- 1Department of Psychology, Saint Joseph’s University, Philadelphia, PA, United States
- 2Department of Psychology, Rutgers University, Piscataway, NJ, United States
Natural variations in parenting are associated with differences in expression of several hormones and neuropeptides which may mediate lasting effects on offspring development, like regulation of stress reactivity and social behavior. Using the bi-parental California mouse, we have demonstrated that parenting and aggression are programmed, at least in part, by paternal behavior as adult offspring model the degree of parental behavior received in development and are more territorial following high as compared to low levels of care. Development of these behaviors may be driven by transient increases in testosterone following paternal retrievals and increased adult arginine vasopressin (AVP) immunoreactivity within the bed nucleus of the stria terminalis (BNST) among high-care (HC) offspring. It remains unclear, however, whether other neuropeptides, such as oxytocin (OT), which is sensitive to gonadal steroids, are similarly impacted by father-offspring interactions. To test this question, we manipulated paternal care (high and low care) and examined differences in adult offspring OT-immunoreactive (OT-ir) within social brain areas as well as basal T and corticosterone (Cort) levels. HC offspring had more OT-ir within the paraventricular nucleus (PVN) and supraoptic nucleus (SON) than low-care (LC) offspring. Additionally, T levels were higher among HC than LC females, but no differences were found in males. There were no differences in Cort indicating that our brief father-pup separations likely had no consequences on stress reactivity. Together with our previous work, our data suggest that social behavior may be programmed by paternal care through lasting influences on the neuroendocrine system.
Introduction
Variability within postnatal environments can have profound consequences on phenotype development in offspring. Stress reactivity and parental behavior, among other things, are programmed by the quality of care received (Bester-Meredith and Marler, 2003; Shannon et al., 2005; Ichise et al., 2006; Uriarte et al., 2007; Rosenfeld et al., 2013). This phenotypic plasticity is accompanied by changes to neural pathways associated with behavioral regulation (Champagne et al., 2004; Weaver et al., 2006; Oomen et al., 2009). While mothers are the primary caregiver in most mammalian species, in an estimated 5%–10% of mammals, fathers also contribute to offspring development (Gubernick and Alberts, 1987; Ziegler et al., 2000; Bester-Meredith and Marler, 2001). Within the bi-parental California mouse (Peromyscus californicus), fathers provide high-care (HC) towards both same and opposite sex offspring, which influence neuroendocrine mechanisms that facilitate similar rather than sexually dimorphic development. Transient increases in testosterone levels (Becker et al., 2010; Chary et al., 2015) and greater arginine vasopressin (AVP) expression in the bed nucleus of the stria terminalis (BNST; Frazier et al., 2006; Yohn et al., 2017), accompany territorial aggression in HC offspring. Whereas in most species parental behavior is accompanied by a reduction in aggression and T, in bi-parental species (Hume and Wynne-Edwards, 2005), like the territorial California mouse (Trainor and Marler, 2001, 2002), T remains high in fathers and is important for maintaining paternal behavior since castration reduces paternal behavior in this species (Trainor and Marler, 2001). The current study aimed to identify whether paternal care, which we have demonstrated programs both territoriality (Yohn et al., 2017) and parental behavior (Bester-Meredith and Marler, 2003; Gleason and Marler, 2013; Becker, unpublished; Leithead, unpublished) in adult California mouse offspring, influences other neuroendocrine mechanisms in addition to T and AVP that may act or interact to shape adult behavior.
The neuropeptide oxytocin (OT) is a likely candidate since it is synthesized in the paraventricular nucleus (PVN) and supraoptic nucleus (SON), with projections to social brain areas (Champagne et al., 2001 rats; Pedersen and Boccia, 2003 rats) that regulate social behaviors (Consiglio et al., 2005 rats) including parenting (Bales et al., 2007 prairie voles; Neumann and van den Burg, 2013 rats) and aggression, particularly parental aggression (Bosch, 2013 “rodents”) and hypothalamus-pituitary-adrenal (HPA) function (Neumann et al., 2000 rats; Engelmann et al., 2006 rats; Rault et al., 2013 pigs). Moreover, the OT system is sensitive to gonadal steroids, such as T (reviews see Pedersen, 1997; Sladek et al., 2000 rat; Gordon et al., 2011), which alone or by aromatization into estradiol acts as a modulator of OT secretion and receptor expression within brain areas (i.e., hypothalamus) that regulate both reproductive and parenting behavior (Johnson et al., 1991 rats; Okabe et al., 2013 mice; Gordon et al., 2017 humans). Furthermore, there is significant overlap in expression of OT and aromatase enzymes within the mammalian brain, with aromatase also mainly expressed within the hypothalamus and limbic system (Naftolin et al., 2001; Trainor et al., 2006; El-Emam Dief et al., 2013). Furthermore, developmental studies, in mandarin voles, indicate paternally deprived offspring have lower OT receptor expression than offspring raised with a father (Wang et al., 2012; Cao et al., 2014). Whether this is due to the absence of the caregiver or a particular behavior displayed by the father is unknown.
In addition to changes in the brain, environmental influences on social behaviors and stress reactivity may be mediated by alterations to endocrine systems (Bale, 2006; Clinton et al., 2008; Lajud et al., 2012; Carini and Nephew, 2013). For example, California mouse offspring experience transient increases in T in response to paternal retrievals (Becker et al., 2010; Chary et al., 2015). It is possible that experiencing these brief increases in T will result in overall increased basal T levels in adulthood since postnatal T is correlated with adult T (Sachser and Proöve, 1988; Lürzel et al., 2010), although this hypothesis has yet to be tested. Additionally, paternal deprivation leads to deficits in social behavior (Yu et al., 2012; Bambico et al., 2015) and increased anxiety (McEwen, 2007; Roberts et al., 2007; Jia et al., 2009; Kim et al., 2013), which may correlate with HPA dysregulation since in response to stress, corticosterone (Cort) is secreted. However, no transient differences in Cort in response to paternal care (Becker et al., 2010; Chary et al., 2015) nor basal Cort dissimilarities between paternal absence or presence (Wang et al., 2012) have been reported.
