptive walking and dual-task walking using one-way ANOVAs or the Kruskal-Wallis test if the assumption of normality was violated (i.e., significant Shapiro-Wilk test). For one-way ANOVAs, the assumption of homogeneity of variance was checked using the Levene's test. If significant, the Welch test was used and main effects were examined using Games-Howell post-hoc tests. Otherwise, main effects were examined with Least Significant Difference post-hoc tests. For the Kruskal–Wallis test, main effects were examined using multiple Mann–Whitney tests. Effect sizes were quantified with omega squared (ω2) for one-way ANOVAs and eta squared (η2) for Kurskal–Wallis tests. There was no correction for multiple comparisons due to the explorative character of the study and given the dependency between the outcome measures.
Pearson's correlation coefficients were determined between clinical test scores and IWW outcome measures for people with PD only. Absolute correlations between 0-0.499, 0.500-0.699, 0.700-0.899, and 0.900-1.000 were regarded as low, moderate, high and very high correlations, respectively (19).
Stepwise discriminant analyses were conducted to determine the added value of IWW outcome measures over clinical test scores in discriminating freezers from non-freezers, using Wilks' lambda method (entry = 3.84 and removal = 2.71) in four different models. Predictor variables were clinical test scores (model 1), IWW gait characteristics of unconstrained walking (model 2), IWW outcome measures of adaptive walking (model 3) and IWW outcome measures of dual-task walking (model 4; Table 2). Subjects were only included if they had values for all possible predictor variables. Three not highly correlated predictor variables with the highest effect sizes for the comparison between freezers and non-freezers were selected per model. All models were cross-validated using the leave-one-out method [i.e., each subject is classified by