- 1Departamento de Análises Clínicas, Toxicológicas e Bromatológicas, Faculdade de Ciências Farmacêuticas de Ribeirão Preto, Universidade de São Paulo, São Paulo, Brazil
- 2Departamento de Morfologia, Fisiologia e Patologia Básica, Faculdade de Odontologia de Ribeirão Preto, Universidade de São Paulo, São Paulo, Brazil
- 3Departamento de Farmacologia, Faculdade de Medicina de Ribeirão Preto, Universidade de São Paulo, São Paulo, Brazil
Depression is a mental illness with a complex and multifactorial etiology, which has been associated with stress and inflammation. Infections, autoimmune diseases, envenomation, and trauma induce an inflammatory response that is characterized by increasing levels of circulating cytokines (e.g., IL-1β) and lipid mediators [e.g., PGE2 and leukotrienes B4 (LTB4)]. Recently, we showed that LTB4 production by the 5-lipoxygenase (5-LO) pathway regulates IL-1β and PGE2 release, reducing tissue damage in a model of sterile inflammation. Since IL-1β and PGE2 increase in serum of stressed patients and potentially trigger depression, we used an animal model of chronic unpredictable stress (CUS) to investigate the potential impact of LTB4 over depression-like symptoms. At basal conditions, 5-LO deficiency (Alox5−/−) reduces the preference for sucrose, while inducing a higher immobilization time on the tail suspension test when compared 129sv. Moreover, Alox5−/− mice present increased caspase-1 expression and elevated levels of IL-1β, IL-17 and PGE2 in the spleen, with increasing corticosterone levels in the frontal cortex but reducing systemic levels. Compared to 129sv mice, CUS induced higher levels of systemic, frontal cortex and hippocampal corticosterone, and also reduced sucrose preference, increased levels of splenic IL-1β, IL-17 and PGE2 and reduced levels of LTB4. Interestingly, CUS exposure did not alter the reduced sucrose preference shown by Alox5−/− mice but greatly enhanced splenic PGE2 production. Compared to Alox5−/− mice at basal conditions, CUS exposure also increased levels of systemic corticosterone, which remained lower than those of CUS-129sv animals. We also observed that treatment with LTB4 decreased caspase-1 expression and systemic levels of corticosterone in CUS-Alox5−/− mice but there was no significant impact on the reduced sucrose preference. Our results demonstrate that LTB4 controls the hypothalamic-pituitary-adrenal (HPA) axis by regulating levels of systemic corticosterone associated with the repression of caspase-1 expression and production of inflammatory mediators. One limitation of our study is that 129sv and Alox5−/− mice were not littermates, not sharing, therefore, the same intra-uterine and preweaning environment. Even so, taken together our results indicate that 5-LO activity is critical for the regulation of stress-induced symptoms, suggesting that the Alox5−/− mouse could be a natural model of corticosterone-independent reduced reward sensitivity.
Introduction
Depression is a serious public health problem and one of the most common psychiatric disorders in Western countries. It may lead to suicide, a tragic fatality accounting for mortality of 800,000 people every year (World Health Organization (WHO), 2017). Depressed patients often exhibit symptoms such as sadness, loss of interest or pleasure (anhedonia), feelings of guilt or low self-esteem, disturbances in sleep or appetite, low energy, and a reduced ability to concentrate (Zunszain et al., 2011). An association between stress, immune response, and psychological disorders has been proposed over the last few decades (Guan et al., 2014). A considerable number of evidence demonstrates that multiple aspects of the immune response are altered during stress or depressive episodes (Maes et al., 1997; Tymen et al., 2013). Indeed, the immune response has been shown to contribute to the pathophysiology of these disorders (Irwin and Miller, 2007). In conditions of chronic psychological stress, such as major depressive disorders, both the cellular and humoral immunity can be persistently activated and contribute to depression.
Depressed and chronically stressed individuals exhibit higher levels of circulating proinflammatory cytokines, chemokines, acute phase proteins, and soluble adhesion molecules or even cerebrospinal fluid (Smith, 1991; Raison et al., 2006; Dantzer et al., 2008). Interestingly, systemic or central administration of cytokines such as IL-1β, IL-6 or TNF-α promotes behavioral perturbations in rats and mice (Dantzer, 2001). Furthermore, mononuclear cells isolated from depressed patients exhibit increased NLRP3 inflammasome and caspase-1 activation, which culminates in elevated systemic levels of IL-18 and IL-1β (Alcocer-Gómez et al., 2014).
Recently, Zhang et al. (2015) reported that mice subjected to chronic mild stress (CMS) have increased IL-1β levels, caspase-1 activity, and NLRP3 inflammasome activation in the hippocampus. Pharmacological inhibition of the inflammasome decreased both IL-1β levels and the CMS-induced depressive-like behavior. Stress induced by pathogenic or non-pathogenic (sterile) stressors triggers the NLRP3 inflammasome in myeloid cells, resulting in the activation of caspase-1, which cleaves the precursor forms of IL-18 and IL-1β cytokines, leading to release of the active cytokines (Strowig et al., 2012; Zhang et al., 2015; Miller and Raison, 2016).
Glucocorticoids, such as cortisol (in humans) and corticosterone (in rodents), are anti-inflammatory hormones (Zen et al., 2011) that increase during chronic stress and depression (Pitman et al., 1988; Cohen et al., 2012; Herane Vives et al., 2015). However, pro-inflammatory cytokines induce glucocorticoid-receptor (GR) resistance (Jeon and Kim, 2016; Rodriguez et al., 2016), suggesting an impairment of glucocorticoid-mediated immunoregulatory mechanisms. Glucocorticoid production is regulated by a negative feedback loop involving GR activation (Smith and Vale, 2006). Furthermore, both isoforms of the enzyme 11β-hydroxysteroid dehydrogenase (11βHSD1 and 11βHSD2) regulate glucocorticoid concentrations. The 11βHSD1 isoform converts inactive 11-ketometabolites into active glucocorticoids in the presence of NADPH. In contrast, the 11βHSD2 isoform metabolizes active glucocorticoids to produce inactive 11-ketometabolites using NAD+ as a cofactor (Krozowski et al., 1999).
In association with the 5-lipoxygenase-activating protein (FLAP), the enzyme 5-lipoxygenase (5-LO) catabolizes arachidonic acid into leukotrienes (LT) B4 (LTB4), C4 (LTC4), D4 (LTD4) and E4 (LTE4; Funk, 2001; Harizi et al., 2008; Rådmark et al., 2015). LTs have been shown to regulate the hypothalamic-pituitary-adrenal (HPA) axis (Hirai et al., 1985). Sato et al. (2008) demonstrated that 5-LO metabolites down-regulate 11βHSD2 activity in trophoblasts in vitro. Moreover, Uz et al. (1999) have shown that glucocorticoids increase the transcriptional and protein levels of 5-LO in the brain. Interestingly, a polymorphism in the gene encoding the enzyme leukotriene A4 hydrolase, which catalyzes LTB4 formation (Haeggström and Funk, 2011), has been associated with susceptibility to depression, especially in women (Zhao et al., 2009). Furthermore, the FLAP inhibitor MK886 induces antidepressant-like effects in mice submitted to the forced swimming test (Uz et al., 2008).
Recently, our research group demonstrated that PGE2 increases IL-1β production by a cyclic adenosine monophosphate (cAMP)-dependent mechanism in a model of sterile inflammation (Zoccal et al., 2016). Moreover, we identified that LTB4 regulates this process by reducing intracellular cAMP levels, which in turn inhibits IL-1β release by innate immune cells (Zoccal et al., 2016). Considering that IL-1β and PGE2 are potential triggers of depression (Müller et al., 2006; Goshen and Yirmiya, 2009), and that LTB4 is able to repress the production of these mediators (Zoccal et al., 2016), we hypothesized that 5-LO deficiency would increase the susceptibility to depression. To investigate this hypothesis, we submitted Alox5−/− (5-LO deficient) and 129sv mice to chronic unpredictable stress (CUS), an experimental model that induces depression-like behaviors such as decreased sucrose preference. Compared to 129sv mice at basal conditions, Alox5−/− animals exhibited an intrinsically lower preference for sucrose and higher levels of IL-1β, IL-17, PGE2, adrenocorticotropic hormone (ACTH), and caspase-1 expression. In contrast, these mice present lower 11βHSD2 and GR expression, as well as reduced levels of corticosterone, 11-dehydrocorticosterone (11DHC), and circulating neutrophils. As expected, 129sv mice exposed to CUS displayed increased levels of splenic proinflammatory and stress-induced mediators, and signs of reduced sucrose preference, which could be associated with anhedonia (Willner, 1997). Intriguingly, most of the analyzed parameters remained at the same levels before and after CUS exposure in Alox5−/− mice. These levels were comparable to those of CUS-129sv. Importantly, exogenous LTB4 administration to CUS-Alox5−/− mice decreased systemic corticosterone levels and caspase-1 expression without affecting sucrose preference.
Materials and Methods
Animals
Male mice (7–8-weeks-old) were used for the experiments. Animals lacking the 5-LO gene (Alox5−/−) were age-matched in all procedures with their genetic background, the wild-type 129sv mouse strain (Chen et al., 1994). The Alox5−/− (129-Alox5tm1Fun/J) and 129sv mice were obtained from The Jackson Laboratory (Bar Harbor, ME, USA) and were bred in the Faculdade de Ciências Farmacêuticas de Ribeirão Preto, Universidade de São Paulo (Ribeirão Preto, SP, Brazil). C57Bl/6 mice, obtained from the Faculdade de Ciências Farmacêuticas de Ribeirão Preto, Universidade de São Paulo, were used as controls to validate our methodology used in the 129sv mice. Mice were kept in 12 h light/dark cycles, with ad libitum access to water and food, except for the period of exposure to stressors (described in “Chronic Unpredictable Stress and Sample Collection” section). All procedures were performed according to the ethical standards established by the National Council for Control of Animal Experimentation (CONCEA) and approved by the Ethics Committee on Animal Use (CEUA) of the Campus of Ribeirão Preto (Protocol number: 14.1.290.53.5).
Chronic Unpredictable Stress and Sample Collection
C57BL/6, 129sv, and Alox5−/− mice were subjected over a period of 14 days to the following daily stressors, which were applied randomly: an alteration in bedding (sawdust removal and replacement with wet sawdust having approximately 5 mm deep water) for 24 h; reversal of the light/dark cycle; 15 min forced swimming; 1 h restraint in a tube. The last stressor was always water and food deprivation for 20 h. The next day, animals underwent a sucrose preference test to evaluate depressive-like behavior (Willner, 1997). Non-stressed animals from each strain were used as controls and these animals were also subjected to 20 h without food and water before the sucrose test. In some experiments, mice exposed or not to CUS were also treated with specific drugs or vehicle, as described below.
At the end of the experiment, mice were anesthetized (75 mg/kg ketamine e 10 mg/kg xylazine) and the blood was immediately collected by cardiac puncture for the analysis of circulating leukocytes. All blood samples were collected at 12:00 pm and in the presence or absence of an anticoagulant and mice were weighed at day zero, and again at day fifteen. Next, the animals were euthanized by cervical dislocation. The spleen and adrenal glands, and the cerebellum, frontal cortex, and hippocampus were weighed after collection.
Sucrose Preference Test
129sv and Alox5−/− mice were individually housed and deprived of food and water (the last stress stimulus) for 20 h. Next, a glass bottle with water and another with water containing 1% sucrose was placed in each cage. The bottles were initially weighed and consumption was monitored after 1 h. For this purpose, the glass bottles were weighed again to estimate the liquid ingestion. The sucrose preference was calculated as described previously (Guo et al., 2009) with the following formula: sucrose consumption/(sucrose consumption + water consumption).
LTB4 Treatment in vivo
Alox5−/− mice were intraperitoneally (i.p.) injected with LTB4 (50 ng in 100 μL; Cayman, Ann Arbor, MI, USA) during the morning. Treatment initiated 1 day before CUS, followed by administration twice a day (12/12 h) during the 14 days of CUS exposure. LTB4 was dissolved in ethanol and diluted in aqueous solution containing 0.45% NaCl, 0.25% dextrose, and 0.01% bovine serum albumin (BSA) followed by filter sterilization. The same volume of ethanol was added to the same aqueous solution to be used as the vehicle control. The LTB4 dose was selected based on previous studies (Zoccal et al., 2016, 2018).
