- 1Department of Genetic and Behavioral Neuroscience, Graduate School of Medicine, Gunma University, Maebashi, Japan
- 2Division of Molecular Science, Graduate School of Science and Technology, Gunma University, Kiryu, Japan
Major depressive disorder (MDD) is a highly prevalent psychiatric disorder worldwide. Several lines of evidence suggest that the dysfunction of somatostatin (SOM) neurons is associated with the pathophysiology of MDD. Importantly, most SOM neurons are γ-aminobutyric acid (GABA) interneurons. However, whether the dysfunction of GABAergic neurotransmission from SOM neurons contributes to the pathophysiology of MDD remains elusive. To address this issue, we investigated the emotional behaviors and relevant molecular mechanism in mice lacking glutamate decarboxylase 67 (GAD67), an isoform of GABA-synthesizing enzyme, specifically in SOM neurons (SOM-GAD67 mice). The SOM-GAD67 mice exhibited anxiety-like behavior in the open-field test without an effect on locomotor activity. The SOM-GAD67 mice showed depression-like behavior in neither the forced swimming test nor the sucrose preference test. In addition, the ability to form contextual fear memory was normal in the SOM-GAD67 mice. Furthermore, the plasma corticosterone level was normal in the SOM-GAD67 mice both under baseline and stress conditions. The expression ratios of p-AktSer473/Akt and p-GSK3βSer9/GSK3β were decreased in the frontal cortex of SOM-GAD67 mice. Taken together, these data suggest that the loss of GAD67 from SOM neurons may lead to the development of anxiety-like but not depression-like states mediated by modification of Akt/GSK3β activities.
Introduction
Major depressive disorder (MDD) affects approximately 10% of the population at some point in their life and is the leading cause of physical impairment, medical comorbidity, and mortality across the world (Penninx et al., 2013; Sato and Yeh, 2013). However, the current treatments are only partially effective, and patients fail to respond to trials with existing antidepressant agents targeting the monoaminergic systems (Fekadu et al., 2009; Kupfer et al., 2012). Clarifying the molecular biology of MDD is desired for developing innovative therapeutics.
Several lines of evidence indicate that the dysfunction of somatostatin (SOM)-expressing cells is likely associated with the pathophysiology of MDD (Fee et al., 2017). In the postmortem brain of patients with MDD, the expression levels of SOM were decreased in the dorsolateral prefrontal cortex (Sibille et al., 2011), the subgenual anterior cingulate cortex (Tripp et al., 2011) and the amygdala (Guilloux et al., 2012). In animal studies, mice subjected to chronic mild stress, an animal model of depression, demonstrated a decrease in the mRNA level of SOM in the prefrontal cortex (Banasr et al., 2017). On the other hand, mice with increased excitability in SOM neurons by deletion of the γ2-subunit of γ-aminobutyric acidA (GABAA) receptors demonstrated anti-anxiety and anti-depressive behaviors (Fuchs et al., 2017). SOM knockout (KO) mice displayed a higher response to stress in plasma corticosterone levels (Zeyda et al., 2001; Lin and Sibille, 2015; Viollet et al., 2017). SOM KO mice displayed no change in emotional behaviors (Zeyda et al., 2001; Viollet et al., 2017) or mild anxiety-like behavior (Lin and Sibille, 2015). Lin and Sibille reported the anxiety-like/depression-like behaviors were pronounced after exposure to chronic mild stress (Lin and Sibille, 2015).
Importantly, most SOM-expressing cells in the central nervous system are GABA interneurons (Kosaka et al., 1988; Kubota et al., 1994; Esclapez and Houser, 1995; Gonchar and Burkhalter, 1997; Uematsu et al., 2008). Neuroimaging studies have demonstrated a reduction in GABA levels in the brains of patients with MDD (Sanacora et al., 1999; Hasler et al., 2007). GABA is synthesized from glutamate by glutamate decarboxylase (GAD). GAD exists in two isoforms, GAD67 and GAD65, which are independently encoded by the GAD1 and GAD2 genes, respectively (Soghomonian and Martin, 1998; Ji et al., 1999). Several studies have demonstrated decreased expressions of GAD67 but not GAD65 in the postmortem brains of patients with MDD (Karolewicz et al., 2010; Scifo et al., 2018), although these changes were not observed by others (Pehrson and Sanchez, 2015). Therefore, the emotional disabilities in patients with MDD may be associated with the dysfunction of GABAergic neurotransmission from SOM neurons, which disrupts an inhibitory control to neural excitability (Fee et al., 2017). Global GAD67 KO mice show cleft palate and omphalocele, and all of them die during the first day after birth (Asada et al., 1997; Kakizaki et al., 2015). We recently developed mice with conditional KO of GAD67 specifically in parvalbumin (PV)-expressing cells (PV-GAD67 mice) or SOM-expressing cells (SOM-GAD67 mice). The PV-GAD67 mice demonstrated oscillational disturbance across cortical layers and schizophrenia-like behavioral abnormalities (Fujihara et al., 2015; Kuki et al., 2015). However, we had yet to investigate the behavioral phenotypes of the SOM-GAD67 mice. Behavioral examination of SOM-GAD67 mice is important for clarifying whether the deficiency of GAD67-mediated GABA in SOM neurons contributes to MDD-related symptoms.
Akt and glycogen synthase kinase-3 β-isoform (GSK3β) are serine/threonine protein kinases that regulate multiple cellular functions including neuroplasticity and cell survival (Descorbeth et al., 2018; Wu et al., 2018). Akt/GSK3β signaling is an important signal that regulates emotional behaviors in rodents (Sui et al., 2008; Bali and Jaggi, 2016; Pan et al., 2016; Slouzkey and Maroun, 2016). Recently, the Akt/GSK3β pathway has attracted attention in the molecular biology of MDD and as a novel target of therapeutic agents (Kitagishi et al., 2012). Interestingly, GABA signaling affects Akt/GSK3β activities (Lu et al., 2012). Therefore, the functional alteration of SOM-expressing GABA neurons may affect Akt/GSK3β activities in the brain.
The aim of this study was to resolve the role of GAD67 in SOM neurons on emotional regulation using SOM-GAD67 mice. We also examined the plasma corticosterone levels and the expression levels of Akt and GSK3β proteins, which are relevant molecules to the pathophysiology of MDD.
Materials and Methods
Ethics Statement
This study was performed in accordance with the Guidelines for Animal Experimentation at Gunma University Graduate School of Medicine and was approved by the Gunma University Ethics Committee (Permit number: 14-006). Every effort was made to minimize the number of animals used and their suffering.
Animals
We previously reported the generation of SOM-GAD67 mice (Kuki et al., 2015). Briefly, SOM-IRES-Cre mice (Taniguchi et al., 2011) were obtained from Jackson Laboratories (Bar Harbor, ME, USA; Stock No: 028864), and GAD67-floxed mice were previously described (Obata et al., 2008; Fujihara et al., 2015). SOMIRES−Cre/+;GAD67flox/flox mice (SOM-GAD67 mice) were obtained by crossing female GAD67flox/flox mice and male SOMIRES−Cre/+;GAD67flox/+ mice. The littermate GAD67flox/flox mice were used as the control. We only used male mice from 8 weeks to 16 weeks of age for the behavioral tests, enzyme immunoassay and western blottings. The animals were housed with 2–3 mice per cage [16.5 × 27 × 12.5 (H) cm] and had free access to food and water. The animal rooms for breeding and experiments were maintained at 22 ± 3°C with a 12-h light-dark cycle (lights on at 6:00, lights off at 18:00). The animals were used only once.