Postnatal paternal care impacts the development of social behaviors and may be regulated by a complex interplay between the hormones OT, T and Cort. For instance, OT expression can be increased via T (El-Emam Dief et al., 2013), and then have a buffering effect on the HPA axis, leading to a decrease in Cort release (Leuner et al., 2012). To examine long-term effects of postnatal paternal interactions on neuroendocrine system development, we manipulated California mouse offspring rearing conditions to receive either HC or low-care (LC). Given that removal of the father leads to decreased OT expression (Wang et al., 2012; Cao et al., 2014), we aimed to assess whether variability in paternal care leads to plasticity within the OT system. In the current study, our primary aim was to assess paternal care impact on OT-immunoreactive (OT-ir) cell distribution in the PVN and SON, with these three areas of the brain sensitive to gonadal steroids (El-Emam Dief et al., 2013) and important in regulating stress response (Dabrowska et al., 2011). Therefore, we also assessed adult basal T and Cort levels since the paternal care manipulation can also have long-term effects on the endocrine system. We hypothesized that PVN and SON OT-ir would be higher in HC than LC offspring. Since California mouse pups experience transient increases in T following paternal retrievals (Becker et al., 2010; Chary et al., 2015) and postnatal surges in T lead to higher adult T levels (Sachser and Proöve, 1988; Lürzel et al., 2010), we predicted higher basal T levels in adult HC than LC offspring. Lastly, as a manipulation check, we measured basal Cort levels to confirm that our modified retrieval manipulation had no lasting effects on stress reactivity; predicting similar basal Cort levels between HC and LC offspring.
Materials and Methods
This study was carried out in accordance with the recommendations of the National Institutes of Health Guide for the Care and Use of Laboratory Animals. The protocol was approved by the Saint Joseph’s University IACUC. Detailed materials and methods are provided as Supplementary Material Information.
Subjects
Brains were collected from 52 California mice adults (120 days) from the same cohort of mice used in Yohn et al. (2017) study. Briefly, experimental animals postnatal days (PND) 15–21 were assigned randomly to either HC (n = 26) or LC (n = 26) postnatal paternal rearing conditions. As previously described by Yohn et al. (2017), we used a modified retrieval manipulation (use of Plexiglas divider) to ensure populations of experimental animals had distinct early life experiences (HC and LC). To establish populations, experimental families were first moved into an observational cage on PND 8. Subsequently, paternal retrieval behavior was manipulated for 20 min daily, across PND 15–21 when pups are more inclined to leave the nest and paternal retrieval behavior is at its highest (Bester-Meredith et al., 1999). During each daily manipulation the mother and non-experimental pups were removed and housed separately. The experimental pup was handled for 30 s and returned to the cage either inside the nest (LC) or outside of the nest (HC). Prior to the pups return to the cage, a Plexiglass divider was inserted into the cage separating the large and small compartments of the observational cage. Results from Yohn et al. (2017) showed that across 7 days, HC experienced greater paternal retrievals than LC offspring since the Plexiglass divider (had plastic mesh barrier for LC group) inhibited father-pup contact during the daily LC manipulations. Separation of father and pup had no effect on paternal behavior in the LC group once the father and pup were reunited (Yohn et al., 2017).
Immunohistochemistry
Adult experimental mice taken from the colony room were euthanized via rapid decapitation. Brains were extracted, immediately fixed in 5% acrolein overnight at 4°C, transferred to a 20% sucrose buffer solution and refrigerated for 48 h, and finally frozen on dry ice and stored at −80°C until cutting. Beginning from approximately Bregma 0.37 through −1.23 (Paxinos et al., 2007; Campi et al., 2013), brains were sliced on a cryostat at 40 um and stored at −80°C in cyroprotectant until staining. Sections were incubated overnight in a previously validated antibody (Trainor et al., 2010) rabbit anti-OT (1:1,000, AB911, Millipore, Temecula, CA, USA) and then for 2 h in goat anti-rabbit IgG (1:250, PI-1,000, Vector Labs, Burlingame, CA, USA) both times diluted in 2% normal goat serum (NGS) phosphate buffer saline (PBS). Next, sections were amplified in Avidin Biotin Complex (Vector Labs, Burlingame, CA, USA) and then visualized using DAB peroxidase substrate kit (Vector Labs, Burlingame, CA, USA). PBS washes occurred before and after incubation, amplification and visualization.
Image Analysis
Sections were photographed on a Leica DM 2000 outfitted with a DFC310 FX digital color camera (Leica) at 10× magnification. For all cell counts, number of OT-ir positive cells were averaged across two images of the PVN (posterior, Bregma −0.70 thru −94) and SON (Bregma −0.70 thru −94; Figure 1A).
