- Department of Neurology, University Hospital Münster, Münster, Germany
Background: Parkinson's disease (PD) is a common neurodegenerative disorder. The contribution of the immune system to its pathogenesis remains incompletely understood.
Methods: In this study, we performed comprehensive immune cell profiling in the cerebrospinal fluid (CSF) and peripheral blood (PB) of PD patients. Ten PD patients were diagnosed according to brain bank criteria and underwent detailed clinical examination, magnetic resonance imaging, PB and CSF immune cell profiling by multiparameter flow cytometry, and cytokine and chemokine measurements by bead-based arrays. Thirteen healthy elderly volunteers served as control population.
Results: The proportions of activated T-lymphocytes and non-classical monocytes in the CSF were increased in patients with PD compared to the control group. In accordance, we found increased levels of the pro-inflammatory cytokines IL-2, IL-6 and TNFα and of the monocyte chemoattractant protein 1 (MCP-1) in the CSF of the included PD patients.
Conclusions: Our data provide novel evidence for a response of the innate and adaptive immune system in the central nervous system of patients with PD.
Introduction
Parkinson's disease (PD) represents the most common neurodegenerative disorder following Alzheimer's disease (1). PD prevalence and incidence increase exponentially with age and peak beyond the age of 80 years (2). Clinically, PD is defined by the presence of classical parkinsonian motor symptoms, accompanied by a variety of non-motor features (3–5).
PD is a slowly progressive neurodegenerative disorder characterized by an early prominent death of dopaminergic neurons in the substantia nigra (6). Affected neurons display cytoplasmic accumulation of proteinaceous aggregates called Lewy bodies, which are mainly composed of α-synuclein and ubiquitin (6). PD results from a complicated interplay of genetic and environmental factors (1). Accumulating research provides evidence for a prominent response of the innate and adaptive immune system in PD (7, 8). In humans, neuropathological studies demonstrated the presence of activated microglia, the resident macrophages of the central nervous system (CNS), in the substantia nigra and other affected brain regions. Additional post-mortem studies found infiltration of the substantia nigra by T lymphocytes, the presence of activated astrocytes, and increased parenchymal cytokine levels (9–13). Functional brain imaging studies detected significant microglial activation in various regions of the CNS (14–16). In particular, PD patients with dementia revealed widespread cortical microglial activation in addition to the subcortical changes (17, 18). Patients with PD have increased cerebrospinal fluid (CSF) levels of inflammatory chemo- and cytokines, including tumor necrosis factor α (TNFα) and interleukin (IL)-6 (12, 19, 20). Genetic studies identified several polymorphisms in genes that are involved in inflammatory processes, such as TNF, IL1B, CD14, and TREM2, as risk factors for PD (21, 22). Moreover, genome wide association studies revealed HLA-DRB5 as another susceptibility locus (23).
CSF was shown to reflect many biochemical and cellular events within the brain parenchyma. It is easily accessible in clinical practice and routinely obtained for a variety of diagnostic purposes. Immunophenotyping of CSF cells may be useful to gain insight into the pathophysiology of CNS disorders, and sharpen diagnostic accuracy or estimation of individual prognosis (24).
In this study, we aimed to determine the immune cell profile in the CSF of patients with PD by using multiparameter flow cytometry. We found an intrathecal increase of non-classical monocyte and activated T-lymphocyte proportions in the PD group compared to healthy elderly controls. Correspondingly, we detected increased concentrations of pro-inflammatory cytokines and the chemokine MCP-1 in the CSF of PD patients. Together, these data provide new lines of evidence for a role of both innate and adaptive immune responses in human PD.
Methods
Protocol Approval, Registration, and Patient Consent
All patients were recruited from the movement disorder unit at the Department of Neurology, University Hospital Münster, Germany. All participants in this study gave written informed consent. The study was approved by the local ethics committee (2014-624-f-S).
Participants and Study Population
We included 10 PD patients that presented to our movement disorder clinic. Patients were diagnosed according to the UK Brain Bank criteria. Exclusion criteria for this study were concomitant autoimmune diseases, anti-inflammatory co-medication (e.g., cytotoxic agents, steroids, non-steroid analgesia), evidence of an acute systemic inflammatory process at the time of CSF withdrawal (elevated erythrocyte sedimentation rate above 25 mm/h, C-reactive protein above 0.5 mg/dL, or leukocytes above 11 × 103/μL), or blood-tinged CSF. Patient characteristics are described in detail in Table 1. The CSF control group consisted of 13 healthy elderly participants with a normal neurologic examination. There was no statistical difference regarding their age. CSF was collected during spinal anesthesia before hip replacement and yielded, in all cases, normal cell counts and protein levels.