Leukocyte Counts and Adrenocorticotropic Hormone (ACTH) Quantification
Blood samples were collected and immediately used for total and differential counts of circulating leukocytes as previously described (Pereira et al., 2013). In one specific set of experiments, blood was collected in tubes with EDTA (BD Microtainer, BD Biosciences, San Jose, CA, USA), centrifuged (400× g for 10 min at 4°C), and the remaining plasma was stored at −20°C. ACTH concentrations were determined with a chemiluminescent immunoassay (CLIA) technology (Liaison ACTH, 313221, DiaSorin, Sallugia, Piemonte, Italy) employing a Liaison Analyzer (DiaSorin, Sallugia, Piemonte, Italy), following the manufacturer’s instructions. The sensitivity of the assay was <1,500 pg/mL.
Mass Spectrometry (MS) Measurements
Corticosterone and 11DHC from serum, frontal cortex, hippocampus, and cerebellum were quantified by mass spectrometry (MS) as previously described (Peti et al., 2018). Tissues were weighed, homogenized (Mixer Homogenizer, Labortechnik, Wasserburg, Bavaria, Germany) in methanol:water (v/v 1:1), centrifuged, and the supernatant was recovered. Supernatants and serum were purified as previously described (Galvão et al., 2016). Samples were analyzed using the mass spectrometer TripleTOF 5600+ (Sciex, Foster, CA, USA) coupled with the liquid chromatography system Nexera (Shimadzu Corp., Kyoto, Japan). Data were processed using PeakView and MultiQuant software. For spleens, PGE2 and LTB4 levels were determined using a LC-MS/MS system Acquity UPLC®—Xevo TQ-S (Waters, Milford, MA, USA). Data were analyzed with MassLynx 4.0 and TargetLynx 4.0 softwares.
Enzymatic Immunoassay (EIA) Measurements
Corticosterone levels were also determined using a specific enzyme immunoassay kit (Corticosterone ELISA kit, Enzo Life Science, New York, USA) according to the manufacturer’s instructions. The reading was taken at 405–420 wavelength and the results were expressed in ng/mL.
Quantification of IL-1β and IL-17 by Enzyme-Linked Immunosorbent Assay
Spleens were homogenized (Mixer Homogenizer, Labortechnik, Wasserburg, Germany) and commercially available Enzyme-Linked Immunosorbent Assay (ELISA) kits (R&D Systems, Minneapolis, MN, USA) were used for quantification of IL-17 and IL-1β levels, according to the manufacturer’s instructions. The sensitivity of the assay was <10 pg/mL.
Determination of T Lymphocyte Populations and Cellular Apoptosis by Flow Cytometry
Spleen cell suspensions were obtained by organ maceration following erythrocytes lysis with ACK lysis buffer (Invitrogen, Carlsbad, CA, USA). Total cell suspensions were adjusted to 1 × 106 cells/mL and flow cytometry was carried out as previously described (Secatto et al., 2012). The following fluorochrome-conjugated monoclonal antibodies were used: anti-CD3 (FITC); anti-CD4 (PE); and anti-CD8 (PerCP; BD Bioscience, San Jose, CA, USA). Samples were analyzed using a FACSCanto Flow Cytometer (BD Bioscience, San Jose, CA, USA). The percentage of CD3+CD4+ and CD3+CD8+ double-positive cells were calculated using the FACSDiva software. Apoptosis was evaluated in spleen using an apoptosis detection kit based on annexin V-FITC/propidium iodide (PI) staining (BD Pharmingen, San Jose, CA, USA), according to the manufacturer’s instructions. Briefly, cellular suspensions were washed twice with PBS, centrifuged at 400× g for 15 min, suspended in the kit buffer, and then incubated with annexin V in the absence of light for 15 min. PI was added to all samples immediately before data acquisition. A total of 10,000 events were acquired from all samples using the FACSCanto flow cytometer (BD Bioscience, San Jose, CA, USA). The results were evaluated using the FACSDiva software and expressed as the percentage of cells in the initial stage of apoptosis, i.e., positive for annexin V and negative for PI (Locachevic et al., 2015).
Quantitative Real Time PCR
Adrenal glands and spleens were collected, and total RNA was extracted using PureLink RNA Mini Kit (ThermoFisher Scientific, Waltham, MA, USA), according to the manufacturer’s instructions. RNA concentration and purity were evaluated using a fluorimetric assay (Qubit, Invitrogen, Carlsbad, CA, USA). Complementary DNA (cDNA) was obtained using the High Capacity cDNA Reverse Transcription kit (Applied Biosytems, Foster City, CA, USA), according to the manufacturer’s instructions. Total cDNA was amplified by real-time reverse transcription polymerase chain reaction (RT-qPCR) using TaqMan primers for the Hsd11b1, Hsd11b2, and Casp1 genes employing StepOne Plus (Applied Biosystems, Foster City, CA, USA). Actb was used as the endogenous control. The reactions were amplified under the following conditions: denaturation at 95°C for 2 min, followed by 40 cycles of 95°C for 2 min and 60°C for 20 s. The expression of the genes Hsd11b1 (Mm01251104_m1, Applied Biosystems, Foster City, CA, USA), Hsd11b2 (Mm00476182_m1, Applied Biosystems, Foster City, CA, USA), and Casp1 (Mm.PT.58.13005595, IDT, San Diego, CA, USA) were normalized by housekeeping gene expression (Actb). The gene expression of 129sv mice was considered as the reference (129sv mice), being 2−ΔΔCT = 1. The primers were obtained commercially and are proprietary; thus, sequences are not available (TaqMan Gene Expression Assay, Applied Biosystems, Foster City, CA, USA).
Immunoblotting for Determination of the Glucocorticoid Receptor (GR)
The spleens and hippocampi from mice subjected or not to CUS were isolated and immediately immersed in RIPA buffer supplemented with protease inhibitors (Roche Diagnostics, Basel, Switzerland). After homogenization and centrifugation at 400× g for 10 min, the supernatant was used to measure GR expression. Equal amounts of protein (50 μg) from each sample were separated by polyacrylamide gel for electrophoresis (10% Tris-HCl) and subsequently transferred to a nitrocellulose membrane, as previously described (Serezani et al., 2005). The membranes were immunolabeled with an anti-GR monoclonal primary antibody (1:10,000; MCA1390, BioRad Antibodies, CA, USA), or anti-GAPDH antibody (1:5,000; Sigma Aldrich, St. Louis, Missouri, USA) as the endogenous control. Following membranes were incubated with the appropriate horseradish peroxidase (HRP)-conjugated secondary IgG (1:10,000; Cell Signaling, Danvers, MA, USA). ECL reagent (Amersham Biosciences, Little Chalfont, UK) was used to reveal by chemiluminescent reactions using a photo documenter (Alliance 4.7, UVITEC, Cambridge, England, UK). The band densities were determined using the ImageJ program1 and normalized by the signal intensity emitted by the endogenous GAPDH control.
Enzymatic Activity of 11βHSD2
Activity of 11βHSD2 was evaluated as described by Kajantie et al. (2003) and Stewart et al. (1995) in samples of spleen, adrenal gland, frontal cortex and cerebellum from 129sv and Alox5−/− mice, exposed or not to CUS. Briefly, all samples were homogenized (Mixer Homogenizer, Labortechnik, Wasserburg, Germany) in KCl buffer (0.154 mol/L; pH 7.6) and centrifuged at 1,000 g for 10 min 4°C. Protein assay was performed on the supernatant using Coomassie blue staining, as described previously by Medeiros et al. (2008). Supernatants (0.50 g/L of spleen protein, 0.15 g/L of adrenal protein, 0.10 g/L of frontal cortex protein or 0.20 g/L of cerebellum protein) were incubated with a solution containing 1.0 mL of phosphate buffer (0.1 mol/L; pH 7.6), 0.8 nmol/L nicotinamide adenine dinucleotide, and 0.2 mol/L corticosterone in a shaking water bath at 37°C. Spleen proteins were incubated for 30 min and the other tissues for 45 min. Enzymatic reactions were stopped with 1.0 mL of methanol. For extraction, 7 mL of dichloromethane was added to the samples and vortexed for 30 s. The upper phase was removed, 2 mL of water were added, and the tubes were mixed again. The lower phase (organic phase) was collected, dried and resuspended in 40 μL (spleen) or 25 μL (other tissues) of methanol. The enzymatic activity was evaluated by the conversion of corticosterone into 11DHC, which were measured by LC-MS/MS (Peti et al., 2018).
Statistical Analyses
Data were analyzed using SPSS V. 20 (IBM, USA). After checking for normality and homoscedasticity, the results were analyzed using Student’s t-test, two- or one-way analysis of variance (ANOVA), when appropriated. Post hoc comparisons were performed with the Newman-Keuls test. Differences were considered significant when p < 0.05.
Results
Alox5−/− Mice Show an Intrinsic HPA Activation and Differential Dynamics of Systemic and Hippocampal Corticosterone
To determine the impact of 5-LO deficiency on stress-induced behavior and immune response, we began by comparing the amount of circulating corticosterone in two distinct mouse strains, C57Bl/6 and 129sv. The former strain has been widely characterized in studies addressing CUS-induced depression-like behavior (Pothion et al., 2004). Both mouse strains exhibited similar levels of serum corticosterone before CUS exposition (Figure 1A). Following 14 days of CUS exposure, serum levels of corticosterone increased significantly in both strains (F(1,22) = 42.06, p < 0.001), but corticosterone levels remained comparable between them (Figure 1A). We observed similar blood neutrophil counts between both mouse strains at basal conditions, which were equally reduced after CUS exposition (F(1,23) = 7.13, p < 0.02; Supplementary Figure S1B). Mononuclear cells were also equally represented in the blood of both strains at basal conditions, but these cells were significantly reduced in the blood of CUS-129sv compared to CUS-C57Bl/6 (interaction CUS vs. strain, F(1,19) = 6.83, p = 0.017; Supplementary Figure S1A). Considering that 129sv mice submitted to CUS presented a similar profile in these parameters to that of C57Bl/6 mouse strain, we were confident to use the 129sv strain as controls of experiments performed with Alox5−/− mice.