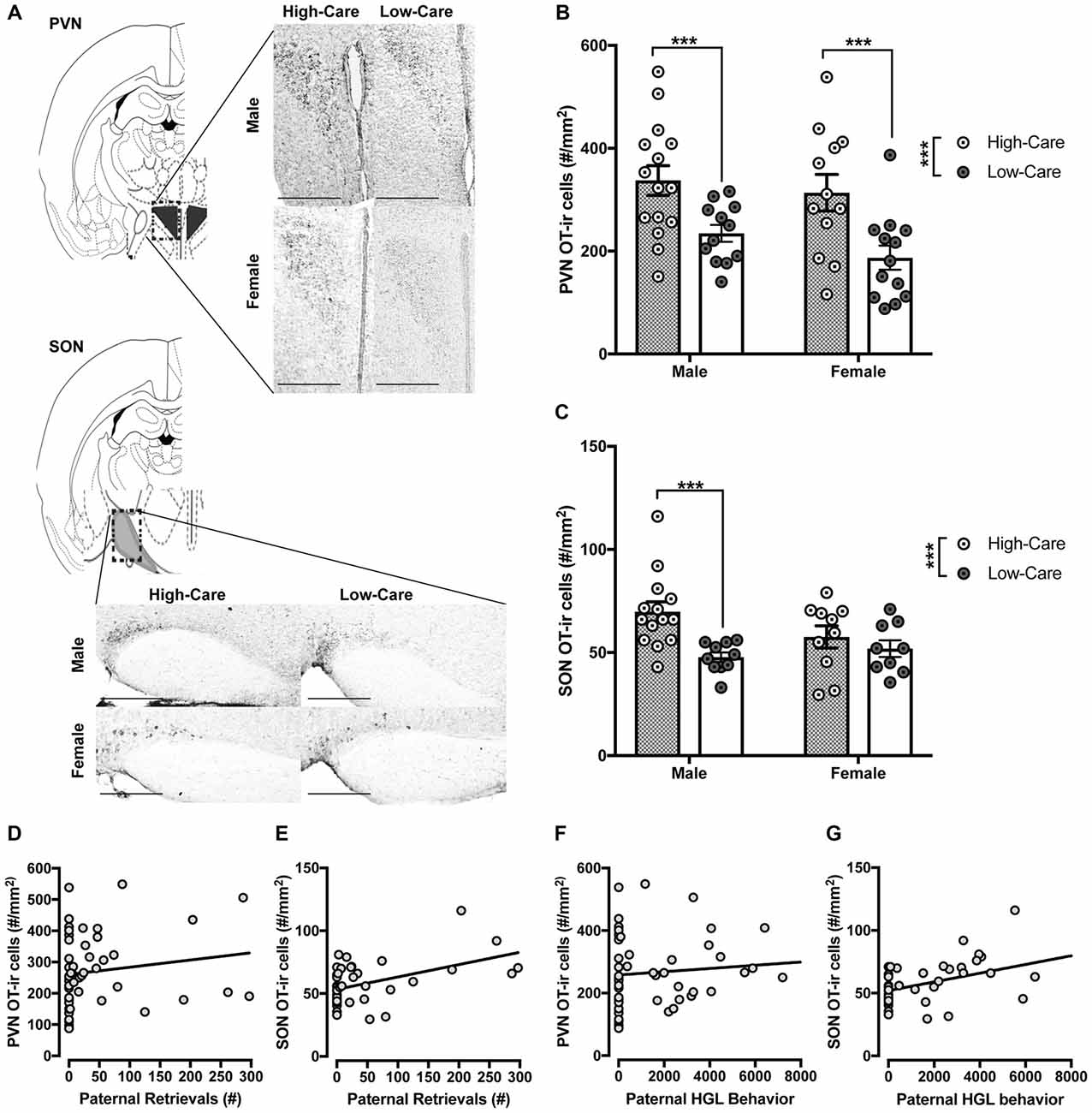
Figure 1. Differences in distribution of oxytocin-immunoreactive (OT-ir) cells between high-care (HC) and low-care (LC) offspring. Representative images of OT-ir staining for each area of interest (atlas images were reproduced from Paxinos mouse atlas, 2007; 10× magnification with scale bar = 500 μm) (A). HC male and female offspring have higher OT-ir cells within the paraventricular nucleus (PVN; B) and supraoptic nucleus (SON; C) compared to LC offspring. Number of postnatal paternal is significantly associated with amount of OT-ir cells within the PVN (D) and SON (E). Amount of postnatal paternal huddling, grooming and licking (HGL) behavior also was significantly associated with distribution of OT-ir cells in these areas (F,G). ***p-value < 0.001, *p-value < 0.05.
Testosterone and Corticosterone Enzyme Immunoassay
Trunk blood was collected at brain extraction, with enough serum from 39 of 52 experimental mice (male = 20, female = 19). After collection, samples were centrifuged and separated, then stored at −80°C until assayed. Plasma T (1:10 dilution) and Cort (1:50 dilution) concentrations were determined using commercial assay kits (Enzo Life Sciences, Farmingdale, NY, USA) previously validated in the California mouse (Chary et al., 2015). The standard curve slope generated for Cort had a slope of 1 (r2 = 0.91) and the slope for T was 0.72 (r2 = 0.87). Inter-assay coefficient of variability values were 1.1% (Cort) and 3.1% (T) with intra-assay coefficient of variability values being 1.87% (Cort) and 2.65% (T). The cross-reactivity of the Cort kits, according to the manufacturer, was 100% for Cort, 28.6% for deoxycorticosterone, 1.7% for progesterone, and negligible for other steroid hormones (>1%). The cross-reactivity of the T kits was 100% for T, 14.64% for 19-hydroxytestosterone, 7.20% for androstendione, and negligible for other steroid hormones (>1%). Kit sensitivity was 26.99 pg/mL for Cort and 5.67 pg/mL for T.
Statistical Analyses
Separate 2 × 2 analysis of variance (ANOVA) were run to assess early life rearing conditions and sex differences on PVN and SON OT-ir and basal T and CORT levels. Post hoc independent samples t-tests for within-sex differences were run. Pearson’s correlations were run to assess relationship between paternal behavior and expression of OT-ir within each area. One animal (female LC) was removed from analyses as cell counts were only obtained from one of the three regions and T levels were three standard deviations above the mean. All statistical analyses were conducted using SPSS (version 23.0, Chicago, IL, USA).
Results
OT-Immunoreactivity
Separate ANOVAs indicated HC offspring had significantly more OT-ir in the PVN (F(1,48) = 17.49, p < 0.001; Figure 1B) and SON (F(1,39) = 9.1, p = 0.004; Figure 1C) than LC offspring. Planned post hoc comparisons revealed HC males had significantly more OT-ir than LC males within the PVN (t(25) = 2.89, p = 0.008; Figure 1B) and SON (t(22) = 3.6, p = 0.002; Figure 1C). Within females, HC offspring had more OT-ir in the PVN (t(23) = 3.01, p = 0.006; Figure 1B) than LC offspring. Unlike males, SON OT was similarly expressed between HC and LC females (p = 0.421; Figure 1C). We observed no effect of sex nor an interaction between rearing condition and sex on OT-ir within these regions (p’s > 0.2).
Relationship Between OT-Immunoreactivity and Early Life Rearing Conditions
Paternal retrievals were positively correlated with PVN (r = 0.44, p = 0.02; Figure 1D) and SON (r = 0.47, p < 0.001; Figure 1E) OT-ir. Additionally, paternal huddling, grooming and licking (HGL) behavior was positively correlated with PVN (r = 0.34, p = 0.016; Figure 1F), SON (r = 0.44, p = 0.003; Figure 1G) OT-ir.
Plasma Corticosterone and Testosterone Concentrations
As expected, males had higher plasma T levels than females (F(1,33) = 7.58, p = 0.009; Figure 2A). While there was no main effect of rearing condition on plasma T concentrations (p = 0.7) nor differences among males (p = 0.69); rearing effects were indicated with higher T levels in HC than LC females, (t(15) = 2.27, p = 0.039). No rearing effects nor an interaction effect on plasma T levels (p’s > 0.05) were found.
