- 1Department of Neurosciences, Biomedicine and Movement Sciences, University of Verona, Verona, Italy
- 2Department of Diagnostics and Public Health, University of Verona, Verona, Italy
- 3Service of Transfusion Medicine, AOUI, Verona, Italy
- 4U.O.C. Infectious Diseases, AOUI, Verona, Italy
- 5U.O.S. Infectious Diseases, AULSS 9 Scaligera, Verona, Italy
AIDS dementia complex (ADC) and HIV-associated neurocognitive disorders (HAND) are complications of HIV-1 infection. Viral infections are risk factors for the development of neurodegenerative disorders. Aging is associated with low-grade inflammation in the brain, i.e., the inflammaging. The molecular mechanisms linking immunosenescence, inflammaging and the pathogenesis of neurodegenerative disorders, such as Alzheimer's disease (AD) and Parkinson's disease, are largely unknown. ADC and HAND share some pathological features with AD and may offer some hints on the relationship between viral infections, neuroinflammation, and neurodegeneration. β2-microglobulin (β2m) is an important pro-aging factor that interferes with neurogenesis and worsens cognitive functions. Several studies published in the 80–90s reported high levels of β2m in the cerebrospinal fluid of patients with ADC. High levels of β2m have also been detected in AD. Inflammatory diseases in elderly people are associated with polymorphisms of the MHC-I locus encoding HLA molecules that, by associating with β2m, contribute to cellular immunity. We recently reported that HLA-C, no longer associated with β2m, is incorporated into HIV-1 virions, determining an increase in viral infectivity. We also documented the presence of HLA-C variants more or less stably linked to β2m. These observations led us to hypothesize that some variants of HLA-C, in the presence of viral infections, could determine a greater release and accumulation of β2m, which in turn, may be involved in triggering and/or sustaining neuroinflammation. ADC is the most severe form of HAND. To explore the role of HLA-C in ADC pathogenesis, we analyzed the frequency of HLA-C variants with unstable binding to β2m in a group of patients with ADC. We found a higher frequency of unstable HLA-C alleles in ADC patients, and none of them was harboring stable HLA-C alleles in homozygosis. Our data suggest that the role of HLA-C variants in ADC/HAND pathogenesis deserves further studies. If confirmed in a larger number of samples, this finding may have practical implication for a personalized medicine approach and for developing new therapies to prevent HAND. The exploration of HLA-C variants as risk factors for AD and other neurodegenerative disorders may be a promising field of study.
Introduction
Neurovirulence is detectable in patients infected with human immunodeficiency virus type 1 (HIV-1) both in the early (i.e., acute infection) and the later stages of the disease. During the acute infection, 25–50% of HIV-1 patients show neurological symptoms within 3–6 weeks after infection whereas central nervous system (CNS) complications appear, to a variable extent, during the course of HIV-1 infection (1). In early studies on HIV-1 neurovirulence, the neurological syndromes were well described, and the most severe CNS form was defined as AIDS dementia complex (ADC) (2).
The cerebral complications of HIV-1 infection, which include disturbances of cognitive, behavioral, motor and autonomic functions, still represent an issue in everyday practice (3). The old concept of ADC (2, 4), a condition not commonly encountered today in Western countries because of the wide use of combination antiretroviral treatment (cART), has been replaced by the wider term HIV-associated neurocognitive disorders (HAND) (3, 5). HAND encompasses cognitive syndromes caused by HIV-1 itself, as opposed to opportunistic infections, and includes HIV-1-associated asymptomatic neurocognitive impairment (ANI), HIV-1-associated mild neurocognitive disorder (MND), and HIV-1-associated dementia (HAD) (5), the latter conditions corresponding to ADC.
HAD represents the most severe form of the spectrum of HIV-related CNS syndromes. Risk factors for HAD were reported by several studies and they include nadir CD4 count, increasing age, substance abuse, anemia, viral co-infection and viral clade subtypes (6, 7). Before the introduction of cART, the prevalence of dementia showed an annual incidence of 7% among subjects in the later stages of infection (4). Following cART introduction, HAD cases dramatically decreased, as newly diagnosed moderate to severe dementia changed from 6.6% in 1989 to 1% in 2000 (8).
The pathogenesis of CNS damage by HIV-1 is multifactorial and mediated by direct and indirect mechanisms (9). The early detection of acute meningoencephalitis in several patients indicates a rapid involvement of the brain (10). HIV-1 enters the CNS either via infected monocytes and lymphocytes or through choroid plexus infection (11, 12). The impairment of the blood-brain barrier is associated with inflammation by cytokine-driven mechanisms. In the brain, HIV-1 mainly replicates in macrophage/microglial cells thus determining the onset of chronic local inflammation. The pathological hallmark of HIV-1 infection in the brain is represented by multinucleated giant cells, which are formed by cellular syncytia of HIV-1 infected macrophages (2, 13). In addition, HIV-1 infection of astrocytes was detected in patients with HIV-1 associated encephalopathy (14). It is noteworthy that astrocyte activation and increased glial fibrillary acidic protein expression do not represent a specific response to HIV-1 infection, but are associated with other neurological conditions, such as neurodegenerative diseases (15, 16).
The persistent HIV-1 infection in the macrophages and microglia causes the releases of the phospholipid ligand PAF, glutamate, arachidonic acid, quinolinic acid, nitric oxide, and several pro-inflammatory cytokines including IL-1β, IL-6, TNFα, and TRAIL: all these factors are involved in neural damage (12, 17–23).
Persistent CNS inflammation and chronic immune activation play an important role in the pathogenesis of neurological diseases (24) where inflammatory mediators, such as neopterin, quinolinic acid, monocyte chemoattractant protein 1 and β2-microglobulin (β2m) were found to be increased (25). Importantly, these markers are also elevated in the CSF from patients with HAD suggesting that increased immune activation is related to more severe damage.
Most likely, the complexity of the pathogenetic model is not related to a one-dimensional and direct pathogenetic event, but rather to multi-dimensional and complex immunopathological processes that are governed by viral as well as by host factors (3).
β2-Microglobulin, HLA-C Variants and HIV Infection
Several old studies reported abnormally high β2m levels in sera of HIV-1 infected patients with high p24 concentrations and a reduced number of lymphocytes (26–28). High levels of β2m were also detected in the cerebrospinal fluid (CSF) of HIV-1 infected patients with ADC (29–32). HIV-infected individuals without dementia showed a consistent correlation between β2m levels in plasma and CSF. Contrarily in patients with dementia, CSF β2m level was found to be increased independently from that in plasma β2m levels, indicating intrathecal β2m production, which was proposed to be used as a marker for HIV-1 dementia (25). This association between β2m high levels and HIV-1 infection has never been fully explained and clarified.
β2m is associated with HLA proteins (A, B, or C) and a small peptide forming the major histocompatibility complex type I (MHC-I), which plays an important role in the activation and modulation of cellular immunity. The interaction between β2m and HLA in the MHC-I complex stabilizes the structure of β2m (33).
The immune complex made by HLA-C, β2m, and peptide expressed on the cell surface tends to dissociate and to generate a pool of free-chains molecules devoid of β2m. This higher HLA-C instability, compared to HLA-A and -B, is caused by the presence of a specific amino acid sequence in the groove that binds the peptide (34), which reduces its plasticity and increases its instability (35).
The binding stability of different HLA-C variants and β2m was documented through the analysis of the relationship between free and β2m associated HLA-C molecules (35). Recently, differences in specific HLA-C domains were reported to influence the peptide binding stability (36), supporting the hypothesis of the existence of HLA-C variants with a higher or a lower binding stability to β2m.
We previously reported that HLA-C is incorporated on HIV-1 virions increasing their infectivity (37–39), that the expression of HIV-1 Env on the cell membrane promotes the formation of HLA-C molecules as free-chain, no longer bound to β2m (40) and that HLA-C variants bind more or less strongly to β2m. We showed that some HLA-C variants may be predominantly associated with β2m on the cell membrane, while other ones are predominant as free-chains, dissociated from β2m (41). In the same study, we reported that some unstable HLA-C variants, such as C*03 or C*07, are also the ones previously described to have a low expression level, while some stable variants such as C*02, C*06, C*12, or C*16, are highly transcribed and expressed (41). HLA-C expression has been associated with a different ability to control HIV-1 infection, with high HLA-C expression levels associated to a better control of HIV-1 infection, and low HLA-C expression levels associated with poor HIV-1 control and rapid progression to AIDS (42–44).