a discriminant function which is based on all subjects except itself; (20)]. The accuracy (i.e., proportion of correctly classified freezers and non-freezers) of discriminant models and cross-validated discriminant models was determined. Furthermore, exact McNemar's tests were performed to establish if one model significantly outperformed the others.
Results
Known-Groups Validity
As expected, freezers performed significantly worst, non-freezers performed in-between, and matched controls performed best on almost all assessments (i.e., clinical tests, unconstrained walking and adaptive walking; Table 3). There was one exception; freezers had significantly better stepping accuracies than non-freezers on the goal-directed stepping task with symmetric stepping stones. No significant group differences were found for IWW outcome measures of dual-task walking.
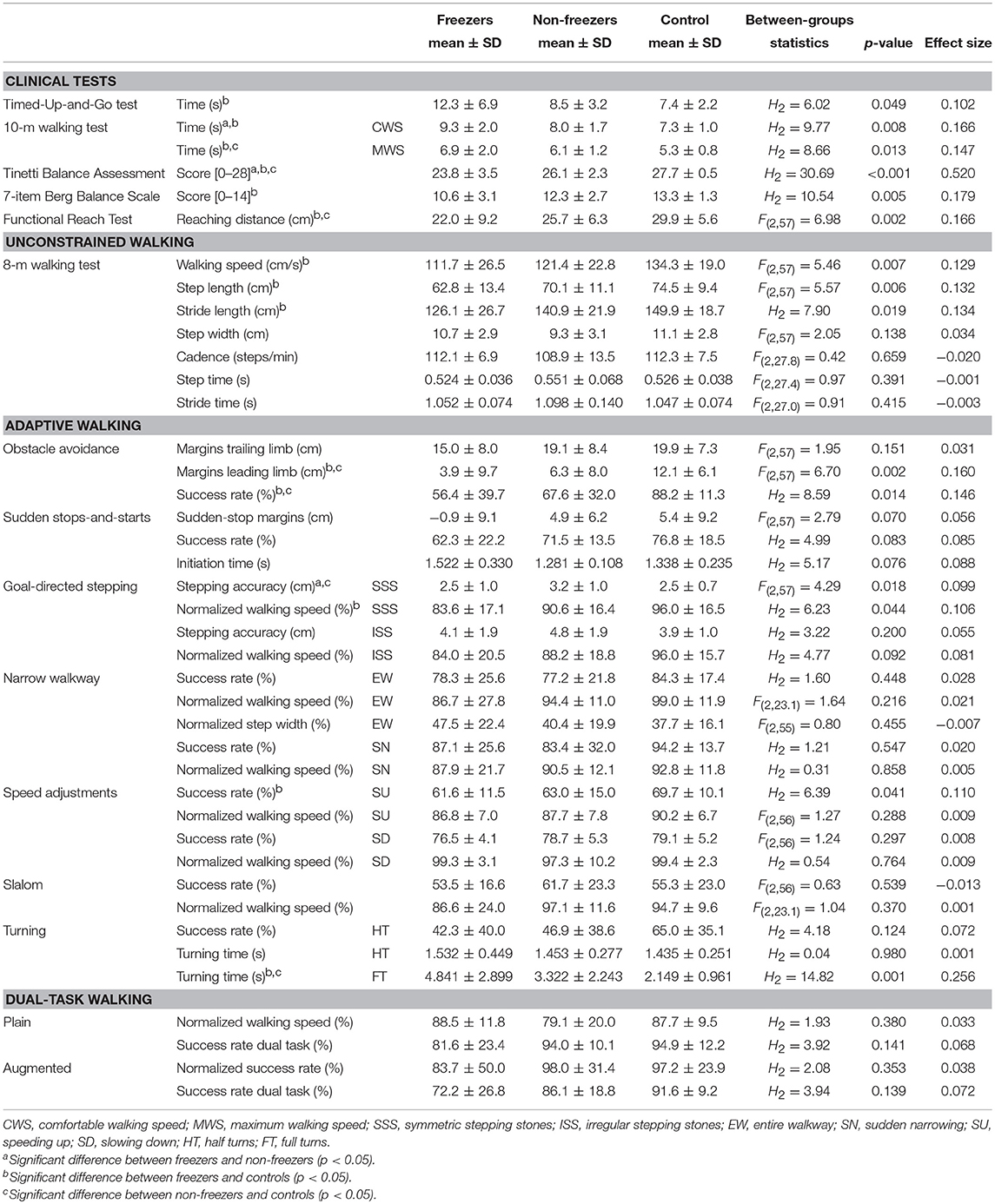
Table 3. Means, standard deviations and between-groups statistics of clinical test scores and Interactive Walkway outcome measures of unconstrained walking, adaptive walking, and dual-task walking for freezers, non-freezers and controls.
Correlations Between Outcome Measures
Of the 42 possible correlations between clinical test scores and IWW gait characteristics, 18 (42.9%) were significant, out of which 17 (40.5%) were high and 1 (2.4%) was moderate (Figure 3). Significant correlations were only found for walking speed, step length and stride length. For IWW outcome measures of adaptive walking, 88 (61.1%) of the possible 144 correlations were significant. Nevertheless, only 9 (6.3%) were high, while 45 (31.3%) were moderate and 34 (23.6%) were low (Figure 3). High correlations were mainly found for turning time of full turns. For IWW outcome measures of dual-task walking, 11 (45.8%) out of the possible 24 correlations were significant, out of which 1 (4.2%) was high, 7 (29.2%) were moderate and 3 (12.5%) were low (Figure 3).