Genotyping
Genotyping of the transgenic mice was performed by PCR using tail genomic DNA. The primer sequences were as follows: Cre allele, 5′-GTCTCTGGTGTAGCTGATGATCCGAA-3′ and 5′-CCCTGTTTCACTATCCAGGTTACGGA-3′; GAD67 allele, 5′-ACCTTGGCAGCTAACTAGGAGGA-3′ and 5′-ACAGATCGGATGGGGAAGCATAA-3′. The lengths of the amplified DNA fragments were as follows: Cre allele, 321 bp; GAD67 allele, 155 bp; loxP-inserted GAD67 allele, 258 bp.
Open-Field Test
Each mouse was placed in the center of an open-field apparatus [50 cm × 50 cm × 40 (H) cm] that was illuminated by light-emitting diodes (30 lux at the center of the field) and allowed to move freely for 5 min. The time spent in the central area of the field (36% of the field) was recorded as the index of interest. The data were collected and analyzed using ImageJ OF4 (O’Hara & Co., Ltd., Tokyo, Japan), which is a modified software that is also based on the public domain ImageJ program. The procedure was referenced to our previous report (Miyata et al., 2016).
Contextual Conditioned Fear Test
Training and testing took place in a chamber [10 × 10 × 12 (H) cm] equipped with a grid floor placed in an acoustic box. The grid floor was wired to an isolated shock generator (MSG-001, Toyo Sangyo Co. Ltd., Japan). The experiments were conducted over the course of two consecutive days. On day 1 (training session), mice were individually placed in the chamber and received scrambled foot-shocks (either 0.2 mA or 0.4 mA, 2 s) at pseudo-random times; 2.5, 5, 9 and 11.5 min, after the start of the session. Thirty seconds after the last foot shock, the mice were returned to the home cage. Twenty-four hours later, the mice were placed in the same chamber as on day 1 for 6 min without foot-shock exposure (test session). Background white-noise (55 dB) was presented during both the training and the test sessions. The protocol was based on the first two consecutive days of Lattal’s method (Lattal et al., 2007) to test contextual fear conditioning. Mouse behavior was recorded and analyzed using Time FZ1 for Contextual and Cued Fear Conditioning Test software (O’Hara & Co., Ltd.). The percentage of duration of freezing behavior in the test session was calculated and compared between the genotypes.
Forced Swimming Test
Each mouse was placed in an acrylic cylinder (22 cm in height, 11.5 cm in diameter) containing 15 cm of water at room temperature (22 ± 3°C). The cylinder was placed in an isolation box. The behavior of each mouse was recorded for 6 min using a CCD camera connected to a personal computer and analyzed using ImageJ PS1 (O’Hara & Co., Ltd.), which is a modified software package that is based on the public domain ImageJ program (developed at the U.S. National Institutes of Health and available at: http://rsb.info.nih.gov/ij). The procedure was the same as that referenced in our previous report (Miyata et al., 2016). The percentage of time spent immobile during the 6-min period was calculated and compared between the genotypes.
Sucrose Preference Test
Sucrose preference test is a well-accepted behavioral test measuring an anhedonia-like state of mice and rats (Katz, 1982; Willner, 1997). Mice preferentially take sweet-taste solution compared with water. The sweet-taste preference disappears in model mice of depression, such as mice subjected to chronic mild stress. This behavioral phenotype disappears by sub-chronic treatment with antidepressant agents (Willner et al., 1987).
One week before the measurement, the mice were provided 2% sucrose solution in a drinking bottle for 24 h to habituate to sweet taste. Each mouse was subjected to water deprivation for 16 h before starting the measurement. Mice were transferred to an individual cage [16.5 × 27 × 12.5 (H) cm], and then two preweighted bottles (one containing tap water and another containing 2% sucrose solution) were presented to each mouse for 4 h. The bottles were weighed again, and the weight difference was considered to be the mouse intake from each bottle. The sum of water and sucrose intake was defined as the total intake, and sucrose preference was expressed as the percentage of sucrose intake from total intake.
Plasma Corticosterone
Blood samples were obtained from the tail vein by a small incision. Immediately after the initial sampling, the mice were restrained in 50-mL Falcon® tubes with air vents for 120 min. The blood samples were collected at 15 and 120 min during the restraint stress. After the cessation of restraint stress, the mice were returned to their home cage. Sixty minutes later, the final blood sampling was conducted. The blood samples were centrifuged at 1,000 g for 10 min at 4°C, and the plasma samples were collected and stored at −80°C until analysis. Blood sampling was conducted between 9:00 and 13:00 on the day of the experiment.
Plasma corticosterone concentrations were determined using a commercially available enzyme immunoassay kit (Enzo Life Sciences, Inc., Farmingdale, NY, USA) following the manufacturer’s instructions.
Western Blot
The mice were killed by decapitation. The frontal cortex (FCx) was quickly dissected on an ice-cold stainless plate, immediately frozen in liquid nitrogen and stored at −80°C until use. The dissection was performed according to the Chiu’s study (Chiu et al., 2007). The tissues were homogenized in ice-cold buffered sucrose (0.32 M) solution containing 20 mM Tris-HCl (pH 7.5), protease inhibitor cocktail (P8340, Sigma-Aldrich, Inc.) and phosphatase inhibitor cocktail (07575-51, Nacalai Tesque, Inc.). The homogenates were centrifuged at 1,000 g for 10 min at 4°C, and the supernatants were collected as the protein samples (S1 fraction). The protein concentrations were determined using a TaKaRa BCA Protein Assay Kit (T9300A, Takara Bio Inc., Japan).
The protein samples were diluted with electrophoresis sample buffer. Proteins (15 μg) were separated by SDS-polyacrylamide gels and transferred to a PVDF membrane. Blots were probed with antibodies to Akt (pan; 1:1,000, #4691, Cell Signaling Technology Japan, K.K.), phospho-Akt (Ser473; 1:2,000, #4060, Cell Signaling Technology, Danvers, MA, USA), phospho-Akt (Thr308; 1:1,000, #13038, Cell Signaling Technology, Danvers, MA, USA), GSK3β (1:1,000, #9832, Cell Signaling Technology, Danvers, MA, USA), phospho-GSK3β (Ser9; 1:1,000, #5558, Cell Signaling Technology, Danvers, MA, USA) and β-actin (1:4,000, M177-3, Medical and Biological Laboratories Co. Ltd., Japan). Immunoblots were developed using horseradish peroxidase-conjugated secondary antibodies (GE Healthcare) and then detected with chemiluminescence reagents (ECL prime, GE Healthcare) and visualized by an Light Capture AE-9672 (ATTO Co., Ltd.). The density of the bands was determined using ImageJ software.
The Akt and GSK3β activities were assessed by calculating the ratio of the band densities of phosphorylated/total proteins. The band densities of β-actin were used as the loading control.
