- 1San Camillo Hospital IRCCS, Venice, Italy
- 2Department of Human Neurosciences, Sapienza University, Policlinico Umberto I Hospital, Rome, Italy
- 3Neurology Department, Campus Biomedico University of Rome, Rome, Italy
Introduction: Eslicarbazepine acetate (ESL) is an antiepileptic drug approved as monotherapy or add-on for the treatment of epilepsy with seizures of focal onset. ESL owns a good profile in terms of efficacy and tolerability, but its effects on EEG activity and connectivity are unknown. The purpose of this study was to investigate EEG activity and connectivity changes after ESL treatment in persons with focal epilepsy (PFE).
Material and Methods: We performed a multicentre, longitudinal, retrospective, quantitative EEG study on a population of 22 PFE, and a group of 40 controls. We investigated the ESL-related changes of EEG power spectral activity and global connectivity [phase locking value (PLV), amplitude envelope correlation (AEC) and amplitude envelope correlation of orthogonalized signals (Ortho-AEC)] for standard frequency bands (delta to gamma). Seizure frequency was evaluated to assess ESL efficacy in our cohort.
Results: ESL significantly enhanced both global power spectral density and connectivity for all frequency bands, similarly for all connectivity measures. When compared to the control group, Post-ESL power was significantly higher in theta and gamma band. Pre-ESL connectivity values were significantly lower than control for all frequency bands. Post-ESL connectivity increased and the gap between the two groups was no longer significant. ESL induced a 52.7 ± 41.1% reduction of seizure frequency, with 55% of clinical responders (reduction of seizures ≥50%).
Discussion: ESL therapy induces significant enhancement of brain activity and connectivity. Post-ESL connectivity profile of epilepsy patients was similar to the one of healthy controls.
Introduction
Eslicarbazepine (ESL) is an antiepileptic drug (AED) approved for the treatment of epilepsy with seizures of focal origin (1). ESL is more effective than placebo as add-on therapy (2), is effective and safe in monotherapy in patients with uncontrolled partial-onset seizures (3–5) and has better side effects profile when compared to carbamazepine and oxcarbazepine (6).
The molecular mechanism on which ESL relies to express its therapeutic action is mainly related to the inactivation of voltage-gated sodium channels, similarly to oxcarbazepine, and carbamazepine (7). This mechanism is very specific, as ESL does not bind the receptors for benzodiazepine, GABA and glutamate (8). Differences between ESL and oxcarbazepine and carbamazepine depend on a variety of factors, but largely on the pharmacokinetic profile (7).
Very little is known on the effects of this medication on the brain cortex as a system. AEDs have an effect on brain networks and although the literature is poor of studies explicitly addressing this topic, there is plenty of evidence that antiepileptic medications influence cortical rhythms and networks as measured by EEG (9–14).
EEG is a sensitive and reliable tool to substantiate cortical function. In particular, each frequency band power (alpha, beta, theta, delta, gamma) owns a functional significance. In epilepsy patients, the study of rapid rhythms can help in better understanding positive AED effects of cognition, attention, perception and language networks. On the opposite, the study of slow frequency bands allows to detect effects on memory, drowsiness and cortical plasticity [for a review see Assenza et al. (15)]. In a recent study our group demonstrated that ESL is an AED with a very good efficacy against seizures with a positive impact on sleepiness, depressive symptoms and quality of life (16).
Here we have performed a multicentric, longitudinal study to investigate the impact of ESL on cortical activity and connectivity, as explored by quantitative EEG, in a population of persons with seizures with focal onset (PFE).
Materials and Methods
Patients and Study Design
Twenty-two PFE (ILAE definition, mean age = 46 ± 16.7 (SD); 46% females) and a control group of forty healthy subjects (mean age = 50.6 ± 19.04; 45% female, p > 0.200 consistently) were enrolled at the epilepsy clinic of Department of Human Neurosciences enrolled of Policlinco Umberto I Universitary Hospital of Rome and of Campus Bio-Medico University of Rome. The study was approved by the ethic committee of Policlinco Umberto I Ethic Board—Rome—and Campus Biomedico University Ethic Board—Rome-. All patients signed a written informed consent. All procedures were performed in agreement with the 1964 Helsinki declaration and its later amendments.
For the epilepsy group the inclusion and exclusion criteria were the following:
Inclusion:
- PFE > 18 years-old;
- two clinical EEG recordings occurring immediately before (< 30 days) ESL assumption and after at least 3 months of ESL assumption;
- resting state EEG free of relevant artifact > 5 min.
Exclusion:
- PFE taking neuroactive drugs other than antiepileptic drugs;
- antiepileptic medication titration in between the two EEG recordings.
- Clinical seizures in the 24 h before EEGs
Healthy subjects were enrolled among the relatives of patients investigated at the outpatient clinic, hospital personnel, and volunteers and were interviewed by a neurologist to rule out medical conditions potentially biasing the study. Patients underwent standard clinical EEG recording before starting eslicarbazepine acetate and after a 3 months period of follow-up. Detailed clinical info is reported in Table S1.