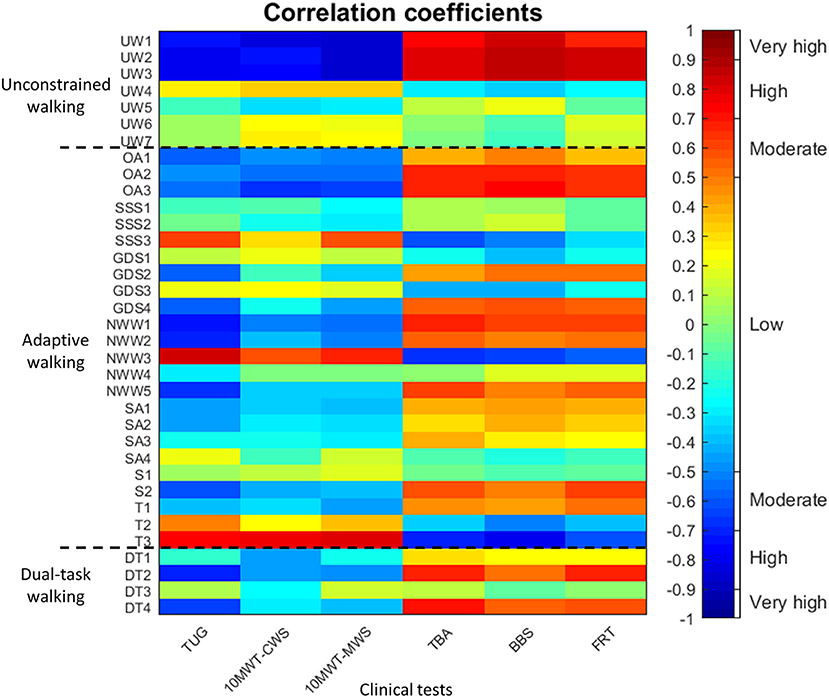
Figure 3. Pearson's correlation coefficients between clinical test scores (x-axis; i.e., Timed Up-and-Go test [TUG], 10-m walking test at comfortable and maximum walking speed [10MWT-CWS, 10MWT-MWS], Tinetti Balance Assessment [TBA], 7-item Berg Balance Scale [BBS] and Functional Reach Test [FRT]) and Interactive Walkway outcome measures (y-axis; i.e., gait characteristics of unconstrained walking [UW1-7], outcome measure of adaptive walking [OA1-3, SSS1-3, GDS1-4, NWW1-3, SA1-4, S1-2, T1-3], and outcome measures of dual-task walking [DT1-4]) in people with Parkinson's disease. The order of the outcome measures on the axes is in agreement with Table 3. The dotted black lines separate the three types of Interactive Walkway tasks (i.e., unconstrained walking, adaptive walking, and dual-task walking). The colorbar provides a visualization of the strength and direction of the correlation.
Discriminant Analyses of Freezers and Non-freezers
For model 1 (clinical tests), group membership (i.e., freezer or non-freezer) was predicted using only the 10-m walking test at comfortable walking speed (p = 0.025, Wilks' lambda = 0.791, Canonical correlation = 0.457), the sole predictor variable contributing significantly to the model. Five of ten freezers (50.0%) and 13 of 14 non-freezers (92.9%) were correctly classified. The accuracy of model 1 and its cross validation were both 75.0%. For model 2 (IWW gait characteristics), none of the predictor variables contributed significantly to the model. For model 3 (IWW outcome measures of adaptive walking), group membership was predicted using stepping accuracy on symmetric stepping stones of the goal-directed stepping task and turning time of full turns (p = 0.005, Wilks' lambda = 0.598, Canonical correlation = 0.634) such that 7 of 10 freezers (70.0%) and 12 of 14 non-freezers (85.7%) were correctly classified, with an accuracy of 79.2%. The accuracy of the cross-validated model was 70.8%. For model 4 (IWW outcome measures of dual-task walking), none of the predictor variables contributed significantly to the model. The results of an exact McNemar's test demonstrated that there was no statistical significant difference in the proportion of freezers and non-freezers identified with models 1 and 3 (p = 0.688).
Discussion
This study aimed to examine the expected added value of IWW assessments in people with PD, focusing on known-groups validity, relations with clinical test scores and discriminating freezers from non-freezers.