Data Analysis
Statistical analyses were conducted using BellCurve for Excel ver. 2.12 (Social Survey Research Information Co., Ltd., Tokyo, Japan). Significant differences between two groups were evaluated by Student’s t-test. Significant differences among the multiple groups were analyzed by one-way and two-way analysis of variance (ANOVA) with a Bonferroni multiple comparison test. Statistical significance was defined as a p-value less than 0.05. The data were expressed as means ± SE.
Results
Behavioral Phenotypes of SOM-GAD67 Mice
To assess the anxiety-like state of mice, we performed the open-field test. The SOM-GAD67 mice exhibited significantly less time spent in the center field than the control mice (Figures 1A,C), but the total path length in the open-field test did not differ between the genotypes (Figures 1B,C).
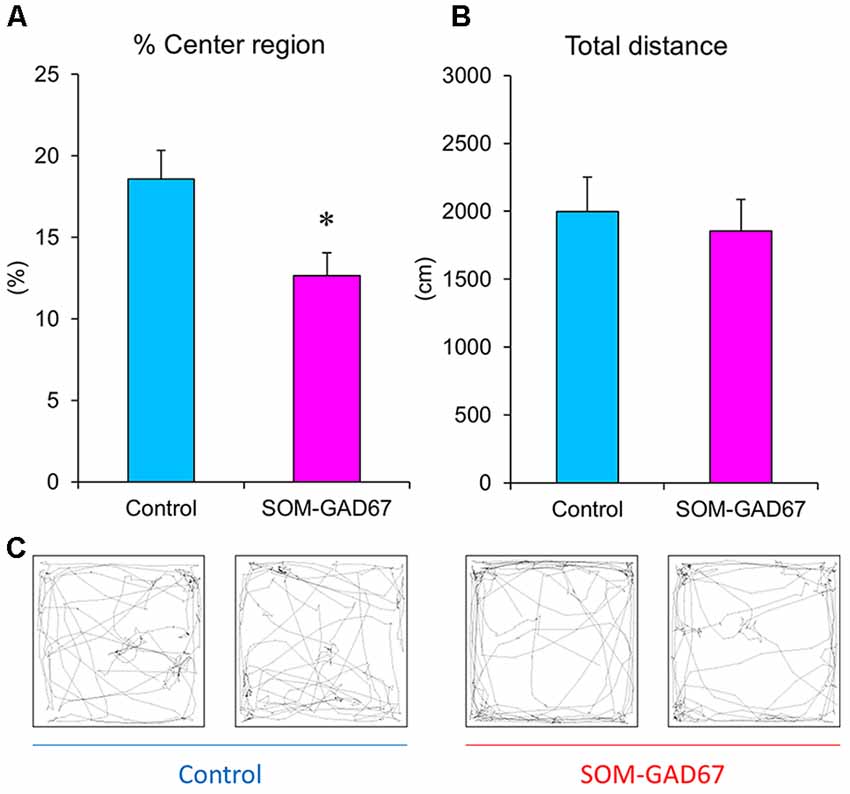
Figure 1. Exploratory behaviors of the somatostatin (SOM)-glutamate decarboxylase 67 (GAD67) mice (n = 8) and control mice (n = 8) in the open-field test. The percent exploration of the center field (A) and total path length (B) for 5 min. (C) Illustrative examples of the travel pathway in two control and two SOM-GAD67 mice. Data represent the means + SE. *p < 0.05 vs. the control mice (Student’s t-test).
Next, we evaluated the ability to form fear memory in mice in the contextual conditioned fear test. The duration of freezing behavior observed in the test session was not significantly different between the genotypes (Figure 2).
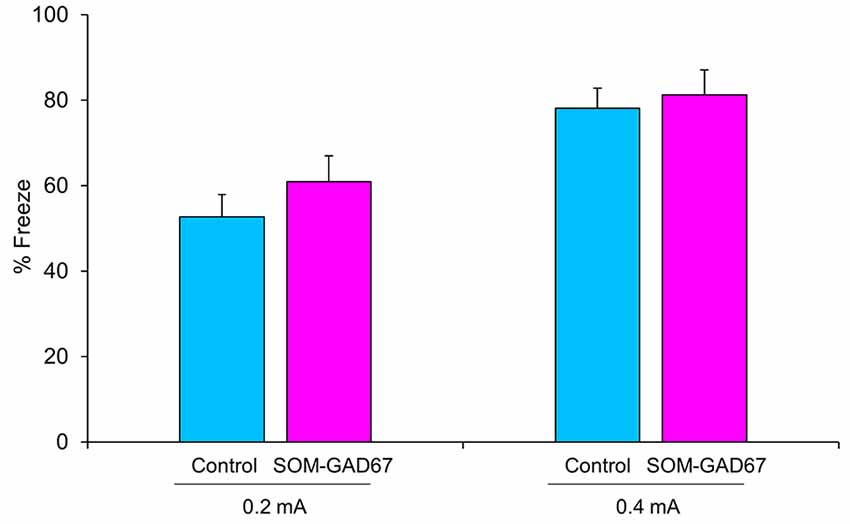
Figure 2. Freezing behavior of the SOM-GAD67 mice and control mice in the contextual conditioned fear test. The mice were subjected to either 0.2 mA (SOM-GAD67; n = 7, control; n = 7) or 0.4 mA (SOM-GAD67; n = 6, control; n = 4) foot-shocks in the conditioning session. Twenty-four hours later, the mice were returned to the same chamber, and freezing behavior was measured for 6 min without foot-shock presentation. The percent duration of freezing is shown. Data represent the means + SE.
We further evaluated the depression-like state in mice in the forced swimming test and the sucrose preference test. In the forced swimming test, the duration of immobility was not significantly different between the genotypes (Figure 3A). In addition, there was also no difference in sucrose preference between the genotypes (Figure 3B).
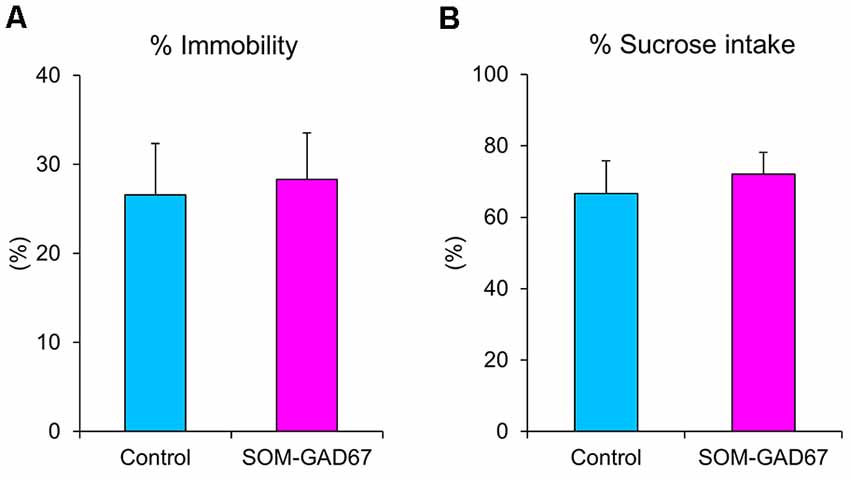
Figure 3. Depression-like behaviors of the SOM-GAD67 mice and control mice in the forced swimming test and the sucrose preference test. (A) The percentage of time spent immobile in the forced swimming test is shown (SOM-GAD67; n = 9, control; n = 9). (B) The percent intake of sucrose in the sucrose preference test is shown (SOM-GAD67; n = 8, control; n = 7). Data represent the means + SE.