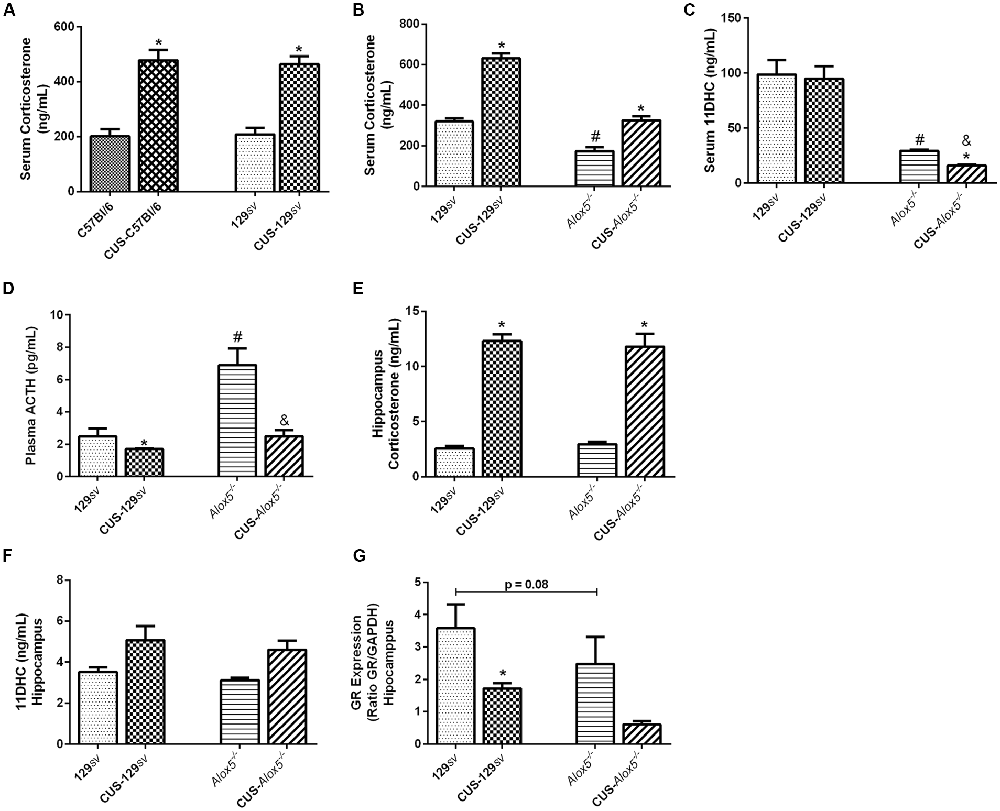
Figure 1. Alox5−/− mice exhibit a natural hypothalamic-pituitary-adrenal (HPA) axis activation and produce different corticosterone amounts in the serum and hippocampus. (A) The C57Bl/6 and 129sv mice were subjected or not to chronic unpredictable stress (CUS) for 14 days. Animals were euthanized on the 15th day and blood was collected for corticosterone quantification. (B–D) Alox5−/− and 129sv mice were subjected or not to CUS for 14 days. Animals were euthanized on the 15th days and blood was collected for quantification of (B) corticosterone, (C) 11-dehydrocorticosterone (11DHC) and (D) adrenocorticotropic hormone (ACTH). (E–G) The brain was also collected and the hippocampus was dissected for (E) corticosterone and (F) 11DHC quantification or (G) glucocorticoid-receptor (GR) expression. Data are presented as the means ± SEM of one experiment (n = 3–11 mice/genotype/group). Two-way analysis of variance (ANOVA) followed by Newman-Keuls Multiple Comparison Test, p < 0.05. *C57Bl/6 or 129sv or Alox5−/− vs. CUS-C57Bl/6 or CUS-129sv or CUS-Alox5−/−, respectively; #129sv vs. Alox5−/−; &CUS-129sv vs. CUS-C57Bl/6 or CUS-Alox5−/−.
Deficiency of 5-LO abrogates the production of LTs and cysteinyl-LTs. Considering that HPA axis activation and corticosterone production are important components of the stress response, we sought to determine the impact of 5-LO deficiency on CUS-induced ACTH release in plasma samples, corticosterone production, and its metabolization into 11DHC in serum. At basal conditions, Alox5−/− exhibited significant lower amounts of circulating corticosterone and 11DHC compared to 129sv mice (Corticosterone, F(1,20) = 15.70, p < 0.001; 11DHC, F(1,20) = 1239.0, p < 0.001; Figures 1B,C).We also found higher ACTH levels in Alox5−/− mice (CUS vs. strain interaction; ACTH, F(1,15) = 7.98, p = 0.013; Figure 1D). Compared to non-stressed 129sv controls, CUS-129sv mice produced 97% more circulating corticosterone and approximately 32% less circulating ACTH (Figures 1B,D). CUS-Alox5−/− mice produced 87% more circulating corticosterone and had a reduction of approximately 64% in ACTH levels (Figures 1B,D). Together these results suggest that 5-LO deficiency results in lower corticosterone concentrations in the circulation, which triggers the activation of the HPA axis, reflected by high levels of plasma ACTH in Alox5−/− mice at basal conditions. Furthermore, these data suggest similar hormonal profiles in the blood of Alox5−/− mice at basal conditions and 129sv exposed to CUS.
Because depression affects primarily the brain, we investigated some aspects of stress response in the hippocampus of 129sv and Alox5−/− mice. Both strains showed similar amounts of corticosterone (Figure 1E) and 11DHC (Figure 1F) at basal conditions. After CUS exposition, corticosterone levels equally increased in the hippocampus in both strains, but no changes were detected in 11DHC levels. Corticosterone levels are self-regulated by activation of GR. Thus, we hypothesized that the discrepancies between serum and hippocampus corticosterone levels in 129sv and Alox5−/− mice could be related to lower GR expression, with consequent deregulation of the negative feedback loop in the HPA axis. We found that cells isolated from the hippocampus of non-stressed Alox5−/− mice showed a trend to reduced GR expression (p = 0.08; Figure 1G). Importantly, hippocampal GR expression was significantly reduced in CUS-129sv, but not CUS-Alox5−/− mice.
In addition, the number of circulating leukocyte was evaluated in the 129sv and Alox5−/− mice exposed or unexposed to CUS. At a basal state, both strains presented similar numbers of circulating mononuclear cells, which decreased significantly after CUS (F(1,20) = 196.1, p < 0.001; Supplementary Figure S1C). However, Alox5−/− mice exhibited approximately 61% less neutrophils compared to 129sv at basal conditions (CUS vs. strain interaction, F(1,20) = 20.16, p < 0.01; Supplementary Figure S1D). Interestingly, CUS exposure also reduced circulating neutrophil numbers by approximately 60% in 129sv mice, reaching similar levels to those of Alox5−/− mice (Supplementary Figure S1D).
Alox5−/− Mice Have a Similar Profile of Stress-Induced Mediators to That of CUS-129sv Mice—Brain Structures Panorama
Contrasting data between blood and hippocampal corticosterone levels prompted us to perform a detailed investigation of different brain structures. Compared to 129sv mice, Alox5−/− animals showed a significant increase in corticosterone production in the frontal cortex at basal conditions (Figure 2A). After CUS exposition, corticosterone levels increased by 420% in CUS-129sv mice compared to non-exposed 129sv animals and remained unaltered in Alox5−/− mice (Figure 2A). As an additional negative control, we measured corticosterone concentrations in the cerebellum. As expected, there were no significant alterations on corticosterone levels in the cerebellum of 129sv or Alox5−/− mice, neither before nor after CUS exposition (Figure 2B). However, Alox5−/− mice presented lower 11DHC in the frontal cortex (F(1,17) = 29.54, p < 0.0001; Figure 2C) and in the cerebellum (F(1,17) = 20.41, p < 0.0003; Figure 2D) compared with 129sv mice. CUS exposure did not modify this profile. In contrast, CUS-129sv mice showed decreased 11DHC levels in both the frontal cortex (F(1,17) = 6.873, p = 0.0179; Figure 2C) and cerebellum (F(1,17) = 45.75, p < 0.0001; Figure 2D) when compared to 129sv animals.
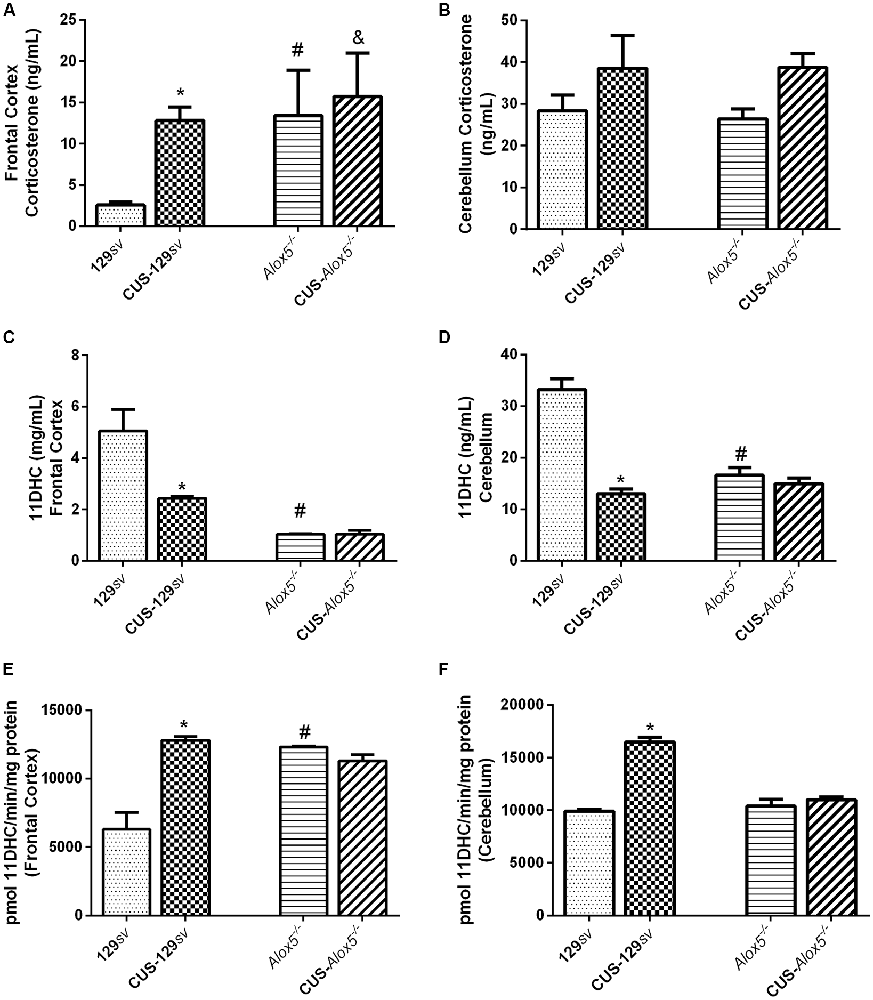
Figure 2. Alox5−/− and CUS-129sv mice exhibit similar hormonal profile in different brain structures. 129sv and Alox5−/− mice were subjected or not to CUS for 14 days. The animals were euthanized on the 15th day and the brains collected to dissect the frontal cortex and cerebellum. Quantification of (A,B) corticosterone (C,D) 11DHC and (E,F) 11β-hydroxysteroid dehydrogenase (11βHSD2) enzymatic activity in the frontal cortex and cerebellum, respectively. Data are presented as the means ± SEM of one experiment (n = 6–11 mice/genotype/group). Two-way ANOVA followed by Newman-Keuls Multiple Comparison Test, p < 0.05. *129sv vs. CUS-129sv; #129sv vs. Alox5−/−; &CUS-129sv vs. CUS-C57Bl/6 or CUS-Alox5−/−.
The 11βHSD1 and 11βHSD2 enzymes are responsible for the metabolism of corticosterone, with 11βHSD1 promoting its production, whereas 11βHSD2 converts it into the inactive metabolite 11DHC. To investigate the potential molecular mechanism regulating the balance between corticosterone and 11DHC, we assessed the enzymatic activity of 11βHSD2 in the frontal cortex and cerebellum. Compared to 129sv mice, 11βHSD2 activity increased in the frontal cortex of Alox5−/− and CUS-129sv mice (F(1,17) = 8.237, p = 0.0106; F(1,17) = 12.39, p = 0.0026; Figure 2E) and cerebellum of CUS-129sv (F(1,17) = 85.16, p < 0.0001; Figure 2F). Despite the increased 11βHSD2 activity in the frontal cortex from CUS-129sv and in Alox5−/− mice, the reduced 11DHC levels suggest that corticosterone is produced at a higher rate than consumed, as a result of a higher 11βHSD1 activity. It is also possible that corticosterone is catabolized by a different pathway that converts it into a metabolite other than 11DHC.
Alox5−/− Mice Exhibit a Natural Low Sucrose Preference at Basal Conditions—Behavior Panorama
We evaluated Alox5−/− and 129sv strains, submitted or not to CUS, for sucrose preference, to determine the involvement of 5-LO deficiency on that behavior. The test assesses the reduction in sugar solution intake and sucrose preference in relation to a control group (Guo et al., 2009). Exposure to CUS decreased sucrose solution, but not total liquid intake by CUS-129sv mice compared to 129sv animals in basal conditions (CUS, F(1,36) = 8.10, p = 0.007, CUS vs. strain, F(1,36) = 4.68, p = 0.03; Figure 3A). This was reflected by lower sucrose preference (F(3,36) = 5.11, p = 0.005; Figure 3B), indicating that 129sv mice, which express 5-LO, develop decreased sucrose preference when exposed to CUS. However, compared to non-stressed 129sv mice, Alox5−/− animals at basal conditions already exhibited lower sucrose preference, which remained unchanged after 14 days of CUS exposure (Figure 3B). These results demonstrate that the Alox5−/− mice present a natural lower sucrose preference, which is comparable to 129sv mice exposed to CUS. Furthermore, that behavior was not altered in Alox5−/− mice. Therefore, impaired LTB4 production could predispose these animals to reach a maximal decreased sucrose preference response that remains unchanged even under conditions of chronic stress. We also weighed the whole body of 129sv and Alox5−/− before and after 14 days of CUS (Figure 3C).CUS exposure significantly reduced the body weight in both mouse strains (F(1,20) = 8.41, p < 0.01). Supporting our previous findings, these results confirm a significant impact of 5-LO deficiency in a natural depressive-like phenotype of Alox5−/− mice that remains mostly unaltered after stressful stimuli.
