- Department of Medical and Surgical Sciences, University of Foggia, Foggia, Italy
Extracellular vesicles (EVs) are small vesicles including microvesicles and exosomes which differ in their distinct size, density, biogenesis, and content. Until recently, EVs were considered as simply scrap products. Nowadays, they are engendering huge interest and their shedding plays a well-recognized role in intercellular communication, not only participating in many physiological processes, but also suspected of being involved in the pathogenesis of many diseases. The present review aims to summarize the latest updates on immune cell-derived EVs, focusing on the current status of knowledge in Multiple Sclerosis. Significant progress has been made on their physical and biological characterization even though many aspects remain unclear and need to be addressed. However, it is worth further investigating in order to deepen the knowledge of this unexplored and fascinating field that could lead to intriguing findings in the evaluation of EVs as biomarkers in monitoring the course of diseases and the response to treatments.
Definition and Characteristics of EVs
Cells use different mechanisms to communicate with each other, such as direct contact or secretion of signal molecules. Recent studies suggest the existence of a previously unknown communication route based on the release into the extracellular space of a special cargo called extracellular vesicles (EVs). They include both microvesicles (MVs), also known as ectosomes, and exosomes (EXOs), which differ in their size, density, biogenesis and content. MVs are defined as small vesicles (100–1,000 nm) budding specifically from the surface of the plasma membrane and are expelled into the extracellular milieu. EXOs (40–100 nm) are lower membrane vesicles coming from inward budding of multivesicular bodies that, by fusing with the plasma membrane, release their contents into the extracellular compartment (1). The characteristic features of the two extracellular vesicle populations are summarized in Table 1.
Until recently, MVs were thought to be an in vitro artifact or considered as a system to eliminate waste products from cells (2, 3). Nowadays, MVs are attracting a lot of interest due to their crucial role in intercellular communication (4).
Physiological Functions of EVs
Many studies have stressed the importance of EVs in the normal activity of the central nervous system (CNS). As a matter of fact, glia and neurons release EVs and, as the current literature points out, they play a pivotal role in many physiological effects in the CNS by maintaining homeostasis, including metabolic support, myelin formation and immune defense (5). EVs have been associated with neurogenesis, modulation of synaptic activity and nerve regeneration. As a matter of fact, during the development of the brain in mice, an intensified production of neuronal stem cells was observed (6, 7). The presence of prominin-1 (CD133), a marker of self-renewal and differentiation, found in the vesicles encouraged some hypotheses on the physiological role of EVs that, by interacting with membrane phospholipids and cholesterol, could activate signal transduction pathways leading to cell differentiation (7). Other researchers have proposed that EVs are able to influence the phenotype of other cells as they carry genetic material encoding transcription factors (8). Moreover, EVs transferring b-galactosidase are also considered as important actors of the axonal guidance process (9). Furthermore, several studies have reported that EVs are able to modulate synaptic activity. Cortical neuron derived exosomes after depolarization have been shown to contain cell adhesion proteins and subunits of neurotransmitter receptors (10). Antonucci et al. (11) confirmed the involvement of microglia-derived EVs in synaptic activity by correlating their presence in the presynaptic space to the increase in the release of excitatory neurotransmitters. The EVs produced by Schwann cells have also been associated with the regeneration of damaged nerves, as they carry ribosome or mRNA useful for the synthesis of proteins necessary for their repair (12).
Pathological Involvement of EVs
Although EVs are also produced in physiological conditions, their number is well known to increase during pathological processes, contributing to the development of cancer, cardiovascular and autoimmune diseases. As far as neurological diseases are concerned, many studies have reported changes related to the number and the function of EVs in some common neurological diseases, as well as stroke and epilepsy (13). Agosta et al. showed elevated rates of MVs of myeloid origin in the cerebrospinal fluids (CSF) of patients affected by Alzheimer's disease (AD) and, therefore, suggested that MVs could be useful markers of microglia activation during neuroinflammation in AD (14). Xue et al. (15) have also reported elevated concentrations of MVs in the plasma of AD patients in correlation with their cognitive decline. Moreover, the involvement of EVs in AD is suggested by the association of Amyloid-beta (Ab) with exosomes and it is highlighted by the accumulation of other exosomal proteins, such as flotillin, in the plaques of AD brains (16). However, to date the role of EVs in AD continues to be debated because, depending on the cell of origin, EVs seem to have a toxic or beneficial effect. Yuyama et al. (17), for example, have suggested a neuroprotective role of EVs in AD. As a matter of fact, EVs could act as scavengers of neurotoxic Ab both either by preventing its aggregations and by inducing its proteolysis (18). Furthermore, the beneficial role of EVs in AD could be explained by the presence of the neuroprotective proteins, such as Cystatin C, inside the exosomes released by mouse primar neurons (19).