On all clinical tests, freezers scored worst, non-freezers scored in-between and controls scored best (Table 3). These known-groups differences were also found for IWW gait characteristics (Table 3); freezers had significantly lower walking speeds and smaller step and stride lengths than controls, which is in agreement with findings of others using marker-based motion registration systems or the Kinect v2 sensor (21, 22). Significant group differences in expected directions were also observed for IWW outcome measures of adaptive walking (Table 3). As in Caetano et al. (3), both freezers and non-freezers had more difficulty adapting walking to suddenly appearing obstacles than controls as reflected by lower obstacle-avoidance success rates. In line with other studies (23, 24), margins of the leading limb were smaller in freezers and non-freezers, which probably increases their risk of tripping in real life. Furthermore, group differences were found for the goal-directed stepping, speed adjustments and full turns tasks. In general, freezers scored worst, non-freezers in between, and controls best. An interesting exception was stepping accuracy on symmetric stepping stones, where freezers had significantly better stepping accuracies than non-freezers. Irregular stepping stones showed the same trend, although this did not reach significance possibly due to the larger within-groups variations for this task (Table 3). It is well-known that visual cues may lead to considerable improvement in walking of freezers (25). This is likely mediated by a better visual exploration of freezers than non-freezers in terms of gaze fixations to task-relevant information (26), which is known to result in a better stepping performance (27). No significant group differences were found for the sudden stops-and-starts, narrow walkway and slalom tasks. Reasons for the null effect for the narrow walkway tasks could be that step width and tandem gait are typically preserved in people with PD (28), which was corroborated by an absence of between-groups differences in step width in our study. For the other tasks, the cueing effect of the visual context may have confounded potential group differences. Hence, one could consider removing these tasks from adaptive walking assessments in people with PD. For dual-task walking, also no significant group differences were found. An explanation could be that task prioritization varied among subjects, leading to large within-groups variations for the outcome measures of dual-task walking which reduced the likelihood of finding significant between-groups differences. Note that other studies have also demonstrated that there were no differences in dual-task interference for gait characteristics and cognitive tasks between people with PD and controls (29). The added value of dual-task walking in a walking ability assessment in PD is therefore questionable [see also Gaßner et al. (30) and Smulders et al. (10)]. Our study not only confirmed these results, but also showed that quantifiable differences between groups are particularly evident for other aspects of adaptive walking (e.g., obstacle avoidance and goal-directed stepping).
The group differences found for the IWW tasks of unconstrained walking, obstacle avoidance, goal-directed stepping, speed adjustments and full turns imply that these tasks could be used in a comprehensive walking ability assessment with the IWW, incorporating the three key aspects of walking ability. Usually, a combination of the three key walking-ability aspects (i.e., stepping, equilibrium, and adaptation) is needed for a successful task performance. Indeed, for most IWW tasks a combination was required strongly tapping into the aspect of walking adaptability, while adaptation was not or only moderately targeted by commonly-used clinical tests that mainly measure steady-state gait and static balance as evidenced by the low correlations (Figure 3). While high correlations between tests suggest redundancy in information content, low or no correlations suggest that tests contain complementary information. IWW gait characteristics and turning time of full turns correlated highly with clinical tests, addressing mainly aspects of stepping and equilibrium. People with PD seem to experience problems when having to deviate from their normal gait pattern (3), which requires dynamic balance control. Balance problems in people with PD and especially freezers are evident in the current study, demonstrated by large effect sizes for balance tests and full turns. Clinicians mainly focus on gait impairments (31), although dynamic balance control is also of great importance during challenging walking tasks. Therefore, in order to obtain a more comprehensive characterization of a subject's walking ability, both unconstrained and adaptive walking should be assessed, for example with obstacle-avoidance and goal-directed stepping.
This study also aimed to determine the expected added value of the IWW over clinical tests in discriminating freezers from non-freezers. We indeed found that IWW adaptive walking tasks discriminated better than clinical tests, although the added value was somewhat limited and the proportion of freezers and non-freezers identified with model 3 did not differ significantly from model 1. Clinical tests performed slightly worse compared to adaptive walking tasks with regard to the percentage of freezers correctly classified (50.0 vs. 70.0%, respectively). The percentage of non-freezers correctly classified was high for both models (92.9 and 85.7%, respectively). IWW gait characteristics and IWW outcome measures of dual-task walking did not contribute significantly to the discriminant analysis. Although we could discriminate freezers from non-freezers, the freezing phenomenon itself was rarely observed. IWW tasks elicited FOG episodes in only 12 out of 466 (2.6%) trials, concerning five freezers and mostly during tasks that included turning [in agreement with literature; (32)]. Explanations for the limited amount of FOG episodes could be the focused attention due to the specific instructions of the IWW tasks, cueing effects of visual content and the fact that we assessed people with PD during the ON state, while the occurrence of FOG episodes increases during the OFF state.