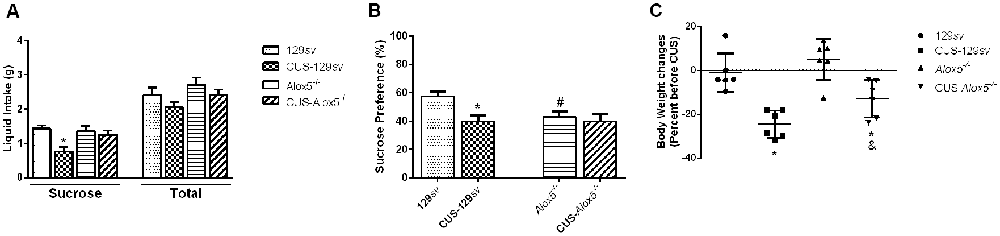
Figure 3. 5-lipoxygenase (5-LO) deficiency results in decreased sucrose preference at basal conditions. 129sv and Alox5−/− mice were exposed or not to CUS for a period of 14 days. Animals were deprived of water and food for 20 h on the 14th day. (A,B) On the 15th day, mice were evaluated for (A) liquid intake and (B) sucrose preference. (C) 129sv and Alox5−/− mice were weighed on day 0, and exposed or not to CUS for a period of 14 days. Animals were weighed again on the 15th day, and the weight changes were expressed as a percentage before CUS. Data are presented as the means ± SEM and depict a representative experiment out of three independent experiments (n = 6–11 mice/genotype/group/experiment). Two-way ANOVA followed by Newman-Keuls Multiple Comparison Test, p < 0.05. *129sv vs. CUS-129sv; #129sv vs. Alox5−/−.
5-LO Deficiency Impacts the Production of Inflammatory Mediators Before and After CUS—Spleen Panorama
Inflammatory mediators such as IL-1β, IL-17 and PGE2 mediate stress responses (Müller et al., 2006; Goshen and Yirmiya, 2009; Guo et al., 2009; Beurel et al., 2013), while 5-LO and LTs play significant roles during inflammatory processes (Filgueiras et al., 2015; Zoccal et al., 2018). Using a model of sterile inflammation, we have shown previously that Alox5−/− mice produce higher amounts of IL-1β and PGE2 compared to 129sv mice (Zoccal et al., 2016). In view of the natural depressive-like behavior of Alox5−/− mice, we thus investigated whether this phenotype correlates with alterations on the splenic production of inflammatory mediators by both mice strains exposed or not to CUS. Compared 129sv mice at basal conditions, levels of IL-1β, IL-17 and PGE2 increased by 164% (F(1,17) = 6.06, p = 0.025; Figure 4A), 83% (F(1,25) = 11.61, p = 0.002; Figure 4B), and 85% (F(1,25) = 5.46, p = 0.028; Figure 4C) in the spleen of CUS-129sv animals, respectively. In addition, LTB4 concentration decreased by almost 25% (t-test, p = 0.054; Figure 4D). IL-1β, IL-17, and PGE2 levels were also elevated in the spleen of Alox5−/− mice by 104%, 59%, and 50% compared to 129sv animals at basal conditions, respectively (Figures 4A–C). However, IL-1β and IL-17 levels were significantly reduced in the spleen of Alox5−/− mice both after CUS exposition, compared to CUS-129sv animals (Figures 4A,B). In contrast to all phenomena described so far, PGE2 increased significantly in the spleen of Alox5−/− mice exposed to CUS when compared to non-stressed Alox5−/− or even CUS-129sv (Figures 4A–C).
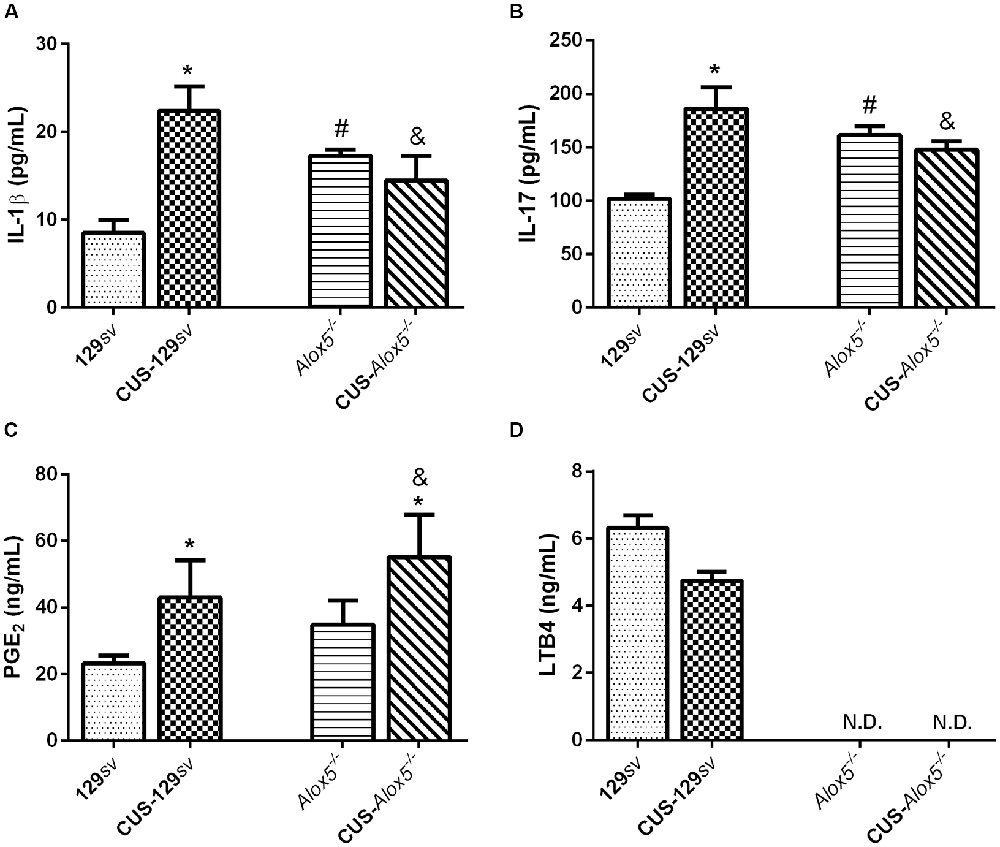
Figure 4. 5-LO regulates the production of inflammatory mediators before and after CUS exposition. 129sv and Alox5−/− mice were exposed or not to CUS for 14 days. Animals were euthanized on the 15th days and spleens were collected for quantification of (A) IL-1β, (B) IL-17, (C) PGE2 and (D) leukotrienes B4 (LTB4). Data are presented as means ± SEM of one representative out of two independent experiments (n = 6 mice/genotype/group/experiment). Two-way ANOVA followed by Newman-Keuls Multiple Comparison Test, p < 0.05. *129sv or Alox5−/− vs. CUS-129sv or CUS-Alox5−/−; #129sv vs. Alox5−/−; &CUS-129sv vs. CUS-Alox5−/−. ND, not determined.
Alox5−/− Mice at Basal Conditions Exhibit Stress-Like Profiles of Caspase-1 Expression and 11βHSD2 Activity—Spleen Panorama
Both 129sv and Alox5−/− mice showed reduced spleen weights after CUS exposition (Figure 5A), explained by increased apoptosis of their splenic cells (F(1,11) = 27.7, p < 0.001; Figure 5B), which was more pronounced in the spleens of CUS-Alox5−/− mice (CUS vs. strain, F(1,11) = 18.6, p < 0.001). Compared to 129sv mice, Alox5−/− animals at basal conditions exhibited higher amounts of splenic IL-1β (Figure 4A). Caspase-1 activation largely drives IL-1β maturation and release (Friedlander et al., 1996; Rano et al., 1997). We thus predicted that caspase-1 expression would be increased in spleens of Alox5−/− mice at basal conditions. As expected, the deficiency in 5-LO related metabolites results in higher caspase-1 expression compared to non-stressed 129sv mice (CUS vs. strain, F(1,8) = 35.94; Figure 5C). Curiously, caspase-1 expression also increased in CUS-129sv mice compared to non-stressed 129sv animals but remained equally expressed in Alox5−/− mice even after CUS exposition (Figure 5C).
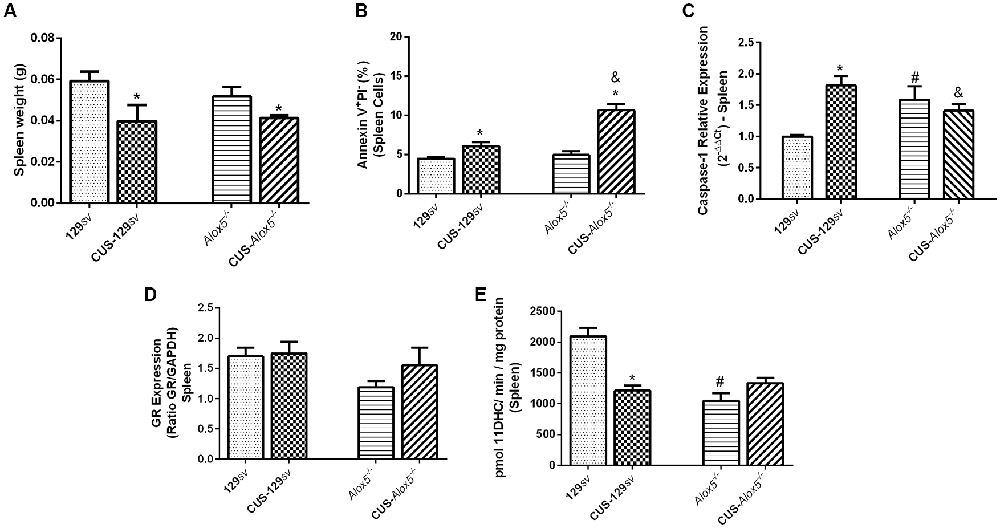
Figure 5. Spleens from Alox5−/− mice have increased caspase-1 expression, but lower 11βHSD2 activity compared to 129sv at basal conditions. Both 129sv and Alox5−/− mouse strains were subjected or not to CUS for 14 days. Animals were euthanized on the 15th day and the spleen was collected. (A) Spleen weighs before and after CUS exposition. (B) Apoptosis assay measured by flow cytometry. (C) Transcriptional caspase-1 expression. (D) Protein GR expression. (E) 11βHSD2 activity. Data are presented as means ± SEM and represent one out of two independent experiments (n = 6 mice/genotype/group/experiment). Two-way ANOVA followed by Newman-Keuls Multiple Comparison Test, p < 0.05. *129sv or Alox5−/− vs. CUS-129sv or CUS-Alox5−/−; #129sv vs. Alox5−/−; &CUS-129sv vs. CUS-Alox5−/−.
Paugh et al. (2015) demonstrated that GR can be cleaved by caspase-1, resulting in glucocorticoid resistance. Systemic corticosterone concentration is self-regulated by GR activation, whereas 5-LO deficiency induces lower levels of circulating corticosterone. We thus hypothesized that lower GR expression would explain this reduction. However, there was no significant change in GR expression between both mouse strains before or after CUS exposition (Figure 5D). Due to its role in corticosterone degradation, we also assessed 11βHSD2 activity, which was significantly reduced in the spleens of Alox5−/− mice (F(1,17) = 17.09, p = 0.0007) and in CUS-129sv mice (F(1,17) = 7.143, p = 0.0161) compared with 129sv (Figure 5E). Together, our results indicate that 5-LO derived metabolites regulate the apoptotic cellular death in the spleen, which associates with increased caspase-1 expression and decreased 11βHSD2 activity at basal conditions, that remains at the same levels after chronic stress.