β2-Microglobulin: A Possible Pathogenetic Role in Hand and Neurodegeneration
β2m is one of the main markers of immune activation and inflammation in CSF in HAND (25, 45, 46).
In the CNS, β2m is involved in the regulation of brain development and plasticity of synapses (47–49). High levels of β2m are potentially neurotoxic, and β2m reduction has a protective effect in animal models of dementia (50). β2m has also been reported to be a pro-aging factor impairing cognitive functions (51).
β2m is responsible for “dialysis-related amyloidosis,” a clinical condition that is caused by β2m accumulation as insoluble protein aggregate (52) in joints, bones, and muscles (53). Studies on fatal hereditary systemic amyloidosis identified a natural variant of β2m which shows a dramatic decrease in thermodynamic stability and a remarkable increase in aggregation propensity (54, 55).
Of interest, high CSF levels of β2m were detected in neurodegenerative conditions, such as Alzheimer's disease (AD) and Parkinson's disease (PD) (56–59), which are caused by protein misfolding and abnormal aggregation (60), but the potential pathogenetic significance of this finding is still unclear.
Symptomatic similarities between HAD/ADC and AD have been documented (61). It has been shown that the Apo-ε4 haplotype, a known risk factor for AD, enhances the infectivity of HIV-1 (62) and that HIV-infected patients harboring the Apo-ε4 allele have excess dementia and peripheral neuropathy (63). ApoE has been found to stabilize and enhance the deposition of β2m amyloid fibrils (62, 64–67). In addition, the pathogenesis and clearance of the amyloid-β peptide (Aβ), the pathological hallmark feature of AD, may be influenced by HIV-1 infection. Enhanced amyloidosis has been reported in patients with HAND (68). Neurofibrillary tangles containing hyperphosphorylated tau protein are a pathological hallmark of AD. A significantly higher concentration of tau has been reported in brain tissues of HAD patients (68–71). The loss of neuroprotective functions of microglial cells has been suggested to contribute to the development of AD. A similar phenomenon is observed in HIV-infected patients since the virus can infect microglial cells, and loss of neuroprotection might trigger neurodegenerative process, leading to HAD (72).
After the report of patients with AD pathology and HIV-1 infection, the hypothesis that HIV-1 could create conditions ripe for AD development, and that a link between the two diseases does exist, has been considered (73). The view that ADC and AD share some pathogenetic pathways may pave the way for future studies comparing the commonalities between the two conditions.
Inflammaging, Viral Infections, and Neurodegenerative Diseases
The term “inflammaging” characterizes a widely accepted paradigm that aging is accompanied by a low-grade chronic up-regulation of pro-inflammatory responses (74). Inflammaging is supposed to interact with processing and production of Aβ and to be important in the prodromal phase of AD (75). Chronic viral infections may contribute to inflammaging. Infections caused by cytomegalovirus (CMV) (76), herpes simplex (HSV) I (77–79), human herpes virus 6 (80), varicella zoster, Epstein-Barr, influenza, arboviruses, rabies and polyoma viruses, coxsackie and other enteroviruses, echoviruses (81, 82) have all been suspected to be associated with increased risk of developing CNS and neurodegenerative diseases, such as AD (83) and PD (84). β2m is involved in several viral infections, such as CMV, HSV, coxsackieviruses, echoviruses, and others (81, 82, 85–88). CMV has the ability to remove β2m from MHC-I molecules when it binds to cells it will infect (86–88), and to express an MHC-I homolog that binds and sequesters β2m (89). A very recent study reports that cell-free particles from the respiratory syncytial virus (RSV) and HSV type 1 may catalyze amyloid aggregation in the extracellular environment, suggesting a new connection between viral infections and neurodegenerative diseases (90).
We thus hypothesized that some HLA-C variants which more easily dissociate from β2m will have a higher proportion of free heavy chains, increasing HIV-1 infectivity and promoting β2m release, which in turn may contribute to chronic inflammation and may be involved in the pathogenesis of HAND. Similar phenomena may also contribute to the pathogenesis of neurodegenerative conditions such as AD and PD, potentially triggered by other infectious agents.
Materials and Methods
Participants
With the introduction of cART for HIV-1, patients developing ADC have become rare; as a consequence, we were able to collect only a very limited number of subjects. From a database of almost 1100 HIV-infected subjects followed since 1985 by the Infectious Diseases Outpatient Clinic, Azienda Ospedaliera Universitaria Integrata Verona, we retrospectively selected patients who had an unequivocal diagnosis of ADC. Among these, only 11 were still available for blood sample collection and DNA extraction and analysis. We analyzed HLA-C variants in 11 HIV-1 infected individuals with ADC/HAD (HIV-ADC group), which was diagnosed using standardized brain imaging and neuropsychological criteria (91, 92).
This study was carried out in accordance with the recommendations of the Declaration of Helsinki. The protocol was approved by the Institutional Review Board of the University of Verona. All the patients, or their tutors in case of severe dementia, gave written informed consent to participate in the study.
The baseline characteristics of the patients (9 men, 2 women) are shown in Table 1. The patients had a median age of 50 years (interquartile range, IQR 39–58); all of them were naive to antiretroviral treatment. CD4+ T lymphocyte count and HIV RNA load were determined at ADC diagnosis. The median CD4+ T lymphocytes nadir was 50 cells/mm3 (IQR 26-157), while the median plasmatic zenith HIV-RNA viral load was 71350 copies/mL (IQR 22300-141000). A lumbar puncture was performed in 9 patients at the time when ADC was diagnosed, with a median CSF HIV-RNA viral load of 204000 copies/mL (IQR 70085-511500). The diagnosis of ADC was made using, in addition to CSF HIV-RNA viral load, brain MRI, mini-mental state examination (MMSE), and full neuropsychological evaluation. The most common findings observed on brain MRI were symmetric periventricular and deep white matter T2 hyperintensity and widespread cerebral atrophy. MMSE was <25 in all ADC patients. A full neuropsychological evaluation was performed in 4 patients and showed mild-to-moderate dementia. The severity of dementia in the remaining 7 patients made full neuropsychological testing difficult.
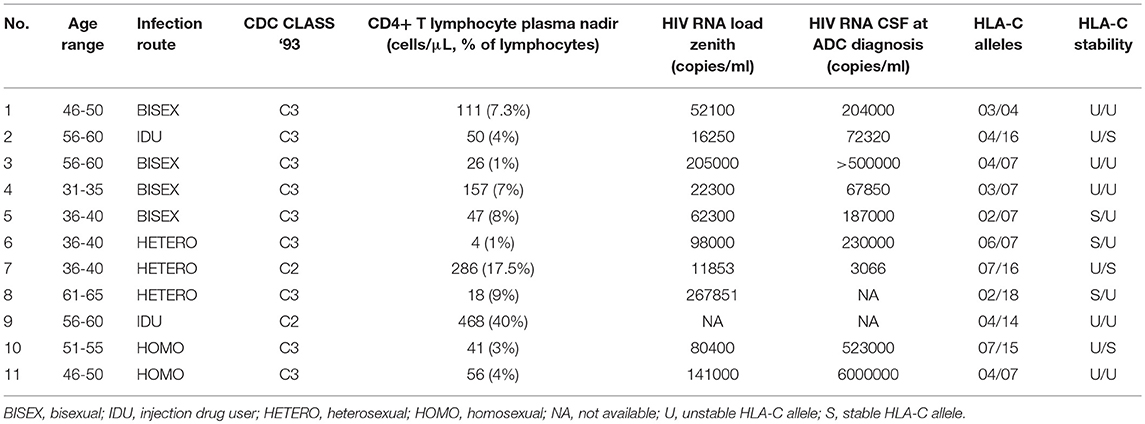
Table 1. Baseline characteristics of HIV-1 infected patients with AIDS dementia complex (HIV-ADC group).
We collected a control group of 16 HIV-infected patients (14 men, 2 women; median age: 46.5 years, IQR 35.5-51) with no neurocognitive disorders and MMSE ≥ 25 (HIV-no-ADC group), whose baseline characteristics are reported in Table 2. Gender and age did not significantly differ between patients and control groups.