As far as Stroke is concerned, many studies have reported an involvement of EVs. Most recently, Nanosight tracking analysis (NTA) utilized to determine EV number in the sera of stroke patients has revealed an increase vs. age-matched controls. Moreover, monocyte-differentiated macrophage cultures challenged with EV fractions were found to be activated to produce proinflammatory interleukins such as TNFa and IL1b (20).
EVs in Multiple Sclerosis
In the pathogenesis of MS, EVs show both protective and damaging functions. As a matter of fact, EVs could be considered to have a beneficial effect during neurological processes by restoring trophic factors, removing damaged cells (21), controlling synaptogenesis (22), and monitoring the functional state of synapses (23) as described above. Nevertheless, accumulating data suggest that EVs could be implicated in the pathogenesis of MS, especially during relapses involved in cellular activation, facilitating the crossing of the blood–brain barrier and, in this way, amplifying inflammation in the CNS (24).
In 1989 Scolding et al. (25) had already published finding the presence of vesicles in the CSF of subjects suffering from Multiple Sclerosis (MS), suggesting their contribution to myelin damage in vivo. More recently, Verderio et al. confirmed these data finding an overproduction of EVs in CSF in subjects with a diagnosis of CIS or MS vs. healthy controls (26). This considerable amount of EVs in CSF was reflected in the remarkable quantity of EVs released by the other cellular components of the blood such as monocytes, leukocytes and platelets, evaluated in MS patients compared to HD (27). Several independent studies report increased levels of EVs in MS patients compared to healthy donors and many authors have measured plasmatic MVs in order to assess a possible correlation between their blood concentration with clinical outcomes and other laboratory tests, as reviewed by Furlan's group (28). In many works these MVs were especially of endothelial origin and associated to the relapsing phase of the disease (29–31). Marco-Ramiro et al. demonstrated a relevant increase in the plasma microparticles coming from the platelets and the endothelium in all clinical forms of MS and in CIS patients (32). Sáenz-Cuesta et al. (27) also extended microvesicle production to platelets, lymphocytes and monocytes. Very recently, a nanoplasmonic approach has confirmed that a significant increase in plasmatic EVs distinguishes CIS and MS patients from controls (33). Moreover, in many cases MVs were used in MS as biomarkers of therapeutic efficacy. The reduction of endothelial MVs has been associated to IFN-β 1b administration (31, 34). Furthermore, Lowery-Nordberg et al.'s prospected study (35) establishes a considerable relationship between the diminished number of MVs and the improvement of the lesions evaluated by MRI. On the contrary, a recent study by Sáenz-Cuesta et al. (27) reported higher levels of plasmatic MVs despite IFN-β and Natalizumab treatment. Dawson et al. proved that Fingolimod suppressed the enzymatic activity of SMase (36), which represents an important step for the MV release. Verderio et al. (26), when observing that EAE mice treated with Fingolimod displayed a reduction in CSF myeloid MVs to baseline levels, hypothesized that the drug might prevent myeloid cell-derived MV shedding from reactive microglia inhibiting the spreading of inflammatory signals throughout the brain.
Although the exact mechanism underlying MV shedding is not yet clearly elucidated, the role of P2X7 receptor (P2X7R) is well recognized. Growing scientific evidence shows that this purinergic receptor, expressed especially on cells of hematopoietic origin (37, 38), is involved in the release of MVs by immune cells especially of the myeloid lineage. As a matter of fact, upon activation of P2X7R by its endogenous ligand ATP or by the synthetic analog Benzoylbenzoyl-ATP (Bz-ATP), microvesicles shedding from the surface of microglia were observed associated with the release of the inflammatory cytokine IL-1B (39).
Very recently, we have reported an elevated amount of microvesicles produced by monocytes of patients affected by MS compared to healthy donors (40). We also observed that treatments currently used in MS affected monocyte-derived microvesicle production. In particular we reported the effects of IFN-β1a and Teriflunomide as first-line treatments able to reduce MV shedding and IL1b gene expression directly related to the duration of the therapy. Considering Fingolimod as a second-line drug, after 12 months of administration, we found the same effect in decreasing MV release.
A short time ago, Furlan et al. demonstrated in the EAE animal model of MS the capability of some interleukins to enhance the production of MVs from cells of myeloid origin without the activation of P2X7R by ATP, suggesting the existence of an alternative pathway that might trigger MV shedding during chronic phlogistic processes (41).
