- 1Department of General Biochemistry, Faculty of Biology and Environmental Protection, University of Lodz, Łódź, Poland
- 2Department of Medical Biochemistry, Medical University of Lodz, Łódź, Poland
- 3Department of Physical Medicine, Medical University of Lodz, Łódź, Poland
- 4Neurorehabilitation Ward, III General Hospital in Lodz, Łódź, Poland
- 5Laboratory of Medical Genetics, Department of Molecular Genetics, Faculty of Biology and Environmental Protection, University of Lodz, Łódź, Poland
Background: Neuroplasticity ensures the improvement of functional status in patients after stroke. The aim of this study was to evaluate the effect of extremely low-frequency electromagnetic field therapy (ELF-EMF) on brain plasticity in the rehabilitation of patients after stroke.
Methods: Forty-eight patients were divided into two groups underwent the same rehabilitation program, but in the study group, the patients additionally were exposed to a standard series of 10 ELF-EMF treatments. To determine the level of neuroplasticity, we measured the plasma level of the brain-derived neurotrophic factor (BDNF), the vascular-endothelial growth factor, as well as BDNF mRNA expression. Additionally, we determined the molecule levels for hepatocyte growth factor, stem cell factor, stromal cell-derived factor 1α, nerve growth factor β, and leukemia inhibitory factor, using 5plex cytokine panel in plasma. After 4 weeks, during which patients had undergone neurorehabilitation and neurological examinations, we assessed functional recovery using the Barthel Index, Mini-Mental State Examination (MMSE), Geriatric Depression Scale, National Institutes of Health Stroke Scale (NIHSS), and the modified Rankin Scale (mRS).
Results: We observed that ELF-EMF significantly increased growth factors and cytokine levels involved in neuroplasticity, as well as promoted an enhancement of functional recovery in post-stroke patients. Additionally, we presented evidence that these effects could be related to the increase of gene expression on the mRNA level. Moreover, a change of BDNF plasma level was positively correlated with the Barthel Index, MMSE, and negatively correlated with GDS.
Conclusion: Extremely low-frequency electromagnetic field therapy improves the effectiveness of rehabilitation of post-stroke patients by improving neuroplasticity processes.
Introduction
Regenerative processes within the brain tissue are limited and regulated by tissue environmental properties, which are affected by changes in the physiology of the organism (Hagg, 2009). Neurotrophic factors affect neurogenesis through the condition of the growth of new neurons and the survival of existing ones (Hylin et al., 2017). Traditionally, neurotrophic factors are divided into three protein families: the classic neurotrophin, ligands of Glial Cell Derived Neurotrophic Factor (GDNF), and neuropoietic cytokines. Neurotrophins are synthesized and secreted by nerve cells in the brain and spinal cord, and the cells of dependent tissue (Hagg, 2009; Lindholm and Saarma, 2010).
Compensatory plasticity of the damaged brain is a completely different process from the plasticity occurring in a normally functioning, healthy brain. This process is initiated under critical conditions: in interactions with oedema, inflammation, apoptosis, metabolic disturbances, and fiber degeneration. It starts immediately after an ischaemic event (Liguz-Lecznar and Kossut, 2013). Moreover, neuroplasticity consists of strengthening the existing nerve pathways and then establishing new connections. Existing, but weaker connections between brain centers undergo activation (Martin et al., 2017). As a result, the defective function can be restored partially or completely, because other cortical or subcortical structures will take over the function of the damaged area. In the brain in animal models, synaptogenesis was found in the area adjacent to tissue damaged by the stroke, and also in regions of the undammed hemisphere (Law et al., 2017).