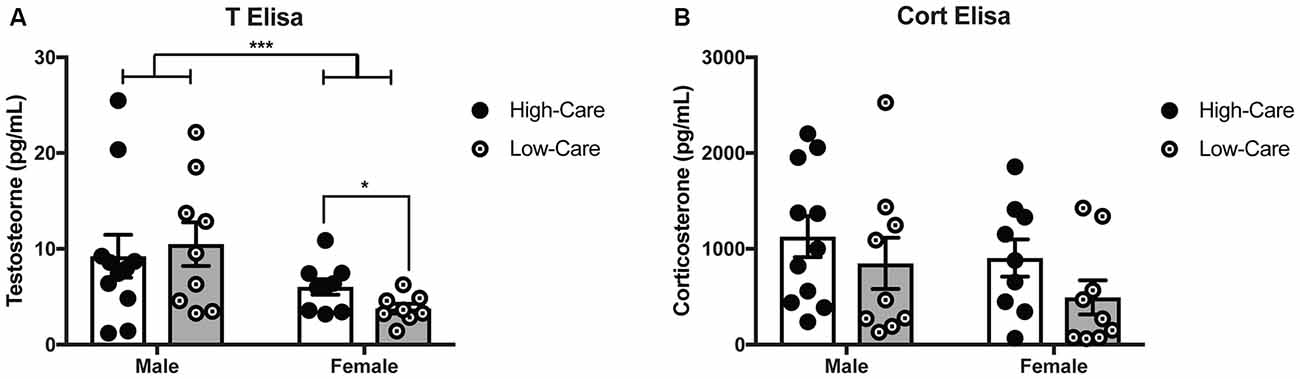
Figure 2. Differences in early life paternal care on hormonal levels. HC females have higher T levels than LC females, with males having higher overall testosterone levels females (A). Corticosterone (Cort) levels did not differ between HC and LC adult offspring across both sexes (B). ***p-value < 0.001, *p-value < 0.05.
No rearing effects, sex differences, nor an interaction were found for plasma Cort concentrations (p’s > 0.05; Figure 2B).
Discussion
Variability in paternal care produced differences in offspring OT-ir. Pups raised under HC conditions had greater OT-ir than LC offspring within the PVN and SON, regions that regulate various behaviors ranging from parenting (Neumann and van den Burg, 2013; Wang et al., 2015) to affective behaviors and autonomic functions (Cao et al., 2014; Yee et al., 2016). Within the PVN, OT expression is linked to the onset and maintenance of both maternal (Neumann and van den Burg, 2013) and paternal care (Kenkel et al., 2014). Since paternal care programs adult offspring social behavior in the California mouse (Bester-Meredith and Marler, 2001; Frazier et al., 2006; Yohn et al., 2017), we suggest that these differences in OT may guide the development of these distinct behavioral phenotypes. Previous studies delineate the relationship between paternal care and OT receptor expression within the PVN and SON (Wang et al., 2012; Cao et al., 2014), our novel findings illustrate susceptibility of PVN, and SON OT-ir to postnatal paternal care. However, unlike paternal deprivation studies, we emphasize the importance of paternal behaviors on adult OT-ir with high levels of both paternal retrievals and HGL behavior positively related to PVN and SON OT-ir. In response to paternal retrievals HC offspring experience transient increases in T (Becker et al., 2010; Chary et al., 2015), with OT expression within these brain areas regulated in part by T (Sladek et al., 2000; Gordon et al., 2011) and the aromatization of T into estradiol (Naftolin et al., 2001; Trainor et al., 2006; El-Emam Dief et al., 2013). The PVN and SON contain high levels of aromatase (El-Emam Dief et al., 2013), which could further explain differences in PVN and SON OT-ir between HC and LC offspring. In the California mouse male and female offspring retrieve their offspring at similar levels as they received during development (Bester-Meredith and Marler, 2003; Becker, unpublished; Leithead, unpublished), however mechanisms for this behavioral transmission are not fully elucidated. Since OT receptor expression is related to level of postnatal care (Francis et al., 2002; Perkeybile et al., 2015), our results suggest a potential mechanism by which parental care is transmitted across generations.
Since sex differences in OT are reported (Lee et al., 2009; Carter, 2014), we tested for paternal effects on OT-ir within each sex even though no overall sex differences were observed. Our analyses revealed HC males and females had more OT-ir within the PVN than LC offspring, suggesting OT-ir is plastic in response to the environment, potentially allowing more adapted social behaviors within HC offspring. However, within the SON, only males were susceptible to variability in care, which may be due to sex differences in social behaviors and physiological functions that SON OT-ir regulates. In females and males SON OT is associated with parenting and other social behaviors (Song et al., 2010; Bales et al., 2011); with SON OT also facilitating uterine contraction and lactation in females (Higashida et al., 2013). While future maternal care may be susceptible to postnatal rearing conditions, other functions like uterine contractions and lactation may be resistant to environmental fluctuations in OT, thus resulting in HC and LC females having similar expression within the SON. Alternatively, this null result could have been confounded by estrous, since SON OT-ir fluctuates in relation to circulating estrogen levels (Shughrue et al., 2002) and we did not track estrous cycle.
Since paternal retrievals induce transient increases in T (Becker et al., 2010; Chary et al., 2015) and postnatal surges in T, may be related to adult T levels (Sachser and Proöve, 1988; Lürzel et al., 2010), we wanted to assess long-term effects of rearing condition on adult basal T levels. Not surprisingly, we found males had higher basal T levels than females. Within females, we observed greater T levels in HC than LC offspring, which is likely a long-term effect of rearing condition. Consistent with Wang et al. (2015) prairie vole study, we found no differences in male T. It is possible that postnatal paternal interactions may not have long term effects on male T, or since males already have high T, that a ceiling effect (Evans et al., 2000) may obscure a potential impact. Seeing as OT expression is associated with a buffering effect on HPA function (Neumann et al., 2000; Leuner et al., 2012) and T can have organizational effects on the HPA axis (Seale et al., 2005; Goel and Bale, 2008), we examined adult basal Cort levels as a manipulation check since parental interactions may influence HPA function (Slotten et al., 2006; Engert et al., 2011). Consistent with paternal deprivation studies and our previous work (Becker et al., 2010; Wang et al., 2012; Chary et al., 2015; Yohn et al., 2017) basal Cort levels were similar between HC and LC offspring, suggesting our manipulation had no lasting effects on Cort. To further test the impact of our manipulation on stress responsivity, future studies could measure Cort levels in adult mice after experiencing a stressful situation.