In recent years several studies have been focused on the evaluation of MV contents. Despite the increasingly recognized relevance of EVs, the content of EVs still remains little known. The different elements inside the EXOs or MVs could influence their biological and immunological effects. Apart from having different surface markers, EVs are thought to contain different bioactive molecules (42–44), including lipids, proteins such as cytokines, receptors, signaling molecules, and integrins as well as nucleic acids and organelles (7, 45, 46), as shown in Figure 1. In many studies various forms of RNA appear to be enclosed in EVs (47, 48) or conjugates with lipoprotein (49, 50) as a form to prevent degradation. For example, miRNA circulating in blood or present in saliva is reported to be incorporated in EXOs (51). EVs, in particular MVs, because of their characteristic of transferring genetic material (52–54), are suspected of being key actors in intercellular communication and in pathogenesis of the disease. In fact, mRNA encapsulated in MVs has been detected in healthy as well as in diseased subjects (55, 56). Patz et al. showed that CSF-derived MVs could regulate neuronal processes by shuttling mRNA, miRNA, and proteins to target cells (57). To date, the role and the contents ofboth EXOs and MVs combined have still been little explored. There are only a few published studies on evRNAs based on array analysis techniques. For example, Valadi et al., performing a microarray assessment exclusively on EXOs produced by murine or human mast cells, observed the presence of mRNA and small RNA related to 1,300 genes, many of which were not present in the cells which the exosome came from Valadi et al. (58). In contrast, until now the advanced high-throughput next-generation sequencing technique (HT-NGS) has only been applied to the limited field of cultured cells, human urine and circulating RNA (59). Nowadays, a lot of experimental evidence is emerging. Very recently, using this technique, Liguori et al. were able to identify circulating miRNA up regulated and down regulated in pediatric MS contributing to a better understanding of the genetic basis of the disease (60). Also recently, data acquired through NGS analysis have led to the global RNA profile of serum being defined and exosomal miRNA being discovered as a potentially useful biomarker to distinguish MS relapse (61). Actually, thanks to their small volume, MVs may spread from the release site and be found in different biological liquids such as serum, urine, synovial and CSF. Peripheral blood is a great source of EVs (62) and certainly more accessible than CSF. On this basis, performing some preliminary experiments aimed at developing the technique, we analyzed RNA isolated from EVs circulating in serum of a small group of CIS patients (63), obtaining interesting evidence that contributes to improving the knowledge about EV function. In a small cohort of subjects, we were able to identify distinct classes of ncRNAs in both the EV subsets, in line with previous data (58, 61, 62, 64). Furthermore, we found some peculiar differences between CIS and healthy donors that may be considered for use in discriminating between the two conditions. It is worth noting that in depth analysis revealed the most representative ncRNAs classes. In addition, the small expression of rRNA found in MVs could be considered distinctive compared to EXOs, characterized by a higher amount of rRNA, as previously published (59). Interestingly, we detected snRNA in both MVs and EXOs, long ncRNA (lncRNA) fragments, and long intergenic ncRNA (lincRNA) fragments in line with the cytoplasmic origin of EVs, considering that RNA degradation occurs in the cytoplasm (65).
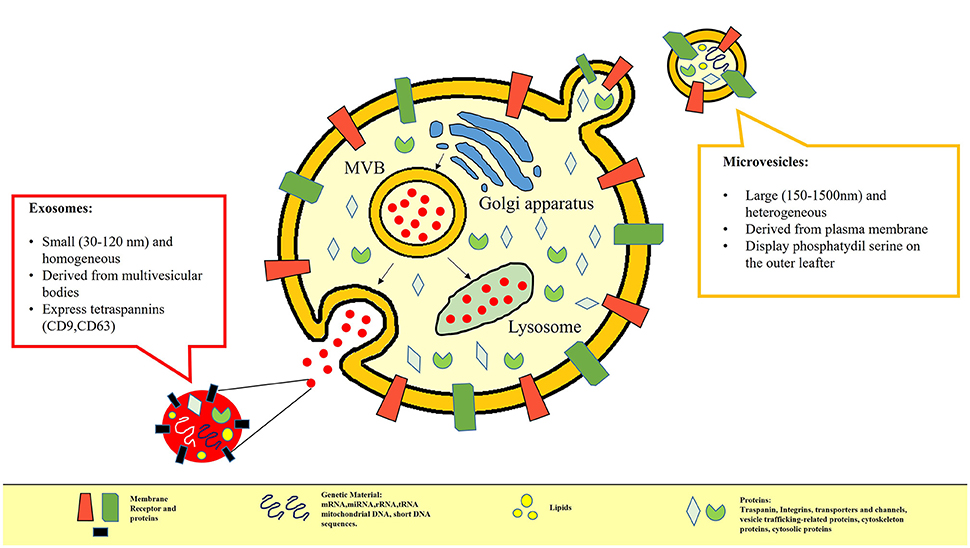
Figure 1. In this picture the differences between MVs and EXOs in terms of different size, biogenesis and content are represented. MVs are small vesicles (100–1,000 nm) which bud directly from the cell plasma membrane and are released into the extracellular environment; EXOs (40–100 nm) are defined as small membrane vesicles formed by inward budding of endosomal membranes called multivesicular bodies that, when fusing with the plasma membrane, release the content in the extracellular compartment. Apart from having different surface markers, Extracellular Vesicles are also considered to be storage pools of diverse bioactive molecules. Their content may include proteins (e.g., signaling molecules, receptors, integrins, and cytokines), bioactive lipids, nucleic acids (e.g., miRNA, mRNA, DNA) and organelles.