Stress Reactivity in SOM-GAD67 Mice
To assess the reactivity to stress, we measured the plasma corticosterone levels in mice under baseline conditions and during a stressful condition. Under baseline, there was no difference in the plasma corticosterone levels between the genotypes. Under conditions of restraint stress, the plasma corticosterone levels were increased in both genotypes, but the elevated plasma corticosterone levels were similar between the genotypes. One hour after cessation of the stress, the plasma corticosterone levels had decreased in both genotypes, but there were no differences in the plasma corticosterone levels between the genotypes (Figure 4).
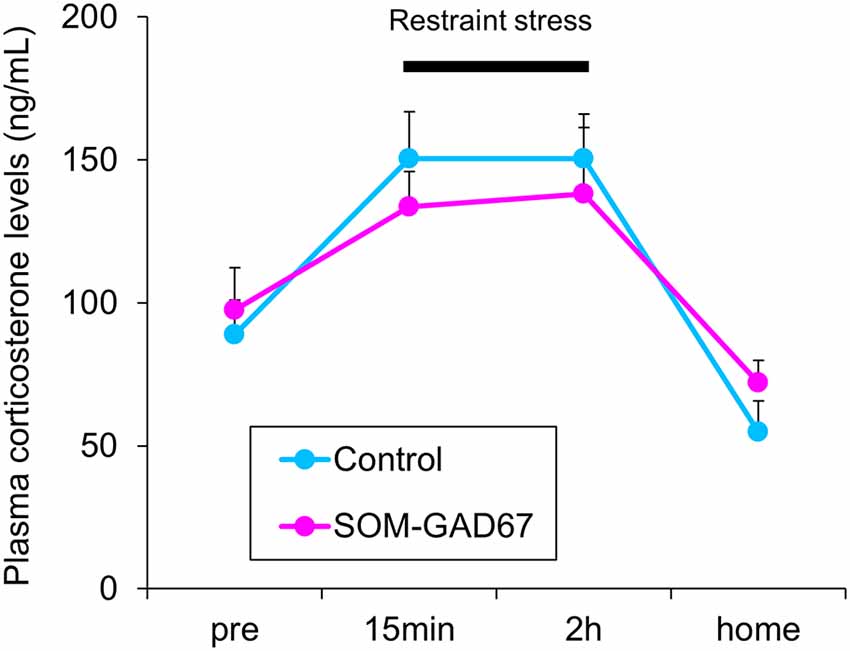
Figure 4. Plasma corticosterone levels in the SOM-GAD67 mice (n = 7) and control mice (n = 7). After the first blood sample collection (pre), the mice were subjected to restraint stress for 120 min. The blood was collected 15 min and 120 min after the beginning of the stress exposure. After the cessation of the stress exposure, the mice were returned to their home cage, and 60 min later, the final blood sample was collected. The plasma corticosterone levels were determined by the enzyme immunoassay kit. Data represent the means + SE.
Cortical Akt/GSK3β Signaling in SOM-GAD67 Mice
To assess the link between Akt/GSK3β signaling and anxiety-like behavior in SOM-GAD67 mice, we examined the expression levels of Akt and GSK3β by Western blotting and calculated the expression ratio of phosphorylated forms/total proteins. The expression ratio of the p-AktSer473/Akt protein was significantly lower in the FCx of the SOM-GAD67 mice than in that of the control mice (Figure 5). On the other hand, the expression ratio of the p-AktThr308/Akt protein was not different between the genotypes. The expression ratio of p-GSK3βSer9/GSK-3β protein was significantly lower in the SOM-GAD67 mice than in the control mice (Figure 5).
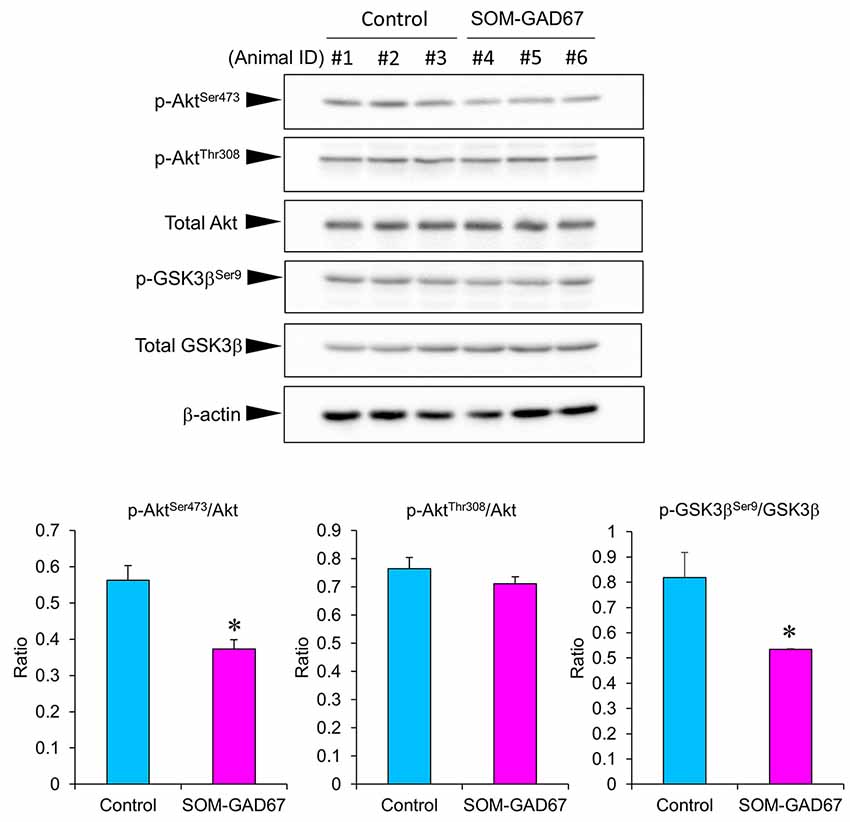
Figure 5. Protein levels of Akt and glycogen synthase kinase-3 β (GSK3β) in the frontal cortex of the SOM-GAD67 mice (n = 3) and control mice (n = 3). The band densities were determined using ImageJ software. The expression ratio of phosphorylated/total proteins was compared between the genotypes. Data represent the means + SE. *p < 0.05 vs. the control mice (Student’s t-test).
Discussion
In the current study, SOM-GAD67 mice exhibited behavioral abnormalities in the open-field test but no effects on locomotor activity. The reduction in exploration of the center region of the open field is accepted as anxiety-like behavior in rodents (Parks et al., 1998). Therefore, the deletion of GAD67 from SOM-expressing GABA neurons induced an anxiogenic-like effect in mice. In the contextual conditioned fear test, the duration of freezing was not different between the genotypes, indicating that the ability to form contextual fear memory is normal in SOM-GAD67 mice. Unexpectedly, the SOM-GAD67 mice showed depression-like behavior in neither the forced swimming test nor the sucrose preference test. The forced swimming test and the sucrose (or saccharine) preference test are accepted as animal models of depression and screening methods for antidepressant agents (Porsolt et al., 1977; Katz, 1982). Therefore, the deletion of GAD67 from SOM-expressing GABA neurons had no effect on the depression-like state in mice. Based on the current behavioral studies, we suggest that GAD67 in SOM neurons mainly regulates the anxiety-like state in mice.