Neuroplasticity is closely related to neurogenesis, wherein fully functioning neuronal cells are generated, resulting from the differentiation of neuronal stem cells (NSCs) present in the adult, fully formed brain. NSCs are characterized by the ability of indefinite mitotic division, potential divisions, and to differentiate into the appropriate morphological phenotype (Klein et al., 2016). The process of neurogenesis occurs in certain brain structures throughout life, nevertheless the rate of proliferation and the ability of the newly formed neurons to survive to reduce with age. Nerve cells are generated in brain regions responsible for learning, memory, and reception of olfactory sensation, primarily in the sub-ventricular zone (SVZ) and the subgranular zone (SGZ), and also in the migration of emergent neuroblasts toward injury (Zelentsova-Levytskyi et al., 2017). Neurogenesis is regulated by many factors, including neurotrophins, growth factors, hormones, neurotransmitters, and microenvironmental factors (Aimone et al., 2014).
Physical therapy, including the use of extremely low-frequency electromagnetic field (ELF-EMF) therapy, is beneficial in restoring the patients after stroke. ELF-EMF demonstrates anti-inflammatory, regenerative, analgesic, and osteogenic action. Moreover, ELF-EMF promotes cell proliferation, protein synthesis, ion transport, and changes in cellular signal transmission (Li, 2017). Our previous studies have shown that ELF-EMF therapy reduces oxidative stress during rehabilitation of post-acute stroke patients (Cichoń et al., 2017a, 2018). Additionally, our recent research, for the first time focused on the effect of ELF-EMF on the potential factors of brain plasticity, indicate that ELF-EMF therapy increases the generation and metabolism of NO – neurotransmitter regulating neurogenesis, and synaptic plasticity (Cichoń et al., 2017b). As part of the broadening the examined issue, the present analyses are a continuation of the parameter evaluation of the same group of post-stroke patients but currently relate to changes in blood levels of growth factors involved in the neuroplasticity process, induced by ELF-EMF therapy. We selected two significant growth factors for analysis, i.e., brain-derived neurotrophic factor (BDNF) and vascular endothelial growth factor (VEGF). BDNF is the most common neurotrophin in the nervous system, and playing an important role as an effective indicator for rehabilitation interventions in relation to brain neuroplasticity improvement (Qiao et al., 2017). VEGF is one of the most important pro-angiogenic factors and is critical for blood vessel growth in the nervous system of vertebrates. VEGF-induced blood vessel growth may be essential for nervous tissue regeneration during the recovery process. Moreover, several recent studies demonstrate that VEGF has significant non-vascular functions in the nervous system, and it can be considered as an important agent for promoting neurogenesis, glial growth, and nerve repair (Rosenstein et al., 2010). It is well documented that the cytokine-mediated inflammatory mechanisms within the central nervous system (CNS) contribute to cognitive impairment due to disorders of neurons and glial cells in acute stroke patients. Therefore, we have also chosen a panel of 5 cytokines (HGF, SCF, SDF-1α, β-NGF, and LIF) simultaneous measured using Bio-Plex System, which may be the important factors involved in the neurochemical features of brain tissue damage and repair.
Materials and Methods
Blood Sample Collection
Blood samples were taken twice: before and after a standard ten sessions of therapy (with an interval of 14 days). They were collected into CPDA1 containing tubes (Sarstedt, Nümbrecht, Germany). For analysis of mRNA expression, a portion of the sample was frozen at -80°C immediately upon collection. The rest of the samples were centrifuged (15 min at 1,500 g) at 25°C, to isolate the plasma. All blood samples were collected at the same time of day (between 7 am and 9 am), under conditions of dietary fasting, and stored using the same protocol.
Subject Presentation
Post-stroke patients with moderate stroke severity (n = 48) were recruited to the study, and were then randomly divided into a study group [ELF-EMF (n = 25; NIHSS scores of 4.9 ± 3.1; aged 48.0 ± 8.0)], and a control group [non-ELF-EMF (n = 23; NIHSS scores of 5.4 ± 2.9; aged 44.8 ± 7.7)]. Clinical and demographic characteristics are shown in Table 1. The same patients were enrolment to our previous study (Cichoń et al., 2017b). Subjects with neurological illness other than stroke, haemorrhagic stroke, chronic or significant acute inflammatory factors, dementia, and/or decreased consciousness in their medical pre-stroke history, were all excluded. The patients had undergone neurorehabilitation as well as internal and neurological examinations, for 4 weeks in Neurorehabilitation Ward III of the General Hospital in Łódź, Poland.