Ebrahimkhani et al. (66), using NGS, have also identified differentially expressed exosomal miRNAs extracted from sera samples in both RRSM and progressive MS patients compared to healthy donors. Indeed, they report dysregulated miRNA that can reasonably distinguish RRSM or SPMS and PPMS patients from healthy controls. It is worth noting that they identified nine miRNA that may help to discriminate between the stages of the disease, providing a useful tool in assisting the determination of the transition from RRMS to SPMS. To date, there are no clinical parameters that allow the passage from one stage to another of the disease to be predicted. Therefore, these findings, if confirmed by longitudinal studies, may well have clinical and economic implications.
Open Issues
Despite the introduction of cutting-edge approaches for their characterization, current knowledge on EVs is still inadequate to provide exhaustive information about their morphology, size, density, phenotype, concentration, and content. The sub-micrometer size of EVs and the intrinsic limitations in methods of characterization of EVs make the development of standardized protocols of sample preparation and of EV measurement conditions very difficult. For example, there is little agreement concerning the concentration of EVs in plasma: values ranging from 200 to 109 EVs uL−1 are reported in the literature (67), hence several orders of magnitude of difference according the method utilized for detection. To date, the various research groups have used different procedures both for the isolation and the analysis of EVs as summarized in Table 2 and in Table 3. Recently, Coumans et al. (68) tried to draw up a methodological guideline to study EVs by providing an expert-based update of recent advances in the protocols and by summarizing currently accepted considerations and recommendations in every stage of analysis from sample collection to extraction, detection, and characterization of EVs. This remains a crucial point that needs to be addressed in future in order to establish internationally recognized criteria for the objective evaluation of the roles of EVs in health and disease, as well as their biomedical potential.
Conclusions
In conclusion, this review summarizes the state of the art on extracellular vesicles in MS. Long gone are the times when EVs were considered as simply scrap products. Nowadays they are known to be important players in physiological processes and in the pathogenesis of many diseases. Although a lot of effort has been made and yet more is still ongoing contributing to the significant progress on the physical and biological characterization of EVs, many aspects still remain not fully elucidated and certainly deserve to be addressed in the near future. Overall, recent scientific evidence seems to be highly promising and strongly encourages more in-depth studies to further explore the potential of EVs as biomarkers in monitoring disease course and response to treatment.
Author Contributions
MB and CA defined the research questions and aims of the study. AA, TM, and MB carried out the literature search and selected relevant papers. MB wrote the first draft of the manuscript. CA revised and corrected the manuscript. All authors read and approved the final manuscript.
Conflict of Interest Statement
The authors declare that the research was conducted in the absence of any commercial or financial relationships that could be construed as a potential conflict of interest.
Acknowledgements
The authors wish to thank Richard Lusardi B.Sc. M.A. (T.E.F.L.) for his revision of the manuscript.
References
1. Raposo G, Stoorvogel W. Extracellular vesicles: exosomes, microvesicles, and friends. J Cell Biol. (2013) 200:373–83. doi: 10.1083/jcb.201211138
3. Turola E, Furlan R, Bianco F, Mattiola M, Verderio C. Microglial microvesicle secretion and intercellular signaling. Front Physiol. (2012) 3:149. doi: 10.3389/fphys.2012.00149
4. Pitt JM, Kroemer G, Zitvogel L. Extracellular vesicles: masters of intercellular communication and potential clinical interventions. J Clin Invest. (2016) 126:1139–43. doi: 10.1172/JCI87316.