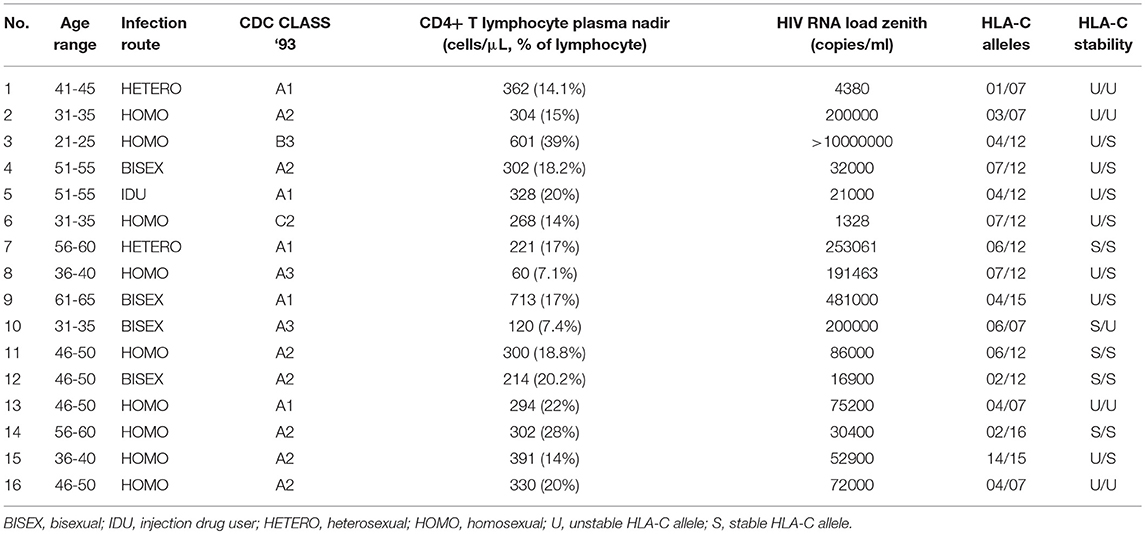
Table 2. Baseline characteristics of the control group of HIV-infected patients without AIDS dementia complex (HIV-no-ADC group).
Patients were classified according to the CDC 1993 classification system, whereby HIV infection was divided into three clinical categories (i.e., A, B and C). Category A included the patients with asymptomatic acute (primary) infection or persistent generalized lymphadenopathy. Category C included the patients with AIDS-indicator conditions. The symptomatic patients, who could not be classified in categories A or C, were included in B one. HIV positive patients were further sub-grouped according to the absolute lymphocyte T CD4+ count (i.e., 1: >500 cells/μL; 2: 200–499 cells/μL; 3: <200 cells/μL) (93). According to the CDC 1993 classification, HIV-related neurological complications were described as HIV-related encephalopathy and associated to category C. Hence, HIV positive patients with ADC were classified in category C, as was the case for our 11 HIV-ADC patients (Table 1).
HLA-C Typing
HLA-C alleles were classified as unstable (HLA-C*01, *03, *04, *07, *14, *17, *18) or stable (C*02, *05, *06, *08, *12, *15, *16) according to previous phylogenetic, structural and densitometry findings (35, 36, 41, 94).
HLA-C typing was carried out at the HLA laboratory of tissue typing, Azienda Ospedaliera Universitaria Integrata (AOUI) of Verona. The laboratory is accredited by the European Federation of Immunogenetics (EFI) since 1999.
DNA was prepared from whole blood using the QIAamp DNA Blood Kit (Qiagen) and HLA-C phototyping was performed by PCR-SSP using the commercial kit “HLA-C SSP kit” BioRad, followed by gel electrophoresis for the detection of positive bands and the interpretation of the data (95).
Data Analysis
We compared HLA-C alleles (stable/unstable) and genotypes distribution between the ADC group and the population of northern Italy, low-resolution typing (96). Guerini and coworkers (2008) described HLA-C allele frequencies in a large Italian population cohort showing a consistent picture of their distribution in Italy. These data can be currently used as reference population for clinical and anthropological studies. Similarly, we compared the HIV-no-ADC group with the same reference population. Finally, we compared the two HIV-infected groups, i.e., HIV-ADC vs. HIV-no-ADC.
The significance of the association between groups of categorical data (stable and unstable alleles) was examined using 2 × 2 contingency tables and the Fisher's exact test, while quantitative data were examined using the Mann Whitney U test. The Fisher's exact test is conservative but guarantees type I error control for small sample sizes.
Genetic associations were conducted using a 2 × 2 contingency table of 2N case-control by allele counts, where N is the number of individuals tested. The strength of association was reported as Odds Ratio (OR) with a 95% confidence interval (CI).
When the statistical significance was tested under the hypothesis of a specific direction of association (i.e., unstable alleles are positively associated with ADC), a one-tailed test was employed. A two-tailed test was instead used when the association was explored without any expectation about the direction of the association between the tested variables. An association was deemed to be statistically significant by setting a significant level of 5%, that is all tests showing a nominal p-value <0.05 were considered to be statistically significant. Given the exploratory nature of this study, no correction for multiple testing was employed.
Results
All 11 HIV-ADC subjects carried unstable HLA-C alleles in homozygosis (U/U, n = 5) or in heterozygosis (U/S, n = 6), but none of them was carrying stable alleles in homozygosis (S/S; Table 1).
Among the 16 HIV-no-ADC subjects, four carried unstable HLA-C alleles in homozygosis (U/U), 4 stable alleles in homozygosis (S/S) and eight were heterozygotes (U/S; Table 2).
The HLA-C stable/unstable allele distribution was different between the HIV-ADC group (stable 27%, unstable 73%) and the northern Italy population [stable 50%, unstable 50%; Fisher exact test, p = 0.029; OR 2.630 [CI: 1.112-∞]; Table 3].
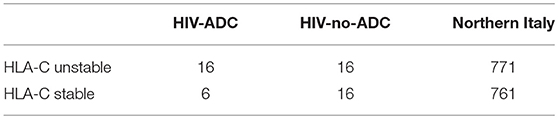
Table 3. HLA-C alleles distribution (stable, unstable) in HIV-infected patients with ADC (HIV-ADC), HIV-infected patients without ADC (HIV-no-ADC), and in northern Italy (96).
No differences in alleles distribution were observed when the HIV-no-ADC group (stable 50%, unstable 50%) was compared with the northern Italy population [Fisher exact test, p = 0.584; OR 0.098 [CI: 0.514-∞]; Table 3].
We also compared the HIV-ADC group with the HIV-no-ADC group, showing a slight difference in stable/unstable HLA-C alleles distribution [Fisher's exact test, p = 0.082; OR 2.618 [CI: 0.870-∞]; Table 3]. The two groups differed neither for sex (Fisher's exact test, p = 1.000) nor for age (Mann Whitney U test, p = 0.312).
The two HIV-infected groups did not differ for HIV RNA zenith level (Mann Whitney U test, p = 0.896), while they were different for CD4+ T lymphocyte nadir (Mann Whitney U test, p = 0.001). The lower CD4 count in HIV-infected patients with ADC is the consequence of the longer duration of HIV-1 infection with worsening immunodeficiency, which was frequent in the pre-cART times.
Finally, we analyzed the distribution of the different HLA-C alleles between the 3 groups.
We found interesting differences on HLA-C subtypes, in that HLA-C*12 appeared to be absent in the HIV-ADC group (0%) when compared either with the reference northern Italy population (17%, p = 0.038) and with the HIV-no-ADC group (25%, p = 0.016; Table 4). No difference was observed for the distribution of HLA-C*12 between the HIV-no-ADC group and the northern Italy population (p = 0.237; Table 4).
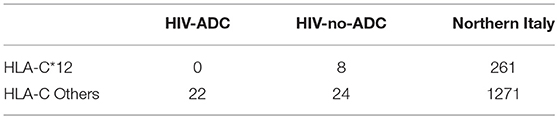
Table 4. HLA-C allele 12 distribution in HIV-infected patients with ADC (HIV-ADC), HIV-infected patients without ADC (HIV-no-ADC), and in northern Italy (96).
Discussion
Our results, despite being very preliminary, and derived from a small sample of patients, may have some implications for better understanding HAND/ADC pathogenesis and eventually improve the treatment of this condition.
ADC was common in patients with HIV-1 infection during disease progression in the pre-cART era (97). The introduction of cART regimens tackled the onset of ADC in these patients and reduced the likelihood and severity of HAND, indicating that the treatment of HIV-1 infection is essential to reduce neurological damage (98). At variance, the prevalence of less severe HAND syndromes is 46% of HIV-1-infected people and is expected to increase in the next years (99). Why HAND prevalence did not significantly decrease after the introduction of cART (100) is poorly understood (3).