In clinical studies, elevated concentrations of cortisol, the end product of the hypothalamic-pituitary-adrenal axis, are observed in the blood of patients with MDD (Schlesser et al., 1980). In addition, the blood concentrations of corticosterone, the major glucocorticoid in rodents, are increased in animal models of depression (Marcilhac et al., 1999; Miyata et al., 2004; Kubera et al., 2013; Iñiguez et al., 2014). We measured the plasma levels of corticosterone in the SOM-GAD67 mice before, during and after the restraint stress. Both genotypes demonstrated a stress-elicited increase in plasma corticosterone levels, but there was no difference between the genotypes at any time period measured. The results indicate that deletion of GAD67 from SOM neurons is not sufficient to affect the reactivity of the hypothalamic-pituitary-adrenal axis. SOM KO mice have been reported to exhibit an enhanced response to stress in plasma corticosterone levels (Zeyda et al., 2001; Lin and Sibille, 2015; Viollet et al., 2017). The hormonal reaction in the current SOM-GAD67 mice was different from that in SOM KO mice. SOM is coreleased with GABA to inhibit excitatory synaptic transmission (Martel et al., 2012). These findings indicate that the effects of SOM and GABA released from SOM neurons on the reactivity of the hypothalamic-pituitary-adrenal axis may be different.
Akt and GSK3β are serine/threonine protein kinases that regulate multiple cellular functions, including neuroplasticity and cell survival (Descorbeth et al., 2018; Wu et al., 2018). The phosphorylation of Akt at the Thr308 and Ser473 sites is needed for its full activation (Bellacosa et al., 1998). Akt at Thr308 is phosphorylated by phosphoinositide-dependent protein kinase-1 (Song et al., 2005). Akt at Ser473 is autophosphorylated or phosphorylated by mechanistic target of rapamycin complex-2 (Toker and Newton, 2000; Jacinto et al., 2006). Akt phosphorylates GSK3β at Ser9 and inhibits its kinase activity (Sutherland et al., 1993; Cross et al., 1995). Decreased activity of Akt and increased activity of GSK3β have been found in the prefrontal and occipital cortex of suicide victims with depressive disorder (Hsiung et al., 2003; Karege et al., 2007, 2011). In addition, Akt/GSK3β signaling is associated with the treatment responses of therapeutic agents of mental illness (Beaulieu et al., 2009; Kim et al., 2009; Zhang et al., 2010; Kitagishi et al., 2012; Costemale-Lacoste et al., 2016). In animal studies, mice lacking Akt2, an isoform of Akt, exhibited anxiety-like and depression-like behaviors (Leibrock et al., 2013). The expression levels of p-AktSer473 and the p-AktSer473/Akt ratio were decreased in the hippocampus in animal models of depression (Xia et al., 2016; Wu et al., 2017). In addition, the expression level of p-GSK3βSer9 in the frontal cortex was decreased in an animal model of depression (Szymańska et al., 2009). Treatments with antidepressant agents normalized these alterations in p-AktSer473 and p-GSK3βSer9 expression in the prefrontal cortex and the hippocampus of those models (Xia et al., 2016; Szymańska et al., 2009; Wu et al., 2017). Furthermore, treatment with a GSK3β inhibitor ameliorated stress-elicited anxiety-like behavior in mice (Bali and Jaggi, 2017). In contrast, treatment with an Akt inhibitor interfered with the neuroprotective and anxiolytic-like effects of therapeutic agents (Pei et al., 2016). These findings indicate that the decreased activity of Akt and the increased activity of GSK3β contribute to the development of anxiety-like and depression-like states in rodents. In the current study, SOM-GAD67 mice demonstrated reductions in the p-AktSer473/Akt ratio and p-GSK3βSer9/GSK3β ratios in the FCx, indicating that Akt kinase activity is decreased and GSK3β kinase activity is increased in the FCx of SOM-GAD67 mice, which are similar to the findings in the postmortem brain of depressive patients. The details of the mechanisms by which GAD67 in SOM neurons regulates Akt/GSK3β activity are still unclear, but impairment of Akt/GSK3β signaling may be associated with the development of an anxiety-like state in SOM-GAD67 mice. Further studies are needed to resolve this relationship.
In this study, SOM-GAD67 mice demonstrated anxiety-like behavior but not depression-like behavior. In psychiatric examinations, subsyndromal anxiety is often comorbid in patients with MDD (Zimmerman et al., 2000; Zbozinek et al., 2012; Steenkamp et al., 2017). Therefore, dysfunction of GAD67 in SOM neurons might be associated with subsyndromal anxiety but not depressive symptoms in patients with MDD.
Conclusion
GAD67 in SOM neurons regulates the anxiety-like state in mice mediated by the modification of cortical Akt/GSK3β activity.
Data Availability
All datasets generated for this study are included in the manuscript.
Ethics Statement
This study was performed in accordance with the Guidelines for Animal Experimentation at Gunma University Graduate School of Medicine and was approved by the Gunma University Ethics Committee (Permit number: 14-006).
Author Contributions
YY supervised the research. SM designed the experimental protocol, conducted the data analysis, interpretation and wrote the first draft of the manuscript. SM, RK and TK carried out animal studies including animal care. KF and KW provided critical suggestions for the research. All authors contributed to and approved the final manuscript.
Funding
This work was supported by Japan Society for the Promotion of Science (JSPS) KAKENHI Grant Number 26290002, 15H05872, 17H05550 and 17K17628 and Takeda Science Foundation.
Conflict of Interest Statement
The authors declare that the research was conducted in the absence of any commercial or financial relationships that could be construed as a potential conflict of interest.
Acknowledgments
We thank Dr. Kunihiko Obata for providing GAD67-floxed mice and his encouragement. We also thank the staff at Bioresource Center, Gunma University Graduate School of Medicine for technical support.