EEG Recording and Analysis
Nineteen channel-EEG was acquired with a Micromed recorder (Micromed, Mogliano Veneto, IT) between 11:00 and 13:00 a.m. and between 16:00 and 18:00 p.m to minimize drowsiness. The electrodes were placed according to the international 10–20 system (Fp1, Fp2, F3, F4, C3, C4, P3, P4, F7, F8, T3, T4, T5, T6, O1, O2, Fz, Cz, Pz). The reference was placed on FPz and the ground on FCz. Impedance was kept below 5 kOhm for all electrodes. The sampling rate was set to 256 Hz. Eye blinks, eye movements and electrocardiogram (EKG) were recorded using dedicated bipolar electrodes. The resting EEG recording lasted 5 min and was performed with patients with closed eyes, seated on a comfortable armchair in a quiet room. Patients were instructed as follows: “Free your mind, do not think about anything and just relax.” Subjects were also asked to keep their regular wake/sleep cycle before participation. Pre ad Post medication EEGs were measured always using the same apparatus.
EEG signals were analyzed using the EEGLab Toolbox (17), the Brainstorm Toolbox (18) for Matlab (The Math Works Inc, Natick, MA), and in home Matlab code. Both EEGLab and Brainstorm are documented and freely available for download online under the GNU general public license (https://sccn.ucsd.edu/eeglab/ and http://neuroimage.usc.edu/brainstorm). EEG pre-processing consisted of: (a) visual inspection for rejection of possible electrographic seizures, interictal epileptic activity, bad channels, (b) [1–70Hz] band-pass filter, (c) [50Hz] notch filter, (d) Independent Component Analysis (ICA) (19) to remove artifacts related to heartbeat and eye movements, (e) spline interpolation of previously rejected bad channels, (f) re-reference to average reference (20, 21).
Epochs containing interictal epileptic activity (spikes, spike and wave complexes, spiky waves, bouffè of monomorphic slow waves) were rejected. Those PFE with one EEG recording containing more than 20% of interictal epileptic activity were excluded (22).
Brain Network Analysis: Resting State Activity and Connectivity
To assess the effect of ESL on brain networks we performed measures of resting state brain activity and connectivity. As measure of activity, we computed the Power Spectrum Density (PSD) by standard FFT approach (Welch procedure: average of non-overlapped windows with a duration of 2 s) for the following frequency bands (delta: 2–4 Hz; theta: 5–7 Hz, alpha: 8–12, Hz, beta1: 13–20 Hz, beta2: 21–29 Hz, gamma: 30–60 Hz). To obtain a measure of global activity, we averaged PSD measures over all channels.
As for connectivity, we focused on the Phase Locking Value (PLV). Phase Locking Value is a very popular measure of non-directional frequency-specific synchronization reflecting long-range integrations. It assesses the extent to which the phase difference between two signals changes over time and is a measure of cortical synchronization based on phase covariance between signals. PLV owns a good time resolution and provides insight on the synchronization of fast changing electrophysiological dynamic. High PLV indicates higher synchronization (23–27). We measured PLV for all possible channel combinations and averaged to obtain a measure of global connectivity. We focused on the same frequency bands as for brain activity (namely, delta: 2–4 Hz; theta: 5–7 Hz, alpha: 8–12, Hz, beta1: 13–20 Hz, beta2: 21–29 Hz, gamma: 30–60 Hz).
The low number of sensors and the detrimental effect of volume conduction on estimation of EEG connectivity might be an issue when assessing connectivity between sensors or brain regions (28). Therefore, we also estimated as additional measures of cortical connectivity. In particular we focused on the Amplitude Envelope Correlation (AEC) and its variant estimated on orthogonalized signals (Ortho-AEC) that regresses out lag = 0 synchrony and minimizes volume conduction issues. To be noted, all connectivity measures estimated after orthogonalization do not only remove the influence of volume conduction, but also the true lag = 0 connectivity. Both AEC and Ortho-AEC capture different features of the connectivity pattern as compared to PLV (29). In further details, AEC measures the linear correlations between the amplitude of the envelops of band-pass filtered signals, relies on the temporal dynamics of relative slow power fluctuations and provides an EEG connectivity measure resembling—at least on some extent—connectivity patterns unveiled by fMRI analysis of BOLD signal (30–33). Finally, to further get rid of the volume conduction bias, we focused on global connectivity and on a within subject pre-post medication paired comparison.
Clinical and Behavioral Assessment
ESL efficacy was measured on the percentage of seizures reduction. We defined as responders those patients with seizure reduction ≥50% and non-responders the remaining ones.
As EEG activity is very sensitive to sleepiness and cognitive performance (19), we searched in patients' files for the Epworth Sleepiness Scale (ESS) and Stanford sleepiness scale to assess sleepiness and the Beck Depression Inventory-II (BDI) scale to assess depression (34, 35). Sleepiness and depression scores were available for 12 out of 22 patients.