Alox5−/− Mice at Basal Conditions Exhibit Stress-Like Profiles of Caspase-1 Expression and 11βHSD2 Activity—Adrenal Panorama
The HPA axis showed increased activation in Alox5−/− mice at basal conditions. We, therefore, investigated the presence of possible molecular changes in the adrenal glands in both mouse strains exposed or not to CUS. As observed for the spleens, CUS exposure reduced adrenal gland weight in both strains (Figure 6A). Caspase-1 expression also increased in adrenal glands of CUS-129sv and Alox5−/− mice at basal conditions (F(1,6) = 15.63 p < 0.01; Figure 6B). In this situation, the adrenal glands of both 129sv and Alox5−/− strains expressed similar levels of 11βHSD1, which remained equally expressed in CUS- Alox5−/−, but increased significantly in CUS-129sv mice (CUS vs. strain, F(1,16) = 27.32, p < 0.001; Figure 6C). Compared to 129sv mice at basal conditions, CUS-129sv and Alox5−/− animals expressed significantly less 11βHSD2 (CUS vs. strain, F(1,7) = 22.26, p < 0.001; Figure 6D). Of note, CUS exposure of Alox5−/− does not seem to cause a significant impact on the expression of any of these enzymes in the adrenal gland (Figures 6C,D). Importantly, at basal conditions Alox5−/− and CUS-129sv mice exhibited lower 11βHSD2 enzymatic activity compared to 129sv mice (F(1,17) = 17.81, p = 0.0006; F(1,17) = 10.85, p = 0.0043 respectively; Figure 6E).
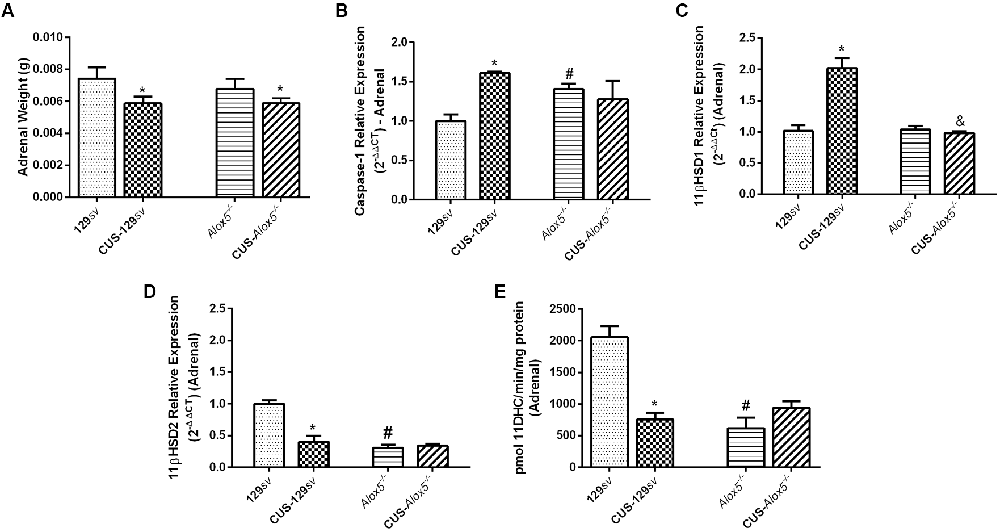
Figure 6. Adrenal glands from Alox5−/− mice have increased caspase-1 expression, but lower 11βHSD2 activity compared to 129sv at basal conditions. 129sv and Alox5−/− mice were subjected or not to CUS for 14 days. Animals were euthanized on the 15th days and the adrenal glands were collected. (A) Adrenal gland weight before and after CUS exposition. (B) Transcriptional expression of caspase-1, (C) 11βHSD1 and (D) 11βHSD2 in adrenal glands. (E) Enzymatic 11βHSD2 activity in adrenal glands. Data are presented as means ± SEM and represent one of two independent experiments (n = 6 mice/genotype/group/experiment). Two-way ANOVA followed by Newman-Keuls Multiple Comparison Test, p < 0.05. *129sv or Alox5−/− vs. CUS-129sv or CUS-Alox5−/−; #129sv vs. Alox5−/−; &CUS-129sv vs. CUS-Alox5−/−.
LTB4 Regulates Levels of Systemic Corticosterone and Caspase-1 Expression
At this point of our study, we have demonstrated that Alox5−/− mice exhibit depressive-like phenotype at basal conditions. Excluding PGE2 production, CUS exposure does not seem to cause a significant impact on this profile, which is very similar to that of CUS-129sv mice. Considering that Alox5−/− animals lack all 5-LO-derived metabolites and LTB4 is the major product of this pathway (Samuelsson, 2000), we performed a pharmacological treatment with this lipid mediator to evaluate its specific involvement on the altered biological and behavioral responses due to 5-LO deficiency (Supplementary Figure S2). Interestingly, LTB4 reduced serum corticosterone levels of CUS-Alox5−/− mice compared to both Alox5−/− and CUS-Alox5−/− mice at basal conditions treated with vehicle (Figure 7A). In contrast, all groups exhibited similar sucrose preference (Figure 7B). Together, these results suggest that, at least in this model, corticosterone does not mediate the decreased sucrose preference. We have shown previously that LTB4 inhibits IL-1β release during sterile inflammation (Zoccal et al., 2016, 2018). Therefore, we evaluated whether this change was associated with the levels of caspase-1 expression. Compared to vehicle-injected CUS-Alox5−/− mice, LTB4 treatment significantly decreased caspase-1 expression in the adrenal glands (F(2,8) = 10.52, p < 0.06; Figure 7C) and in the spleen (F(2,12) = 5.91, p = 0.016; Figure 7D). These results could help to explain the high levels of IL-1β production of Alox5−/− mice (Figure 4A). Taken together, our results indicate that, besides inhibiting intracellular cAMP production (Zoccal et al., 2016, 2018), LTB4 also inhibits caspase-1 expression to suppress IL-1β release. Moreover, these data demonstrate that LTB4 also regulates the levels of systemic corticosterone production after CUS exposure.
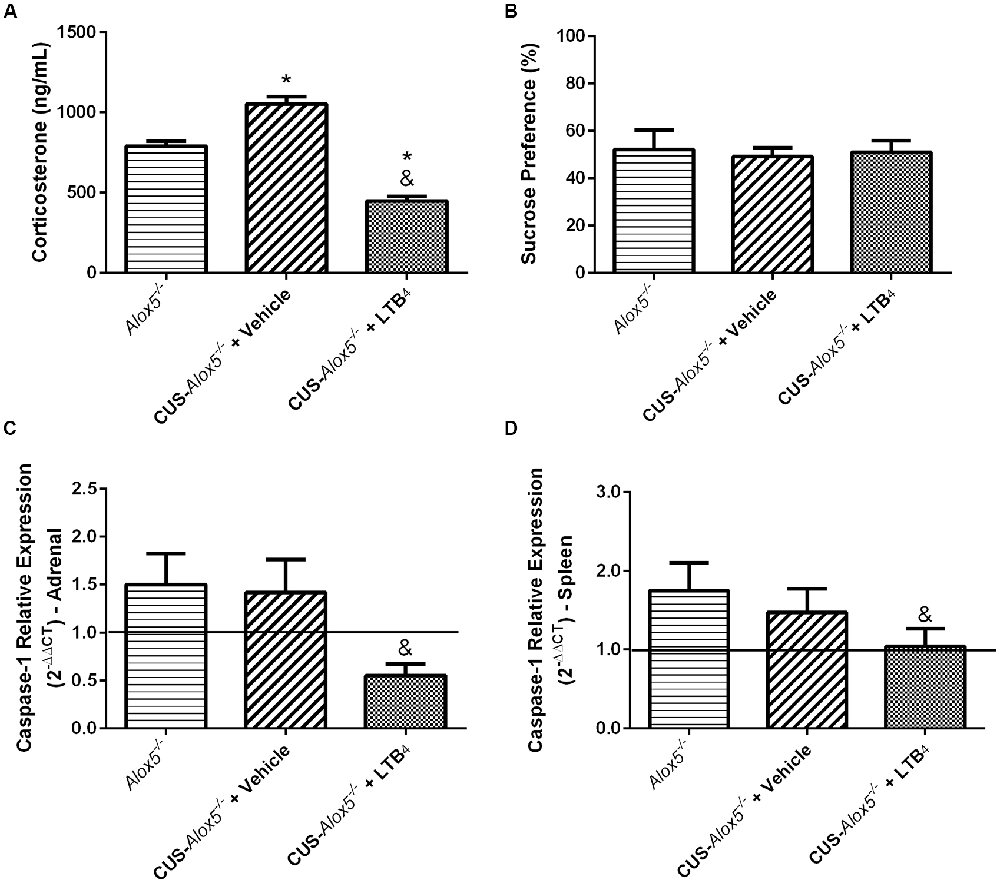
Figure 7. Exogenous LTB4 decreases corticosterone production and caspase-1 expression, but not decreased sucrose preference in Alox5−/− mice. Animals were subjected or not to CUS over a period of 14 days. CUS-Alox5−/− mice were treated twice a day (12 h/12 h) with LTB4 (50 ng/animal/100 μL i.p.) or vehicle (100 μL i.p.). (A) Blood was collected on the 15th day of quantification of circulating corticosterone. (B) Before euthanasia on the 15th day, mice were evaluated for sucrose preference. (C) Adrenal glands and (D) spleens were removed for evaluation of the transcriptional caspase-1 expression. In (C,D), 129sv mice at basal conditions were used as a reference (continuous line), and Alox5−/− mice at basal conditions were used as controls. Data are presented as means ± SEM of one experiment with n = 6–8 mice/genotype/group. Two-way ANOVA followed by Newman-Keuls Multiple Comparison Test, p < 0.05. *Alox5−/− vs. CUS-Alox5−/− + Vehicle or CUS-Alox5−/− + LTB4; &CUS-Alox5−/− + Vehicle vs. CUS-Alox5−/− + LTB4.
Discussion
5-lipooxygenase (5-LO), encoded by the Alox5 gene, catalyzes the formation of (LTB4) which is important for the regulation of the HPA-axis and consequently corticosterone levels. This study showed that conditional Alox5−/− mice present reduced sucrose preference, suggesting reduced reward sensitivity. Furthermore, caspase-1, IL-1β, IL-17 and PGE2 increased in these animals. In frontal cortex corticosterone also increased, although its systemic levels decreased. The observed alterations were in general similar to those observed in the CUS-induced changes in control animals. In this case, however, systemic corticosterone levels increased and, notably, LTB4 levels decreased. LTB4 administration restored caspase-1 levels and systemic corticosterone levels, but not reduced sucrose preference.
In the past few years, several reports have provided evidence for the direct relationship between depression and inflammation. However, the role of 5-LO-derived metabolites in depression onset and progression is unclear (Uz et al., 2008; Athayde et al., 2009; Chu and Praticò, 2009; Joshi and Praticò, 2013; Joshi et al., 2015). LTB4 is the main product of 5-LO activation. CUS exposure of 129sv mice, however, had a modest impact over LTB4 production in the spleen (p = 0.054). In line with these findings, reduced (CUS-129sv) or absence (Alox5−/−) LTB4 production influenced several parameters associated with stress-induced behavior, although we cannot exclude the influence of other 5-LO related metabolites. Alox5−/− animals at basal conditions already exhibited lower interest for sucrose. We also detected, using the tail suspension test (TST), that Alox5−/− mice exhibited a natural depressive-like behavior, assessed through the immobility induced by their inability or reluctance to maintain effort rather than a generalized hypoactivity. CUS exposition, however, did not change that behavior. Besides that, we observed that CUS exposure did not cause a significant impact on the immobility time of 129sv, indicating that 14 days of CUS exposure were not enough to change this behavior, contrasting with sucrose preference (data not shown). Furthermore, exposure to CUS did not impact any of the evaluated behavioral parameters in Alox5−/− mice, which were, however, similar to those observed in CUS-129sv animals. This finding shows that 5-LO-deficiency results in decreased sucrose preference that is not modified by chronic stress, possibly indicating a ceiling effect. One limitation of our study is that 129sv and Alox5−/− mice were not littermates, not sharing, therefore, the same intra-uterine and preweaning environment. This limitation is relevant because the maternal behavior could have been affected by 5-LO deletion and, consequently, affected the phenotype of the adult offspring (Gingrich and Hen, 2000). Although the pharmacological results, discussed above, suggest that even this possibility exists, the involvement of LTB4 in the observed behavioral changes is likely. Future studies are necessary to confirm whether the phenotypes observed here were replicated when 129sv and Alox5−/− were born and weaned by the same mother.