5. Frühbeis C, Fröhlich D, Kuo WP, Krämer-Albers EM. Extracellular vesicles as mediators of neuron-glia communication. Front Cell Neurosci. (2013) 7:182. doi: 10.3389/fncel.2013.00182
6. Marzesco AM, Janich P, Wilsch-Brauninger M Dubreuil V, Langenfeld K, Corbeil D, et al. Release of extracellular membrane particles carrying the stem cell marker prominin-1 (CD133) from neural progenitors and other epithelial cells. J Cell Sci. 118(Pt 13):2849–58. doi: 10.1242/jcs.02439
7. Marzesco AM. Prominin-1 (CD133): new insights on stem & cancer stem cell biology. In: Prominin-1-Containing Membrane Vesicles: Origins, Formation, and Utility; Advances in Experimental Medicine and Biology, Vol. 777. New York, NY: Springer (2013), p. 41–54. doi: 10.1007/978-1-4614-5894-4_3
8. Ratajczak J, Wysoczynski M, Hayek F, Janowska-Wieczorek A, Ratajczak MZ. Membrane-derived microvesicles: important and underappreciated mediators of cell-to-cell communication. Leukemia (2006) 20:1487–95. doi: 10.1038/sj.leu.2404296
9. Bakhti M, Winter C, Simons M. Inhibition of myelin membrane sheath formation by oligodendrocyte-derived exosome-like vesicles. J Biol Chem. (2011) 286:787–96. doi: 10.1074/jbc.M110.190009
10. Faure J, Lachenal G, Court M, Hirrlinger J, Chatellard-Causse C, Blot B, et al. Exosomes are released by cultured cortical neurons. Mol Cell Neurosci. (2006) 31:642–8. doi: 10.1016/j.mcn.2005.12.003
11. Antonucci F, Turola E, Riganti L, Caleo M, Gabrielli M, Perrotta C, et al. Microvesicles released from microglia stimulate synaptic activity via enhanced sphingolipid metabolism. EMBO J. (2012) 31:1231–40. doi: 10.1038/emboj.2011.489
12. Court FA, Midha R, Cisterna BA, Grochmal J, Shakhbazau A, Hendriks WT, et al. Morphological evidence for a transport of ribosomes from Schwann cells to regenerating axons. Glia (2011) 59:1529–39. doi: 10.1002/glia.21196
13. Lai CP, Breakefield XO. Role of exosomes/microvesicles in the nervous sys-tem and use in emerging therapies. Front Physiol. (2012) 3:228. doi: 10.3389/fphys.2012.00228
14. Agosta F, Dalla Libera D, Spinelli EG, Finardi A, Canu E, Bergami A, et al. Myeloid microvesicles in cerebrospinal fluid are associated with myelin damage and neuronal loss in mild cognitive impairment and Alzheimer disease. Ann Neurol. (2014) 76:813–25. doi: 10.1002/ana.24235
15. Xue S, Cai X, Li W, Zhang Z, Dong W, Hui G. Elevated plasma endothelial microparticles in Alzheimer's disease. Dement Geriatr Cogn Disord. (2012) 34:174–80. doi: 10.1159/000343491
16. Bitsikas V, Riento K, Howe JD, Barry NP, Nichols BJ. The role of flotillins in regulating Aβ production, investigated using Flotillin 1-/-, Flotillin 2-/- double knockout mice. PLoS ONE (2014) 9:e85217. doi: 10.1371/journal.pone.0085217
17. Yuyama K, Sun H, Sakai S, Mitsutake S, Okada M, Tahara H, et al. Decreased amyloid-β pathologies by intracerebral loading of glycosphingolipid-enriched exosomes in Alzheimer model mice. J Biol Chem. (2014) 289:24488–98. doi: 10.1074/jbc.M114.577213
18. Yuyama K, Sun H, Usuki S, Sakai S, Hanamatsu H, Mioka T, et al. A potential function for neuronal exosomes: sequestering intracerebral amyloid-β peptide. FEBS Lett. (2015) 589:84–8. doi: 10.1016/j.febslet.2014.11.027
19. Ghidoni R, Paterlini A, Albertini V, Glionna M, Monti E, Schiaffonati L, et al. Cystatin C is released in association with exosomes: a new tool of neuronal communication which is unbalanced in Alzheimer's disease. Neurobiol Aging (2011) 32:1435–42. doi: 10.1016/j.neurobiolaging.2009.08.013
20. Couch Y, Akbar N, Davis S, Fischer R, Dickens AM, Neuhaus AA, et al. Inflammatory stroke extracellular vesicles induce macrophage activation. Stroke (2017) 48:2292–6. doi: 10.1161/STROKEAHA.117.017236
21. Olah, M, Amor S, Brouwer N, Vinet J, Eggen B, Biber K, et al. Identification of a microglia phenotype supportive of remyelination. Glia (2012) 60:306–21. doi: 10.1002/glia.21266