In both subject groups the same therapeutic program (aerobic exercise 30 min, neurophysiological routines 60 min, and 15 min psychological therapy) was used. Furthermore, ELF-EMF therapy was conducted using a Magnetronic MF10 generator (EiE Elektronika i Elektromedycyna, Otwock, Poland). Both groups were treated for the same amount of time (15 min), but sham exposures were administrated to the non-ELF-EMF subjects. Subjects were excluded from the ELF-EMF group who had electronic and/or metal implants (pacemakers, etc.). ELF-EMF therapy with specific parameters (magnetic induction of 5 mT, 40 Hz, rectangular and bipolar waveforms) was conducted in the ELF-EMF group. The pelvic girdle of the patients was exposed to the electromagnetic field.
Determination of BDNF Level in Plasma
Plasma samples were diluted ten times (using a diluents buffer) before measurement of BDNF concentration, using a Human BDNF ELISA Kit (Abcam, Cambridge, MA, United States), in accordance with the manufacturer’s protocol. The intensity of the color was measured at 450 nm (Schiavone et al., 2017).
Determination of BDNF Expression in Whole Blood Samples
Isolation of RNA and Reverse Transcription
Frozen whole blood samples (-80°C) were lysed using TRI Reagent® (Sigma-Aldrich, St. Louis, MO, United States), after which phase separation was performed. Then, an InviTrap Spin Universal RNA Mini Kit (Stratec Biomedical Systems, Birkenfeld, Germany) was used to purify the RNA-containing aqueous phase. The quantity and purity of RNA were estimated using a Synergy HTX Multi-Mode Microplate Reader, equipped with a Take3 Micro-Volume Plate (BioTek Instruments, Inc., Winooski, VT, United States). RNA samples were diluted to 20 ng/μL and transcribed into cDNA with a High-Capacity cDNA Reverse Transcription Kit (Applied BiosystemsTM, Waltham, MA, United States). All steps were performed according to the manufacturers’ recommendations.
Real-Time PCR
Expression levels of the studied genes were obtained using the following TaqMan probes: Hs02718934_s1 for human BDNF gene, and Hs02786624_g1 as an endogenous control, which was a human GAPDH gene (Life Technologies, Carlsbad, CA, United States). Real-time PCRs were performed in a CFX96 real-time PCR system (Bio-Rad Laboratories, Hercules, CA, United States) using a TaqMan Universal Master Mix II, without UNG (Life Technologies, Carlsbad, CA, United States). All procedures were performed according to the manufacturers’ protocols. Relative expressions of the studied genes were calculated using the equation 2-ΔCt, where ΔCt = Cttargetgene – CtGAPDH.
Determination of VEGF in Plasma
Measurement of VEGF concentration was conducted using a VEGF Human ELISA Kit (Novex® Life Technologies, Carlsbad, CA, United States), according to the manufacturer’s protocol. The intensity of the color was measured at 450 nm (Ling et al., 2015).
Analysis of Plasma Cytokine Levels
The level of HGF, SCF, SDF-1α, β-NGF, and LIF plasma growth factors were indicated using a Human Cytokine 5-plex assay kit (Bio-Rad, Hercules, CA, United States), on a Bio-Plex® 200 system (Bio-Rad, Hercules, CA, United States). Growth factors were measured in accordance with the manufacturer’s protocol (Zanotta et al., 2016).