Multiparameter Flow Cytometry and CSF Analysis
Multiparameter flow cytometry of immune cells in PB and CSF samples was done as described previously (24, 29). During lumbar puncture CSF was sampled into polypropylene tubes. All CSF samples were processed in < 20 min. Cells were isolated from CSF by centrifugation (15 min, 290 g, 4°C) and subsequent incubation in VersaLyse buffer (Beckman Coulter, Germany). PB samples were collected in EDTA monovettes and cells were isolated by using VersaLyse buffer. For immunostainings, the following fluorochrome-conjugated antibodies were used: CD14-FITC, CD138-PE, HLA-DR-ECD, CD3-PC5.5, CD56-PC7, CD4-APC, CD19-APC-Alexafluor700, CD16-APC-Alexafluor750, CD8-PacificBlue, and CD45-KromeOrange (all from Beckman-Coulter). Data acquisition was performed with a Navios flow cytometer (Beckman-Coulter). Gating strategy for Leukocytes and Monocytes is described and illustrated in Supplementary Figure 1.
Quantification of Cytokines and Chemokines in the Serum and Cerebrospinal Fluid
CSF was sampled, and supernatants were obtained by centrifugation as described above. The CSF supernatants were then stored at −20°C until analysis of cytokines (IL-2, IL-4, IL-5, IL-6, IL-9, IL-10, IL-13, IL-17A, IL-17F, IL-21, IL-22, IFNy, TNFa) and chemokines (CCL11, CCL17, CCL20, CXCL1, CXCL5, CXCL9, CXCL11, IL-8, IP-10, MCP-1, MIP-1a, MIP-1b, RANTES) using a bead-based cytokine array (LEGENDplex; BioLegend) according to the manufacturer's instructions.
Statistics
Statistical analysis was performed using Graphpad Prism 6. All data are reported as mean ± standard deviation, and the pre-chosen significance level for all confirmatory tests was p < 0.05. Flow cytometry data were analyzed by using the Mann-Whitney test, presuming a non-Gaussian distribution.
Results
The Immune Cell Profile of Patients With PD
Multicolor flow cytometry did not detect significant differences in the proportions or absolute numbers of granulocytes, monocytes, and lymphocytes in the CSF of patients with PD compared to healthy elderly controls (Figure 1, and data not shown). Interestingly, subpopulation analysis of innate immune cells revealed an intrathecal shift in cell proportions from classical monocytes (defined as CD14+/CD16−) to non-classical monocytes (CD14+/CD16+; Figure 1). This intrathecal shift was not reflected in the PB (Figure 1). Adaptive immune cell subgroup analysis demonstrated no differences in the levels of B lymphocytes (data not shown), but an increase in the fractions of both total T lymphocytes and activated (defined by HLA-DR expression) T lymphocytes in the CSF (Figure 2). The CD4/CD8 T lymphocyte ratio remained unchanged, but specifically CD8+ T lymphocytes showed a larger fraction of HLA-DR activated cells (Figure 2). Both CD4+ and CD8+ T lymphocyte activation was also increased in the PB (Figure 2). However, we detected no significant alterations in the absolute cell numbers of monocyte or T lymphocyte subsets (data not shown).
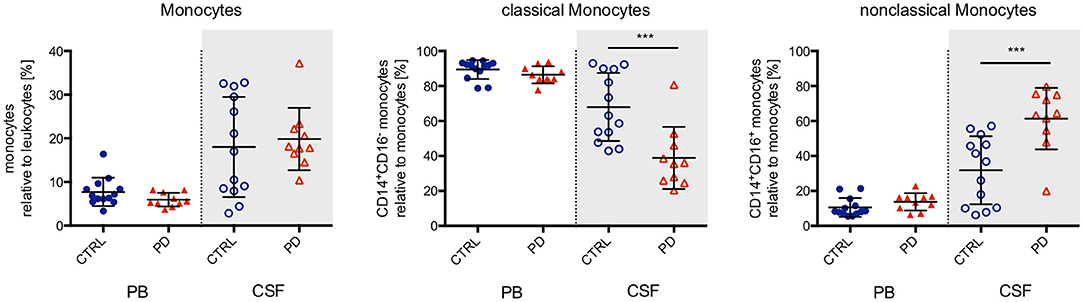
Figure 1. Monocytes in the peripheral blood and cerebrospinal fluid. The proportion of monocytes in relation to total leukocytes was determined in the peripheral blood (PB, closed symbols, white background) and cerebrospinal fluid (CSF, open symbols, gray background) from healthy elderly controls (n = 13; blue circles) and PD patients (n = 10; red triangles). While the proportion of total monocytes did not differ between study groups, monocyte subgroup analysis based on expression of the surface marker CD16 demonstrated a highly significant decrease of classical monocytes (CD14+CD16−) and a concomitant highly significant increase of non-classical monocytes (CD14+CD16+). For statistical analysis, the Mann-Whitney test was performed (***p < 0.001).