The latter is also a limitation of this study, since medication may improve gait impairments and could therefore lead to smaller group differences in walking ability. However, we still found significant between-groups differences, which may indicate that the IWW is a sensitive evaluation tool of walking ability. Another limitation is the relatively small sample size of the discriminant analyses (i.e., 10 freezers and 14 non-freezers). We therefore needed to pre-select predictor variables for the models to prevent overfitting, since the smallest group needs to exceed the number of predictor variables. Finally, the significant difference between freezers and non-freezers in disease severity (i.e., Hoehn and Yahr stage; Table 1) might have influenced the results of this study by increasing the group differences of walking ability outcome measures.
In conclusion, the IWW assessment exhibited expected differences between freezers, non-freezers and healthy controls, with most IWW outcome measures reflecting combinations of stepping, equilibrium, and adaptation; key aspects of walking that are addressed separately in most clinical tests. IWW adaptive walking tasks also contributed to a slightly better discrimination of freezers from non-freezers. Hence, it seems fair to conclude that the IWW is of added value in people with PD when assessing walking ability. The IWW tasks of adaptive walking evaluate more complex gait in comparison with clinical tests, which fits an assessment of walking ability in the early stages of PD where ceiling effects can occur. Future studies should examine the responsiveness of the IWW outcome measures on an individual level and in response to levodopa treatment (i.e., by examining differences in walking ability between the ON and OFF state). In addition, since the impairments in walking ability evaluated with the IWW are linked to walking-related falls, future studies are warranted to examine the clinical potential of the IWW for assessing fall risk and informing on tailored falls prevention programs in people with PD or other populations prone to declines in walking ability (e.g., elderly, stroke). Note that the current study is helpful in that regard, by informing on the subtasks and associated outcome measures providing complementary information with a decent between-groups contrast.
Data Availability Statement
The raw data supporting the conclusions of this manuscript will be made available by the authors, without undue reservation, to any qualified researcher.
Ethics Statement
All subjects gave written informed consent, and the study was approved by the local medical ethics committee (P15.232).
Author Contributions
DG conceived and designed the study, acquired the data, analyzed, and interpreted the data and wrote the paper. MR, JM, and JvH conceived and designed the study and wrote the paper. Each of the authors has read and concurs with the content in the final manuscript.
Funding
This work is part of the research program Technology in Motion (TIM [628.004.001]), which is financed by the Netherlands Organization for Scientific Research (NWO). The funder had no role in the study design, data collection and analysis, interpretation of data, decision to publish, or writing of the manuscript.
Conflict of Interest Statement
The authors declare that the research was conducted in the absence of any commercial or financial relationships that could be construed as a potential conflict of interest.
Acknowledgments
We would like to acknowledge Bert Coolen for customizing the IWW software to the specific purpose of this study. We would also like to thank Elma Ouwehand for her help with the measurements. This work is part of the research program Technology in Motion (TIM [628.004.001]), which is financed by the Netherlands Organization for Scientific Research (NWO).
Supplementary Material
The Supplementary Material for this article can be found online at: https://www.frontiersin.org/articles/10.3389/fneur.2018.01096/full#supplementary-material
Supplementary Video. Video of Interactive Walkway tasks of unconstrained walking, adaptive walking and dual-task walking in a person with Parkinson's disease with dyskinesia. The subject had consented to the making of the video for publication purposes.
Supplementary Material. Data pre-processing.