Clinical Parameters Determination
The stroke-related neurologic deficit was measured using The National Institutes of Health Stroke Scale (NIHSS). Functional status was evaluated using the Barthel Index of Activities of Daily Living (ADL) and modified Rankin Scale (mRS), and cognitive status using the Mini-Mental State Examination (MMSE). Depression, the most common affective complication after stroke, was estimated using the Geriatric Depression Scale (GDS) (Cichoń et al., 2017a). The NIHSS, ADL, MMSE, GDS, and mRS were conducted either on the same day as the blood sampling or the afternoon before, in both groups.
Data Analysis
All experiments were performed in duplicate and calculated as mean values. For all subjects, the values of parameters before their treatments were used as the output value (100%). Data from the experiments performed on these same subjects after appropriate treatments were expressed as a percentage of the output value. Values obtained in this way were expressed as mean ± SD.
The all statistical analyses were performed using Stats Direct statistical software v.2.7.2. To avoid committing a type 1 error statistical analysis was performed using multiple comparison methods. First, the Shapiro–Wilk test was used to assess normal distribution of variables. Next, the results were analyzed for equality of variance using Levene’s test. The significance of the differences between the values was analyzed using ANOVA, followed by Tukey’s range test for multiple comparisons (for data with normal distribution and equality of variance) or non-parametric the Kruskal–Wallis test (when variables had other than normal distribution or had no equality of variance) (Bijak et al., 2012, 2013).
Additionally, we performed a correlation analysis between the changes in both experimental and clinical parameters. For these analyses, a Spearman’s rank correlation was used, with the Spearman’s rank correlation coefficient and the probability of correlation designated. For all analyses, a level of p < 0.05 was accepted as statistically significant.
Results
In our comparative analysis, we demonstrated the effect of ELF-EMF therapy on various neurotrophic factors. Particularly relevant findings relate to the level of BDNF as the most prevalent growth factor in the CNS, which is essential for the development of CNS and neuronal plasticity. The plasma level of BDNF in the ELF-EMF group after ten sessions of rehabilitation was significantly higher compared to the non-ELF-EMF group (p < 0.0001). The increase of the BDNF level in the ELF-EMF group was about 200% (p < 0.0001), while in the non-ELF-EMF group it was comparable (p > 0.05) (Figure 1A). We also evaluated the effect of ELF-EMF on gene expression in the whole blood samples of BDNF. We demonstrated that after ELF-EMF therapy, expression of BDNF increased about 195% (p < 0.0001), while in the non-ELF-EMF group it did not change (Figure 1B). Because of the crucial role of BDNF participates in the formation of appropriate synaptic connections in the brain, it seems that ELF-EMF may serve as a therapeutic treatment to improve the neuroplasticity after stroke. Moreover, as proved to be significant differences in the level of VEGF caused by ELF-EMF application. VEGF is crucial for cross-talk between the cardiovascular and nervous systems, which is particularly important in the case of brain stroke that damages both blood vessels and nervous tissue. After treatment, the VEGF plasma concentration in the ELF-EMF group increased about 50% (p < 0.001), but in the non-ELF-EMF group it remained unchanged (p > 0.05) (Figure 2). The raw data of both BDNF and VEGF plasma level has been shown in Table 2). We also assessed five cytokine concentrations in plasma using the Luminex platform. Two of these (βNGF and LIF) presented as out of range, both before and after treatment (<2.57 pg/ml and 1.92 pg/ml, respectively) (Table 3). After treatments, hepatocyte growth factor (HGF) and stem cell factor (SCF) levels in plasma were elevated in the ELF-EMF group (p < 0.01 and p < 0.05, respectively), but the SDF-1α level was comparable in both groups (p > 0.05) (Table 3 and Figure 3). HGF is another (like VEGF) angiogenic factor that also produces neurotrophic effects in CNS. HGF plays pivotal roles in the nervous system during nerve regeneration process, by acting on neuronal or non-neuronal cells. HGF concentration after ELF-EMF therapy increased about 35% (p < 0.01), and in the non-ELF-EMF group remained unchanged (p > 0.05) (Figure 3A). SCF is a cytokine belonging to the control factors of the differentiation of stem cells to neurons and glia. Given the capacity of SCF to induction of regenerate of cells lost through brain injury, this cytokine seems particularly essential for the course of neuroplasticity processes. SCF level in the ELF-EMF group was higher by about 25% after treatment (p < 0.05), and in the non-ELF-EMF group was unchanged (p > 0.05) (Figure 3B). After therapy, the change of SDF-1α concentration was low and not statistically significant in either group (Table 3 and Figure 3C).