22. Roumier A, Béchade C, Poncer JC, Smalla KH, Tomasello E, Vivier E, et al. Impaired synaptic function in the microglial KARAP/DAP12-deficient mouse. J Neurosci. (2004) 24:11421–8. doi: 10.1523/JNEUROSCI.2251-04.2004
23. Wake H, Moorhouse AJ, Jinno S, Kohsaka S, Nabekura J. Resting microglia directly monitor the functional state of synapses in vivo and determine the fate ofischemic terminals. J Neurosci. (2009) 29:3974–80. doi: 10.1523/JNEUROSCI.4363-08.2009
24. Sáenz-Cuesta M, Osorio-Querejeta I, Otaegui D. Extracellular vesicles in Multiple Sclerosis: what are they telling us? Front Cell Neurosci. (2014) 8:100. doi: 10.3389/fncel.2014.00100
25. Scolding NJ, Frith S, Linington C, Morgan BP, Campbell AK, Compston DA. Myelin oligodendrocyte glycoprotein (MOG) is a surface marker of oligodendrocytematuration. J Neuroimmunol. (1989) 22:169–176. doi: 10.1016/0165-5728(89)90014-3
26. Verderio C, Muzio L, Turola E, Bergami A, Novellino L, Ruffini F, et al. Myeloidmicrovesicles are a marker and therapeutic target for neuroinflammation. Ann Neurol. (2012) 72:610–24. doi: 10.1002/ana.23627
27. Sáenz-Cuesta M, Irizar H, Castillo-Triviño T, Muñoz-Culla M, Osorio-Querejeta I, Prada A, et al. Circulating microparticles reflect treatment effects and clinical status in Multiple Sclerosis. Biomark Med. (2014) 8:653–61. doi: 10.2217/bmm.14.9
28. Carandini T, Colombo F, Finardi A, Casella G, Garzetti L, Verderio C, Furlan R. Microvesicles: what is the role in Multiple Sclerosis? Front Neurol. (2015) 6:111. doi: 10.3389/fneur.2015.00111
29. Minagar A, Jy W, Jimenez JJ, Sheremata WA, Mauro LM, Mao WW, et al. Elevated plasma endothelial microparticles in Multiple Sclerosis. Neurology (2001) 56:1319–24. doi: 10.1212/WNL.56.10.1319
30. Jy W, Minagar A, Jimenez JJ, Sheremata WA, Mauro LM, Horstman LL, et al. Endothelial microparticles (EMP) bind and activate monocytes: elevated EMP monocyte conjugates in Multiple Sclerosis. Front Biosci. (2004) 9:3137–44. doi: 10.2741/1466
31. Jimenez J, Jy W, Mauro LM, Horstman LL, Ahn ER, Ahn YS, et al. Elevated endothelial microparticle monocyte complexes induced by Multiple Sclerosis plasma and the inhibitory effects of interferon-beta1b on release of endothelial microparticles, formation and trans endothelial migration of monocyte- endothelial microparticle complexes. Mult Scler. (2005) 11:310–5. doi: 10.1191/1352458505ms1184oa
32. Marcos-Ramiro B, Oliva Nacarino P, Serrano-Pertierra E, Blanco-Gelaz MA, Weksler BB, Romero IA, et al. Microparticles in Multiple Sclerosis and clinically isolated syndrome: effect on endothelial barrier function. BMC Neurosci. (2014) 15:110. doi: 10.1186/1471-2202-15-110
33. Mallardi A, Nuzziello N, Liguori M, Avolio C, Palazzo G. Counting of peripheral extracellular vesicles in Multiple Sclerosis patients by an improved nanoplasmonic assay and dynamic light scattering. Colloids Surf B Biointerfaces (2018) 168:134–42. doi: 10.1016/j.colsurfb.2018.02.006
34. Sheremata WA, Jy W, Delgado S, Minagar A, McLarty J, Ahn Y. Interferon-β1a reduces plasma CD31+ endothelial microparticles (CD31+EMP) in Multiple Sclerosis. J Neuroinflammation (2006) 3:23. doi: 10.1186/1742-2094-3-23
35. Lowery-Nordberg M, Eaton E, Gonzalez-Toledo E, Harris MK, Chalamidas K, McGee-Brown J, et al. The effects of high dose interferon-beta1a on plasma microparticles: correlation with MRI parameters. J Neuroinflammation (2011) 8:43. doi: 10.1186/1742-2094-8-43
36. Dawson G, Qin J. Gilenya (FTY720) inhibits acid sphingomyelinase by a mechanism similar to tricyclic antidepressants. Biochem Biophys Res Commun. (2011) 404:321–3. doi: 10.1016/j.bbrc.2010.11.115
37. Collo G, Neidhart S, Kawashima E, Kosco-Vilbois M, North RA, Buell G. Tissue distribution of the P2X7 receptor. Neuropharmacology (1997) 36:1277–83.