Statistical Analysis
Statistical analysis was performed using the IBM SPSS Statistics (Ver. 24) and Matlab (Mathworks). Data distribution was checked by means of Kolmogorov-Smirnov test. The effect of ESL on global cortical power and connectivity within the epilepsy group was tested with a repeated measure ANOVA with Band (six levels: Delta, Theta, Alpha, Beta1, Beta2, Gamma) and Time (two levels: Pre-ESL and Post-ESL) as within subject factors. In order to compare the epilepsy group and the control group, we applied a mixed model repeated measure ANOVA, with Band (six levels: Delta, Theta, Alpha, Beta1, Beta2, Gamma) as within subject factor and Group two levels: Control and Pre-ESL (or Post-ESL) as between subjects factor. The Greenhouse-Geisser correction was applied when needed. A paired t-test was used to study changes of behavioral scale scores. The significance level was set to alpha = 0.05.
Results
Pre-post Comparison Within the Epilepsy Group
EEG Activity
The repeated measure ANOVA showed a significant main effect of the factor Time [F(1, 21) = 7.288, p = 0.013], suggesting a global increase of connectivity after ESL. No significant Band by Time interaction was found [F (3.081, 64.692) = 2.657, p = 0.054], ruling out a specific ESL effect for selected frequency bands (Figure 1). A significant main effect of the factor Band [F (2.598, 54.551) = 1077.993, p < 0.001] was also found, suggesting an expected difference in power across bands.
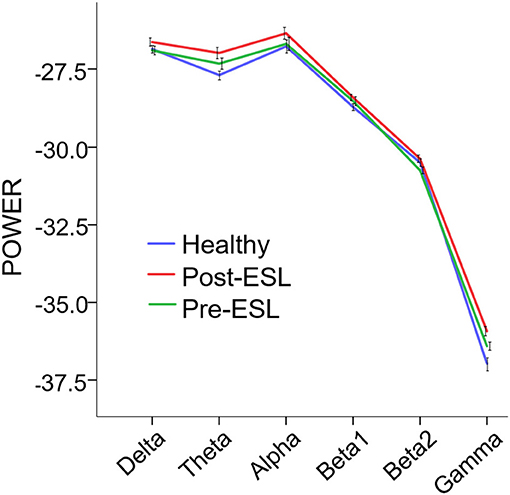
Figure 1. Power Spectral Density (PSD) Pre and Post eslicarbazepine acetate and for the control group. PSD is expressed as average across channels. Error bars represent standard error of the mean. PSD was globally higher post-eslicarbazepine acetate than before.
EEG Connectivity
The repeated measure ANOVA revealed a significant main effect of the factor Time [F (1, 21) = 4.359, p = 0.049] related to a global increase of connectivity after ESL (Figure 2). No significant Band by Time interaction was found [F (2.702, 56.736) = 0.196, p = 0.881], ruling out a specific ESL effect for selected frequency bands. A significant Band main effect [F (2.088, 43.843) = 6.101, p = 0.004] confirmed the expected connectivity modulation over frequency bands. Similar results were found on different measures of brain connectivity. For AEC a significant factor Time [F (1, 21) = 5.277, p = 0.032], together with lack of Band by Time interaction, suggested a global higher connectivity level Post-ESL as compared to Pre-ESL. A similar trend, although without reaching statistical significance, was revealed by the analysis of the orthogonalized version of AEC [Factor Time: F(1, 21) = 2.527, p = 0.127] (Figure 2).
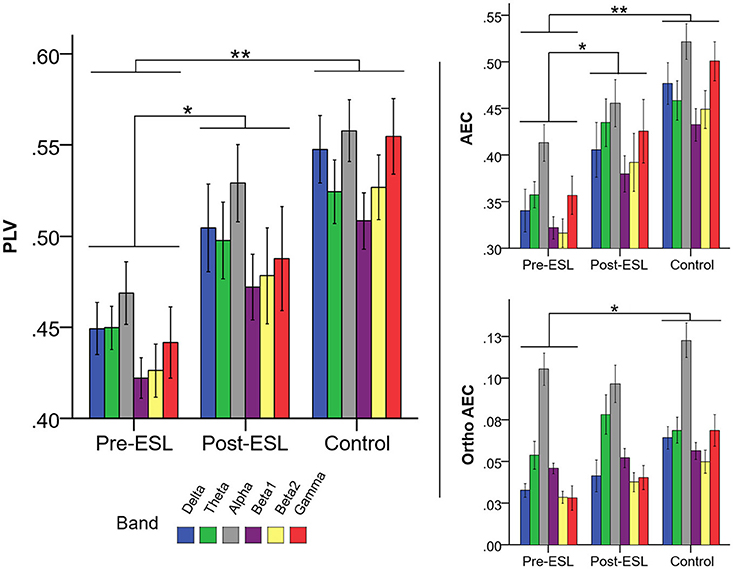
Figure 2. Connectivity Pre and Post eslicarbazepine acetate and for the control group. Left panel shows PLV, right panel shows AEC, and AEC computed on orthogonalized data. Connectivity was higher post-eslicarbazepine acetate than before. Pre-ESL connectivity values were significantly lower than control for all frequency bands. Post-ESL connectivity increased and the gap between the two groups was no longer significant. A similar pattern was observed for all connectivity measures under investigation. *denotes p < 0.05; **denotes p < 0.001.