References
Asada, H., Kawamura, Y., Maruyama, K., Kume, H., Ding, R. G., Kanbara, N., et al. (1997). Cleft palate and decreased brain γ-aminobutyric acid in mice lacking the 67-kDa isoform of glutamic acid decarboxylase. Proc. Natl. Acad. Sci. U S A 94, 6496–6499. doi: 10.1073/pnas.94.12.6496
Bali, A., and Jaggi, A. S. (2016). Investigations on GSK-3β/NF-kB signaling in stress and stress adaptive behavior in electric foot shock subjected mice. Behav. Brain Res. 302, 1–10. doi: 10.1016/j.bbr.2016.01.014
Bali, A., and Jaggi, A. S. (2017). Anti-stress effects of a GSK-3β inhibitor, AR-A014418, in immobilization stress of variable duration in mice. J. Basic Clin. Physiol. Pharmacol. 28, 315–325. doi: 10.1515/jbcpp-2016-0157
Banasr, M., Lepack, A., Fee, C., Duric, V., Maldonado-Aviles, J., DiLeone, R., et al. (2017). Characterization of GABAergic marker expression in the chronic unpredictable stress model of depression. Chronic Stress 1, 1–13. doi: 10.1177/2470547017720459
Beaulieu, J.-M., Gainetdinov, R. R., and Caron, M. G. (2009). Akt/GSK3 signaling in the action of psychotropic drugs. Annu. Rev. Pharmacol. Toxicol. 49, 327–347. doi: 10.1146/annurev.pharmtox.011008.145634
Bellacosa, A., Chan, T. O., Ahmed, N. N., Datta, K., Malstrom, S., Stokoe, D., et al. (1998). Akt activation by growth factors is a multiple-step process: the role of the PH domain. Oncogene 17, 313–325. doi: 10.1038/sj.onc.1201947
Chiu, K., Lau, W. M., Lau, H. T., So, K.-F., and Chang, R. C.-C. (2007). Micro-dissection of rat brain for RNA or protein extraction from specific brain region. J. Vis. Exp. 7:269. doi: 10.3791/269
Costemale-Lacoste, J. F., Guilloux, J. P., and Gaillard, R. (2016). The role of GSK-3 in treatment-resistant depression and links with the pharmacological effects of lithium and ketamine: a review of the literature. Encephale 42, 156–164. doi: 10.1016/j.encep.2016.02.003
Cross, D. A. E., Alessi, D. R., Cohen, P., Andjelkovich, M., and Hemmings, B. A. (1995). Inhibition of glycogen synthase kinase-3 by insulin mediated by protein kinase B. Nature 378, 785–789. doi: 10.1038/378785a0
Descorbeth, M., Figueroa, K., Serrano-Illán, M., and De León, M. (2018). Protective effect of docosahexaenoic acid on lipotoxicity-mediated cell death in Schwann cells: implication of PI3K/AKT and mTORC2 pathways. Brain Behav. 8:e01123. doi: 10.1002/brb3.1123
Esclapez, M., and Houser, C. R. (1995). Somatostatin neurons are a subpopulation of GABA neurons in the rat dentate gyrus: evidence from colocalization of pre-prosomatostatin and glutamate decarboxylase messenger RNAs. Neuroscience 64, 339–355. doi: 10.1016/0306-4522(94)00406-u
Fee, C., Banasr, M., and Sibille, E. (2017). Somatostatin-positive gamma-aminobutyric acid interneuron deficits in depression: cortical microcircuit and therapeutic perspectives. Biol. Psychiatry 82, 549–559. doi: 10.1016/j.biopsych.2017.05.024
Fekadu, A., Wooderson, S. C., Markopoulo, K., Donaldson, C., Papadopoulos, A., and Cleare, A. J. (2009). What happens to patients with treatment-resistant depression? A systematic review of medium to long term outcome studies. J. Affect. Disord. 116, 4–11. doi: 10.1016/j.jad.2008.10.014
Fuchs, T., Jefferson, S. J., Hooper, A., Yee, P.-H., Maguire, J., and Luscher, B. (2017). Disinhibition of somatostatin-positive GABAergic interneurons results in an anxiolytic and antidepressant-like brain state. Mol. Psychiatry 22, 920–930. doi: 10.1038/mp.2016.188
Fujihara, K., Miwa, H., Kakizaki, T., Kaneko, R., Mikuni, M., Tanahira, C., et al. (2015). Glutamate decarboxylase 67 deficiency in a subset of GABAergic neurons induces schizophrenia-related phenotypes. Neuropsychopharmacology 40, 2475–2486. doi: 10.1038/npp.2015.117
Gonchar, Y., and Burkhalter, A. (1997). Three distinct families of GABAergic neurons in rat visual cortex. Cereb. Cortex 7, 347–358. doi: 10.1093/cercor/7.4.347
Guilloux, J.-P., Douillard-Guilloux, G., Kota, R., Wang, X., Gardier, A. M., Martinowich, K., et al. (2012). Molecular evidence for BDNF- and GABA-related dysfunctions in the amygdala of female subjects with major depression. Mol. Psychiatry 17, 1130–1142. doi: 10.1038/mp.2011.113
Hasler, G., van der Veen, J. W., Tumonis, T., Meyers, N., Shen, J., and Drevets, W. C. (2007). Reduced prefrontal glutamate/glutamine and γ-aminobutyric acid levels in major depression determined using proton magnetic resonance spectroscopy. Arch. Gen. Psychiatry 64, 193–200. doi: 10.1001/archpsyc.64.2.193
Hsiung, S. C., Adlersberg, M., Arango, V., Mann, J. J., Tamir, H., and Liu, K. P. (2003). Attenuated 5-HT1A receptor signaling in brains of suicide victims: involvement of adenylyl cyclase, phosphatidylinositol 3-kinase, Akt and mitogen-activated protein kinase. J. Neurochem. 87, 182–194. doi: 10.1046/j.1471-4159.2003.01987.x
Iñiguez, S. D., Riggs, L. M., Nieto, S. J., Dayrit, G., Zamora, N. N., Shawhan, K. L., et al. (2014). Social defeat stress induces a depression-like phenotype in adolescent male c57BL/6 mice. Stress 17, 247–255. doi: 10.3109/10253890.2014.910650
Jacinto, E., Facchinetti, V., Liu, D., Soto, N., Wei, S., Jung, S. Y., et al. (2006). SIN1/MIP1 maintains rictor-mTOR complex integrity and regulates Akt phosphorylation and substrate specificity. Cell 127, 125–137. doi: 10.1016/j.cell.2006.08.033
Ji, F., Kanbara, N., and Obata, K. (1999). GABA and histogenesis in fetal and neonatal mouse brain lacking both the isoforms of glutamic acid decarboxylase. Neurosci. Res. 33, 187–194. doi: 10.1016/s0168-0102(99)00011-5
Kakizaki, T., Oriuchi, N., and Yanagawa, Y. (2015). GAD65/GAD67 double knockout mice exhibit intermediate severity in both cleft palate and omphalocele compared with GAD67 knockout and VGAT knockout mice. Neuroscience 288, 86–93. doi: 10.1016/j.neuroscience.2014.12.030
Karege, F., Perroud, N., Burkhardt, S., Fernandez, R., Ballmann, E., La Harpe, R., et al. (2011). Alterations in phosphatidylinositol 3-kinase activity and PTEN phosphatase in the prefrontal cortex of depressed suicide victims. Neuropsychobiology 63, 224–231. doi: 10.1159/000322145