Of importance, Alox5−/− mice at basal conditions displayed increased levels of circulating ACTH, indicating that 5-LO deficiency triggers the HPA axis activation even in the absence of stressful stimuli. However, systemic levels of corticosterone were reduced in Alox5−/− mice at basal conditions. They increased after exposure to CUS but did not reach the same levels of CUS-129sv mice. Therefore, our data corroborate the proposal that 5-LO and related metabolites regulate the activity of HPA axis (Joshi and Praticò, 2013). We hypothesized that 11βHSD activity would be associated with the reduced levels of circulating corticosterone. To test this, we investigated the expression and/or activity of 11βHSD isoforms. The regulation of 11βHSD1 and 11βHSD2 expression is a complex process that directly controls corticosterone levels. Interestingly, IL-1β increases the expression of 11βHSD1 (Tetsuka et al., 1999), while reducing the expression and activity of 11βHSD2 (Chisaka et al., 2005). In line with these facts, our results show that CUS-129sv mice presented increased IL-1β levels and 11βHSD1 expression in the spleen, concomitantly with reduced 11βHSD2 expression. The reduced activity of 11βHSD2 in their spleens or adrenal glands, however, suggest that 5-LO and related metabolites engage distinct molecular mechanisms to regulate levels of systemic corticosterone.
Contrasting with the spleen and adrenal glands, the frontal cortex of Alox5−/− and CUS-129sv mice exhibited increased 11βHSD2 activity. These discrepancies suggest that 5-LO deficiency or CUS exposition results in organ-dependent changes. Future studies are needed to elucidate the mechanisms underlying such differences.
The basal inflammatory state of an individual is highly relevant in the context depression (Maes et al., 1997). We demonstrated that Alox5−/− mice at basal conditions already exhibit increased levels of splenic IL-1β and IL-17, which are associated with behavioral changes. They also develop exacerbated inflammatory responses and become highly susceptible to a model of sterile inflammation (Zoccal et al., 2016), suggesting that the molecular mechanisms underlying the behavioral modifications of Alox5−/− mice at basal conditions are directly involved with a deregulated basal inflammatory profile. Thus, non-stressed Alox5−/− animals could already release cytokines at high levels (i.e., the ceiling effect), justifying why stress did not cause a further increase of these mediators.
Strikingly, we found that CUS exposition results in increased PGE2 production, whereby CUS-Alox5−/− mice exhibited even higher levels compared to CUS-129sv. We have demonstrated that PGE2 boosts IL-1β production by innate immune cells (Zoccal et al., 2016, 2018). The release of this cytokine is conditioned to the activation of inflammatory caspases (Friedlander et al., 1996; Rano et al., 1997). Higher levels of PGE2 were associated with increased caspase-1 expression in basal conditions of Alox5−/− and CUS-129sv mice, suggesting that, beyond PGE2 signaling, LTB4 also represses caspase-1 expression to limit IL-1β release. Paugh et al. (2015) demonstrated that higher caspase-1 expression augments GR cleavage, resulting in elevated glucocorticoid resistance. GR regulation is an important checkpoint during corticosterone production. Of note, GR expression was significantly reduced in the hippocampus of CUS-129sv, suggesting that levels of systemic corticosterone increase due a disruption on negative feedback, which was not observed in Alox5−/−. Despite the increased circulating levels of ACTH in Alox5−/− mice, they exhibited reduced corticosterone levels. These levels increased after CUS but remained significantly lower compared to CUS-129sv mice. Thus, 5-LO and related metabolites might regulate the systemic levels of corticosterone by promoting the expression and signaling of the ACTH receptor (MC2R) in the adrenal glands. However, future studies are needed to test this hypothesis.
Compared to Alox5−/− mice at basal conditions, CUS-Alox5−/− show increased levels of systemic corticosterone. Treatment with LTB4 reduced circulating corticosterone but did not cause any alteration on sucrose preference. Considering that Alox5−/− mice are unable to produce other molecules beyond LTB4, we cannot discard the involvement and synergy between LTC4, D4 or E4 in sucrose preference behavior. A recent study demonstrated that the knockdown if the cysteinyl-LT receptor 1 in the hippocampus reverses the depression-like behavior of mice submitted to CMS (Yu et al., 2016). LTB4 treatment was unable to reduce the decreased sucrose preference, but regulated caspase-1 expression, IL-1β release, and corticosterone production. These findings suggest that, while LTB4 controls the production of mediators involved in the inflammatory response, decreased sucrose preference could be directly regulated by cysteinyl-LTs. Further investigation will reveal the real contribution of these cysteinyl-LTs and LTB4 to the onset and progression of depression. Patients with major depressive disorders have lower levels of eicosanoid precursors, the polyunsaturated fatty acids (PUFA), which is associated with the severity of depressive symptoms (Adams et al., 1996). Antidepressant effects in these patients might involve eicosanoid cascades such as PGE2 formation (Su, 2009). Drugs such as imipramine, desipramine, and clomipramine reduce systemic levels of corticosterone in rats submitted to force swimming stress test, which prolonged swimming time (Yamada et al., 1987). Similar to LTB4 treatment, several antidepressants reduced caspase-1 expression and IL-1β production (Alcocer-Gómez et al., 2017). While LTB4 treatment did not affect sucrose preference, decreased inflammation induced by the treatment with LTB4 or antidepressants might account for changes in other behavioral parameters. A limitation of this study is that there was no group treated with antidepressant. Therefore, future studies comparing the effects of LTB4 and antidepressants in 129sv and Alox5−/− mice are needed to address this problem.
A previous work demonstrated that exogenous administration of LTB4 activates the HPA axis via BLT1 receptor, increasing plasma levels of ACTH and corticosterone while downregulating the inflammatory response during asthma (Zhang et al., 2010). However, our study is the first to demonstrate that 5-LO and related metabolites promote differential regulation of the systemic levels of ACTH and corticosterone, impacting stress and inflammation. This is highly relevant to explain the controversial findings of the role of LTB4 in infectious diseases. It is well recognized that LTB4 increases phagocytosis of microorganisms in vitro (Serezani et al., 2005; Secatto et al., 2014; Prado et al., 2017), as does dexamethasone (van der Goes et al., 2000). However, the results regarding LTB4 function in vivo are conflicting. For example, different outcomes have been described for experimental tuberculosis using LTB4 pharmacological inhibition or gene deletion. Indeed, treatment with MK886 inhibits LTB4 production and increases bacterial burden and mortality of mice infected with Mycobacterium tuberculosis (Peres et al., 2007). In contrast, Alox5−/− mice are more resistant to M. tuberculosis infection, have lower bacterial burden, and survive longer than 129sv animals (Bafica et al., 2005). These opposing results generate a paradox regarding the importance of LTB4 in the control of long-lived bacterial infections such as M. tuberculosis. Based on our present data, we suggest that the low levels of corticosterone observed in Alox5−/− mice favors the host immune response by promoting a quick pathogen elimination. Although major depression is a polygenic condition and depends on the interaction with environmental factors (Kendler et al., 1995), the results indicate that a decrease in 5-LO expression could be associated with this disorder.
In summary (Figure 8; Supplementary Table S1), we demonstrated that CUS results in significant modifications in 129sv mice, increasing corticosterone levels and reducing GR expression in hippocampus. In addition, the HPA axis is activated, resulting in increased circulating corticosterone and a series of other organ responses, such as increased caspase-1 expression in the spleen and adrenal glands; increased levels of splenic 11βHSD1 expression and IL-1β and PGE2 production; decreased levels of splenic LTB4 and 11βHSD2 expression. Basically, the same phenomena occur in Alox5−/− mice at basal conditions. 5-LO and related metabolites control caspase-1 expression, IL-1β, and PGE2 release. This inflammatory environment possibly increases HPA activation. The adrenal glands, however, seem to be unable to produce enough corticosterone, which results in lower circulating amounts of this hormone, even after CUS exposition.
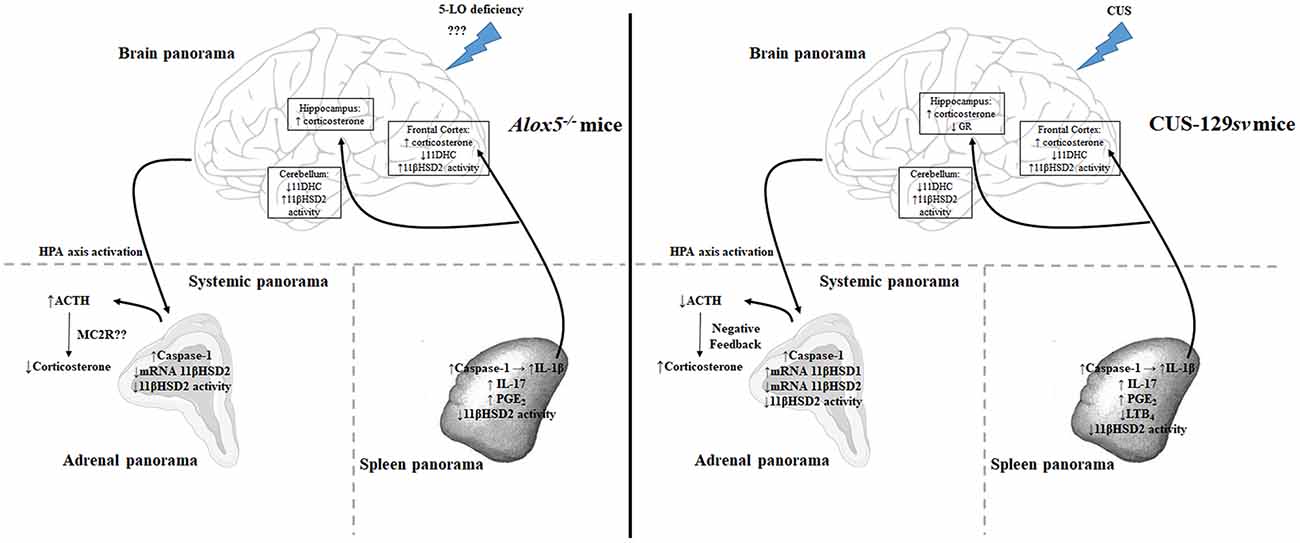
Figure 8. Alox5−/− mice display a depressed-like behavior at basal conditions comparable to that of 129sv animals exposed to CUS. In general, corticosterone levels and 11βHSD2 activity increased and 11DHC levels decreased in the brains of Alox5−/− and CUS-129sv mice. These animals also exhibited increased caspase-1 expression in the adrenal gland and spleen associated with increased levels of splenic IL-1β. In addition, 5-LO deficiency at basal conditions or CUS exposure of 129sv mice decreased 11βHSD2 mRNA expression and/or activity in the adrenal gland and spleen, while increasing IL-17 and PGE2 or decreasing LTB4 production in the spleen. However, 5-LO deficiency culminates in elevated basal activation of the HPA axis, reflected by high levels of circulating ACTH but reduced levels of systemic corticosterone, possibly due to the impaired expression of the ACTH receptor (MC2R) in the adrenal glands (systemic panorama—left panel). In contrast, CUS-129sv mice exhibited reduced circulating levels of ACTH and increased corticosterone (systemic panorama—right panel) and decreased GR expression in the hippocampus (brain panorama—right panel).
In conclusion, this study provides important insights into the role of 5-LO and related metabolites in the control of stress-induced mediators and inflammation. suggesting that Alox5−/− mice could be a novel model for the understanding of the molecular mechanisms responsible for decreased sensitivity to rewards.