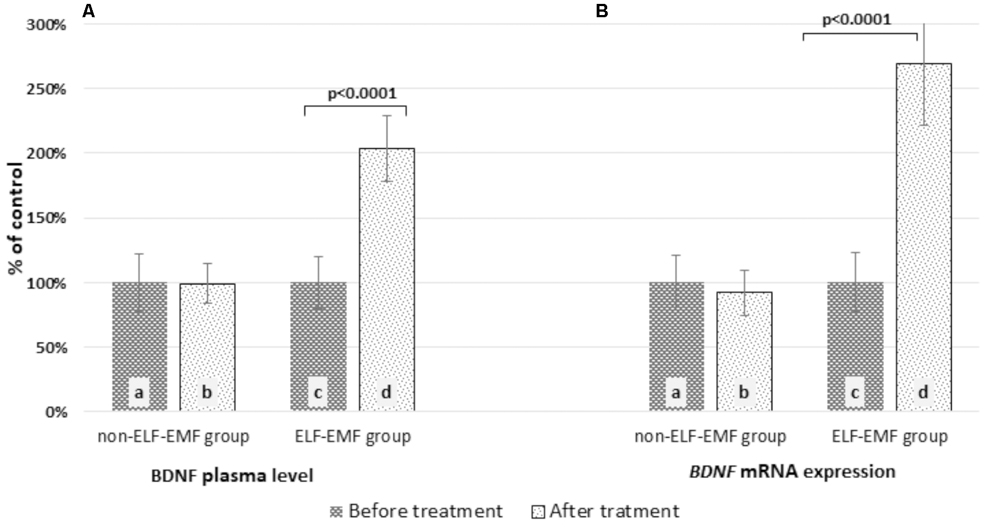
FIGURE 1. Comparison of the BDNF level obtained from the ELF-EMF group vs. the non-ELF-EMF group. (A) BDNF plasma concentration. Statistical significance between ELF-EMF and non-ELF-EMF groups: b vs. d: p < 0.0001. (B) BDNF mRNA expression. Statistical significance between ELF-EMF and non-ELF-EMF groups: b vs. d: p < 0.0001.
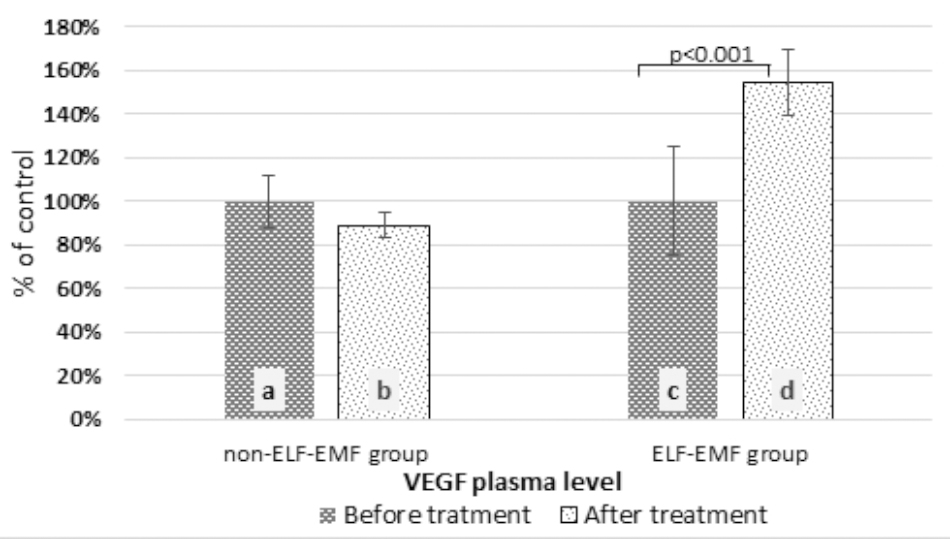
FIGURE 2. Comparison of the plasma VEGF level obtained from the ELF-EMF group vs. the non-ELF-EMF group. Statistical significance between ELF-EMF and non-ELF-EMF groups: b vs. d: p < 0.001.