Karege, F., Perroud, N., Burkhardt, S., Schwald, M., Ballmann, E., La Harpe, R., et al. (2007). Alteration in kinase activity but not in protein levels of protein kinase B and glycogen synthase kinase-3β in ventral prefrontal cortex of depressed suicide victims. Biol. Psychiatry 61, 240–245. doi: 10.1016/j.biopsych.2006.04.036
Karolewicz, B., Maciag, D., O’Dwyer, G., Stockmeier, C. A., Feyissa, A. M., and Rajkowska, G. (2010). Reduced level of glutamic acid decarboxylase-67 kDa in the prefrontal cortex in major depression. Int. J. Neuropsychopharmacol. 13, 411–420. doi: 10.1017/s1461145709990587
Katz, R. J. (1982). Animal model of depression: pharmacological sensitivity of a hedonic deficit. Pharmacol. Biochem. Behav. 16, 965–968. doi: 10.1016/0091-3057(82)90053-3
Kim, J. Y., Duan, X., Liu, C. Y., Jang, M.-H., Guo, J. U., Pow-anpongkul, N., et al. (2009). DISC1 regulates new neuron development in the adult brain via modulation of AKT-mTOR signaling through KIAA1212. Neuron 63, 761–773. doi: 10.1016/j.neuron.2009.08.008
Kitagishi, Y., Kobayashi, M., Kikuta, K., and Matsuda, S. (2012). Roles of PI3K/AKT/GSK3/mTOR pathway in cell signaling of mental illnesses. Depress. Res. Treat. 2012:752563. doi: 10.1155/2012/752563
Kosaka, T., Wu, J. Y., and Benoit, R. (1988). GABAergic neurons containing somatostatin-like immunoreactivity in the rat hippocampus and dentate gyrus. Exp. Brain Res. 71, 388–398. doi: 10.1007/bf00247498
Kubera, M., Curzytek, K., Duda, W., Leskiewicz, M., Basta-Kaim, A., Budziszewska, B., et al. (2013). A new animal model of (chronic) depression induced by repeated and intermittent lipopolysaccharide administration for 4 months. Brain. Behav. Immun. 31, 96–104. doi: 10.1016/j.bbi.2013.01.001
Kubota, Y., Hattori, R., and Yui, Y. (1994). Three distinct subpopulations of GABAergic neurons in rat frontal agranular cortex. Brain Res. 649, 159–173. doi: 10.1016/0006-8993(94)91060-x
Kuki, T., Fujihara, K., Miwa, H., Tamamaki, N., Yanagawa, Y., and Mushiake, H. (2015). Contribution of parvalbumin and somatostatin-expressing GABAergic neurons to slow oscillations and the balance in beta-gamma oscillations across cortical layers. Front. Neural Circuits 9:6. doi: 10.3389/fncir.2015.00006
Kupfer, D. J., Frank, E., and Phillips, M. L. (2012). Major depressive disorder: new clinical, neurobiological, and treatment perspectives. Lancet 379, 1045–1055. doi: 10.1016/S0140-6736(11)60602-8
Lattal, K. M., Barrett, R. M., and Wood, M. A. (2007). Systemic or intrahippocampal delivery of histone deacetylase inhibitors facilitates fear extinction. Behav. Neurosci. 121, 1125–1131. doi: 10.1037/0735-7044.121.5.1125
Leibrock, C., Ackermann, T. F., Hierlmeier, M., Lang, F., Borgwardt, S., and Lang, U. E. (2013). Akt2 deficiency is associated with anxiety and depressive behavior in mice. Cell. Physiol. Biochem. 32, 766–777. doi: 10.1159/000354478
Lin, L. C., and Sibille, E. (2015). Somatostatin, neuronal vulnerability and behavioral emotionality. Mol. Psychiatry 20, 377–387. doi: 10.1038/mp.2014.184
Lu, F. F., Su, P., Liu, F., and Daskalakis, Z. J. (2012). Activation of GABAB receptors inhibits protein kinase B/glycogen synthase kinase 3 signaling. Mol. Brain 5:41. doi: 10.1186/1756-6606-5-41
Marcilhac, A., Faudon, M., Anglade, G., Hery, F., and Siaud, P. (1999). An investigation of serotonergic involvement in the regulation of ACTH and corticosterone in the olfactory bulbectomized rat. Pharmacol. Biochem. Behav. 63, 599–605. doi: 10.1016/s0091-3057(99)00024-6
Martel, G., Dutar, P., Epelbaum, J., and Viollet, C. (2012). Somatostatinergic systems: an update on brain functions in normal and pathological aging. Front. Endocrinol. 3:154. doi: 10.3389/fendo.2012.00154
Miyata, S., Hirano, S., and Kamei, J. (2004). Diabetes attenuates the antidepressant-like effect mediated by the activation of 5-HT1A receptor in the mouse tail suspension test. Neuropsychopharmacology 29, 461–469. doi: 10.1038/sj.npp.1300354
Miyata, S., Kurachi, M., Okano, Y., Sakurai, N., Kobayashi, A., Harada, K., et al. (2016). Blood transcriptomic markers in patients with late-onset major depressive disorder. PLoS One 11:e0150262. doi: 10.1371/journal.pone.0150262
Obata, K., Hirono, M., Kume, N., Kawaguchi, Y., Itohara, S., and Yanagawa, Y. (2008). GABA and synaptic inhibition of mouse cerebellum lacking glutamate decarboxylase 67. Biochem. Biophys. Res. Commun. 370, 429–433. doi: 10.1016/j.bbrc.2008.03.110
Pan, B., Huang, X.-F., and Deng, C. (2016). Chronic administration of aripiprazole activates GSK3β-dependent signalling pathways and up-regulates GABAA receptor expression and CREB1 activity in rats. Sci. Rep. 6:30040. doi: 10.1038/srep30040
Parks, C. L., Robinson, P. S., Sibille, E., Shenk, T., and Toth, M. (1998). Increased anxiety of mice lacking the serotonin1A receptor. Proc. Natl. Acad. Sci. U S A 95, 10734–10739. doi: 10.1073/pnas.95.18.10734
Pehrson, A. L., and Sanchez, C. (2015). Altered γ-aminobutyric acid neurotransmission in major depressive disorder: a critical review of the supporting evidence and the influence of serotonergic antidepressants. Drug Des. Devel. Ther. 9, 603–624. doi: 10.2147/DDDT.S62912
Pei, B., Yang, M., Qi, X., Shen, X., Chen, X., and Zhang, F. (2016). Quercetin ameliorates ischemia/reperfusion-induced cognitive deficits by inhibiting ASK1/JNK3/caspase-3 by enhancing the Akt signaling pathway. Biochem. Biophys. Res. Commun. 478, 199–205. doi: 10.1016/j.bbrc.2016.07.068
Penninx, B. W. J. H., Milaneschi, Y., Lamers, F., and Vogelzangs, N. (2013). Understanding the somatic consequences of depression: biological mechanisms and the role of depression symptom profile. BMC Med. 11:129. doi: 10.1186/1741-7015-11-129
Porsolt, R. D., Bertin, A., and Jalfre, M. (1977). Behavioral despair in mice: a primary screening test for antidepressants. Arch. Int. Pharmacodyn. Ther. 229, 327–336.