Comparison Between Epilepsy and Control Groups
EEG Activity
The comparison between Pre-ESL and controls revealed no significant difference between groups [Factor Group: F (1, 60) = 0.695, p > 0.200], but a significant Band by Group interaction [F(5, 300) = 3.298, p = 0.006]. The post-hoc tests, however, did not reveal any significant difference after Bonferroni correction. The comparison between Post-ESL and controls also revealed a significant Band by Group interaction [F (5, 300) = 4.979, p < 0.001] related to a significantly higher Post-ESL power for Theta and Gamma bands (Bonferroni-corrected post-hoc comparisons p = 0.018 and p = 0.06 respectively).
EEG Connectivity
The Comparison of PLV values between Pre-ESL and Controls revealed a significant factor Group [F (1, 60) = 15.565, p < 001] and no interaction, suggesting that PLV connectivity was significantly higher in the controls as compared to epilepsy patients before ESL administration. Notably, no significant Group difference nor interactions were found when comparing Controls vs. Post-ESL [main factor p = 0.129, interaction factor p > 0.200], suggesting that ESL reduced the gap between the epilepsy group and controls for all frequency bands (Figure 2).
The same profile was confirmed for the other two measures of brain connectivity, namely AEC, and its orthogonalized version. Indeed, in all cases the comparison between Controls and Pre-ESL revealed a significant Group factor and no significant interactions [AEC: Factor Group F (1, 60) = 22.815, p < 0.001, Group by Band interaction p > 0.200; Orthogonalized AEC: Factor Group F (1, 60) = 10.281, p = 0.002, Group by Band interaction p > 0.200], whereas the comparison between Controls and Post-ESL did not reveal any significant Factor Group or Group by Band interaction [AEC: Factor Group F (1, 60) = 3.875, p = 0.054, Group by Band interaction p > 0.200; Orthogonalized AEC: Factor Group F (1, 60) = 3.351, p = 0.064, Group by Band interaction p > 0.200] (Figure 2).
Clinical and Behavioral Assessment
Twelve patients responded to ESL (54.6%; 6 seizure free) after an average period of treatment of 83.1 ± 19.6 days. None of our patients increased the seizure frequency. The mean seizure reduction was 53.2 ± 42.6% (mean ± standard deviation; median 50%, ranges 0–100%). In the subgroup of patients with available behavioral data, after ESL, sleepiness assessment showed an improvement of ESS (ESS Pre-ESL 6.3 ± 3.1; ESS Post ESL 3.88 ± 2.7; p < 0.001) and an unchanged SSS (p = 0.08). Depression values were also ameliorated after ESL (BDI, p = 0.006) with respect to the Pre.
Discussion
In the present study we demonstrated that ESL treatment induces cortical EEG activity and connectivity changes in a population of PFE.
The power increase within the epilepsy group was broadband, from delta to gamma. A broadband cortical activity enhancement can depend upon an increased number of neurons firing together or the same group of neurons firing more synchronously (36). The joint increase of activity and connectivity might support a better and stronger engagement of large cortical regions in resting state networks. It is well-known that the epileptic focus modifies the connectivity of the affected cortex with its physiologically-connected regions so that patients with epilepsy show lower global connectivity than controls, with connectivity levels pairing more severe epilepsy conditions (37). We confirmed that patients exhibits lower connectivity levels as compared to controls but–importantly- we also demonstrated that after a 3 months course of eslicarbazepine there was a significant connectivity increase, up to a level very close to that of healthy subjects.
As we observed a selective power increase in theta and gamma band when comparing Post-ESL vs. healthy controls, we could also speculate that ESL exerts a specific action on each frequency band. Although this finding might be a false positive as Pre-ESL theta and gamma activity was slightly higher in epilepsy patients than healthy (Figure 1), we should note that gamma oscillations facilitate synchronization and information transfer, are a positive indicator of brain function and are often associated to clinical improvement (38). Theta (and delta) oscillations can indicate drowsiness (but in our cohort there was no clinical sleepiness increase), augmented cognitive load and network reshaping through long-lasting brain plasticity (36, 39, 40). Finally, the increase of alpha and beta oscillations might improve the cortical resilience to epileptic activity. Alpha oscillations are prominently engaged in visual and attention networks and play an inhibitory role (41–45). Beta activity is largely a residential rhythm of the pyramidal and extra-pyramidal motor network and is considered the player of information gating involved in the maintenance of the status quo of neuronal networks (46, 47).