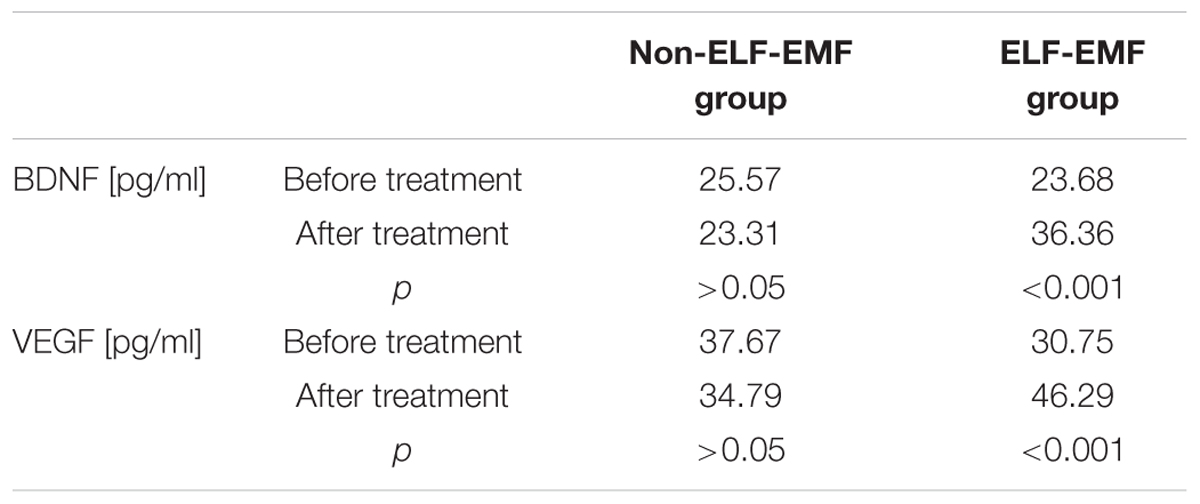
TABLE 2. Plasma levels (in pg/ml) of BDNF and VEGF measured before and after treatment in ELF-EMF and non-ELF-EMF groups.
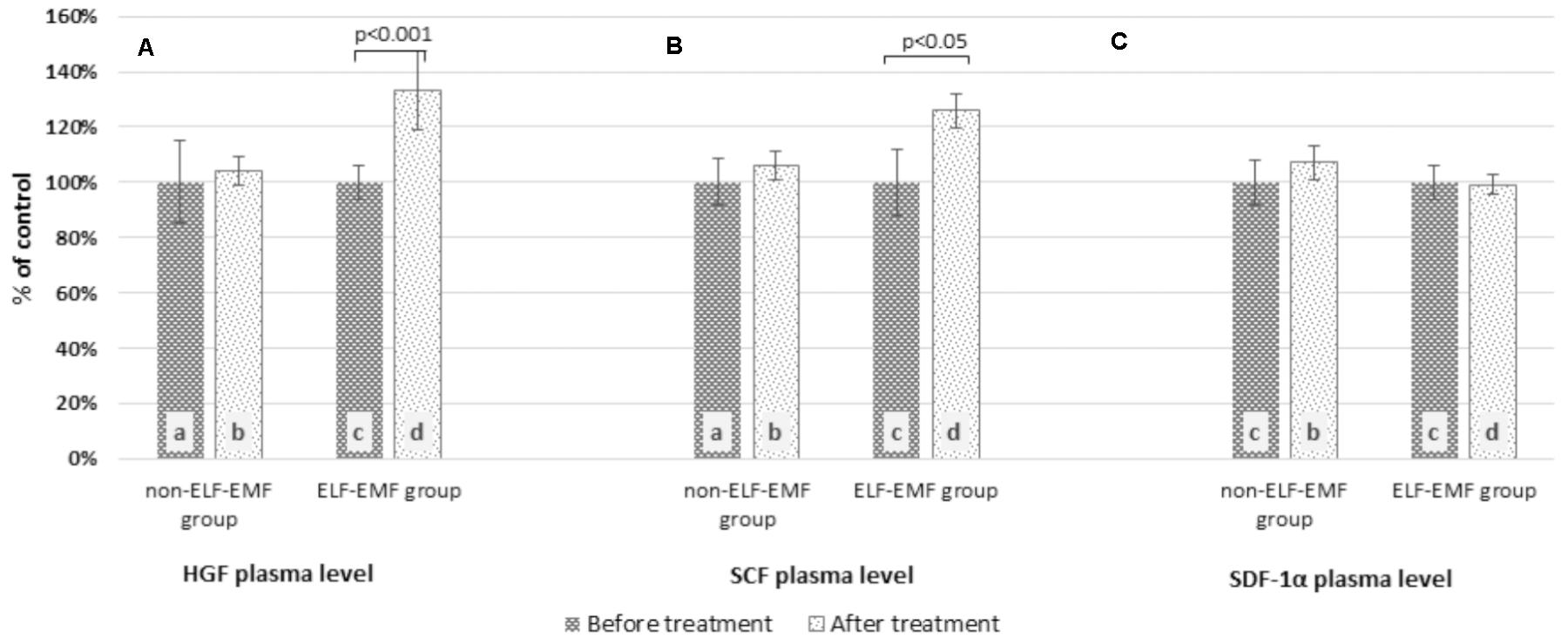
FIGURE 3. Comparison of the plasma cytokine profile obtained from the ELF-EMF group vs the non-ELF-EMF group. (A) HGF plasma level. Statistical significance between ELF-EMF and non-ELF-EMF groups: b vs. d: p < 0.01. (B) SCF plasma level. Statistical significance between ELF-EMF and non-ELF-EMF groups: b vs. d: p < 0.05. (C) SDF-1α plasma level.
Additionally, we estimated the clinical status of patients using NIHSS, ADL, mRS, MMSE, and the GDS in both groups. Stroke-related neurologic deficit estimated using NIHSS in ELF-EMF group decreased about 65% more than in non-ELF-EMF group (p < 0.001) (Figure 4). Functional status assessed by ADL in both groups increased (p < 0.001 and p < 0.001), but ΔADL in both groups was comparable (p > 0.05), whereas assessed by mRS decreased in both group, and decline in ELF-EMF group was more about 50% than in non-ELF-EMF group (p < 0.01) (Figure 4). A better improvement after ELF-EMF therapy was observed in cognitive impairment estimated by MMSE, with about a 35% higher growth (Figure 4). Depressive syndrome measured in GDS decreased significantly, while ΔGDS gained about 45% better results in the ELF-EMF group than the non-ELF-EMF group (Figure 4).