References
1. Balasubramanian CK, Clark DJ, Fox EJ. Walking adaptability after a stroke and its assessment in clinical settings. Stroke Res Treat. (2014) 2014:591013. doi: 10.1155/2014/591013
2. Bloem BR, Valkenburg VV, Slabbekoorn M, van Dijk JG. The multiple tasks test. Strategies in Parkinson's disease. Exp Brain Res. (2001) 137:478–86. doi: 10.1016/S0966-6362(01)00141-2
3. Caetano MJD, Lord SR, Allen NE, Brodie MA, Song J, Paul SS, et al. Stepping reaction time and gait adaptability are significantly impaired in people with Parkinson's disease: implications for fall risk. Parkins Relat Disord. (2018) 47:32–8. doi: 10.1016/j.parkreldis.2017.11.340
4. Giladi N, Horak FB, Hausdorff JM. Classification of gait disturbances: distinguishing between continuous and episodic changes. Mov Disord. (2013) 28:1469–73. doi: 10.1002/mds.25672
5. Stuart S, Lord S, Hill E, Rochester L. Gait in Parkinson's disease: a visuo-cognitive challenge. Neurosci Biobehav Rev. (2016) 62:76–88. doi: 10.1016/j.neubiorev.2016.01.002
6. Ashburn A, Stack E, Ballinger C, Fazakarley L, Fitton C. The circumstances of falls among people with Parkinson's disease and the use of falls diaries to facilitate reporting. Disabil Rehabil. (2008) 30:1205–12. doi: 10.1080/09638280701828930
7. Rudzinska M, Bukowczan S, Stozek J, Zajdel K, Mirek E, Chwała W, et al. Causes and consequences of falls in Parkinson disease patients in a prospective study. Neurol Neurochir Pol. (2013) 47:423–30. doi: 10.5114/ninp.2013.38222
8. Geerse DJ, Coolen BH, Roerdink M. Kinematic validation of a multi-Kinect v2 instrumented 10-meter walkway for quantitative gait assessments. PLoS ONE (2015) 10:e0139913. doi: 10.1371/journal.pone.0139913
9. Geerse DJ, Coolen BH, Roerdink M. Walking-adaptability assessments with the Interactive Walkway: between-systems agreement and sensitivity to task and subject variations. Gait Posture (2017) 54:194–201. doi: 10.1016/j.gaitpost.2017.02.021
10. Smulders K, Esselink RA, Weiss A, Kessels RP, Geurts AC, Bloem BR. Assessment of dual tasking has no clinical value for fall prediction in Parkinson's disease. J Neurol. (2012) 259:1840–7. doi: 10.1007/s00415-012-6419-4
11. Hughes AJ, Daniel SE, Kilford L, Lees AJ. Accuracy of clinical diagnosis of idiopathic Parkinson's disease. a clinico-pathological study of 100 cases. J Neurol Neurosurg Psychiatry (1992) 55:181–4. doi: 10.1136/jnnp.55.3.181
12. Hoehn MM, Yahr MD. Parkinsonism: onset, progression and mortality. Neurology (1967) 17:427–42. doi: 10.1212/WNL.17.5.427
13. Goetz CG, Tilley BC, Shaftman SR, Stebbins GT, Fahn S, Martinez-Martin P, et al. Movement disorder society-sponsored revision of the Unified Parkinson's Disease Rating Scale (MDS-UPDRS): scale presentation and clinimetric testing results. Mov Disord. (2008) 23:2129–170. doi: 10.1002/mds.22340
14. Nieuwboer A, Rochester L, Herman T, Vandenberghe W, Emil GE, Thomaes T, et al. Reliability of the new freezing of gait questionnaire: agreement between patients with Parkinson's disease and their carers. Gait Posture (2009) 30:459–63. doi: 10.1016/j.gaitpost.2009.07.108
15. Marinus J, Visser M, Verwey NA, Verhey FR, Middelkoop HA, Stiggelbout AM, et al. Assessment of cognition in Parkinson's disease. Neurology (2003) 61:1222–8. doi: 10.1212/01.WNL.0000091864.39702.1C
16. Carson N, Leach L, Murphy KJ. A re-examination of Montreal Cognitive Assessment (MoCA) cutoff scores. Int J Geriatr Psychiatry (2018) 33:379–88. doi: 10.1002/gps.4756
17. Bloem BR, Marinus J, Almeida Q, Dibble L, Nieuwboer A, Post B, et al. Measurement instruments to assess posture, gait, and balance in Parkinson's disease: critique and recommendations. Mov Disord. (2016) 31:1342–55. doi: 10.1002/mds.26572
18. Geerse D, Coolen B, Kolijn D, Roerdink M. Validation of foot placement locations from ankle data of a Kinect v2 sensor. Sensors (2017) 17:2301. doi: 10.3390/s17102301
19. Mukaka MM. Statistics corner: a guide to appropriate use of correlation coefficient in medical research. Malawi Med J. (2012) 24:69–71.