To answer this question, we hypothesized that the development of HAND could be associated with the expression of specific unstable variants of HLA-C and the subsequent CNS release and deposition of free β2m, which has been reported to be neurotoxic and to deteriorate cognitive functions (50, 51). To this aim, from a large database of nearly 1100 HIV-infected subjects, we retrospectively selected patients who had an unequivocal diagnosis of ADC, the most severe form of HAND. Only 11 among them were still available for sample collection, DNA extraction, and analysis.
Our findings showed a higher frequency of unstable HLA-C alleles in HIV-1 infected patients who developed ADC in comparison to northern Italy general population. This finding argues in favor of a possible involvement of β2m in triggering and/or sustaining the development of neurologic complications of AIDS. In contrast, the HIV-infected population without ADC showed the same distribution than the general population of northern Italy, thus excluding the hypothesis that the higher frequency of unstable HLA-C alleles is associated with a higher susceptibility to HIV-1 infection. The present data raise the question whether patients expressing unstable HLA-C alleles in the control group did not develop ADC. We speculate that unstable alleles might predispose to ADC pathogenesis, but other viral and host factors (e.g., time of infection, HIV subtypes, immunological response) could contribute to the development of HAND/ADC. Moreover, the degree of stability vary across different alleles, and exploring single HLA-C variants may help better stratify the risk of ADC/HAND.
We observed an unexpected lower frequency of HLA-C*12, a stable HLA-C variant, in HIV-ADC subjects. Recent studies reported a protective role of HLA-C*12 in HIV-1 infection (101, 102). It would be tempting to speculate that the presence of HLA-C*12 might have some kind of protective effect on the risk of developing ADC in HIV-infected individuals. A study on a higher number of cases, allowing the analysis of the effect of single HLA-C alleles on the risk of developing neurological diseases in HIV-1 patients is definitely needed, to confirm this very preliminary but also very tempting observation.
HIV-1 neurovirulence could contribute to the dissociation of β2m from HLA-C molecules, as a consequence of the association of HIV-1 Env with unstable HLA-C variants (40). A three amino acid position in HIV-1 gp120 glycoprotein was recently reported to be associated with HAND (99).
The treatment of HAND is still is an issue in the era of cART (103, 104), as the improvement of neurocognitive symptoms to cART is variable (105), mild neurocognitive impairment is found in some patients, and factors predicting a response in single patients are unknown. Indeed, several studies demonstrated that cART is not effective on HAND in all HIV-1 positive patients (106, 107).
It would be reasonable to use antiretroviral drugs with high CNS penetration/effectiveness score, such as darunavir, abacavir or raltegravir (108, 109), to reduce the risk of HAND. However, some antiretroviral drugs are potentially neurotoxic: they may increase the production of Aβ protein by neurons and reduce its microglial phagocytosis, leading to the deposition of amyloid plaques in the CNS (68, 110). If our hypothesis is confirmed by further studies, an antiretroviral therapy with CNS-targeted drugs might be considered for patients with unstable HLA-C variants, paying attention to the possible side effects of some of them.
Effective cART suppresses HIV-1 replication and increases the immune system activity, thus determining a beneficial effect on the CNS through the reduction of HIV viral load, viral neurotoxic proteins production, and neuroinflammation. However, neuroinflammation may still be active as microglial activation in specific brain areas, including the hippocampus and temporal cortex, whereas in pre-cART era these changes were more common in the basal ganglia (111). Chronic inflammation and other immune mechanisms triggered by viral infection, rather than direct HIV-1 involvement, may thus play a key role in HAND pathogenesis.
ADC is rare nowadays due to the cART, and, despite our efforts, we were not able to recruit a larger ADC sample. We are fully aware that our data are preliminary and should be considered with caution. However, they are appealing and may pave the way for recruiting a larger population of patients through a multicenter study. If confirmed in larger populations of patients, these findings would suggest that HIV-infected patients carrying specific unstable variants of HLA-C should undergo strict monitoring of neuropsychological and neurological symptoms to promptly recognize early HAND stages. Moreover, CSF β2m levels might be explored as a molecular biomarker to recognize early HAND stages, given the absence of specific tests for this condition (112).
The observation we reported may be a starting point to explore whether some HLA-C variants are associated with higher risk of CNS viral infection, neuroinflammation and neurodegenerative disorders (113, 114). HLA-C*07, which is one of the most unstable variants (36), was found to be associated with AD (115). Similarly, very old studies associated HLA-C*03, another unstable HLA-C variant, to higher AD risk (116–118).
There are many features in common between HAND/ADC and AD, including clinical features that overlap between the two conditions (61). Aβ peptide biogenesis and clearance may be influenced by HIV-1 infection (119). Increased Aβ amyloid has been reported in up to 72% of HIV-1 patients with HAND (68), but also in 38% of those without HIV-1 but no cognitive symptoms (73, 119). We may speculate that shared pathogenetic mechanisms in response to neurotropic viral infections (e.g., herpesviridae) may contribute to Aβ amyloid deposition in AD patients without HIV-1 and that neuroinflammation due to β2m release in patients with unstable HLA-C alleles may accelerate this phenomenon.
The large number of HIV-infected patients under cART with a long-life expectancy due to a nearly complete control of viral load raises concern about their risk of cognitive dysfunction in the presence of cardiovascular risk factors (e.g., smoking, hypertension, diabetes, hypercholesterolemia) (120) that are known to increase also the risk of AD (121). The presence of unstable HLA-C variants might be explored as a possible predictor for worse cognitive performance at older age in HIV-infected patients with cardiovascular risk factors.
Conclusions
Our very preliminary data suggests that HIV-1 infected individuals carrying unstable HLA-C allelic variants may be at higher risk of CNS complications. Specifically, we observed that alleles encoding unstable HLA-C variants were more frequent in HIV-1 infected patients who developed ADC, the most severe HAND subtype. The identification of specific variants of HLA-C as risk factors for HAND can provide a contribution both in the field of personalized medicine and for the development of new therapies, aimed at preventing and/or reducing neurocognitive damage in AIDS patients.
Given the potential commonalities between HIV-induced aging, neurocognitive decline and AD that represent a promising future hot research topic (73), our findings might be helpful for better understanding the pathogenesis, and for identifying disease-modifying therapeutic strategies to be offered to patients at higher risk of neurodegenerative disorders at presymptomatic disease stages.
Author Contributions
DZ conceived, designed supervised, and coordinated the study, conducted data analysis and took care of drafting and writing the manuscript. MS, SM, FP, and ED were involved in samples collection, extraction, and analysis. EG and VM conducted HLA-C typing. SR, EL, MM, and ML contributed to patients selection, clinical data collection, and manuscript writing. GM conducted genetic and statistical analysis. MGR helped to coordinate the study and assisting in manuscript preparation. ST and DG contributed to planning the study, to the analysis and collection of neurologic and virologic data and to manuscript drafting and writing.
Funding
This research received no specific grant from any funding agency in the public, commercial, or not-for-profit sector. Since the cancelation of the AIDS National program, it has been very difficult to pursue basic research in the field of HIV/AIDS in Italy. As a consequence, this study was conducted with leftover nonperishable materials and reagents from previous grants (Europrise Network of Excellence, Life Science Programme, European Commission), and limited funding support from the Department of Neurosciences, Biomedicine and Movement Sciences, University of Verona, through the FUR 2016.
Conflict of Interest Statement
The authors declare that the research was conducted in the absence of any commercial or financial relationships that could be construed as a potential conflict of interest.
Acknowledgments
SM and FP participated in this study as the fulfillment of their Ph.D. degree. We thank Dr. G. Gandini, Service of Transfusion Medicine AOUI Verona, and Prof. M. Tinazzi, University of Verona, for supporting and encouraging this study. We thank Dr. A. Beretta for the stimulating discussions on the topic of the interaction between HLA-C, HIV-1 infection, and neurological diseases.