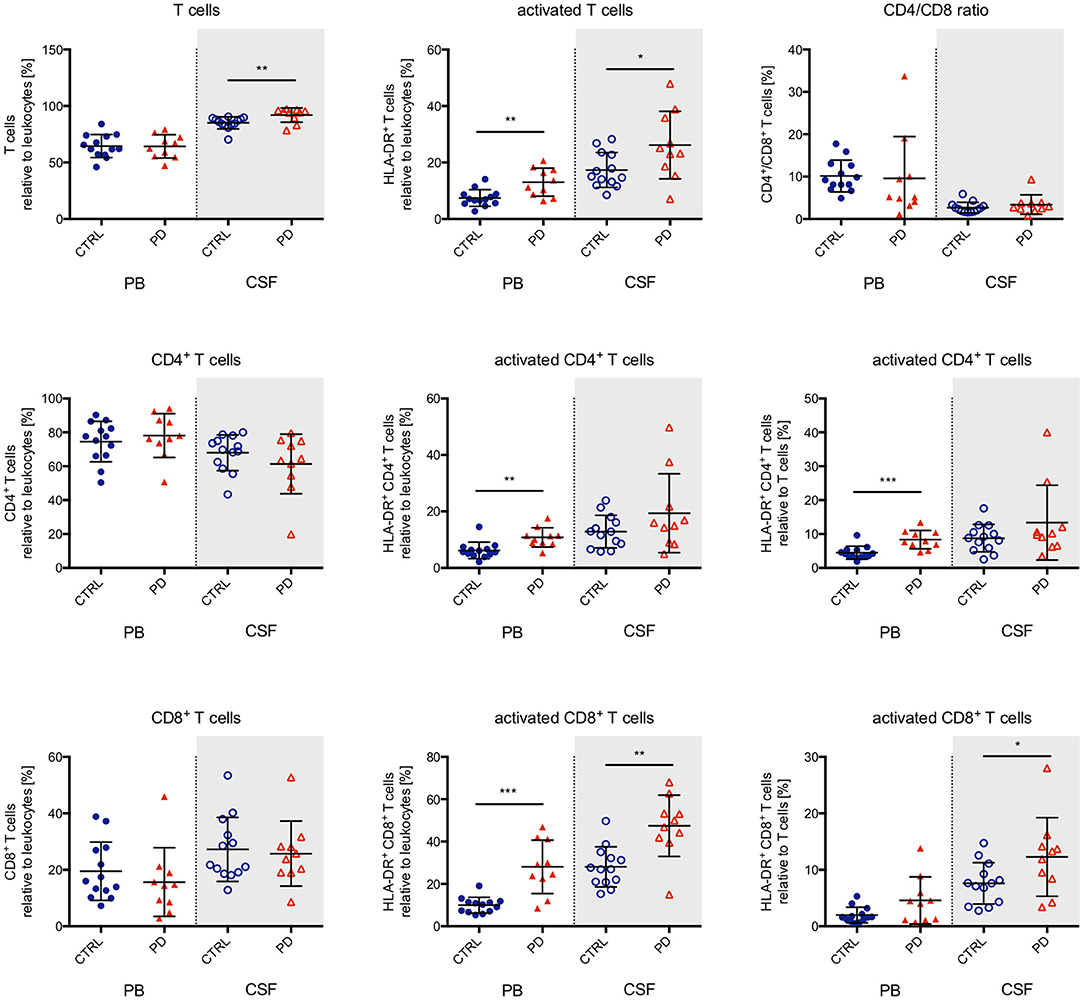
Figure 2. Lymphocytes in the peripheral blood and cerebrospinal fluid. The proportion of T lymphocytes in relation to total leukocytes was determined in the peripheral blood (PB, closed symbols, white background) and cerebrospinal fluid (CSF, open symbols, gray background) from healthy elderly controls (n = 13; blue circles) and PD patients (n = 10; red triangles). We found a significant increase of total and activated T lymphocytes in the CSF. CD4 and CD8 expression did not differ between study groups, but specifically CD8+ T lymphocytes displayed a larger fraction of HLA-DR expressing activated cells, both in the PB and CSF. For statistical analysis, the Mann-Whitney test was performed (*p < 0.05, **p < 0.01, ***p < 0.001).
The Cytokine and Chemokine Profile of Patients With PD
Using a bead-based cytokine array, we detected no significant differences in cytokine (IL-2, IL-4, IL-5, IL-6, IL-9, IL-10, IL-13, IL-17A, IL-17F, IL-21, IL-22, IFNy, TNFa) and chemokine (CCL11, CCL17, CCL20, CXCL1, CXCL5, CXCL9, CXCL11, IL-8, IP-10, MCP-1, MIP-1a, MIP-1b, RANTES) levels in the serum (data not shown) of PD. Interestingly, we found an increase of the pro-inflammatory cytokines IL-2, IL-6, and TNFα as well as of the pro-migratory chemokine MCP-1 (monocyte chemoattractant protein-1) in the CSF of PD patients, whereas anti-inflammatory IL-9 was decreased (Figure 3 A+B, only detected cytokines and chemokines shown).