Our results demonstrate developmental plasticity within the OT system in response to the postnatal paternal environment which may be mediated by transient changes in T subsequent to paternal retrievals during development. Our study is the first to illustrate long-term effects of paternal care on basal T levels in females, which may mediate transmission of social behaviors, like parenting and aggression in territorial species. Future studies are needed to examine the relationship between postnatal increases in T in response to paternal retrievals and adult OT expression to delineate whether the interplay between postnatal T and adult OT mediate changes in social behavior. Our results emphasize the critical role fathers hold in the development of the neuroendocrine system in both males and females.
Author Contributions
CY designed, conducted the behavioral manipulations, collected both the tissue and plasma used in this study. Additionally, CY ran all the statistical analyses and wrote the manuscript. AL assisted in behavioral manipulations, tissue collection and in conducting the Elisa’s. She also helped in the manuscript preparation by giving thoughtful feedback/input on each draft. JF contributed to this project through conducting the immunohistochemistry and imaging all stained sections. AG assisted with this project by scoring behavioral videos collected during each of the 7 days of experimental manipulation. He also assisted with the Elisa’s. EB was the principal investigator of this project and gave guidance/assistance on all phases of this project. Additionally, she assisted in the manuscript preparation.
Funding
This research was supported by a Psi Chi Grant to EB and a Sigma Xi Grant to CY.
Conflict of Interest Statement
The authors declare that the research was conducted in the absence of any commercial or financial relationships that could be construed as a potential conflict of interest.
Acknowledgments
We thank Joey Collins, Kieran Slattery, Jamie Palmer, Seattle Heiman and Linda Muller for their assistance.
Abbreviations
AVP, arginine vasopressin; BNST, bed nucleus of the stria terminalis; CORT, corticosterone; HC, highcare; HGL, huddling, licking and grooming behavior; HPA, hypothalamic-pituitary-adrenal; -ir, immunoreactive; LC, lowcare; NGS, normal goat serum; OT, oxytocin; PBS, phosphate buffer saline; PVN, paraventricular nucleus; SON, supraoptic nucleus; T, testosterone.
Supplementary Material
The Supplementary Material for this article can be found online at: https://www.frontiersin.org/articles/10.3389/fnbeh.2018.00181/full#supplementary-material
References
Bale, T. L. (2006). Stress sensitivity and the development of affective disorders. Horm. Behav. 50, 529–533. doi: 10.1016/j.yhbeh.2006.06.033
Bales, K. L., Boone, E., Epperson, P., Hoffman, G., and Carter, C. S. (2011). Are behavioral effects of early experience mediated by oxytocin? Front. Psychiatry 2:24. doi: 10.3389/fpsyt.2011.00024
Bales, K. L., van Westerhuyzen, J. A., Lewis-Reese, A. D., Grotte, N. D., Lanter, J. A., and Carter, C. S. (2007). Oxytocin has dose-dependent developmental effects on pair-bonding and alloparental care in female prairie voles. Horm. Behav. 52, 274–279. doi: 10.1016/j.yhbeh.2007.05.004
Bambico, F. R., Lacoste, B., Hattan, P. R., and Gobbi, G. (2015). Father absence in the monogamous California mouse impairs social behavior and modifies dopamine and glutamate synapses in the medial prefrontal cortex. Cereb. Cortex 25, 1163–1175. doi: 10.1093/cercor/bht310
Becker, E. A., Moore, B. M., Auger, C., and Marler, C. A. (2010). Paternal behavior increases testosterone levels in offspring of the California mouse. Horm. Behav. 58, 385–389. doi: 10.1016/j.yhbeh.2010.03.019
Bester-Meredith, J. K., and Marler, C. A. (2001). Vasopressin and aggression in cross-fostered California mice (Peromyscus californicus) and white-footed mice (Peromyscus leucopus). Horm. Behav. 40, 51–64. doi: 10.1006/hbeh.2001.1666
Bester-Meredith, J. K., and Marler, C. A. (2003). Vasopressin and the transmission of paternal behavior across generations in mated, cross-fostered Peromyscus mice. Behav. Neurosci. 117, 455–463. doi: 10.1037/0735-7044.117.3.455
Bester-Meredith, J. K., Young, L. J., and Marler, C. A. (1999). Species differences in paternal behavior and aggression in Peromyscus and their associations with vasopressin immunoreactivity and receptors. Horm. Behav. 36, 25–38. doi: 10.1006/hbeh.1999.1522
Bosch, O. J. (2013). Maternal aggression in rodents: brain oxytocin and vasopressin mediate pup defence. Philos. Trans. R. Soc. Lond. B Biol. Sci. 368:20130085. doi: 10.1098/rstb.2013.0085
Campi, K. L., Jameson, C. E., and Trainor, B. C. (2013). Sexual dimorphism in the brain of the monogamous California mouse (Peromyscus californicus). Brain behav. evol. 81, 236–249. doi: 10.1159/000353260
Cao, Y., Wu, R., Tai, F., Zhang, X., Yu, P., An, X., et al. (2014). Neonatal paternal deprivation impairs social recognition and alters levels of oxytocin and estrogen receptor α mRNA expression in the MeA and NAcc and serum oxytocin in mandarin voles. Horm. Behav. 65, 57–65. doi: 10.1016/j.yhbeh.2013.11.005