38. Caragnano M, Tortorella P, Bergami A, Ruggieri M, Livrea P, Specchio LM, et al. Monocytes P2X7 purinergic receptor is modulated by glatiramer acetate in Multiple Sclerosis. J Neuroimmunol. (2012) 245:93–7. doi: 10.1016/j.jneuroim.2012.02.002
39. Bianco F, Perrotta C, Novellino L, Francolini M, Riganti L, Menna E, et al. Acid sphingomyelinase activity triggers microparticle release from glial cells. EMBO J. (2009) 28:1043–54. doi: 10.1038/emboj.2009
40. Blonda M, Amoruso A, Grasso R, Di Francescantonio V, Avolio C. Multiple Sclerosis treatments affect monocyte-derived microvesicle production. Front Neurol. (2017) 8:422. doi: 10.3389/fneur.2017.00422
41. Colombo F, Bastoni M, Nigro A, Podini P, Finardi A, Casella G, et al. Cytokines stimulate the release of microvesicles from myeloid cells independently from the P2X7 Receptor/Acid sphingomyelinase pathway. Front Immunol. (2018) 9:204. doi: 10.3389/fimmu.2018.00204
42. Hugel B, Martínez MC, Kunzelmann C, Freyssinet JM. Membrane microparticles: two sides of the coin. Physiology (2005) 20:22–7. doi: 10.1152/physiol.00029.2004
43. Morel O, Toti F, Hugel B, Freyssinet JM. Cellular microparticles: a disseminated storage pool of bioactive vascular effectors. Curr Opin Hematol. (2004) 11:156–64. doi: 10.1097/01.moh.0000131441.10020.87
44. MacKenzie A, Wilson HL, Kiss-Toth E, Dower SK, North RA, Surprenant A. Rapid secretion of interleukin-1β by microvesicle shedding. Immunity (2001) 15:825–35. doi: 10.1016/S1074-7613(01)00229-1
45. Li J, Zhang Y, Liu Y, Dai X, Li W, Cai X, et al. Microvesicle-mediated transfer of microRNA-150 from monocytes to endothelial cells promotes angiogenesis. J Biol Chem. (2013) 288:23586–96. doi: 10.1074/jbc.M113.489302
46. Ratajczak J, Miekus K, Kucia M, Zhang J, Reca R, Dvorak P, et al. Embryonic stem cell-derived microvesicles reprogram hematopoietic progenitors: evidence for horizontal transfer of mRNA and protein delivery. Leukemia (2006) 20:847–56. doi: 10.1038/sj.leu.2404132
47. Roberts CT Jr, Kurre P. Vesicle trafficking and RNA transfer add complexity and connectivity to cell-cell communication. Cancer Res. (2013) 73:3200–5. doi: 10.1158/0008-5472.CAN-13-0265
48. Vickers KC, Remaley AT. Lipid-based carriers of microRNAs and intercellular communication. Curr Opin Lipidol. (2012) 23:91–7. doi: 10.1097/MOL.0b013e328350a425
49. Vickers KC, Palmisano BT, Shoucri BM, Shamburek RD, Remaley AT. MicroRNAs are transported in plasma and delivered to recipient cells by high-density lipoproteins. Nat Cell Biol. (2011) 13:423–33. doi: 10.1038/ncb2210
50. Gallo A, Tandon M, Alevizos I, Illei GG, The majority of microRNAs detectable in serum and saliva is concentrated in exosomes. PLoS ONE (2012) 7:e30679. doi: 10.1371/journal.pone.0030679
51. Kanada M, Bachmann MH, Hardy JW, Frimannson DO, Bronsart L, Wang A, et al. Differential fates of biomolecules delivered to target cells via extracellular vesicles. Proc Natl Acad Sci USA. (2015) 112:E1433–42. doi: 10.1073/pnas.1418401112
52. Mittelbrunn M, Gutierrez-Vazquez C, Villarroya-Beltri C, González S, Sánchez-Cabo F, González MA, et al. Unidirectional transfer ofmicroRNA-loaded exosomes from T cells to antigen-presenting cells. Nat Commun. (2011) 2:282. doi: 10.1038/ncomms1285
53. Okoye IS, Coomes SM, Pelly VS, Czieso S, Papayannopoulos V, Tolmachova, T, et al. MicroRNA-containing T-regulatory-cell-derived exosomes suppress pathogenic T helper 1 cells. Immunity (2014) 41:89–103. doi: 10.1016/j.immuni.2014.05.019
54. Hunter MP, Ismail N, Zhang X, Aguda BD, Lee EJ, Yu L, et al. Detection ofmicroRNA expression in human peripheral blood microvesicles. PLoS ONE (2008) 3:e3694. doi: 10.1371/journal.pone.0003694
55. Povero D, Eguchi A, Li H, Johnson CD, Papouchado BG, Wree A, et al. Circulating extracellular vesicles with specific proteome and livermicroRNAs are potential biomarkers for liver injury in experimental fatty liver disease. PLoS ONE (2014)9:e113651. doi: 10.1371/journal.pone.0113651