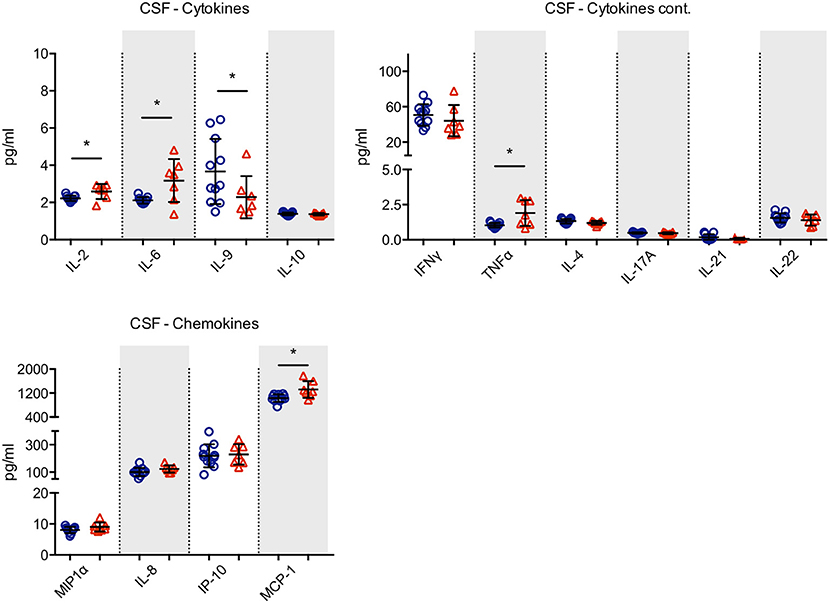
Figure 3. Cytokine and chemokine production in the cerebrospinal fluid. The concentrations of cytokines and chemokines were determined in the cerebrospinal fluid from healthy elderly controls (n = 11; blue circles) and PD patients (n = 7; red triangles) by a bead-based cytokine array. We found a significant increase of the pro-inflammatory cytokines IL-2, IL-6, and TNFα as well as of the pro-migratory chemokine MCP-1 in the CSF of PD patients, whereas anti-inflammatory IL-9 was decreased. For statistical analysis, the Mann-Whitney test was performed (*p < 0.05).
Discussion
In summary, phenotyping of CSF immune cells by multiparameter flow cytometry in patients with PD revealed a strong phenotypical shift of intrathecal monocytes and an increased percentage of activated T lymphocytes. In accordance, the levels of pro-inflammatory cytokines and MCP-1 were higher in the CSF of PD patients.
Evidence for a response of the innate immune system in the disease course of PD has been reported previously by neuropathological and functional brain imaging studies, genetics and CSF cytokine profiling assays (22). The hallmark response of innate immunity had been increased microglia activation and microglia recruitment to affected brain areas (13). Our results showing a strong shift from classical (CD14+CD16−) to non-classical (CD14+CD16+) monocytes within the intrathecal compartment represent a novel line of evidence for a response of innate immunity within the CNS. In contrast to a previous study that reported a significant increase in the proportion of PB classical monocytes in patients with PD (30), we found no significant differences in the proportion of peripheral monocyte subtypes. Monocytes originate from myeloid precursors in the bone marrow and are divided into two major subpopulations, the classical CD14+CD16− and the non-classical CD14+CD16+ monocytes (31). Classical monocytes are highly plastic and, upon recruitment to inflamed tissue, modify their phenotype according to the requirements of the specific microenvironment. They can differentiate into macrophages and are involved in tissue maintenance, pathogen clearance and induction of adaptive immune responses. Non-classical monocytes are thought to patrol along blood vessels and to be involved in tissue homeostasis and local regeneration, however recent reports describe them as the primary inflammatory monocyte subtype with properties for antigen presentation (32, 33). Together, our data showing a strong increase in the proportion of CSF, but not PB, non-classical monocytes in patients with PD and previous evidence of increased classical monocytes in the PB of patients with PD suggest the occurrence of specific intrathecal monocyte activation. Thus, it is tempting to speculate that in PD classical monocytes are recruited from the periphery across the blood-liquor barrier to give rise to non-classical monocytes within the CSF.
Changes in the chemokine profile have been reported in PD patients in different stages of the disease (34). Interestingly, we found increased levels of MCP-1 (CCL-2) in the CSF of PD patients. The MCP-1 receptor CCR2 is expressed on monocytes and MCP-1/CCR2 signaling is involved in the regulation of migration and infiltration of monocytes into host tissues (35). Increased levels of MCP-1 had been previously described in both serum and CSF samples of PD patients, and higher levels of MCP-1 correlated with manifestation of cognitive impairment and depression (30, 36, 37). However, only classical monocytes express high levels of CCR2. Therefore, the shift in monocyte subsets might be related (1) to an enhanced evasion of classical monocytes into the brain tissue or (2) classical monocytes increasingly developing into non-classical monocytes. We found no evidence for a peripheral expansion of non-classical monocytes and their subsequent migration into the CNS since the specific monocyte subtype composition was confined to the CSF compartment. Monocytes invading the CNS might develop into macrophages within the brain parenchyma, thus strengthening the innate arm of the CNS immune system (30, 38).