References
1. Nathanson N, Cook DG, Kolson DL, Gonzalez-Scarano F. Pathogenesis of HIV encephalopathy. Ann N Y Acad Sci. (1994) 724:87–106. doi: 10.1111/j.1749-6632.1994.tb38898.x
2. Navia BA, Jordan BD, Price RW. The AIDS dementia complex: I. Clini Featur Ann Neurol. (1986) 19:517–24. doi: 10.1002/ana.410190602
3. Eggers C, Arendt G, Hahn K, Husstedt IW, Maschke M, Neuen-Jacob E, et al. HIV-1-associated neurocognitive disorder: epidemiology, pathogenesis, diagnosis, and treatment. J Neurol. (2017) 264:1715–27. doi: 10.1007/s00415-017-8503-2
4. Mcarthur JC, Hoover DR, Bacellar H, Miller EN, Cohen BA, Becker JT, et al. Dementia in AIDS patients: incidence and risk factors. Multicenter AIDS Cohort Study. Neurology (1993) 43:2245–52. doi: 10.1212/WNL.43.11.2245
5. Antinori A, Arendt G, Becker JT, Brew BJ, Byrd DA, Cherner M, et al. Updated research nosology for HIV-associated neurocognitive disorders. Neurology (2007) 69:1789–99. doi: 10.1212/01.WNL.0000287431.88658.8b
6. Valcour V, Yee P, Williams AE, Shiramizu B, Watters M, Selnes O, et al. Lowest ever CD4 lymphocyte count (CD4 nadir) as a predictor of current cognitive and neurological status in human immunodeficiency virus type 1 infection–The Hawaii Aging with HIV Cohort. J Neurovirol. (2006) 12:387–91. doi: 10.1080/13550280600915339
7. Jayadev S, Garden GA. Host and viral factors influencing the pathogenesis of HIV-associated neurocognitive disorders. J Neuroimmune Pharmacol. (2009) 4:175–89. doi: 10.1007/s11481-009-9154-6
8. Mcarthur JC. HIV dementia: an evolving disease. J Neuroimmunol. (2004) 157:3–10. doi: 10.1016/j.jneuroim.2004.08.042
9. Nath A. Eradication of human immunodeficiency virus from brain reservoirs. J Neurovirol. (2015) 21:227–34. doi: 10.1007/s13365-014-0291-1
10. Gendelman HE, Lipton SA, Tardieu M, Bukrinsky MI, Nottet HS. The neuropathogenesis of HIV-1 infection. J Leukoc Biol. (1994) 56:389–98. doi: 10.1002/jlb.56.3.389
11. Fujimura RK, Bockstahler LE, Goodkin K, Werner T, Brack-Werner R, Shapshak P. Neuropathology and virology of hiv associated dementia. Rev Med Virol. (1996) 6, 141–50. doi: 10.1002/(SICI)1099-1654(199609)6:3<141::AID-RMV141>3.0.CO;2-1
12. Kaul M, Zheng J, Okamoto S, Gendelman HE, Lipton SA. HIV-1 infection and AIDS: consequences for the central nervous system. Cell Death Differ. (2005) 12(Suppl. 1):878–92. doi: 10.1038/sj.cdd.4401623
13. Mattson MP, Haughey NJ, Nath A. Cell death in HIV dementia. Cell Death Differ. (2005) 12 (Suppl. 1):893–904. doi: 10.1038/sj.cdd.4401577
14. Ranki A, Nyberg M, Ovod V, Haltia M, Elovaara I, Raininko R, et al. Abundant expression of HIV Nef and Rev proteins in brain astrocytes in vivo is associated with dementia. AIDS (1995) 9:1001–8. doi: 10.1097/00002030-199509000-00004
15. Zhou BY, Liu Y, Kim B, Xiao Y, He JJ. Astrocyte activation and dysfunction and neuron death by HIV-1 Tat expression in astrocytes. Mol Cell Neurosci. (2004) 27:296–305. doi: 10.1016/j.mcn.2004.07.003
16. Vanzani MC, Iacono RF, Caccuri RL, Troncoso AR, Berria MI. Regional differences in astrocyte activation in HIV-associated dementia. Medicina (2006) 66:108–12.
17. Brenneman DE, Westbrook GL, Fitzgerald SP, Ennist DL, Elkins KL, Ruff MR, et al. Neuronal cell killing by the envelope protein of HIV and its prevention by vasoactive intestinal peptide. Nature (1988) 335:639–42. doi: 10.1038/335639a0
18. Kaiser PK, Offermann JT, Lipton SA. Neuronal injury due to HIV-1 envelope protein is blocked by anti-gp120 antibodies but not by anti-CD4 antibodies. Neurology (1990) 40:1757–61. doi: 10.1212/WNL.40.11.1757
19. Gelbard HA, Dzenko KA, Diloreto D, Del Cerro C, Del Cerro M, Epstein LG. Neurotoxic effects of tumor necrosis factor alpha in primary human neuronal cultures are mediated by activation of the glutamate AMPA receptor subtype: implications for AIDS neuropathogenesis. Dev Neurosci. (1993) 15:417–22. doi: 10.1159/000111367
20. Nottet HS, Jett M, Flanagan CR, Zhai QH, Persidsky Y, Rizzino A, et al. A regulatory role for astrocytes in HIV-1 encephalitis. An overexpression of eicosanoids, platelet-activating factor, and tumor necrosis factor-alpha by activated HIV-1-infected monocytes is attenuated by primary human astrocytes. J Immunol. (1995) 154:3567–3581.
21. Adamson DC, Wildemann B, Sasaki M, Glass JD, Mcarthur JC, Christov VI, et al. Immunologic NO synthase: elevation in severe AIDS dementia and induction by HIV-1 gp41. Science (1996) 274:1917–21. doi: 10.1126/science.274.5294.1917
22. Kerr SJ, Armati PJ, Pemberton LA, Smythe G, Tattam B, Brew BJ. Kynurenine pathway inhibition reduces neurotoxicity of HIV-1-infected macrophages. Neurology (1997) 49:1671–81. doi: 10.1212/WNL.49.6.1671
23. Miura Y, Misawa N, Kawano Y, Okada H, Inagaki Y, Yamamoto N, et al. Tumor necrosis factor-related apoptosis-inducing ligand induces neuronal death in a murine model of HIV central nervous system infection. Proc Natl Acad Sci USA. (2003) 100:2777–82. doi: 10.1073/pnas,.2628048100
24. Manji H, Jager HR, Winston A. HIV, dementia and antiretroviral drugs: 30 years of an epidemic. J Neurol Neurosurg Psychiatr. (2013) 84:1126–37. doi: 10.1136/jnnp-2012-304022
25. Enting RH, Foudraine NA, Lange JM, Jurriaans S, Van Der Poll T, Weverling GJ, et al. Cerebrospinal fluid beta2-microglobulin, monocyte chemotactic protein-1, and soluble tumour necrosis factor alpha receptors before and after treatment with lamivudine plus zidovudine or stavudine. J Neuroimmunol. (2000) 102:216–21. doi: 10.1016/S0165-5728(99)00219-2
26. Mulder JW, Krijnen P, Coutinho RA, Bakker M, Goudsmit J, Lange JM. Serum beta 2-microglobulin levels in asymptomatic HIV-1-infected subjects during long-term zidovudine treatment. Genitourin Med. (1991) 67:188–93. doi: 10.1136/sti.67.3.188
27. Alfieri R, Chirianni A, Mancino T, Remondelli P, Russo P, Liuzzi G, et al. Serum beta 2-microglobulin levels and p24 antigen, lymphocyte depletion and disease progression in human immunodeficiency virus infection. Int J Clin Lab Res. (1992) 22:48–51. doi: 10.1007/BF02591394
29. Bhalla RB, Safai B, Pahwa S, Schwartz MK. Beta 2-microglobulin as a prognostic marker for development of AIDS. Clin Chem. (1985) 31:1411–2.