20. Kuligowski J, Pérez-Guaita D, Quintás G. Application of discriminant analysis and cross-validation on proteomics data. Methods Mol Biol. (2016) 1362:175–84. doi: 10.1007/978-1-4939-3106-4_11
21. Sofuwa O, Nieuwboer A, Desloovere K, Willems AM, Chavret F, Jonkers I. Quantitative gait analysis in Parkinson's disease: comparison with a healthy control group. Arch Phys Med Rehabil. (2005) 86:1007–13. doi: 10.1016/j.apmr.2004.08.012
22. Eltoukhy M, Kuenze C, Oh J, Jacopetti M, Wooten S, Signorile J. Microsoft Kinect can distinguish differences in over-ground gait between older persons with and without Parkinson's disease. Med Eng Phys. (2017) 44:1–7. doi: 10.1016/j.medengphy.2017.03.007
23. Galna B, Murphy AT, Morris ME. Obstacle crossing in people with Parkinson's disease: foot clearance and spatiotemporal deficits. Hum Mov Sci. (2010) 29:843–52. doi: 10.1016/j.humov.2009.09.006
24. Vitório R, Lirani-Silva E, Baptista AM, Barbieri FA, dos Santos PC, Teixeira-Arroyo C, et al. Disease severity affects obstacle crossing in people with Parkinson's disease. Gait Posture (2014) 40:266–9. doi: 10.1016/j.gaitpost.2014.03.003
25. Lee SJ, Yoo JY, Ryu JS, Park HK, Chung SJ. The effects of visual and auditory cues on freezing of gait in patients with Parkinson disease. Am J Phys Med Rehabil. (2012) 91:2–11. doi: 10.1097/PHM.0b013e31823c7507
26. Hunt D, Stuart S, Nell J, Hausdorff JM, Galna B, Rochester L, et al. Do people with Parkinson's disease look at task relevant stimuli when walking? An exploration of eye movements. Behav Brain Res. (2018) 348:82–9. doi: 10.1016/j.bbr.2018.03.003
27. Young WR, Hollands MA. Can telling older adults where to look reduce falls? Evidence for a causal link between inappropriate visual sampling and suboptimal stepping performance. Exp Brain Res. (2010) 204:103–13. doi: 10.1007/s00221-010-2300-9
28. Bloem BR, Grimbergen YA, Cramer M, Willemsen MD, Zwinderman AH. Prospective assessment of falls in Parkinson's disease. J Neurol. (2001) 248:950–8. doi: 10.1007/s004150170047
29. Rochester L, Galna B, Lord S, Burn D. The nature of dual-task interference during gait in incident Parkinson's disease. Neuroscience (2014) 265:83–94. doi: 10.1016/j.neuroscience.2014.01.041
30. Gaßner H, Marxreiter F, Steib S, Kohl Z, Schlachetzki JCM, Adler W, et al. Gait and cognition in Parkinson's Disease: cognitive impairment is inadequately reflected by gait performance during dual task. Front Neurol. (2017) 8:550. doi: 10.3389/fneur.2017.00550
31. Park JH, Kang YJ, Horak FB. What is wrong with balance in Parkinson's Disease? J Mov Disord. (2015) 8:109–14. doi: 10.14802/jmd.15018
Keywords: Parkinson's disease, walking ability assessment, Interactive Walkway, unconstrained walking, adaptive walking, dual-task walking
Citation: Geerse DJ, Roerdink M, Marinus J and van Hilten JJ (2018) Assessing Walking Adaptability in Parkinson's Disease: “The Interactive Walkway”. Front. Neurol. 9:1096. doi: 10.3389/fneur.2018.01096
Received: 01 August 2018; Accepted: 30 November 2018;
Published: 12 December 2018.
Edited by:
Tim Anderson, University of Otago, New ZealandReviewed by:
Fernanda Irrera, Sapienza University of Rome, ItalyRou-Shayn Chen, Chang Gung Memorial Hospital, Taiwan
Copyright © 2018 Geerse, Roerdink, Marinus and van Hilten. This is an open-access article distributed under the terms of the Creative Commons Attribution License (CC BY). The use, distribution or reproduction in other forums is permitted, provided the original author(s) and the copyright owner(s) are credited and that the original publication in this journal is cited, in accordance with accepted academic practice. No use, distribution or reproduction is permitted which does not comply with these terms.
*Correspondence: Daphne J. Geerse, d.j.geerse@vu.nl