In addition to the well-established response of innate immunity, more recent evidence has suggested a role for the adaptive immune system in PD. Genome-wide association studies have established an association of PD with alleles of the major histocompatibility complex (MHC) (23). Previous PB immune cell phenotyping had demonstrated increased LRRK2 levels in peripheral lymphocytes, which are involved in the regulation of T cell activation and division (39). Neuropathological studies have reported T cell infiltration into the substantia nigra (13). Interestingly, quantitative analysis demonstrated a substantial accumulation of CD8+ T lymphocytes and to a lesser degree of CD4+ T lymphocytes within the substantia nigra, although animal models suggested a more enigmatic functional role of the latter in the disease process (10). Our data support the neuropathological post-mortem results and provide the first direct in vivo evidence in man showing increased invasion of T lymphocytes into the intrathecal compartment and their functional activation. Recently, an elegant series of immunological studies led to the current concept of the contribution of T lymphocytes to the pathogenesis of PD: degenerating dopaminergic neurons present modified α-synuclein-derived peptides via MHC class I molecules on their surface and release them to the extracellular space, where they activate microglia, and drain into the periphery outside the CNS. In lymph nodes, they activate antigen-presenting cells, which present the α-synuclein fragments via MHC II surface molecules leading to T lymphocyte activation. The latter infiltrate the CNS and accumulate at sites of inflammation, where they are re-stimulated by α-synuclein-presenting neurons and microglia. This may result in exacerbated inflammation, oxidative stress, and neuronal injury (7, 10, 40, 41). Our data contribute to these findings by providing direct in vivo evidence for activated T lymphocytes in the intrathecal compartment.
In accordance to previous studies, we found increased levels of the pro-inflammatory cytokines IL-2, IL-6, and TNFα (12, 20). However, we were not able to detect a cytokine pattern indicating the enrichment of a specific T-helper (TH) subtype. Interestingly, IL-9 was decreased in PD patients, a pleiotropic cytokine with supposed regulatory effects in CNS autoimmunity (42). Low patient numbers and the assays' detection limits might affect our results or explain differences between them and previous studies. Concomitant autoimmune diseases, anti-inflammatory co-medication, or evidence of an acute inflammatory process at the time of CSF withdrawal were exclusion criteria for patients in the present study. However, we cannot exclude the possibility of unknown comorbidities that may affect results in the presented patient cohort.
In conclusion, our results demonstrate a shift of monocyte subsets and activation of T lymphocytes in the CSF of PD patients. Although it remains unclear whether such alterations play a primary or secondary role in neurodegeneration, our results provide a new tier of evidence for activation of both innate and adaptive immune responses in the disease course of PD. Moreover, dysregulated immune cells may represent interesting molecular targets accessible as biomarkers for the identification of disease-associated neuroinflammatory processes and amenable to therapeutic intervention. Such intervention could have positive clinical effects and potentially modify the disease course. Future studies are necessary to corroborate our findings. Correlation analysis based on larger cohorts will be necessary to link CSF immune cell responses to PD disease stages and other clinical and paraclinical parameters. Additional cellular surface markers and translational research will be required to unveil the cascade of events leading to altered CSF monocyte and lymphocyte phenotypes and define their origins and exact cellular identities.
Author Contributions
JBS conceived, organized and executed the research project and reviewed the manuscript. MP conceived the research project, performed data analysis and wrote the first draft of the manuscript. GM helped to conceive the study and reviewed the manuscript. CCG performed data analysis and reviewed the manuscript. HW helped to conceive the study and reviewed the manuscript. SGM helped to conceive the study and reviewed the manuscript. TR conceived the research project, performed data analysis and reviewed the manuscript. TW conceived and supervised the research project and reviewed the manuscript.