30. Brew BJ, Bhalla RB, Fleisher M, Paul M, Khan A, Schwartz MK, et al. Cerebrospinal fluid beta 2 microglobulin in patients infected with human immunodeficiency virus. Neurology (1989) 39:830–4. doi: 10.1212/WNL.39.6.830
31. Sonnerborg AB, Von Stedingk LV, Hansson LO, Strannegard OO. Elevated neopterin and beta 2-microglobulin levels in blood and cerebrospinal fluid occur early in HIV-1 infection. AIDS (1989) 3:277–83. doi: 10.1097/00002030-198905000-00005
32. Mcarthur JC, Nance-Sproson TE, Griffin DE, Hoover D, Selnes OA, Miller EN, et al. The diagnostic utility of elevation in cerebrospinal fluid beta 2-microglobulin in HIV-1 dementia. Multicenter AIDS cohort study. Neurology (1992) 42:1707–12. doi: 10.1212/WNL.42.9.1707
33. Halabelian L, Ricagno S, Giorgetti S, Santambrogio C, Barbiroli A, Pellegrino S, et al. Class I major histocompatibility complex, the trojan horse for secretion of amyloidogenic beta2-microglobulin. J Biol Chem. (2014) 289:3318–27. doi: 10.1074/jbc.M113.524157
34. Zemmour J, Parham P. Distinctive polymorphism at the HLA-C locus: implications for the expression of HLA-C. J Exp Med. (1992) 176:937–50. doi: 10.1084/jem.176.4.937
35. Sibilio L, Martayan A, Setini A, Lo Monaco E, Tremante E, Butler RH, et al. A single bottleneck in HLA-C assembly. J Biol Chem. (2008) 283:1267–74. doi: 10.1074/jbc.M708068200
36. Kaur G, Gras S, Mobbs JI, Vivian JP, Cortes A, Barber T, et al. Structural and regulatory diversity shape HLA-C protein expression levels. Nat Commun. (2017) 8:15924. doi: 10.1038/ncomms15924
37. Matucci A, Rossolillo P, Baroni M, Siccardi AG, Beretta A, Zipeto D. HLA-C increases HIV-1 infectivity and is associated with gp120. Retrovirology (2008) 5:68. doi: 10.1186/1742-4690-5-68
38. Baroni M, Matucci A, Scarlatti G, Soprana E, Rossolillo P, Lopalco L, et al. HLA-C is necessary for optimal human immunodeficiency virus type 1 infection of human peripheral blood CD4 lymphocytes. J Gen Virol. (2010) 91:235–41. doi: 10.1099/vir.0.015230-0
39. Zipeto D, Beretta A. HLA-C and HIV-1: Friends or Foes? Retrovirology (2012) 9:39. doi: 10.1186/1742-4690-9-39
40. Serena M, Parolini F, Biswas P, Sironi F, Blanco Miranda A, Zoratti E, et al. HIV-1 Env associates with HLA-C free-chains at the cell membrane modulating viral infectivity. Sci Rep. (2017) 7:40037. doi: 10.1038/srep40037
41. Parolini F, Biswas P, Serena M, Sironi F, Muraro V, Guizzardi E, et al. Stability and expression levels of HLA-C on the cell membrane modulate HIV-1 infectivity. J Virol. (2018) 92:e01711–01717. doi: 10.1128/JVI.01711-17
42. Kulkarni S, Savan R, Qi Y, Gao X, Yuki Y, Bass SE, et al. Differential microRNA regulation of HLA-C expression and its association with HIV control. Nature (2011) 472:495–8. doi: 10.1038/nature09914
43. Apps R, Qi Y, Carlson JM, Chen H, Gao X, Thomas R, et al. Influence of HLA-C expression level on HIV control. Science (2013) 340:87–91. doi: 10.1126/science.1232685
44. Kulkarni S, Qi Y, O'huigin C, Pereyra F, Ramsuran V, Mclaren P, et al. Genetic interplay between HLA-C and MIR148A in HIV control and Crohn disease. Proc Natl Acad Sci USA. (2013) 110:20705–10. doi: 10.1073/pnas.1312237110
45. Brew BJ, Bhalla RB, Paul M, Sidtis JJ, Keilp JJ, Sadler AE, et al. Cerebrospinal fluid beta 2-microglobulin in patients with AIDS dementia complex: an expanded series including response to zidovudine treatment. AIDS (1992) 6:461–5. doi: 10.1097/00002030-199205000-00004
46. Brew BJ, Dunbar N, Pemberton L, Kaldor J. Predictive markers of AIDS dementia complex: CD4 cell count and cerebrospinal fluid concentrations of beta 2-microglobulin and neopterin. J Infect Dis. (1996) 174:294–8. doi: 10.1093/infdis/174.2.294
47. Huh GS, Boulanger LM, Du H, Riquelme PA, Brotz TM, Shatz CJ. Functional requirement for class I MHC in CNS development and plasticity. Science (2000) 290:2155–9. doi: 10.1126/science.290.5499.2155
48. Shatz CJ. MHC class I: an unexpected role in neuronal plasticity. Neuron (2009) 64:40–5. doi: 10.1016/j.neuron.2009.09.044
49. Lee H, Brott BK, Kirkby LA, Adelson JD, Cheng S, Feller MB, et al. Synapse elimination and learning rules co-regulated by MHC class I H2-Db. Nature (2014) 509:195–200. doi: 10.1038/nature13154
50. Giorgetti S, Raimondi S, Cassinelli S, Bucciantini M, Stefani M, Gregorini G, et al. Beta2-Microglobulin is potentially neurotoxic, but the blood brain barrier is likely to protect the brain from its toxicity. Nephrol Dial Transplant. (2009) 24:1176–81. doi: 10.1093/ndt/gfn623
51. Smith LK, He Y, Park JS, Bieri G, Snethlage CE, Lin K, et al. Beta2-microglobulin is a systemic pro-aging factor that impairs cognitive function and neurogenesis. Nat Med. (2015) 21:932–7. doi: 10.1038/nm.3898
52. Natalello A, Relini A, Penco A, Halabelian L, Bolognesi M, Doglia SM, et al. Wild type beta-2 microglobulin and DE loop mutants display a common fibrillar architecture. PLoS ONE (2015) 10:e0122449. doi: 10.1371/journal.pone.0122449
53. Gejyo F, Yamada T, Odani S, Nakagawa Y, Arakawa M, Kunitomo T, et al. A new form of amyloid protein associated with chronic hemodialysis was identified as beta 2-microglobulin. Biochem Biophys Res Commun. (1985) 129:701–6. doi: 10.1016/0006-291X(85)91948-5
54. Mangione PP, Esposito G, Relini A, Raimondi S, Porcari R, Giorgetti S, et al. Structure, folding dynamics, and amyloidogenesis of D76N beta2-microglobulin: roles of shear flow, hydrophobic surfaces, and alpha-crystallin. J Biol Chem. (2013) 288:30917–30. doi: 10.1074/jbc.M113.498857
55. Le Marchand T, De Rosa M, Salvi N, Sala BM, Andreas LB, Barbet-Massin E, et al. Conformational dynamics in crystals reveal the molecular bases for D76N beta-2 microglobulin aggregation propensity. Nat Commun. (2018) 9:1658. doi: 10.1038/s41467-018-04078-y
56. Carrette O, Demalte I, Scherl A, Yalkinoglu O, Corthals G, Burkhard P, et al. A panel of cerebrospinal fluid potential biomarkers for the diagnosis of Alzheimer's disease. Proteomics (2003) 3:1486–94. doi: 10.1002/pmic.200300470
57. Hunot S, Hirsch EC. Neuroinflammatory processes in Parkinson's disease. Ann Neurol. (2003) 53(Suppl. 3):S49–58. discussion: S58–60. doi: 10.1002/ana.10481
58. Zhang J, Goodlett DR, Montine TJ. Proteomic biomarker discovery in cerebrospinal fluid for neurodegenerative diseases. J Alzheimers Dis. (2005) 8:377–86. doi: 10.3233/JAD-2005-8407
59. Svatonova J, Borecka K, Adam P, Lanska V. Beta2-microglobulin as a diagnostic marker in cerebrospinal fluid: a follow-up study. Dis Mark. (2014) 2014:495402. doi: 10.1155/2014/495402
60. Merlini G, Bellotti V. Molecular mechanisms of amyloidosis. N Engl J Med. (2003) 349:583–96. doi: 10.1056/NEJMra023144
61. Kuhlmann I, Minihane AM, Huebbe P, Nebel A, Rimbach G. Apolipoprotein E genotype and hepatitis C, HIV and herpes simplex disease risk: a literature review. Lipids Health Dis. (2010) 9:8. doi: 10.1186/1476-511X-9-8