This study was not designed to compare multiple antiepileptic medications, however we would like to underline that ESL has a similar tolerability profile to recent AED such as levetiracetam (only mild impact on cognition and EEG) and behaves potentially better than carbamazepine and topiramate, which selectively increase delta and theta bands, reduce faster cortical rhythms and more often result in cognitive impairment (11, 13).
Very recently the study of brain connectivity and networks has led to a new conceptualization of focal epilepsy, showing that it is neither involving the entire brain nor a single cortical spot, rather specific brain networks (48). Epileptic activity drives a certain degree of plastic reorganization of brain networks (49). The healthy connections across multiple areas become impaired and patients show increased connectivity of the networks close to the epileptic focus (local networks) and decreased connectivity of long-range networks (37). This re-organization can be clinically relevant, as it can improve the accuracy of the diagnosis (50), help the presurgical planning (51), and represent a marker of the efficacy of epilepsy surgery, as clinical outcome correlates with the degree of restoration of network architecture (52). Rather than be simple proxy of the underlying pathology, network reorganization is therefore a key aspect, that makes focal epilepsy a network disorder. Here we demonstrated a consistent change of cortical connectivity unveiled by three different measures and support the concept of focal epilepsy as a disorder affecting the cortical networks (19).
Future studies should rely on a sample size sufficiently large to allow the characterization of the topographical distribution of the effects in relation to the location of the epileptic focus and to other determinant variables, such as: etiology, disease duration, age (54). This experiment was not directly designed for a clinical application and the results found on group level cannot be applied at single subject level in a clinical setting. Nonetheless, our study demonstrated that simple measures extracted from EEG recording performed in a clinical setting can unveil remarkable effects of medication and, once replicated on larger and better controlled cohorts, might be considered for possible future clinical applications. Such approach could be of translational interest as it might be hypothesized to pursue specific and positive changes of brain activity and connectivity with pharmacological and non-invasive brain stimulation treatments (53, 55, 56).
In conclusion, in this proof-of-principle study we demonstrated that ESL treatment produced an increase in global cortical power and connectivity and encourage the study of quantitative EEG as a potential tool for assessing epilepsy and the effects of antiepileptic medications.
Author Contributions
GP, OM, PP, MT, VD, and GA: Study design; OM, PP, MT, LR, JL, MB, CD, and GA: Patients recruitment; GP, MT, VD, and GA: Data analysis; GP, GA, OM, VD, and MT: Data interpretation; GP, OM, and GA: Manuscript preparation; All: Manuscript revision.
Funding
This Investigator-Initiated Study and the publication fees were funded by Eisai s.r.l. GP has been supported by a Ministry of Health Operating Grant to the San Camillo IRCCS Venice.
Conflict of Interest Statement
The authors declare that the research was conducted in the absence of any commercial or financial relationships that could be construed as a potential conflict of interest.
Acknowledgments
The Authors acknowledge the contribution of the patients and their kind support.
Supplementary Material
The Supplementary Material for this article can be found online at: https://www.frontiersin.org/articles/10.3389/fneur.2018.01054/full#supplementary-material
References
1. Elger C, Halász P, Maia J, Almeida L, Soares-da-Silva P. Efficacy and safety of eslicarbazepine acetate as adjunctive treatment in adults with refractory partial-onset seizures: a randomized, double-blind, placebo-controlled, parallel-group phase III study. Epilepsia (2009) 50:454–63. doi: 10.1111/j.1528-1167.2008.01946.x
2. Gil-Nagel A, Elger C, Ben-Menachem E, Halász P, Lopes-Lima J, Gabbai AA, et al. Efficacy and safety of eslicarbazepine acetate as add-on treatment in patients with focal-onset seizures: Integrated analysis of pooled data from double-blind phase III clinical studies. Epilepsia (2013) 54:98–107. doi: 10.1111/j.1528-1167.2012.03605.x
3. Jacobson MP, Pazdera L, Bhatia P, Grinnell T, Cheng H, Blum D. Efficacy and safety of conversion to monotherapy with eslicarbazepine acetate in adults with uncontrolled partial-onset seizures: a historical-control phase III study. BMC Neurol. (2015) 15:46. doi: 10.1186/s12883-015-0305-5
4. Sperling MR, Harvey J, Grinnell T, Cheng H, Blum D. Efficacy and safety of conversion to monotherapy with eslicarbazepine acetate in adults with uncontrolled partial-onset seizures: a randomized historical-control phase III study based in North America. Epilepsia (2015) 56:546–55. doi: 10.1111/epi.12934