Sanacora, G., Mason, G. F., Rothman, D. L., Behar, K. L., Hyder, F., Petroff, O. A., et al. (1999). Reduced cortical γ-aminobutyric acid levels in depressed patients determined by proton magnetic resonance spectroscopy. Arch. Gen. Psychiatry 56, 1043–1047. doi: 10.1001/archpsyc.56.11.1043
Sato, S., and Yeh, T. L. (2013). Challenges in treating patients with major depressive disorder: the impact of biological and social factors. CNS Drugs 27, S5–S10. doi: 10.1007/s40263-012-0028-8
Schlesser, M. A., Winokur, G., and Sherman, B. M. (1980). Hypothalamic-pituitary-adrenal axis activity in depressive illness. Its relationship to classification. Arch. Gen. Psychiatry 37, 737–743. doi: 10.1001/archpsyc.1980.01780200015001
Scifo, E., Pabba, M., Kapadia, F., Ma, T., Lewis, D. A., Tseng, G. C., et al. (2018). Sustained molecular pathology across episodes and remission in major depressive disorder. Biol. Psychiatry 83, 81–89. doi: 10.1016/j.biopsych.2017.08.008
Sibille, E., Morris, H. M., Kota, R. S., and Lewis, D. A. (2011). GABA-related transcripts in the dorsolateral prefrontal cortex in mood disorders. Int. J. Neuropsychopharmacol. 14, 721–734. doi: 10.1017/s1461145710001616
Slouzkey, I., and Maroun, M. (2016). PI3-kinase cascade has a differential role in acquisition and extinction of conditioned fear memory in juvenile and adult rats. Learn. Mem. 23, 723–731. doi: 10.1101/lm.041806.116
Soghomonian, J. J., and Martin, D. L. (1998). Two isoforms of glutamate decarboxylase: why? Trends Pharmacol. Sci. 19, 500–505. doi: 10.1016/s0165-6147(98)01270-x
Song, G., Ouyang, G., and Bao, S. (2005). The activation of Akt/PKB signaling pathway and cell survival. J. Cell. Mol. Med. 9, 59–71. doi: 10.1111/j.1582-4934.2005.tb00337.x
Steenkamp, L. R., Hough, C. M., Reus, V. I., Jain, F. A., Epel, E. S., James, S. J., et al. (2017). Severity of anxiety- but not depression- is associated with oxidative stress in major depressive disorder. J. Affect. Disord. 219, 193–200. doi: 10.1016/j.jad.2017.04.042
Sui, L., Wang, J., and Li, B.-M. (2008). Role of the phosphoinositide 3-kinase-Akt-mammalian target of the rapamycin signaling pathway in long-term potentiation and trace fear conditioning memory in rat medial prefrontal cortex. Learn. Mem. 15, 762–776. doi: 10.1101/lm.1067808
Sutherland, C., Leighton, I. A., and Cohen, P. (1993). Inactivation of glycogen synthase kinase-3β by phosphorylation: new kinase connections in insulin and growth-factor signalling. Biochem. J. 296, 15–19. doi: 10.1042/bj2960015
Szymańska, M., Suska, A., Budziszewska, B., Jaworska-Feil, L., Basta-Kaim, A., Leśkiewicz, M., et al. (2009). Prenatal stress decreases glycogen synthase kinase-3 phosphorylation in the rat frontal cortex. Pharmacol. Rep. 61, 612–620. doi: 10.1016/s1734-1140(09)70113-6
Taniguchi, H., He, M., Wu, P., Kim, S., Paik, R., Sugino, K., et al. (2011). A resource of Cre driver lines for genetic targeting of GABAergic neurons in cerebral cortex. Neuron 71, 995–1013. doi: 10.1016/j.neuron.2011.07.026
Toker, A., and Newton, A. C. (2000). Akt/protein kinase B is regulated by autophosphorylation at the hypothetical PDK-2 site. J. Biol. Chem. 275, 8271–8274. doi: 10.1074/jbc.275.12.8271
Tripp, A., Kota, R. S., Lewis, D. A., and Sibille, E. (2011). Reduced somatostatin in subgenual anterior cingulate cortex in major depression. Neurobiol. Dis. 42, 116–124. doi: 10.1016/j.nbd.2011.01.014
Uematsu, M., Hirai, Y., Karube, F., Ebihara, S., Kato, M., Abe, K., et al. (2008). Quantitative chemical composition of cortical GABAergic neurons revealed in transgenic venus-expressing rats. Cereb. Cortex 18, 315–330. doi: 10.1093/cercor/bhm056
Viollet, C., Simon, A., Tolle, V., Labarthe, A., Grouselle, D., Loe-Mie, Y., et al. (2017). Somatostatin-IRES-Cre mice: between knockout and wild-type? Front. Endocrinol. 8:131. doi: 10.3389/fendo.2017.00131
Willner, P. (1997). Validity, reliability and utility of the chronic mild stress model of depression: a 10-year review and evaluation. Psychopharmacology 134, 319–329. doi: 10.1007/s002130050456
Willner, P., Towell, A., Sampson, D., Sophokleous, S., and Muscat, R. (1987). Reduction of sucrose preference by chronic unpredictable mild stress and its restoration by a tricyclic antidepressant. Psychopharmacology 93, 358–364. doi: 10.1007/bf00187257
Wu, Z., Wang, G., Wei, Y., Xiao, L., and Wang, H. (2018). PI3K/AKT/GSK3β/CRMP-2-mediated neuroplasticity in depression induced by stress. Neuroreport 29, 1256–1263. doi: 10.1097/WNR.0000000000001096
Wu, R., Zhang, H., Xue, W., Zou, Z., Lu, C., Xia, B., et al. (2017). Transgenerational impairment of hippocampal Akt-mTOR signaling and behavioral deficits in the offspring of mice that experience postpartum depression-like illness. Prog. Neuropsychopharmacol. Biol. Psychiatry 73, 11–18. doi: 10.1016/j.pnpbp.2016.09.008
Xia, B., Chen, C., Zhang, H., Xue, W., Tang, J., Tao, W., et al. (2016). Chronic stress prior to pregnancy potentiated long-lasting postpartum depressive-like behavior, regulated by Akt-mTOR signaling in the hippocampus. Sci. Rep. 6:35042. doi: 10.1038/srep35042
Zbozinek, T. D., Rose, R. D., Wolitzky-Taylor, K. B., Sherbourne, C., Sullivan, G., Stein, M. B., et al. (2012). Diagnostic overlap of generalized anxiety disorder and major depressive disorder in a primary care sample. Depress. Anxiety 29, 1065–1071. doi: 10.1002/da.22026
Zeyda, T., Diehl, N., Paylor, R., Brennan, M. B., and Hochgeschwender, U. (2001). Impairment in motor learning of somatostatin null mutant mice. Brain Res. 906, 107–114. doi: 10.1016/s0006-8993(01)02563-x
Zhang, K., Yang, C., Xu, Y., Sun, N., Yang, H., Liu, J., et al. (2010). Genetic association of the interaction between the BDNF and GSK3B genes and major depressive disorder in a Chinese population. J. Neural Transm. 117, 393–401. doi: 10.1007/s00702-009-0360-4
Keywords: GABA, GAD, somatostatin, depression, anxiety, Akt, GSK3β
Citation: Miyata S, Kumagaya R, Kakizaki T, Fujihara K, Wakamatsu K and Yanagawa Y (2019) Loss of Glutamate Decarboxylase 67 in Somatostatin-Expressing Neurons Leads to Anxiety-Like Behavior and Alteration in the Akt/GSK3β Signaling Pathway. Front. Behav. Neurosci. 13:131. doi: 10.3389/fnbeh.2019.00131
Received: 26 March 2019; Accepted: 04 June 2019;
Published: 18 June 2019.
Edited by:
Denise Manahan-Vaughan, Ruhr University Bochum, GermanyReviewed by:
Etienne Sibille, Centre for Addiction and Mental Health (CAMH), CanadaMario U. Manto, University of Mons, Belgium
Copyright © 2019 Miyata, Kumagaya, Kakizaki, Fujihara, Wakamatsu and Yanagawa. This is an open-access article distributed under the terms of the Creative Commons Attribution License (CC BY). The use, distribution or reproduction in other forums is permitted, provided the original author(s) and the copyright owner(s) are credited and that the original publication in this journal is cited, in accordance with accepted academic practice. No use, distribution or reproduction is permitted which does not comply with these terms.
*Correspondence: Shigeo Miyata, s_miyata@gunma-u.ac.jp; Yuchio Yanagawa, yuchio@gunma-u.ac.jp