62. Burt TD, Agan BK, Marconi VC, He W, Kulkarni H, Mold JE, et al. Apolipoprotein (apo) E4 enhances HIV-1 cell entry in vitro, and the APOE epsilon4/epsilon4 genotype accelerates HIV disease progression. Proc Natl Acad Sci USA. (2008) 105:8718–23. doi: 10.1073/pnas.0803526105
63. Corder EH, Robertson K, Lannfelt L, Bogdanovic N, Eggertsen G, Wilkins J, et al. HIV-infected subjects with the E4 allele for APOE have excess dementia and peripheral neuropathy. Nat Med. (1998) 4:1182–4. doi: 10.1038/2677
64. Yamaguchi I, Hasegawa K, Takahashi N, Gejyo F, Naiki H. Apolipoprotein E inhibits the depolymerization of beta 2-microglobulin-related amyloid fibrils at a neutral pH. Biochemistry (2001) 40:8499–507. doi: 10.1021/bi0027128
65. Naiki H, Yamamoto S, Hasegawa K, Yamaguchi I, Goto Y, Gejyo F. Molecular interactions in the formation and deposition of beta2-microglobulin-related amyloid fibrils. Amyloid (2005) 12:15–25. doi: 10.1080/13506120500032352
66. Yamamoto S, Hasegawa K, Yamaguchi I, Goto Y, Gejyo F, Naiki H. Kinetic analysis of the polymerization and depolymerization of beta(2)-microglobulin-related amyloid fibrils in vitro. Biochim Biophys Acta (2005) 1753:34–43. doi: 10.1016/j.bbapap.2005.07.007
67. Myers SL, Jones S, Jahn TR, Morten IJ, Tennent GA, Hewitt EW, et al. A systematic study of the effect of physiological factors on beta2-microglobulin amyloid formation at neutral pH. Biochemistry (2006) 45:2311–21. doi: 10.1021/bi052434i
68. Xu J, Ikezu T. The comorbidity of HIV-associated neurocognitive disorders and Alzheimer's disease: a foreseeable medical challenge in post-HAART era. J Neuroimmune Pharmacol. (2009) 4:200–12. doi: 10.1007/s11481-008-9136-0
69. Andersson L, Blennow K, Fuchs D, Svennerholm B, Gisslen M. Increased cerebrospinal fluid protein tau concentration in neuro-AIDS. J Neurol Sci. (1999) 171:92–6. doi: 10.1016/S0022-510X(99)00253-1
70. Brew BJ, Pemberton L, Blennow K, Wallin A, Hagberg L. CSF amyloid beta42 and tau levels correlate with AIDS dementia complex. Neurology (2005) 65:1490–2. doi: 10.1212/01.wnl.0000183293.95787.b7
71. Anthony IC, Ramage SN, Carnie FW, Simmonds P, Bell JE. Accelerated Tau deposition in the brains of individuals infected with human immunodeficiency virus-1 before and after the advent of highly active anti-retroviral therapy. Acta Neuropathol. (2006) 111:529–38. doi: 10.1007/s00401-006-0037-0
72. Streit WJ, Xue QS. Alzheimer's disease, neuroprotection, and CNS immunosenescence. Front Pharmacol. (2012) 3:138. doi: 10.3389/fphar.2012.00138
73. Chakradhar S. A tale of two diseases: aging HIV patients inspire a closer look at Alzheimer's disease. Nat Med. (2018) 24:376–7. doi: 10.1038/nm0418-376
74. Franceschi C, Campisi J. Chronic inflammation (inflammaging) and its potential contribution to age-associated diseases. J Gerontol A Biol Sci Med Sci. (2014) 69(Suppl. 1):S4–9. doi: 10.1093/gerona/glu057
75. Giunta B, Fernandez F, Nikolic WV, Obregon D, Rrapo E, Town T, et al. Inflammaging as a prodrome to Alzheimer's disease. J Neuroinflammation (2008) 5:51. doi: 10.1186/1742-2094-5-51
76. Barnes LL, Capuano AW, Aiello AE, Turner AD, Yolken RH, Torrey EF, et al. Cytomegalovirus infection and risk of Alzheimer disease in older black and white individuals. J Infect Dis. (2015) 211:230–7. doi: 10.1093/infdis/jiu437
77. Itzhaki RF, Cosby SL, Wozniak MA. Herpes simplex virus type 1 and Alzheimer's disease: the autophagy connection. J Neurovirol. (2008) 14:1–4. doi: 10.1080/13550280701802543
78. Itzhaki RF, Wozniak MA. Herpes simplex virus type 1 in Alzheimer's disease: the enemy within. J Alzheimers Dis. (2008) 13:393–405. doi: 10.3233/JAD-2008-13405
79. Itzhaki RF, Wozniak MA. Alzheimer's disease-like changes in herpes simplex virus type 1 infected cells: the case for antiviral therapy. Rejuvenation Res. (2008) 11:319–20. doi: 10.1089/rej.2008.0673
80. Westman G, Blomberg J, Yun Z, Lannfelt L, Ingelsson M, Eriksson BM. Decreased HHV-6 IgG in Alzheimer's Disease. Front Neurol. (2017) 8:40. doi: 10.3389/fneur.2017.00040
81. Ward T, Powell RM, Pipkin PA, Evans DJ, Minor PD, Almond JW. Role for beta2-microglobulin in echovirus infection of rhabdomyosarcoma cells. J Virol. (1998) 72:5360–5.
82. Triantafilou M, Triantafilou K, Wilson KM, Takada Y, Fernandez N, Stanway G. Involvement of beta2-microglobulin and integrin alphavbeta3 molecules in the coxsackievirus A9 infectious cycle. J Gen Virol. (1999) 80:2591–600. doi: 10.1099/0022-1317-80-10-2591
83. Itzhaki RF, Lathe R, Balin BJ, Ball MJ, Bearer EL, Braak H, et al. Microbes and Alzheimer's disease. J Alzheimers Dis. (2016) 51:979–84. doi: 10.3233/JAD-160152
84. Jang H, Boltz DA, Webster RG, Smeyne RJ. Viral parkinsonism. Biochim Biophys Acta (2009) 1792:714–21. doi: 10.1016/j.bbadis.2008.08.001
85. Cooper EH, Forbes MA, Hambling MH. Serum beta 2-microglobulin and C reactive protein concentrations in viral infections. J Clin Pathol. (1984) 37:1140–3. doi: 10.1136/jcp.37.10.1140
86. Grundy JE, Mckeating JA, Ward PJ, Sanderson AR, Griffiths PD. Beta 2 microglobulin enhances the infectivity of cytomegalovirus and when bound to the virus enables class I HLA molecules to be used as a virus receptor. J Gen Virol. (1987) 68:793–803. doi: 10.1099/0022-1317-68-3-793
87. Mckeating JA, Griffiths PD, Grundy JE. Cytomegalovirus in urine specimens has host beta 2 microglobulin bound to the viral envelope: a mechanism of evading the host immune response? J Gen Virol. (1987) 68 (Pt 3):785–92.
88. Stannard LM. Beta 2 microglobulin binds to the tegument of cytomegalovirus: an immunogold study. J Gen Virol. (1989) 70:2179–84. doi: 10.1099/0022-1317-70-8-2179
89. Browne H, Smith G, Beck S, Minson T. A complex between the MHC class I homologue encoded by human cytomegalovirus and beta 2 microglobulin. Nature (1990) 347:770–2. doi: 10.1038/347770a0
90. Ezzat K, Pernemalm M, Palsson S, Roberts TC, Jarver P, Dondalska A, et al. The viral protein Corona directs viral pathogenesis and amyloid aggregation. bioRxiv [Preprint] (2018). doi: 10.1101/246785
91. Heaton RK, Cysique LA, Jin H, Shi C, Yu X, Letendre S, et al. Neurobehavioral effects of human immunodeficiency virus infection among former plasma donors in rural China. J Neurovirol. (2008) 14:536–49. doi: 10.1080/13550280802378880
92. Thames AD, Streiff V, Patel SM, Panos SE, Castellon SA, Hinkin CH. The role of HIV infection, cognition, and depression in risky decision-making. J Neuropsychiatry Clin Neurosci. (2012) 24:340–8. doi: 10.1176/appi.neuropsych.11110340
93. MMWR Recommendations and Report 1993 revised classification system for HIV infection and expanded surveillance case definition for AIDS among adolescents and adults. MMWR Recommend Rep. (1992) 41:1–19.
94. Turner S, Ellexson ME, Hickman HD, Sidebottom DA, Fernandez-Vina M, Confer DL, et al. Sequence-based typing provides a new look at HLA-C diversity. J Immunol. (1998) 161:1406–13.