Carini, L. M., and Nephew, B. C. (2013). Effects of early life social stress on endocrinology, maternal behavior and lactation in rats. Horm. Behav. 64, 634–641. doi: 10.1016/j.yhbeh.2013.08.011
Carter, C. S. (2014). Oxytocin pathways and the evolution of human behavior. Annu. Rev. Psychol. 65, 17–39. doi: 10.1146/annurev-psych-010213-115110
Champagne, F. A., Chretien, P., Stevenson, C. W., Zhang, T. Y., Gratton, A., and Meaney, M. J. (2004). Variations in nucleus accumbens dopamine associated with individual differences in maternal behavior in the rat. J. Neurosci. 24, 4113–4123. doi: 10.1523/JNEUROSCI.5322-03.2004
Champagne, F. A., Diorio, J., Sharma, S., and Meaney, M. J. (2001). Naturally occurring variations in maternal behavior in the rat are associated with differences in estrogen-inducible central oxytocin receptors. Proc. Natl. Acad. Sci. U S A 98, 12736–12741. doi: 10.1073/pnas.221224598
Chary, M. C., Cruz, J. P., Bardi, M., and Becker, E. A. (2015). Paternal retrievals increase testosterone levels in both male and female California mouse (Peromyscus californicus) offspring. Horm. Behav. 73, 23–29. doi: 10.1016/j.yhbeh.2015.05.013
Clinton, S., Miller, S., Watson, S. J., and Akil, H. (2008). Prenatal stress does not alter innate novelty-seeking behavioral traits, but differentially affects individual differences in neuroendocrine stress responsivity. Psychoneuroendocrinology 33, 162–177. doi: 10.1016/j.psyneuen.2007.10.012
Consiglio, A. R., Borsoi, A., Pereira, G. A., and Lucion, A. B. (2005). Effects of oxytocin microinjected into the central amygdaloid nucleus and bed nucleus of stria terminalis on maternal aggressive behavior in rats. Physiol. Behav. 85, 354–362. doi: 10.1016/j.physbeh.2005.05.002
Dabrowska, J., Hazra, R., Ahern, T. H., Guo, J. D., McDonald, A. J., Mascagni, F., et al. (2011). Neuroanatomical evidence for reciprocal regulation of the corticotrophin-releasing factor and oxytocin systems in the hypothalamus and the bed nucleus of the stria terminalis of the rat: implications for balancing stress and affect. Psychoneuroendocrinology 36, 1312–1326. doi: 10.1016/j.psyneuen.2011.03.003
El-Emam Dief, A., Caldwell, J. D., and Jirikowski, G. F. (2013). Colocalization of P450 aromatase and oxytocin immunostaining in the rat hypothalamus. Horm. Metab. Res. 45, 273–276. doi: 10.1055/s-0032-1327680
Engelmann, M., Ebner, K., Landgraf, R., and Wotjak, C. T. (2006). Effects of Morris water maze testing on the neuroendocrine stress response and intrahypothalamic release of vasopressin and oxytocin in the rat. Horm. Behav. 50, 496–501. doi: 10.1016/j.yhbeh.2006.04.009
Engert, V., Efanov, S. I., Dedovic, K., Dagher, A., and Pruessner, J. C. (2011). Increased cortisol awakening response and afternoon/evening cortisol output in healthy young adults with low early life parental care. Psychopharmacology 214, 261–268. doi: 10.1007/s00213-010-1918-4
Evans, M. R., Goldsmith, A. R., and Norris, S. R. (2000). The effects of testosterone on antibody production and plumage coloration in male house sparrows (Passer domesticus). Behav. Ecol. Sociobiol. 47, 156–163. doi: 10.1007/s002650050006
Francis, D. D., Young, L. J., Meaney, M. J., and Insel, T. R. (2002). Naturally occurring differences in maternal care are associated with the expression of oxytocin and vasopressin (V1a) receptors: gender differences. J. Neuroendocrinol. 14, 349–353. doi: 10.1046/j.0007-1331.2002.00776.x
Frazier, C. R., Trainor, B. C., Cravens, C. J., Whitney, T. K., and Marler, C. A. (2006). Paternal behavior influences development of aggression and vasopressin expression in male California mouse offspring. Horm. Behav. 50, 699–707. doi: 10.1016/j.yhbeh.2006.06.035
Gleason, E. D., and Marler, C. A. (2013). Non-genomic transmission of paternal behaviour between fathers and sons in the monogamous and biparental California mouse. Proc. Biol. Sci. 280:20130824. doi: 10.1098/rspb.2013.0824
Goel, N., and Bale, T. L. (2008). Organizational and activational effects of testosterone on masculinization of female physiological and behavioral stress responses. Endocrinology 149, 6399–6405. doi: 10.1210/en.2008-0433
Gordon, I., Martin, C., Feldman, R., and Leckman, J. F. (2011). Oxytocin and social motivation. Dev. Cogn. Neurosci. 1, 471–493. doi: 10.1016/j.dcn.2011.07.007
Gordon, I., Pratt, M., Bergunde, K., Zagoory-Sharon, O., and Feldman, R. (2017). Testosterone, oxytocin, and the development of human parental care. Horm. Behav. 93, 184–192. doi: 10.1016/j.yhbeh.2017.05.016
Gubernick, D. J., and Alberts, J. R. (1987). The biparental care system of the California mouse, Peromyscus californicus. J. Comp. Psychol. 101, 169–177. doi: 10.1037/0735-7036.101.2.169
Higashida, H., Lopatina, O., and Sarwat, A. (2013). “Regulation of oxytocin and vasopressin secretion,” in Oxytocin, Vasopressin, and Related Peptides in the Regulation of Behavior, eds E. Choleris, D. W. Pfaff and M. Kavaliers (Cambridge: Cambridge University Press), 44–55.