Conflict of Interest Statement
GM has received speaker honoraria and compensation for serving on Scientific Advisory Boards for LFB Pharma and Alexion Pharma. GM received research support from the Deutsche Forschungsgemeinschaft (DFG, grant number ME4050/4-1), from the Gemeinnützige Hertie Stiftung, from the Innovative Medical Research (IMF) program of the Westfälische Wilhelms-University Münster, and from the Ministerium für Innovation, Wissenschaft und Forschung (MIWF) des Landes Nordrhein-Westfalen. CCG received speaker honoraria and travel expenses for attending meetings from Bayer Health Care, Genzyme, and Novartis Pharma GmbH. HW received compensation for serving on Scientific Advisory Boards/Steering Committees, for Bayer Healthcare, Biogen Idec, Sanofi—Genzyme, Merck Serono, and Novartis. He has received speaker honoraria and travel support from Bayer Vital GmbH, Bayer Schering AG, Biogen, CSL Behring, EMD Serono, Fresenius Medical Care, Genzyme, Merck Serono, Omniamed, Novartis, and Sanofi Aventis. He has received compensation as a consultant from Biogen Idec, Merck Serono, Novartis, Roche, and Sanofi-Genzyme. HW also received research support from Bayer Healthcare, Bayer Vital, Biogen Idec, Merck Serono, Novartis, Sanofi—Genzyme, Sanofi US, and TEVA Pharma as well as the German Ministry for Education and Research (BMBF), Deutsche Forschungsgesellschaft (DFG), Else Kröner Fresenius Foundation, Fresenius Foundation, Hertie Foundation, Merck Serono, Novartis, NRW Ministry of Education and Research, Interdisciplinary Center for Clinical Studies (IZKF) Muenster, RE Children's Foundation. SGM received honoraria for lecturing, travel expenses for attending meetings, and financial research support from Almirall, Bayer Health Care, Biogen, Diamed, Genzyme, Merck Serono, Novartis, Novo Nordisk, ONO Pharma, Roche, Sanofi-Aventis, and Teva. TR received travel reimbursements from Merck Serono and financial research support from Sanofi Genzyme and Novartis and honoraria for lecturing from Sanofi Genzyme, Roche, Biogen, Merck, and Teva. TW has received lecture fees from UCB, Zambon, Bial, Licher, Teva, Bayer, and Abbvie, and worked as a consultant for Archimedes, UCB, and Abbvie.
The remaining authors declare that the research was conducted in the absence of any commercial or financial relationships that could be construed as a potential conflict of interest.
Acknowledgments
We want to express our great gratitude to Gerhard Schmidt (1944–2016) who suffered from Parkinson's disease for more than 10 years and his family. With donations that were collected during the funeral of GMZH, they crucially helped to realize this project.
Supplementary Material
The Supplementary Material for this article can be found online at: https://www.frontiersin.org/articles/10.3389/fneur.2018.01081/full#supplementary-material
References
1. Kalia LV, Lang AE. Parkinson's disease. Lancet (2015) 386:896–912. doi: 10.1016/S0140-6736(14)61393-3
2. Pringsheim T, Jette N, Frolkis A, Steeves TDL. The prevalence of Parkinson's disease: a systematic review and meta-analysis. Mov Disord. (2014) 29:1583–90. doi: 10.1002/mds.25945
3. Massano J, Bhatia KP. Clinical approach to Parkinson's disease: features, diagnosis, and principles of management. Cold Spring Harb Perspect Med. (2012) 2:a008870. doi: 10.1101/cshperspect.a008870
4. Postuma RB, Berg D, Stern M, Poewe W, Olanow CW, Oertel W, et al. MDS clinical diagnostic criteria for Parkinson's disease. Mov Disord. (2015) 30:1591–601. doi: 10.1002/mds.26424
5. Chaudhuri KR, Healy DG, Schapira AHV, National Institute for Clinical Excellence. Non-motor symptoms of Parkinson's disease: diagnosis and management. Lancet Neurol. (2006) 5:235–45. doi: 10.1016/S1474-4422(06)70373-8
6. Dickson DW. Parkinson's disease and parkinsonism: neuropathology. Cold Spring Harb Perspect Med. (2012) 2:1–15. doi: 10.1101/cshperspect.a009258
7. Mosley RL, Hutter-Saunders JA, Stone DK, Gendelman HE. Inflammation and adaptive immunity in Parkinson's disease. Cold Spring Harb Perspect Med. (2012) 2:a009381. doi: 10.1101/cshperspect.a009381
8. Perry VH. Innate inflammation in Parkinson's disease. Cold Spring Harb Perspect Med. (2012) 2:a009373. doi: 10.1101/cshperspect.a009373
9. Damier P, Hirsch EC, Zhang P, Agid Y, Javoy-Agid F. Glutathione peroxidase, glial cells and Parkinson's disease. Neuroscience (1993) 52:1–6.
10. Brochard V, Combadière B, Prigent A, Laouar Y, Perrin A, Beray-Berthat V, et al. Infiltration of CD4+ lymphocytes into the brain contributes to neurodegeneration in a mouse model of Parkinson disease. J Clin Invest. (2009) 119:182–92. doi: 10.1172/JCI36470
11. Imamura K, Hishikawa N, Ono K, Suzuki H, Sawada M, Nagatsu T, et al. Cytokine production of activated microglia and decrease in neurotrophic factors of neurons in the hippocampus of Lewy body disease brains. Acta Neuropathol. (2005) 109:141–50. doi: 10.1007/s00401-004-0919-y
12. Mogi M, Harada M, Riederer P, Narabayashi H, Fujita K, Nagatsu T. Tumor necrosis factor-alpha (TNF-alpha) increases both in the brain and in the cerebrospinal fluid from parkinsonian patients. Neurosci Lett. (1994) 165:208–10. doi: 10.1016/0304-3940(94)90746-3
13. McGeer PL, Itagaki S, Boyes BE, McGeer EG. Reactive microglia are positive for HLA-DR in the substantia nigra of Parkinson's and Alzheimer's disease brains. Neurology (1988) 38:1285–91.