Ethics Statement
This study was carried out in accordance with the recommendations of National Council for Control of Animal Experimentation (CONCEA). The protocol was approved by the Ethics Committee on Animal Use (CEUA) of the Campus of Ribeirão Preto (Protocol number: 14.1.290.53.5).
Author Contributions
GL designed the research, analyzed the data and prepared the figures. GL and MP executed the main experimental procedures and wrote the article. KZ, PP, MB, and AP assisted with laboratory procedures. MF assisted with initial CUS protocol. LF, CS, EDB and FG analyzed the data and discussed the hypothesis. FG performed statistical analysis. LF conceived and supervised the project, designed the experiments, obtained the funding grants, helped with the data interpretation, wrote and discussed the manuscript and acted as scientific advisor.
Funding
This work was supported by the São Paulo Research Foundation (Fundação de Amparo à Pesquisa do Estado de São Paulo, FAPESP; grant #2014/07125-6 to LF; EMU grant #2015/00658-1 to LF), Coordenação de Aperfeiçoamento de Pessoal de Nível Superior (CAPES—Finance Code 001), and from Conselho Nacional de Pesquisa (CNPq). The funders had no role in the study design, data collection and analysis, decision to publish, or preparation of the article.
Conflict of Interest Statement
The authors declare that the research was conducted in the absence of any commercial or financial relationships that could be construed as a potential conflict of interest.
Acknowledgments
We would like to thank the Laboratório de Fisiologia from Departamento de Patologia Clínica, Universidade de Campinas (UNICAMP) for help with ACTH measurements, and Fabiana R. Morais and Celia Ap. da Silva for technical assistance.
Supplementary Material
The Supplementary Material for this article can be found online at: https://www.frontiersin.org/articles/10.3389/fnbeh.2019.00073/full#supplementary-material
Footnotes
References
Adams, P. B., Lawson, S., Sanigorski, A., and Sinclair, A. J. (1996). Arachidonic acid to eicosapentaenoic acid ratio in blood correlates positively with clinical symptoms of depression. Lipids 31, S157–S161. doi: 10.1007/bf02637069
Alcocer-Gómez, E., Casas-Barquero, N., Williams, M. R., Romero-Guillena, S. L., Cañadas-Lozano, D., Bullón, P., et al. (2017). Antidepressants induce autophagy dependent-NLRP3-inflammasome inhibition in major depressive disorder. Pharmacol. Res. 121, 114–121. doi: 10.1016/j.phrs.2017.04.028
Alcocer-Gómez, E., de Miguel, M., Casas-Barquero, N., Núñez-Vasco, J., Sánchez-Alcazar, J. A., Fernández-Rodríguez, A., et al. (2014). NLRP3 inflammasome is activated in mononuclear blood cells from patients with major depressive disorder. Brain Behav. Immun. 36, 111–117. doi: 10.1016/j.bbi.2013.10.017
Athayde, L. A., Oliveira-Pelegrin, G. R., Nomizo, A., Faccioli, L. H., and Alves Rocha, M. J. (2009). Blocking central leukotriene synthesis affects vasopressin release during sepsis. Neuroscience 160, 829–836. doi: 10.1016/j.neuroscience.2009.03.004
Bafica, A., Scanga, C. A., Serhan, C., Machado, F., White, S., Sher, A., et al. (2005). Host control of Mycobacterium tuberculosis is regulated by 5-lipoxygenase-dependent lipoxin production. J. Clin. Invest. 115, 1601–1606. doi: 10.1172/JCI23949
Beurel, E., Harrington, L. E., and Jope, R. S. (2013). Inflammatory T helper 17 cells promote depression like behavior in mice. Biol. Psychiatry 73, 622–630. doi: 10.1016/j.biopsych.2012.09.021
Chen, X. S., Sheller, J. R., Johnson, E. N., and Funk, C. D. (1994). Role of leukotrienes revealed by targeted disruption of the 5-lipoxygenase gene. Nature 372, 179–182. doi: 10.1038/372179a0
Chisaka, H., Johnstone, J. F., Premyslova, M., Manduch, Z., and Challis, J. R. G. (2005). Effect of pro-inflammatory cytokines on expression and activity of 11β-hydroxysteroid dehydrogenase type 2 in cultured human term placental trophoblast and human choriocarcinoma JEG-3 cells. J. Soc. Gynecol. Investig. 12, 303–309. doi: 10.1016/j.jsgi.2005.02.003
Chu, J., and Praticò, D. (2009). The 5-lipoxygenase as a common pathway for pathological brain and vascular aging. Cardiovasc. Psychiatry Neurol. 2009:174657. doi: 10.1155/2009/174657
Cohen, S., Janicki-Deverts, D., Doyle, W. J., Miller, G. E., Frank, E., Rabin, B. S., et al. (2012). Chronic stress, glucocorticoid receptor resistance, inflammation and disease risk. Proc. Natl. Acad. Sci. U S A 109, 5995–5999. doi: 10.1073/pnas.1118355109
Dantzer, R. (2001). Cytokine-induced sickness behavior: where do we stand? Brain Behav. Immun. 15, 7–14. doi: 10.1006/brbi.2000.0613
Dantzer, R., O’Connor, J. C., Freund, G. G., Johnson, R. W., and Kelley, K. W. (2008). From inflammation to sickness and depression: when the immune system subjugates the brain. Nat. Rev. Neurosci. 9, 46–57. doi: 10.1038/nrn2297
Filgueiras, L. R., Brandt, S. L., Wang, S., Wang, Z., Morris, D. L., Evans-Molina, C., et al. (2015). Leukotriene B4-mediated sterile inflammation favors susceptibility to sepsis in murine type 1 diabetes. Sci. Signal. 8:ra10. doi: 10.1126/scisignal.2005568
Friedlander, R. M., Gagliardini, V., Rotello, R. J., and Yuan, J. (1996). Functional role of interleukin 1β (IL-1β) in IL-lβ-converting enzyme-mediated apoptosis. J. Exp. Med. 184, 717–724. doi: 10.1084/jem.184.2.717
Funk, C. D. (2001). Prostaglandins and leukotrienes: advances in eicosanoid biology. Science 294, 1871–1875. doi: 10.1126/science.294.5548.1871
Galvão, A. F., Petta, T., Flamand, N., Bollela, V. R., Silva, C. L., Jarduli, L. R., et al. (2016). Plasma eicosanoid profiles determined by high-performance liquid chromatography coupled with tandem mass spectrometry in stimulated peripheral blood from healthy individuals and sickle cell anemia patients in treatment. Anal. Bioanal. Chem. 408, 3613–3623. doi: 10.1007/s00216-016-9445-8
Gingrich, J. A., and Hen, R. (2000). The broken mouse: the role of development, plasticity and environment in the interpretation of phenotypic changes in knockout mice. Curr. Opin. Neurobiol. 10, 146–152. doi: 10.1016/s0959-4388(99)00061-6
Goshen, I., and Yirmiya, R. (2009). Interleukin-1 (IL-1): a central regulator of stress responses. Front. Neuroendocrinol. 30, 30–45. doi: 10.1016/j.yfrne.2008.10.001
Guan, X. T., Shao, F., Xie, X., Chen, L., and Wang, W. (2014). Effects of aspirin on immobile behavior and endocrine and immune changes in the forced swimming test: comparison to fluoxetine and imipramine. Pharmacol. Biochem. Behav. 124, 361–366. doi: 10.1016/j.pbb.2014.07.002
Guo, J. Y., Li, C. Y., Ruan, Y. P., Sun, M., Qi, X. L., Zhao, B. S., et al. (2009). Chronic treatment with celecoxib reverses chronic unpredictable stress-induced depressive-like behavior via reducing cyclooxygenase-2 expression in rat brain. Eur. J. Pharmacol. 612, 54–60. doi: 10.1016/j.ejphar.2009.03.076
Haeggström, J. Z., and Funk, C. D. (2011). Lipoxygenase and leukotriene pathways: biochemistry, biology and roles in disease. Chem. Rev. 111, 5866–5898. doi: 10.1021/cr200246d
Harizi, H., Corcuff, J. B., and Gualde, N. (2008). Arachidonic-acid-derived eicosanoids: roles in biology and immunopathology. Trends Mol. Med. 14, 461–469. doi: 10.1016/j.molmed.2008.08.005
Herane Vives, A. H., De Angel, V., Papadopoulos, A., Strawbridge, R., Wise, T., Young, A. H., et al. (2015). The relationship between cortisol, stress and psychiatric illness: new insights using hair analysis. J. Psychiatr. Res. 70, 38–49. doi: 10.1016/j.jpsychires.2015.08.007
Hirai, A., Tahara, K., Tamura, Y., Saito, H., Terano, T., and Yoshida, S. (1985). Involvement of 5-lipoxygenase metabolites in ACTH-stimulated corticosteroidogenesis in rat adrenal glands. Prostaglandins 30, 749–767. doi: 10.1016/0090-6980(85)90005-x
Irwin, M. R., and Miller, A. H. (2007). Depressive disorders and immunity: 20 years of progress and discovery. Brain Behav. Immun. 21, 374–383. doi: 10.1016/j.bbi.2007.01.010
Jeon, S. W., and Kim, Y. U. (2016). Neuroinflammation and cytokine abnormality in major depression: cause or consequence in that illness? World J. Psychiatry 6, 283–293. doi: 10.5498/wjp.v6.i3.283
Joshi, Y. B., Giannopoulos, P. F., and Praticò, D. (2015). The 12/15-lipoxygenase as an emerging therapeutic target for Alzheimer’s disease. Trends Pharmacol. Sci. 36, 181–186. doi: 10.1016/j.tips.2015.01.005
Joshi, Y. B., and Praticò, D. (2013). The involvement of 5-lipoxygenase activating protein in anxiety-like behavior. J. Psychiatr. Res. 47, 694–698. doi: 10.1016/j.jpsychires.2012.12.011
Kajantie, E., Dunkel, L., Turpeinen, U., Stenman, U. H., Wood, P. J., Nuutila, M., et al. (2003). Placental 11β-hydroxysteroid dehydrogenase-2 and fetal cortisol/cortisone shuttle in small preterm infants. J. Clin. Endocrinol. Metab. 88, 493–500. doi: 10.1210/jc.2002-021378
Kendler, K. S., Kessler, R. C., Walters, E. E., MacLean, C., Neale, M. C., Heath, A. C., et al. (1995). Stressful life events, genetic liability, and onset of an episode of major depression in women. Am. J. Psychiatry 152, 833–842. doi: 10.1176/ajp.152.6.833
Krozowski, Z., Li, K. X. Z., Koyama, K., Smith, R. E., Obeyesekere, V. R., Stein-Oakley, A., et al. (1999). The type I and type II 11β-hydroxysteroid dehydrogenase enzymes. J. Steroid Biochem. Mol. Biol. 69, 391–401. doi: 10.1016/S0960-0760(99)00074-6
Locachevic, G. A., Pereira, P. A. T., Secatto, A., Fontanari, C., Galvão, A. F., Prado, M. K. B., et al. (2015). Erythropoietin exacerbates inflammation and increases the mortality of histoplasma capsulatum-infected mice. Mediators Inflamm. 2015:786319. doi: 10.1155/2015/786319
Maes, M., Bosmans, E., De Jongh, R., Kenis, G., Vandoolaeghe, E., and Neels, H. (1997). Increased serum IL-6 and IL-1 receptor antagonist concentrations in major depression and treatment resistant depression. Cytokine 9, 853–858. doi: 10.1006/cyto.1997.0238
Medeiros, A. I., Sá-Nunes, A., Turato, W. M., Secatto, A., Frantz, F. G., Sorgi, C. A., et al. (2008). Leukotrienes are potent adjuvant during fungal infection: effects on memory T cells. J. Immunol. 181, 8544–8551. doi: 10.4049/jimmunol.181.12.8544
Miller, A. H., and Raison, C. L. (2016). The role of inflammation in depression: from evolutionary imperative to modern treatment target. Nat. Rev. Immunol. 16, 22–34. doi: 10.1038/nri.2015.5