Hume, J. M., and Wynne-Edwards, K. E. (2005). Castration reduces male testosterone, estradiol, and territorial aggression, but not paternal behavior in biparental dwarf hamsters (Phodopus campbelli). Horm. Behav. 48, 303–310. doi: 10.1016/j.yhbeh.2005.04.001
Ichise, M., Vines, D. C., Gura, T., Anderson, G. M., Suomi, S. J., Higley, J. D., et al. (2006). Effects of early life stress on [11C]DASB positron emission tomography imaging of serotonin transporters in adolescent peer- and mother-reared rhesus monkeys. J. Neurosci. 26, 4638–4643. doi: 10.1523/JNEUROSCI.5199-05.2006
Jia, R., Tai, F., An, S., Zhang, X., and Broders, H. (2009). Effects of neonatal paternal deprivation or early deprivation on anxiety and social behaviors of the adults in mandarin voles. Behav. Processes 82, 271–278. doi: 10.1016/j.beproc.2009.07.006
Johnson, A. E., Coirini, H., Insel, T. R., and McEwen, B. S. (1991). The regulation of oxytocin receptor binding in the ventromedial hypothalamic nucleus by testosterone and its metabolites. Endocrinology 128, 891–896. doi: 10.1210/endo-128-2-891
Kenkel, W. M., Suboc, G., and Carter, C. S. (2014). Autonomic, behavioral and neuroendocrine correlates of paternal behavior in male prairie voles. Physiol. Behav. 128, 252–259. doi: 10.1016/j.physbeh.2014.02.006
Kim, J. G., Jung, H. S., Kim, K. J., Min, S. S., and Yoon, B. J. (2013). Basal blood corticosterone level is correlated with susceptibility to chronic restraint stress in mice. Neurosci. Lett. 555, 137–142. doi: 10.1016/j.neulet.2013.09.031
Lajud, N., Roque, A., Cajero, M., Gutiérrez-Ospina, G., and Torner, L. (2012). Periodic maternal separation decreases hippocampal neurogenesis without affecting basal corticosterone during the stress hyporesponsive period, but alters HPA axis and coping behavior in adulthood. Psychoneuroendocrinology 37, 410–420. doi: 10.1016/j.psyneuen.2011.07.011
Lee, H. J., Macbeth, A. H., Pagani, J. H., and Young, W. S. III. (2009). Oxytocin: the great facilitator of life. Prog. Neurobiol. 88, 127–151. doi: 10.1016/j.pneurobio.2009.04.001
Leuner, B., Caponiti, J. M., and Gould, E. (2012). Oxytocin stimulates adult neurogenesis even under conditions of stress and elevated glucocorticoids. Hippocampus 22, 861–868. doi: 10.1002/hipo.20947
Lürzel, S., Kaiser, S., and Sachser, N. (2010). Social interaction, testosterone, and stress responsiveness during adolescence. Physiol. Behav. 99, 40–46. doi: 10.1016/j.physbeh.2009.10.005
McEwen, B. S. (2007). Physiology and neurobiology of stress and adaptation: central role of the brain. Physiol. Rev. 87, 873–904. doi: 10.1152/physrev.00041.2006
Naftolin, F., Horvath, T. L., and Balthazart, J. (2001). Estrogen synthetase (aromatase) immunohistochemistry reveals concordance between avian and rodent limbic systems and hypothalami. Exp. Biol. Med. Maywood 226, 717–725. doi: 10.1177/153537020222600802
Neumann, I. D., and van den Burg, E. (2013). “Oxytocin and vasopressin release and their receptor-mediated intracellular pathways that determine their behavioral effects,” in Oxytocin, Vasopressin, and Related Peptides in the Regulation of Behavior, eds E. Choleris, D. Pfaff, and M. Kavaliers (Cambridge: Cambridge University Press), 27–43.
Neumann, I. D., Wigger, A., Torner, L., Holsboer, F., and Landgraf, R. (2000). Brain oxytocin inhibits basal and stress-induced activity of the hypothalamo-pituitary-adrenal axis in male and female rats: partial action within the paraventricular nucleus. J. Neuroendocrinol. 12, 235–243. doi: 10.1046/j.1365-2826.2000.00442.x
Okabe, S., Kitano, K., Nagasawa, M., Mogi, K., and Kikusui, T. (2013). Testosterone inhibits facilitating effects of parenting experience on parental behavior and the oxytocin neural system in mice. Physiol. Behav. 118, 159–164. doi: 10.1016/j.physbeh.2013.05.017
Oomen, C. A., Girardi, C. E., Cahyadi, R., Verbeek, E. C., Krugers, H., Joels, M., et al. (2009). Opposite effects of early maternal deprivation on neurogenesis in male versus female rats. PLoS One 4:e3675. doi: 10.1371/journal.pone.0003675
Paxinos, G., Koutcherov, Y., Halliday, G. M., and Watson, C., Wang, H. (2007). Atlas of the Developing Mouse Brain: at E17.5, P0, and P6. Amsterdam: Academic Press.
Pedersen, C. A. (1997). Oxytocin control of maternal behavior. Ann. N Y Acad. Sci. 807, 126–145. doi: 10.1111/j.1749-6632.1997.tb51916.x
Pedersen, C. A., and Boccia, M. L. (2003). Oxytocin antagonism alters rat dams’ oral grooming and upright posturing over pups. Physiol. Behav. 80, 233–241. doi: 10.1016/j.physbeh.2003.07.011
Perkeybile, A. M., Delaney-Busch, N., Hartman, S., Grimm, K. J., and Bales, K. L. (2015). Intergenerational transmission of alloparental behavior and oxytocin and vasopressin receptor distribution in the prairie vole. Front. Behav. Neurosci. 9:191. doi: 10.3389/fnbeh.2015.00191
Rault, J. L., Carter, C. S., Garner, J. P., Marchant-Forde, J. N., Richert, B. T., and Lay, D. C. Jr. (2013). Repeated intranasal oxytocin administration in early life dysregulates the HPA axis and alters social behavior. Physiol. Behav. 112–113, 40–48. doi: 10.1016/j.physbeh.2013.02.007
Roberts, M. L., Buchanan, K. L., Hasselquist, D., and Evans, M. R. (2007). Effects of testosterone and corticosterone on immunocompetence in the zebra finch. Horm. Behav. 51, 126–134. doi: 10.1016/j.yhbeh.2006.09.004
Rosenfeld, C. S., Johnson, S. A., Ellersieck, M. R., and Roberts, R. M. (2013). Interactions between parents and parents and pups in the monogamous California mouse (Peromyscus californicus). PLoS One 8:e75725. doi: 10.1371/journal.pone.0075725
Sachser, N., and Proöve, E. (1988). Plasma-testosterone development in colony and individually housed male guinea pigs. Ethology 79, 62–70. doi: 10.1111/j.1439-0310.1988.tb00699.x