14. Ouchi Y, Yoshikawa E, Sekine Y, Futatsubashi M, Kanno T, Ogusu T, et al. Microglial activation and dopamine terminal loss in early Parkinson's disease. Ann Neurol. (2005) 57:168–75. doi: 10.1002/ana.20338
15. Gerhard A. TSPO imaging in parkinsonian disorders. Clin Transl Imaging (2016) 4:183–190. doi: 10.1007/s40336-016-0171-1
16. Gerhard A, Pavese N, Hotton G, Turkheimer F, Es M, Hammers A, et al. In vivo imaging of microglial activation with [11C](R)-PK11195 PET in idiopathic Parkinson's disease. Neurobiol Dis. (2006) 21:404–12. doi: 10.1016/j.nbd.2005.08.002
17. Edison P, Ahmed I, Fan Z, Hinz R, Gelosa G, Ray Chaudhuri K, et al. Microglia, amyloid, and glucose metabolism in Parkinson's disease with and without dementia. Neuropsychopharmacology (2013) 38:938–49. doi: 10.1038/npp.2012.255
18. Iannaccone S, Cerami C, Alessio M, Garibotto V, Panzacchi A, Olivieri S, et al. In vivo microglia activation in very early dementia with Lewy bodies, comparison with Parkinson's disease. Parkinsonism Relat Disord. (2013) 19:47–52. doi: 10.1016/j.parkreldis.2012.07.002
19. Müller T, Blum-Degen D, Przuntek H, Kuhn W. Interleukin-6 levels in cerebrospinal fluid inversely correlate to severity of Parkinson's disease. Acta Neurol Scand. (1998) 98:142–4.
20. Jiménez-Jiménez FJ, Alonso-Navarro H, García-Martín E, Agúndez JAG. Cerebrospinal fluid biochemical studies in patients with Parkinson's disease: toward a potential search for biomarkers for this disease. Front Cell Neurosci. (2014) 8:369. doi: 10.3389/fncel.2014.00369
21. Rayaprolu S, Mullen B, Baker M, Lynch T, Finger E, Seeley WW, et al. TREM2 in neurodegeneration: evidence for association of the p.R47H variant with frontotemporal dementia and Parkinson's disease. Mol Neurodegener. (2013) 8:19. doi: 10.1186/1750-1326-8-19
22. Hirsch EC, Hunot S. Neuroinflammation in Parkinson's disease: a target for neuroprotection? Lancet Neurol. (2009) 8:382–97. doi: 10.1016/S1474-4422(09)70062-6
23. Hamza TH, Zabetian CP, Tenesa A, Laederach A, Montimurro J, Yearout D, et al. Common genetic variation in the HLA region is associated with late-onset sporadic Parkinson's disease. Nat Genet. (2010) 42:781–5. doi: 10.1038/ng.642
24. Pawlowski M, Lueg G, Gross CC, Johnen A, Krämer J, Weckesser M, et al. Relevance of raised cerebrospinal fluid monocyte levels in patients with frontotemporal dementia. Neurobiol Aging (2017) 62:45–52. doi: 10.1016/j.neurobiolaging.2017.09.032
25. Hoehn MM, Yahr MD. Parkinsonism: onset, progression and mortality. Neurology (1967) 17:427–42. doi: 10.1212/WNL.17.5.427
26. Tomlinson CL, Stowe R, Patel S, Rick C, Gray R, Clarke CE. Systematic review of levodopa dose equivalency reporting in Parkinson's disease. Mov Disord. (2010) 25:2649–53. doi: 10.1002/mds.23429
27. Goetz CG, Tilley BC, Shaftman SR, Stebbins GT, Fahn S, Martinez-Martin P, et al. Movement Disorder Society-sponsored revision of the Unified Parkinson's Disease Rating Scale (MDS-UPDRS): scale presentation and clinimetric testing results. Mov Disord. (2008) 23:2129–70. doi: 10.1002/mds.22340
28. Nasreddine ZS, Phillips NA, Bédirian V, Charbonneau S, Whitehead V, Collin I, et al. The Montreal Cognitive Assessment, MoCA: a brief screening tool for mild cognitive impairment. J Am Geriatr Soc. (2005) 53:695–9. doi: 10.1111/j.1532-5415.2005.53221.x
29. Lueg G, Gross CC, Lohmann H, Johnen A, Kemmling A, Deppe M, et al. Clinical relevance of specific T-cell activation in the blood and cerebrospinal fluid of patients with mild Alzheimer's disease. Neurobiol Aging (2015) 36:81–9. doi: 10.1016/j.neurobiolaging.2014.08.008