5. Sperling MR, French J, Jacobson MP, Pazdera L, Gough M, Cheng H, et al. Conversion to eslicarbazepine acetate monotherapy A pooled analysis of 2 phase III studies. Neurology (2016) 86:1095–102. doi: 10.1212/WNL.0000000000002497
6. Villanueva V, Serratosa J, Guillamón E, Garcés M, Giráldez B, Toledo M, et al. Long-term safety and efficacy of eslicarbazepine acetate in patients with focal seizures: results of the 1-year ESLIBASE retrospective study. Epilepsy Res. (2014) 108:1243–52. doi: 10.1016/j.eplepsyres.2014.04.014
7. Soares-da-Silva P, Pires N, Bonifácio MJ, Loureiro AI, Palma N, Wright LC. Eslicarbazepine acetate for the treatment of focal epilepsy: an update on its proposed mechanisms of action. Pharmacol Res Perspect. (2015) 3:e00124. doi: 10.1002/prp2.124
8. Łuszczki JJ. Third-generation antiepileptic drugs: mechanisms of action, pharmacokinetics and interactions. Pharmacol Rep. (2009) 61:197–216. doi: 10.1016/S1734-1140(09)70024-6
9. Marciani M, Gigli G, Stefanini F, Sabbadini M, Stefani N, Maschio M, et al. Effect of carbamazepine on EEG background activity and on interictal epileptiform abnormalities in focal epilepsy. Int J Neurosci. (1993) 70:107–16. doi: 10.3109/00207459309000566
10. Marciani MG, Spanedda F, Bassetti MA, Maschio M, Gigli GL, Mattia D, et al. Effect of lamotrigine on EEG paroxysmal abnormalities and background activity: a computerized analysis. Br J Clin Pharmacol. (1996) 42:621–7. doi: 10.1111/j.1365-2125.1996.tb00057.x
11. Mecarelli O, Piacenti A, Pulitano P, Vicenzini E, Rizzo C, Rinalduzzi S, et al. Clinical and electroencephalographic effects of topiramate in patients with epilepsy and healthy volunteers. Clin Neuropharmacol. (2001) 24:284–9. doi: 10.1097/00002826-200109000-00005
12. Wang W, Li J, Wu X. Quantitative EEG effects of topiramate. Clin Electroencephalogr. (2003) 34:87–92. doi: 10.1177/155005940303400208
13. Mecarelli O, Vicenzini E, Pulitano P, Vanacore N, Romolo FS, Piero VD, et al. Clinical, cognitive, and neurophysiologic correlates of short-term treatment with carbamazepine, oxcarbazepine, and levetiracetam in healthy volunteers. Ann Pharmacother. (2004) 38:1816–22. doi: 10.1345/aph.1E136
14. Salinsky M, Storzbach D, Oken B, Spencer D. Topiramate effects on the EEG and alertness in healthy volunteers: a different profile of antiepileptic drug neurotoxicity. Epilep Behav. (2007) 10:463–9. doi: 10.1016/j.yebeh.2006.12.011
15. Assenza G, Capone F, di Biase L, Ferreri F, Florio L, Guerra A, et al. Oscillatory activities in neurological disorders of elderly: biomarkers to target for neuromodulation. Front Aging Neurosci. (2017) 9:189. doi: 10.3389/fnagi.2017.00189
16. Assenza G, Mecarelli O, Lanzone J, Assenza F, Tombini M, Di Lazzaro V, et al. The ROME (retrospective observational multicenter study on eslicarbazepine) study: efficacy and behavioural effects of Eslicarbazepine acetate as adjunctive therapy for adults with partial onset seizures in real life. Seizure (2018) 58:35–40. doi: 10.1016/j.seizure.2018.03.028
17. Delorme A, Makeig S. EEGLAB: an open source toolbox for analysis of single-trial EEG dynamics including independent component analysis. J Neurosci Methods (2004) 134:9–21. doi: 10.1016/j.jneumeth.2003.10.009
18. Tadel F, Baillet S, Mosher JC, Pantazis D, Leahy RM. Brainstorm: a user-friendly application for MEG/EEG analysis. Comput Intell Neurosci. (2011) 2011:879716. doi: 10.1155/2011/879716
19. Pellegrino G, Tombini M, Curcio G, Campana C, Di Pino G, Assenza G, et al. Slow activity in focal epilepsy during sleep and wakefulness. Clin EEG Neurosci. (2016) 48:1550059416652055. doi: 10.1177/1550059416652055
20. Pellegrino G, Hedrich T, Chowdhury R, Hall JA, Lina JM, Dubeau F, et al. Source localization of the seizure onset zone from ictal EEG/MEG data. Hum. Brain Mapp. (2016) 37:2528–46. doi: 10.1002/hbm.23191
21. Pellegrino G, Hedrich T, Chowdhury RA, Hall JA, Dubeau F, Lina JM, et al. Clinical yield of magnetoencephalography distributed source imaging in epilepsy: a comparison with equivalent current dipole method. Hum Brain Mapp. (2018) 39:218–31. doi: 10.1002/hbm.23837
22. Pellegrino G, Machado A, von Ellenrieder N, Watanabe S, Hall JA, Lina JM, et al. Hemodynamic response to interictal epileptiform discharges addressed by personalized EEG-fNIRS recordings. Front Neurosci. (2016) 10:102. doi: 10.3389/fnins.2016.00102
23. Pellegrino G, Maran M, Turco C, Weis L, Di Pino G, Piccione F, et al. Bilateral transcranial Direct Current Stimulation (tDCS) reshapes resting-state brain networks: a magnetoencephalography (MEG) assessment. Neural Plasticity (2018) 2018:2782804. doi: 10.1155/2018/2782804
24. Lachaux JP, Rodriguez E, Martinerie J, Varela FJ. Measuring phase synchrony in brain signals. Hum Brain Mapp. (1999) 8:194–208.