95. Bunce M, O'neill CM, Barnardo MC, Krausa P, Browning MJ, Morris PJ, et al. Phototyping: comprehensive DNA typing for HLA-A, B, C, DRB1, DRB3, DRB4, DRB5 & DQB1 by PCR with 144 primer mixes utilizing sequence-specific primers (PCR-SSP). Tissue Antigens (1995) 46:355–67. doi: 10.1111/j.1399-0039.1995.tb03127.x
96. Guerini FR, Fusco C, Mazzi B, Favoino B, Nocera G, Agliardi C, et al. HLA-Cw allele frequencies in northern and southern Italy. Transpl Immunol. (2008) 18:286–9. doi: 10.1016/j.trim.2007.08.003
97. Ghafouri M, Amini S, Khalili K, Sawaya BE. HIV-1 associated dementia: symptoms and causes. Retrovirology (2006) 3:28. doi: 10.1186/1742-4690-3-28
98. Ances BM, Clifford DB. HIV-associated neurocognitive disorders and the impact of combination antiretroviral therapies. Curr Neurol Neurosci Rep. (2008) 8:455–61. doi: 10.1007/s11910-008-0073-3
99. Ogishi M, Yotsuyanagi H. Prediction of HIV-associated neurocognitive disorder (HAND) from three genetic features of envelope gp120 glycoprotein. Retrovirology (2018) 15:12. doi: 10.1186/s12977-018-0401-x
100. Saylor D, Dickens AM, Sacktor N, Haughey N, Slusher B, Pletnikov M, et al. HIV-associated neurocognitive disorder–pathogenesis and prospects for treatment. Nat Rev Neurol. (2016) 12:234–48. doi: 10.1038/nrneurol.2016.27
101. Lin Z, Kuroki K, Kuse N, Sun X, Akahoshi T, Qi Y, et al. HIV-1 Control by NK Cells via Reduced Interaction between KIR2DL2 and HLA-C(*)12:02/C(*)14:03. Cell Rep. (2016) 17:2210–20. doi: 10.1016/j.celrep.2016.10.075
102. Chikata T, Tran GV, Murakoshi H, Akahoshi T, Qi Y, Naranbhai V, et al. HLA class I-mediated HIV-1 control in vietnamese infected with HIV-1 subtype A/E. J Virol. (2018) 92:e01749–17. doi: 10.1128/JVI.01749-17
103. Mcarthur JC, Brew BJ. HIV-associated neurocognitive disorders: is there a hidden epidemic? AIDS (2010) 24:1367–70. doi: 10.1097/QAD.0b013e3283391d56
104. Sanford R, Fellows LK, Ances BM, Collins DL. Association of brain structure changes and cognitive function with combination antiretroviral therapy in HIV-positive individuals. JAMA Neurol. (2018) 75:72–9. doi: 10.1001/jamaneurol.2017.3036
105. Cysique LA, Vaida F, Letendre S, Gibson S, Cherner M, Woods SP, et al. Dynamics of cognitive change in impaired HIV-positive patients initiating antiretroviral therapy. Neurology (2009) 73:342–8. doi: 10.1212/WNL.0b013e3181ab2b3b
106. Sacktor N, Mcdermott MP, Marder K, Schifitto G, Selnes OA, Mcarthur JC, et al. HIV-associated cognitive impairment before and after the advent of combination therapy. J Neurovirol. (2002) 8:136–42. doi: 10.1080/13550280290049615
107. Heaton RK, Clifford DB, Franklin DRJr, Woods SP, Ake C, Vaida F, et al. HIV-associated neurocognitive disorders persist in the era of potent antiretroviral therapy: charter study. Neurology (2010) 75:2087–96. doi: 10.1212/WNL.0b013e318200d727
108. Hammond ER, Crum RM, Treisman GJ, Mehta SH, Marra CM, Clifford DB, et al. The cerebrospinal fluid HIV risk score for assessing central nervous system activity in persons with HIV. Am J Epidemiol. (2014) 180:297–307. doi: 10.1093/aje/kwu098
109. Calcagno A, Barco A, Trunfio M, Bonora S. CNS-targeted antiretroviral strategies: when are they needed and what to choose. Curr HIV/AIDS Rep. 15:84–91. (2018). doi: 10.1007/s11904-018-0375-2
110. Giunta B, Ehrhart J, Obregon DF, Lam L, Le L, Jin J, et al. Antiretroviral medications disrupt microglial phagocytosis of beta-amyloid and increase its production by neurons: implications for HIV-associated neurocognitive disorders. Mol Brain (2011) 4:23. doi: 10.1186/1756-6606-4-23
111. Langford D, Marquie-Beck J, De Almeida S, Lazzaretto D, Letendre S, Grant I, et al. Relationship of antiretroviral treatment to postmortem brain tissue viral load in human immunodeficiency virus-infected patients. J Neurovirol. (2006) 12:100–7. doi: 10.1080/13550280600713932
112. Price RW, Epstein LG, Becker JT, Cinque P, Gisslen M, Pulliam L, et al. Biomarkers of HIV-1 CNS infection and injury. Neurology (2007) 69:1781–8. doi: 10.1212/01.wnl.0000278457.55877.eb
113. De Chiara G, Marcocci ME, Sgarbanti R, Civitelli L, Ripoli C, Piacentini R, et al. Infectious agents and neurodegeneration. Mol Neurobiol. (2012) 46:614–38. doi: 10.1007/s12035-012-8320-7
114. Wyss-Coray T, Rogers J. Inflammation in Alzheimer disease-a brief review of the basic science and clinical literature. Cold Spring Harb Perspect Med. (2012) 2:a006346. doi: 10.1101/cshperspect.a006346
115. Lehmann DJ, Barnardo MC, Fuggle S, Quiroga I, Sutherland A, Warden DR, et al. Replication of the association of HLA-B7 with Alzheimer's disease: a role for homozygosity? J Neuroinflammation (2006) 3:33. doi: 10.1186/1742-2094-3-33
116. Henschke PJ, Bell DA, Cape RD. Alzheimer's disease and HLA. Tissue Antigens (1978) 12:132–5. doi: 10.1111/j.1399-0039.1978.tb01308.x
117. Renvoize EB, Hambling MH, Pepper MD, Rajah SM. Possible association of Alzheimer's disease with HLA-BW15 and cytomegalovirus infection. Lancet (1979) 1:1238. doi: 10.1016/S0140-6736(79)91914-7
118. Walford RL. Immunology and aging Philip Levine Award. Am J Clin Pathol. (1980) 74:247–53. doi: 10.1093/ajcp/74.3.247
119. Achim CL, Adame A, Dumaop W, Everall IP, Masliah E, Neurobehavioral Research C. Increased accumulation of intraneuronal amyloid beta in HIV-infected patients. J Neuroimmune Pharmacol. (2009) 4:190–9. doi: 10.1007/s11481-009-9152-8
120. Ciccarelli N, Grima P, Fabbiani M, Baldonero E, Borghetti A, Milanini B, et al. Baseline CD4(+) T-cell count and cardiovascular risk factors predict the evolution of cognitive performance during 2-year follow-up in HIV-infected patients. Antivir Ther. (2015) 20:433–40. doi: 10.3851/IMP2925
Keywords: HIV, AIDS, HLA, AIDS dementia complex (ADC), HIV-associated neurocognitive disorders (HAND), β2-microglobulin (β2m)
Citation: Zipeto D, Serena M, Mutascio S, Parolini F, Diani E, Guizzardi E, Muraro V, Lattuada E, Rizzardo S, Malena M, Lanzafame M, Malerba G, Romanelli MG, Tamburin S and Gibellini D (2018) HIV-1-Associated Neurocognitive Disorders: Is HLA-C Binding Stability to β2-Microglobulin a Missing Piece of the Pathogenetic Puzzle? Front. Neurol. 9:791. doi: 10.3389/fneur.2018.00791
Received: 20 June 2018; Accepted: 03 September 2018;
Published: 21 September 2018.
Edited by:
Avindra Nath, National Institute of Neurological Disorders and Stroke (NINDS), United StatesReviewed by:
Dianne T. Langford, Lewis Katz School of Medicine, Temple University, United StatesOlimpia Meucci, College of Medicine, Drexel University, United States
Copyright © 2018 Zipeto, Serena, Mutascio, Parolini, Diani, Guizzardi, Muraro, Lattuada, Rizzardo, Malena, Lanzafame, Malerba, Romanelli, Tamburin and Gibellini. This is an open-access article distributed under the terms of the Creative Commons Attribution License (CC BY). The use, distribution or reproduction in other forums is permitted, provided the original author(s) and the copyright owner(s) are credited and that the original publication in this journal is cited, in accordance with accepted academic practice. No use, distribution or reproduction is permitted which does not comply with these terms.
*Correspondence: Donato Zipeto, ZG9uYXRvLnppcGV0b0B1bml2ci5pdA==