56. Zampetaki A, Kiechl S, Drozdov I, Willeit P, Mayr U, Prokopi M, et al. Plasma micro-RNA profiling reveals loss of endothelial miR-126 and other microRNAs in type 2diabetes. Circ Res. (2010) 107:810–7. doi: 10.1161/CIRCRESAHA.110.226357
57. Patz S, Trattnig C, Grünbacher G, Ebner B, Gülly C, Novak A, et al. More than cell dust: microparticles isolated from cerebrospinal fluid of brain injured patients are messengers carrying mRNAs, miRNAs, and proteins. J Neurotrauma (2013) 30:1232–42. doi: 10.1089/neu.2012.2596
58. Valadi H, Ekstrom K, Bossios A, Sjöstrand M, Lee JJ, Lötvall JO. Exosome-mediated transfer ofmRNAs and microRNAs is a novel mechanism of genetic exchange between cells. Nat Cell Biol. (2007) 9:654–9. doi: 10.1038/ncb1596
59. Huang X, Yuan T, Tschannen M, Sun Z, Jacob H, Du M, et al. Characterization of human plasma-derived exosomal RNAs by deep sequencing. BMC Genom. (2013) 14:319. doi: 10.1186/1471-2164-14-319
60. Liguori M, Nuzziello N, Licciulli F, Consiglio A, Simone M, Viterbo RG, et al. Combined microRNAs and mRNAs expression analysis in pediatric Multiple Sclerosis: an integrated approach to uncover novel pathogenic mechanisms of the disease. Hum Mol Genet. (2017) 27:66–79. doi: 10.1093/hmg/ddx385
61. Selmaj I, Cichalewska M, Namiecinska M, Galazka G, Horzelski W, Selmaj KW, et al. Global exosome transcriptome profiling reveals biomarkers for Multiple Sclerosis. Ann Neurol. (2017)81:703–17. doi: 10.1002/ana.24931
62. Vlassov AV, Magdaleno S, Setterquist R, Conrad R. Exosomes: current knowledge of their composition, biological functions, and diagnostic and therapeutic potentials. Biochim Biophys Acta (2012) 1820:940–8. doi: 10.1016/j.bbagen.2012.03.017
63. Nuzziello N, Blonda M, Licciulli F, Liuni S, Amoruso A, Valletti A, et al. Molecular characterization of peripheral extracellular vesicles in clinically isolated syndrome: preliminary suggestions from a pilot study. Med Sci. (2017) 5:E19. doi: 10.3390/medsci5030019
64. Cha DJ, Franklin JL, Dou Y, Liu Q, Higginbotham JN, Demory Beckler M, et al. KRAS-dependent sorting of miRNA to exosomes. Elife (2015) 4:e07197. doi: 10.7554/eLife.07197
65. Van Balkom BWM, Eisele ASD, Pegtel M, Bervoets S, Verhaar MC. Quantitative and qualitative analysis of small RNAs in human endothelial cells and exosomes provides insights into localized RNA processing, degradation and sorting. J Extracell Vesicles (2015) 4:26760. doi: 10.3402/jev.v4.26760
66. Ebrahimkhani S, Vafaee F, Young PE, Hur SSJ, Hawke S, Devenney E, et al. Exosomal microRNA signatures in Multiple Sclerosis reflect disease status. Sci Rep. (2017) 7:14293. doi: 10.1038/s41598-017-14301-3
67. Arraud N, Linares R, Tan S, Gounou C, Pasquet JM, Mornet S, et al. Extracellular vesicles from blood plasma: determination of their morphology, size, phenotype and concentration. J Thromb Haemost. (2014) 12:614–27. doi: 10.1111/jth.12554
Keywords: extracellular vesicles, microvesicles, exosomes, Multiple Sclerosis, biomarkers of neurodegenerative disorders
Citation: Blonda M, Amoruso A, Martino T and Avolio C (2018) New Insights Into Immune Cell-Derived Extracellular Vesicles in Multiple Sclerosis. Front. Neurol. 9:604. doi: 10.3389/fneur.2018.00604
Received: 13 March 2018; Accepted: 06 July 2018;
Published: 13 August 2018.
Edited by:
Björn Tackenberg, Philipps University of Marburg, GermanyReviewed by:
Vincent Van Pesch, Université Catholique de Louvain, BelgiumPatrick Joseph Waters, University of Oxford, United Kingdom
Copyright © 2018 Blonda, Amoruso, Martino and Avolio. This is an open-access article distributed under the terms of the Creative Commons Attribution License (CC BY). The use, distribution or reproduction in other forums is permitted, provided the original author(s) and the copyright owner(s) are credited and that the original publication in this journal is cited, in accordance with accepted academic practice. No use, distribution or reproduction is permitted which does not comply with these terms.
*Correspondence: Maria Blonda, maria.blonda@unifg.it