Seale, J. V., Wood, S. A., Atkinson, H. C., Lightman, S. L., and Harbuz, M. S. (2005). Organizational role for testosterone and estrogen on adult hypothalamic-pituitary-adrenal axis activity in the male rat. Endocrinology 146, 1973–1982. doi: 10.1210/en.2004-1201
Shannon, C., Schwandt, M. L., Champoux, M., Shoaf, S. E., Suomi, S. J., Linnoila, M., et al. (2005). Maternal absence and stability of individual differences in CSF 5-HIAA concentrations in rhesus monkey infants. Am. J. Psychiatry 162, 1658–1664. doi: 10.1176/appi.ajp.162.9.1658
Shughrue, P. J., Dellovade, T. L., and Merchenthaler, I. (2002). Estrogen modulates oxytocin gene expression in regions of the rat supraoptic and paraventricular nuclei that contain estrogen receptor-β. Prog. Brain Res. 139, 15–29. doi: 10.1016/s0079-6123(02)39004-6
Sladek, C. D., Swenson, K. L., Kapoor, R., and Sidorowicz, H. E. (2000). The role of steroid hormones in the regulation of vasopressin and oxytocin release and mRNA expression in hypothalamo-neurohypophysial explants from the rat. Exp. Physiol. 85, 171S–177S. doi: 10.1111/j.1469-445x.2000.tb00021.x
Slotten, H. A., Kalinichev, M., Hagan, J. J., Marsden, C. A., and Fone, K. C. (2006). Long-lasting changes in behavioural and neuroendocrine indices in the rat following neonatal maternal separation: gender-dependent effects. Brain Res. 1097, 123–132. doi: 10.1016/j.brainres.2006.04.066
Song, Z., Tai, F., Yu, C., Wu, R., Zhang, X., Broders, H., et al. (2010). Sexual or paternal experiences alter alloparental behavior and the central expression of ERα and OT in male mandarin voles (Microtus mandarinus). Behav. Brain Res. 214, 290–300. doi: 10.1016/j.bbr.2010.05.045
Trainor, B. C., Kyomen, H. H., and Marler, C. A. (2006). Estrogenic encounters: how interactions between aromatase and the environment modulate aggression. Front. Neuroendocrinol. 27, 170–179. doi: 10.1016/j.yfrne.2005.11.001
Trainor, B. C., and Marler, C. A. (2001). Testosterone, paternal behavior, and aggression in the monogamous California mouse (Peromyscus californicus). Horm. Behav. 40, 32–42. doi: 10.1006/hbeh.2001.1652
Trainor, B. C., and Marler, C. A. (2002). Testosterone promotes paternal behaviour in a monogamous mammal via conversion to oestrogen. Proc. Biol. Sci. 269, 823–829. doi: 10.1098/rspb.2001.1954
Trainor, B. C., Takahashi, E. Y., Silva, A. L., Crean, K. K., and Hostetler, C. (2010). Sex differences in hormonal responses to social conflict in the monogamous California mouse. Horm. Behav. 58, 506–512. doi: 10.1016/j.yhbeh.2010.04.008
Uriarte, N., Breigeiron, M. K., Benetti, F., Rosa, X. F., and Lucion, A. B. (2007). Effects of maternal care on the development, emotionality, and reproductive functions in male and female rats. Dev. Psychobiol. 49, 451–462. doi: 10.1002/dev.20241
Wang, B., Li, Y., Wu, R., Zhang, S., and Tai, F. (2015). Behavioral responses to pups in males with different reproductive experiences are associated with changes in central OT, TH and OTR, D1R, D2R mRNA expression in mandarin voles. Horm. Behav. 67, 73–82. doi: 10.1016/j.yhbeh.2014.11.013
Wang, J., Tai, F., Yan, X., and Yu, P. (2012). Paternal deprivation alters play-fighting, serum corticosterone and the expression of hypothalamic vasopressin and oxytocin in juvenile male mandarin voles. J. Comp. Physiol. A Neuroethol. Sens. Neural Behav. Physiol. 198, 787–796. doi: 10.1007/s00359-012-0748-8
Weaver, I. C., Meaney, M. J., and Szyf, M. (2006). Maternal care effects on the hippocampal transcriptome and anxiety-mediated behaviors in the offspring that are reversible in adulthood. Proc. Natl. Acad. Sci. U S A 103, 3480–3485. doi: 10.1073/pnas.0507526103
Yee, J. R., Kenkel, W. M., Frijling, J. L., Dodhia, S., Onishi, K. G., Tovar, S., et al. (2016). Oxytocin promotes functional coupling between paraventricular nucleus and both sympathetic and parasympathetic cardioregulatory nuclei. Horm. Behav. 80, 82–91. doi: 10.1016/j.yhbeh.2016.01.010
Yohn, C. N., Leithead, A. B., and Becker, E. A. (2017). Increased vasopressin expression in the BNST accompanies paternally induced territoriality in male and female California mouse offspring. Horm. Behav. 93, 9–17. doi: 10.1016/j.yhbeh.2017.03.008
Yu, P., An, S., Tai, F., Zhang, X., He, F., Wang, J., et al. (2012). The effects of neonatal paternal deprivation on pair bonding, NAcc dopamine receptor mRNA expression and serum corticosterone in mandarin voles. Horm. Behav. 61, 669–677. doi: 10.1016/j.yhbeh.2012.02.028
Ziegler, T. E., Wegner, F. H., Carlson, A. A., Lazaro-Perea, C., and Snowdon, C. T. (2000). Prolactin levels during the periparturitional period in the biparental cotton-top tamarin (Saguinus oedipus): interactions with gender androgen levels, and parenting. Horm. Behav. 38, 111–122. doi: 10.1006/hbeh.2000.1606
Keywords: oxytocin, testosterone, corticosterone, paternal care, Peromyscus californicus
Citation: Yohn CN, Leithead AB, Ford J, Gill A and Becker EA (2018) Paternal Care Impacts Oxytocin Expression in California Mouse Offspring and Basal Testosterone in Female, but Not Male Pups. Front. Behav. Neurosci. 12:178. doi: 10.3389/fnbeh.2018.00181
Received: 31 May 2018; Accepted: 02 August 2018;
Published: 29 August 2018.
Edited by:
Erica R. Glasper, University of Maryland, College Park, United StatesReviewed by:
Brian Trainor, University of California, Davis, United StatesKaren Lisa Bales, University of California, Davis, United States
Copyright © 2018 Yohn, Leithead, Ford, Gill and Becker. This is an open-access article distributed under the terms of the Creative Commons Attribution License (CC BY). The use, distribution or reproduction in other forums is permitted, provided the original author(s) and the copyright owner(s) are credited and that the original publication in this journal is cited, in accordance with accepted academic practice. No use, distribution or reproduction is permitted which does not comply with these terms.
*Correspondence: Christine N. Yohn, Y3kyNTNAc2NhcmxldG1haWwucnV0Z2Vycy5lZHU=