25. Varela F, Lachaux J-P, Rodriguez E, Martinerie J. The brainweb: phase synchronization and large-scale integration. Nat Rev Neurosci. (2001) 2:229. doi: 10.1038/35067550
26. Aydore S, Pantazis D, Leahy RM. A note on the phase locking value and its properties. Neuroimage (2013) 74:231–44. doi: 10.1016/j.neuroimage.2013.02.008
27. Hutchison RM, Womelsdorf T, Allen EA, Bandettini PA, Calhoun VD, Corbetta M, et al. Dynamic functional connectivity: promise, issues, and interpretations. Neuroimage (2013) 80:360–78. doi: 10.1016/j.neuroimage.2013.05.079
28. Brunner C, Billinger M, Seeber M, Mullen TR, Makeig S. Volume conduction influences scalp-based connectivity estimates. Front Comput Neurosci. (2016) 10:121. doi: 10.3389/fncom.2016.00121
29. Hipp JF, Hawellek DJ, Corbetta M, Siegel M, Engel AK. Large-scale cortical correlation structure of spontaneous oscillatory activity. Nat Neurosci. (2012) 15:884. doi: 10.1038/nn.3101
30. De Pasquale F, Della Penna S, Snyder AZ, Lewis C, Mantini D, Marzetti L, et al. Temporal dynamics of spontaneous MEG activity in brain networks. Proc Natl Acad Sci. USA. (2010) 107:6040–5. doi: 10.1073/pnas.0913863107
31. Brookes MJ, Hale JR, Zumer JM, Stevenson CM, Francis ST, Barnes GR, et al. Measuring functional connectivity using MEG: methodology and comparison with fcMRI. Neuroimage (2011) 56:1082–104. doi: 10.1016/j.neuroimage.2011.02.054
32. Hipp JF, Siegel M. BOLD fMRI correlation reflects frequency-specific neuronal correlation. Curr Biol. (2015) 25:1368–74. doi: 10.1016/j.cub.2015.03.049
33. Hacker CD, Snyder AZ, Pahwa M, Corbetta M, Leuthardt EC. Frequency-specific electrophysiologic correlates of resting state fMRI networks. Neuroimage (2017) 149:446–57. doi: 10.1016/j.neuroimage.2017.01.054
34. Hoddes E, Zarcone V, Smythe H, Phillips R, Dement W. Quantification of sleepiness: a new approach. Psychophysiology (1973) 10:431–6. doi: 10.1111/j.1469-8986.1973.tb00801.x
35. Błaszczyk B, Czuczwar SJ. Epilepsy coexisting with depression. Pharmacol Rep. (2016) 68:1084–92. doi: 10.1016/j.pharep.2016.06.011
36. Assenza G, Pellegrino G, Tombini M, Di Pino G, Di Lazzaro V. Wakefulness delta waves increase after cortical plasticity induction. Clin Neurophysiol. (2015) 126:1221–7. doi: 10.1016/j.clinph.2014.09.029
37. Englot DJ, Hinkley LB, Kort NS, Imber BS, Mizuiri D, Honma SM, et al. Global and regional functional connectivity maps of neural oscillations in focal epilepsy. Brain (2015) 138:2249–62. doi: 10.1093/brain/awv130
38. Larsen KM, Pellegrino G, Birknow MR, Kjær TN, Baaré WFC, Didriksen M, et al. 22q11. 2 Deletion syndrome is associated with impaired auditory steady-state gamma response. Schizophr Bull. (2017) 44:388–97. doi: 10.1093/schbul/sbx058
39. Assenza G, Di Lazzaro V. A useful electroencephalography (EEG) marker of brain plasticity: delta waves. Neural Regenerat Res. (2015) 10:1216. doi: 10.4103/1673-5374.162698
40. Pellegrino G, Tomasevic L, Herz DM, Larsen KM, Siebner HR. Theta activity in the left dorsal premotor cortex during action re-evaluation and motor reprogramming. Front Hum Neurosci. (2018) 12:364. doi: 10.3389/fnhum.2018.00364
41. Pfurtscheller G, Da Silva FL. Event-related EEG/MEG synchronization and desynchronization: basic principles. Clin Neurophysiol. (1999) 110:1842–57. doi: 10.1016/S1388-2457(99)00141-8
42. Hanslmayr S, Sauseng P, Doppelmayr M, Schabus M, Klimesch W. Increasing individual upper alpha power by neurofeedback improves cognitive performance in human subjects. Appl Sychophysiol Biofeedback (2005) 30:1–10. doi: 10.1007/s10484-005-2169-8
43. Thut G, Nietzel A, Brandt SA, Pascual-Leone A. α-Band electroencephalographic activity over occipital cortex indexes visuospatial attention bias and predicts visual target detection. J Neurosci. (2006) 26:9494–502. doi: 10.1523/JNEUROSCI.0875-06.2006
44. Vecchio F, Tombini M, Buffo P, Assenza G, Pellegrino G, Benvenga A, et al. Mobile phone emission increases inter-hemispheric functional coupling of electroencephalographic alpha rhythms in epileptic patients. Int J Psychophysiol. (2012) 84:164–71. doi: 10.1016/j.ijpsycho.2012.02.002
45. da Silva FL. EEG and MEG: relevance to neuroscience. Neuron (2013) 80:1112–28. doi: 10.1016/j.neuron.2013.10.017
46. Engel AK, Fries P. Beta-band oscillations—signalling the status quo? Curr Opin Neurobiol. (2010) 20:156–65. doi: 10.1016/j.conb.2010.02.015
47. Pellegrino G, Tomasevic L, Tombini M, Assenza G, Bravi M, Sterzi S, et al. Inter-hemispheric coupling changes associate with motor improvements after robotic stroke rehabilitation. Restor Neurol Neurosci. (2012) 30:497–510. doi: 10.3233/RNN-2012-120227.