Müller, N., Schwarz, M. J., Dehning, S., Douhe, A., Cerovecki, A., Goldstein-Müller, B., et al. (2006). The cyclooxygenase-2 inhibitor celecoxib has therapeutic effects in major depression: results of a double-blind, randomized, placebo controlled, add-on pilot study to reboxetine. Mol. Psychiatry 11, 680–684. doi: 10.1038/sj.mp.4001805
Paugh, S. W., Bonten, E. J., Savic, D., Ramsey, L. B., Thierfelder, W. E., Gurung, P., et al. (2015). NALP3 inflammasome upregulation and CASP1 cleavage of the glucocorticoid receptor cause glucocorticoid resistance in leukemia cells. Nat. Genet. 47, 607–614. doi: 10.1038/ng.3283
Pereira, P. A. T., Trindade, B. C., Secatto, A., Nicolete, R., Peres-Buzalaf, C., Ramos, S. G., et al. (2013). Celecoxib improves host defense through prostaglandin inhibition during Histoplasma capsulatum infection. Mediators Inflamm. 2013:950981. doi: 10.1155/2013/950981
Peres, C. M., de Paula, L., Medeiros, A. I., Sorgi, C. A., Soares, E. G., Carlos, D., et al. (2007). Inhibition of leukotriene biosynthesis abrogates the host control of Mycobacterium tuberculosis. Microbes Infect. 9, 483–489. doi: 10.1016/j.micinf.2007.01.006
Peti, A. P. F., Locachevic, G. A., Prado, M. K. B., Moraes, L. A. B., and Faccioli, L. H. (2018). High-resolution multiple reaction monitoring method for quantification of steroidal hormones in plasma. J. Mass Spectrom. 53, 423–431. doi: 10.1002/jms.3998
Pitman, D. L., Ottenweller, J. E., and Natelson, B. H. (1988). Plasma corticosterone levels during repeated presentation of two intensities of restraint stress: chronic stress and habituation. Physiol. Behav. 43, 47–55. doi: 10.1016/0031-9384(88)90097-2
Pothion, S., Bizot, J. C., Trovero, F., and Belzung, C. (2004). Strain differences in sucrose preference and in the consequences of unpredictable chronic mild stress. Behav. Brain Res. 155, 135–146. doi: 10.1016/j.bbr.2004.04.008
Prado, M. K. B., Locachevic, G. A., Zoccal, K. F., Paula-Silva, F. W. G., Fontanari, C., Ferreira, J. C., et al. (2017). Leukotriene B4 is essential for lung host defence and alpha-defensin-1 production during Achromobacter xylosoxidans infection. Sci. Rep. 7:17658. doi: 10.1038/s41598-017-17993-9
Rådmark, O., Werz, O., Steinhilber, D., and Samuelsson, B. (2015). 5-Lipoxygenase, a key enzyme for leukotriene biosynthesis in health and disease. Biochim. Biophys. Acta 1851, 331–339. doi: 10.1016/j.bbalip.2014.08.012
Raison, C. L., Capuron, L., and Miller, A. H. (2006). Cytokines sing the blues: inflammation and the pathogenesis of depression. Trends Immunol. 27, 24–31. doi: 10.1016/j.it.2005.11.006
Rano, T. A., Timkey, T., and Peterson, E. P. (1997). A combinatorial approach for determining protease specificities: application to interleukin-1β converting enzyme (ICE). Chem. Biol. 4, 149–155. doi: 10.1016/s1074-5521(97)90258-1
Rodriguez, J. M., Monsalves-Alvarez, M., Henriquez, S., Llanos, M. N., and Troncoso, R. (2016). Glucocorticoid resistance in chronic diseases. Steroids 115, 182–192. doi: 10.1016/j.steroids.2016.09.010
Samuelsson, B. (2000). The discovery of the leukotrienes. Am. J. Respir. Crit. Care Med. 161, S2–S6. doi: 10.1164/ajrccm.161.supplement_1.ltta-1
Sato, K., Chisaka, H., Okamura, K., and Challis, J. R. G. (2008). Effect of the interaction between lipoxygenase pathway and progesterone on the regulation of hydroxysteroid 11-beta dehydrogenase 2 in cultured human term placental trophoblasts. Biol. Reprod. 78, 514–520. doi: 10.1095/biolreprod.107.064717
Secatto, A., Rodrigues, L. C., Serezani, C. H., Ramos, S. G., Dias-Baruffi, M., Faccioli, L. H., et al. (2012). 5-lipoxygenase deficiency impairs innate and adaptive immune responses during fungal infection. PLoS One 7:e31701. doi: 10.1371/journal.pone.0031701
Secatto, A., Soares, E. M., Locachevic, G. A., Assis, P. A., Paula-Silva, F. W. G., Serezani, C. H., et al. (2014). The leukotriene B4/BLT1 axis is a key determinant in susceptibility and resistance to histoplasmosis. PLoS One 9:e85083. doi: 10.1371/journal.pone.0085083
Serezani, C. H., Aronoff, D. M., Jancar, S., Mancuso, P., and Peters-Golden, M. (2005). Leukotrienes enhance the bactericidal activity of alveolar macrophages against Klebsiella pneumoniae through the activation of NADPH oxidase. Blood 106, 1067–1075. doi: 10.1182/blood-2004-08-3323
Smith, R. S. (1991). The macrophage theory of depression. Med. Hypotheses 35, 298–306. doi: 10.1016/0306-9877(91)90272-z
Smith, S. M., and Vale, W. W. (2006). The role of the hypothalamic-pituitary-adrenal axis in neuroendocrine responses to stress. Dialogues Clin. Neurosci. 8, 383–395.
Stewart, P. M., Rogerson, F. M., and Mason, J. I. (1995). Type 2 11 beta-hydroxysteroid dehydrogenase messenger ribonucleic acid and activity in human placenta and fetal membranes: its relationship to birth weight and putative role in fetal adrenal steroidogenesis. J. Clin. Endocrinol. Metab. 80, 885–890. doi: 10.1210/jcem.80.3.7883847
Strowig, T., Henao-Mejia, J., Elinav, E., and Flavell, R. (2012). Inflammasomes in health and disease. Nature 481, 278–286. doi: 10.1038/nature10759
Su, K. P. (2009). Biological mechanism of antidepressant effect of omega-3 fatty acids: how does fish oil act as a ‘mind-body interface’? Neurosignals 17, 144–152. doi: 10.1159/000198167
Tetsuka, M., Haines, L. C., Milne, M., Simpson, G. E., and Hillier, S. G. (1999). Regulation of 11β-hydroxysteroid dehydrogenase type 1 gene expression by LH and interleukin-1β in cultured rat granulosa cells. J. Endocrinol. 163, 417–423. doi: 10.1677/joe.0.1630417
Tymen, S. D., Rojas, I. G., Zhou, X., Fang, Z. J., Zhao, Y., and Marucha, P. T. (2013). Restraint stress alters neutrophil and macrophage phenotypes during wound healing. Brain Behav. Immun. 28, 207–217. doi: 10.1016/j.bbi.2012.07.013
Uz, T., Dimitrijevic, N., Imbesi, M., Manev, H., and Manev, R. (2008). Effects of MK-886, a 5-lipoxygenase activating protein (FLAP) inhibitor and 5-lipoxygenase deficiency on the forced swimming behavior of mice. Neurosci. Lett. 436, 269–272. doi: 10.1016/j.neulet.2008.03.041
Uz, T., Dwivedi, Y., Savani, P. D., Impagnatiello, F., Pandey, G., and Manev, H. (1999). Glucocorticoids stimulate inflammatory 5-lipoxygenase gene expression and protein translocation in the brain. J. Neurochem. 73, 693–699. doi: 10.1046/j.1471-4159.1999.0730693.x
van der Goes, A., Hoekstra, K., van den Berg, T. K., and Dijkstra, C. D. (2000). Dexamethasone promotes phagocytosis and bacterial killing by human monocytes/macrophages in vitro. J. Leukoc. Biol. 67, 801–807. doi: 10.1002/jlb.67.6.801
Willner, P. (1997). Validity, reliability and utility of the chronic mild stress model of depression: a 10-year review and evaluation. Psychopharmacology 134, 319–329. doi: 10.1007/s002130050456
World Health Organization (WHO). (2017). Depression. Available online at: http://www.who.int/mediacentre/factsheets/fs369/en/. [Accessed on January 17, 2011].
Yamada, K., Minoru, T., and Tetsuo, S. (1987). Effects of antidepressants on serum corticosterone level and swimming time in forced swimming rats: application to screening test of antidepressants. Res. Comm. Psychol. Psychiatr. Behav. 12, 96–104.
Yu, X. B., Dong, R. R., Wang, H., Lin, J. R., An, Y. Q., Du, Y., et al. (2016). Knockdown of hippocampal cysteinyl leukotriene receptor 1 prevents depressive behavior and neuroinflammation induced by chronic mild stress in mice. Psychopharmacology 233, 1739–1749. doi: 10.1007/s00213-015-4136-2
Zen, M., Canova, M., Campana, C., Bettio, S., Nalotto, L., Rampudda, M., et al. (2011). The kaleidoscope of glucocorticoid effects on immune system. Autoimmun. Rev. 10, 305–310. doi: 10.1016/j.autrev.2010.11.009
Zhang, S. J., Deng, Y. M., Zhu, Y. L., Dong, X. W., Jiang, J. X., and Xie, Q. M. (2010). Intracerebroventricular injection of leukotriene B4 attenuates antigen-induced asthmatic response via BLT1 receptor stimulating HPA-axis in sensitized rats. Respir. Res. 11:39. doi: 10.1186/1465-9921-11-39
Zhang, Y., Liu, L., Liu, Y. Z., Shen, X. L., Wu, T. Y., Zhang, T., et al. (2015). NLRP3 inflammasome mediates chronic mild stress-induced depression in mice via neuroinflammation. Int. J. Neuropsychopharmacol. 18:pyv006. doi: 10.1093/ijnp/pyv006
Zhao, J., Quyyumi, A. A., Patel, R., Zafari, A. M., Veledar, E., Onufrak, S., et al. (2009). Sex-specific association of depression and a haplotype in leukotriene A4 hydrolase gene. Psychosom. Med. 71, 691–696. doi: 10.1097/psy.0b013e3181b05c57
Zoccal, K. F., Ferreira, G. Z., Prado, M. K. B., Gardinassi, L. G., Sampaio, S. V., and Faccioli, L. H. (2018). LTB4 and PGE2 modulate the release of MIP-1α and IL-1β by cells stimulated with Bothrops snake venoms. Toxicon 150, 289–296. doi: 10.1016/j.toxicon.2018.06.066
Zoccal, K. F., Sorgi, C. A., Hori, J. I., Paula-Silva, F. W. G., Arantes, E. C., Serezani, C. H., et al. (2016). Opposing roles of LTB4 and PGE2 in regulating the inflammasome-dependent scorpion venom-induced mortality. Nat. Commun. 7:10760. doi: 10.1038/ncomms10760
Keywords: chronic unpredictable stress, depression, 5-lipoxygenase, prostaglandins, leukotrienes, IL-1β, caspase-1, glucocorticoid receptor
Citation: Locachevic GA, Prado MKB, Zoccal KF, Pereira PAT, Sorgi CA, Bortolanza M, Peti APF, Fogaça MV, Guimarães FS, Del Bel E and Faccioli LH (2019) Paradoxical Effect of LTB4 on the Regulation of Stress-Induced Corticosterone Production. Front. Behav. Neurosci. 13:73. doi: 10.3389/fnbeh.2019.00073
Received: 16 January 2019; Accepted: 26 March 2019;
Published: 16 April 2019.
Edited by:
Mathias V. Schmidt, Max-Planck-Institut für Psychiatrie, GermanyCopyright © 2019 Locachevic, Prado, Zoccal, Pereira, Sorgi, Bortolanza, Peti, Fogaça, Guimarães, Del Bel and Faccioli. This is an open-access article distributed under the terms of the Creative Commons Attribution License (CC BY). The use, distribution or reproduction in other forums is permitted, provided the original author(s) and the copyright owner(s) are credited and that the original publication in this journal is cited, in accordance with accepted academic practice. No use, distribution or reproduction is permitted which does not comply with these terms.
*Correspondence: Lúcia H. Faccioli, faccioli@fcfrp.usp.br
† These authors have contributed equally to this work