30. Grozdanov V, Bliederhaeuser C, Ruf WP, Roth V, Fundel-Clemens K, Zondler L, et al. Inflammatory dysregulation of blood monocytes in Parkinson's disease patients. Acta Neuropathol. (2014) 128:651–63. doi: 10.1007/s00401-014-1345-4
31. Ziegler-Heitbrock L, Ancuta P, Crowe S, Dalod M, Grau V, Hart DN, et al. Nomenclature of monocytes and dendritic cells in blood. Blood (2010) 116:e74–80. doi: 10.1182/blood-2010-02-258558
32. Zondler L, Müller K, Khalaji S, Bliederhäuser C, Ruf WP, Grozdanov V, et al. Peripheral monocytes are functionally altered and invade the CNS in ALS patients. Acta Neuropathol. (2016) 132:391–411. doi: 10.1007/s00401-016-1548-y
33. Mitchell AJ, Roediger B, Weninger W. Monocyte homeostasis and the plasticity of inflammatory monocytes. Cell Immunol. (2014) 291:22–31. doi: 10.1016/j.cellimm.2014.05.010
34. Chen H, O'Reilly EJ, Schwarzschild MA, Ascherio A. Peripheral inflammatory biomarkers and risk of Parkinson's disease. Am J Epidemiol. (2008) 167:90–5. doi: 10.1093/aje/kwm260
35. Stansfield BK, Ingram DA. Clinical significance of monocyte heterogeneity. Clin Transl Med. (2015) 4:5. doi: 10.1186/s40169-014-0040-3
36. Hall S, Janelidze S, Surova Y, Widner H, Zetterberg H, Hansson O. Cerebrospinal fluid concentrations of inflammatory markers in Parkinson's disease and atypical parkinsonian disorders. Sci Rep. (2018) 8:13276. doi: 10.1038/s41598-018-31517-z
37. Lindqvist D, Hall S, Surova Y, Nielsen HM, Janelidze S, Brundin L, et al. Cerebrospinal fluid inflammatory markers in Parkinson's disease–associations with depression, fatigue, and cognitive impairment. Brain Behav Immun. (2013) 33:183–9. doi: 10.1016/j.bbi.2013.07.007
38. Mildner A, Schmidt H, Nitsche M, Merkler D, Hanisch U-K, Mack M, et al. Microglia in the adult brain arise from Ly-6ChiCCR2+ monocytes only under defined host conditions. Nat Neurosci. (2007) 10:1544–53. doi: 10.1038/nn2015
39. Cook DA, Kannarkat GT, Cintron AF, Butkovich LM, Fraser KB, Chang J, et al. LRRK2 levels in immune cells are increased in Parkinson's disease. NPJ Park Dis. (2017) 3:11. doi: 10.1038/s41531-017-0010-8
40. Mosley RL, Gendelman HE. T cells and Parkinson's disease. Lancet Neurol. (2017) 16:769–71. doi: 10.1586/ern.11.163
41. Sulzer D, Alcalay RN, Garretti F, Cote L, Kanter E, Agin-Liebes J, et al. T cells from patients with Parkinson's disease recognize α-synuclein peptides. Nature (2017) 546:656–61. doi: 10.1038/nature22815
Keywords: Parkinson's disease, cerebrospinal fluid, immune cells, T lymphocytes, monocytes
Citation: Schröder JB, Pawlowski M, Meyer zu Hörste G, Gross CC, Wiendl H, Meuth SG, Ruck T and Warnecke T (2018) Immune Cell Activation in the Cerebrospinal Fluid of Patients With Parkinson's Disease. Front. Neurol. 9:1081. doi: 10.3389/fneur.2018.01081
Received: 10 September 2018; Accepted: 27 November 2018;
Published: 18 December 2018.
Edited by:
Thomas Skripuletz, Hannover Medical School, GermanyReviewed by:
Anne-Katrin Pröbstel, University of California, San Francisco, United StatesLars Tönges, University Hospitals of the Ruhr-University of Bochum, Germany
Copyright © 2018 Schröder, Pawlowski, Meyer zu Hörste, Gross, Wiendl, Meuth, Ruck and Warnecke. This is an open-access article distributed under the terms of the Creative Commons Attribution License (CC BY). The use, distribution or reproduction in other forums is permitted, provided the original author(s) and the copyright owner(s) are credited and that the original publication in this journal is cited, in accordance with accepted academic practice. No use, distribution or reproduction is permitted which does not comply with these terms.
*Correspondence: Tobias Warnecke, tobias.warnecke@ukmuenster.de
†These authors have contributed equally to this work