48. Moshé SL, Perucca E, Ryvlin P, Tomson T. Epilepsy: new advances. Lancet (2015) 385:884–98. doi: 10.1016/S0140-6736(14)60456-6
49. Gleichgerrcht E, Kocher M, Bonilha L. Connectomics and graph theory analyses: novel insights into network abnormalities in epilepsy. Epilepsia (2015) 56:1660–8. doi: 10.1111/epi.13133
50. Douw L, de Groot M, van Dellen E, Heimans JJ, Ronner HE, Stam CJ, et al. ‘Functional connectivity’ is a sensitive predictor of epilepsy diagnosis after the first seizure. PLoS ONE (2010) 5:e10839. doi: 10.1371/journal.pone.0010839.
51. van Dellen E, Douw L, Hillebrand A, de Witt Hamer PC, Baayen JC, Heimans JJ, et al. Epilepsy surgery outcome and functional network alterations in longitudinal MEG: a minimum spanning tree analysis. Neuroimage (2014) 86:354–63. doi: 10.1016/j.neuroimage.2013.10.010
52. Douw L, Baayen H, Bosma I, Klein M, Vandertop P, Heimans J, et al. Treatment-related changes in functional connectivity in brain tumor patients: a magnetoencephalography study. Exp Neurol. (2008) 212:285–90. doi: 10.1016/j.expneurol.2008.03.013
53. Tombini M, Pellegrino G, Pasqualetti P, Assenza G, Benvenga A, Fabrizio E, et al. Mobile phone emissions modulate brain excitability in patients with focal epilepsy. Brain Stimul. (2013) 6:448–54. doi: 10.1016/j.brs.2012.07.006.
54. Hedrich T, Pellegrino G, Kobayashi E, Lina JM, Grova C. Comparison of the spatial resolution of source imaging techniques in high-density EEG and MEG. Neuroimage. (2017) 157:531–544. doi: 10.1016/j.neuroimage.2017.06.022
55. Giambattistelli F, Tomasevic L, Pellegrino G, Porcaro C, Melgari JM, Rossini PM, et al. The spontaneous fluctuation of the excitability of a single node modulates the internodes connectivity: a TMS-EEG study. Hum Brain Mapp. (2014) 35:1740–9. doi: 10.1002/hbm.22288
Keywords: eslicarbazepine, EEG, antiepileptic drugs, epilepsy, connectivity, focal epilepsy, networks, power
Citation: Pellegrino G, Mecarelli O, Pulitano P, Tombini M, Ricci L, Lanzone J, Brienza M, Davassi C, Di Lazzaro V and Assenza G (2018) Eslicarbazepine Acetate Modulates EEG Activity and Connectivity in Focal Epilepsy. Front. Neurol. 9:1054. doi: 10.3389/fneur.2018.01054
Received: 10 July 2018; Accepted: 20 November 2018;
Published: 11 December 2018.
Edited by:
Fernando Cendes, Universidade Estadual de Campinas, BrazilReviewed by:
Eugenio Abela, King's College London, United KingdomLuiz Eduardo Betting, São Paulo State University, Brazil
Copyright © 2018 Pellegrino, Mecarelli, Pulitano, Tombini, Ricci, Lanzone, Brienza, Davassi, Di Lazzaro and Assenza. This is an open-access article distributed under the terms of the Creative Commons Attribution License (CC BY). The use, distribution or reproduction in other forums is permitted, provided the original author(s) and the copyright owner(s) are credited and that the original publication in this journal is cited, in accordance with accepted academic practice. No use, distribution or reproduction is permitted which does not comply with these terms.
*Correspondence: Giovanni Pellegrino, giovannipellegrino@gmail.com