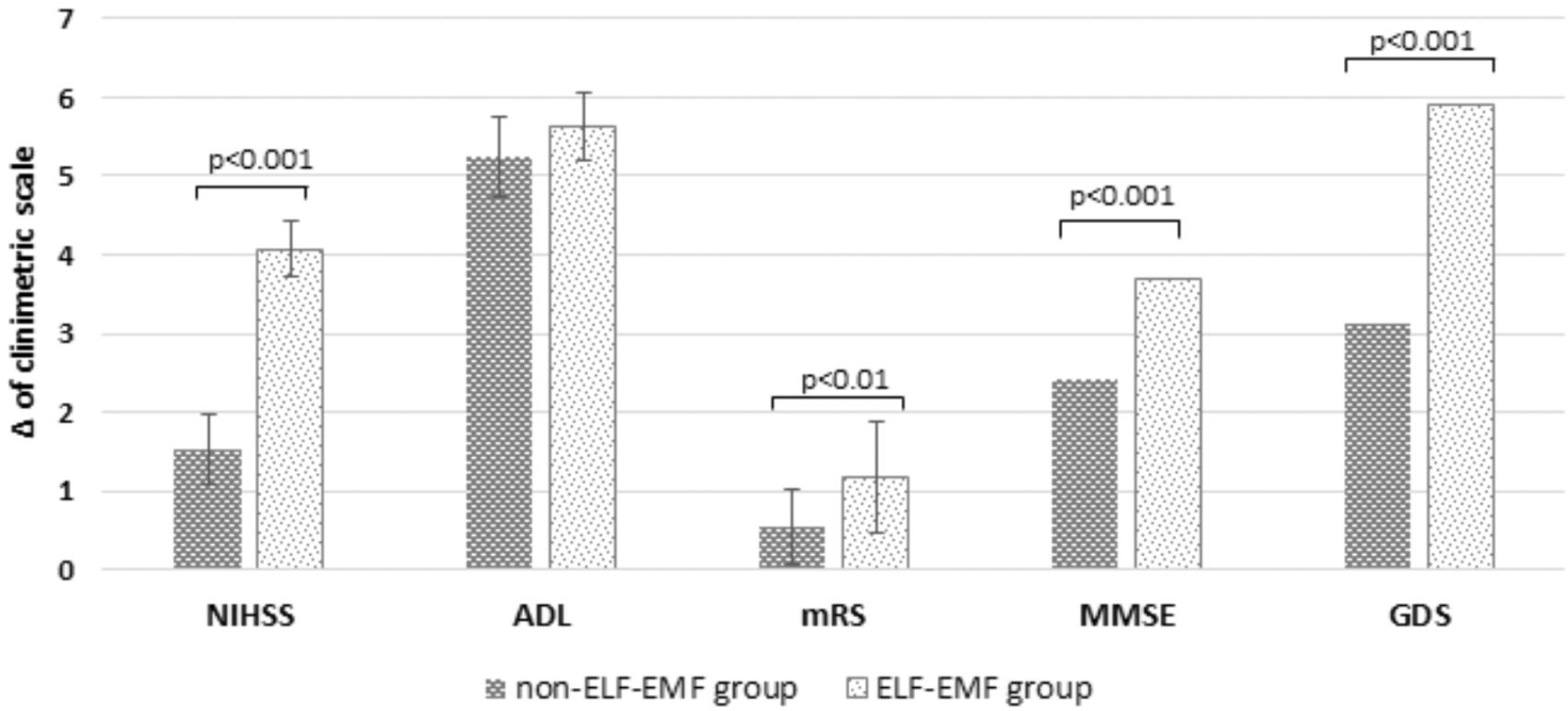
FIGURE 4. Clinical parameters NIHSS, ADL, mRS, MMSE, and GDS as measured in the study vs. the control group. Data is shown here as a delta of scores obtained before and after the standard series of treatments (Δ NIHSS = the decline of NIHSS; ΔADL = the gain of ADL; Δ mRS = the decline of mRS; Δ MMSE = the gain of MMSE; Δ GDS = the decline of GDS).
Subsequently, we performed a correlation analysis between the plasma level of the most important neurotrophic factor, BDNF, and clinical parameters. Correlation parameters indicated a significant positive correlation between changes (Δ) of BDNF plasma level and ΔADL, as well as between ΔBNDF and ΔMMSE, and a negative correlation between ΔBNDF and ΔGDS (Figure 5). The detailed process of these relationships, which confirms the correlation and the numeric data shown, is presented in Table 3.
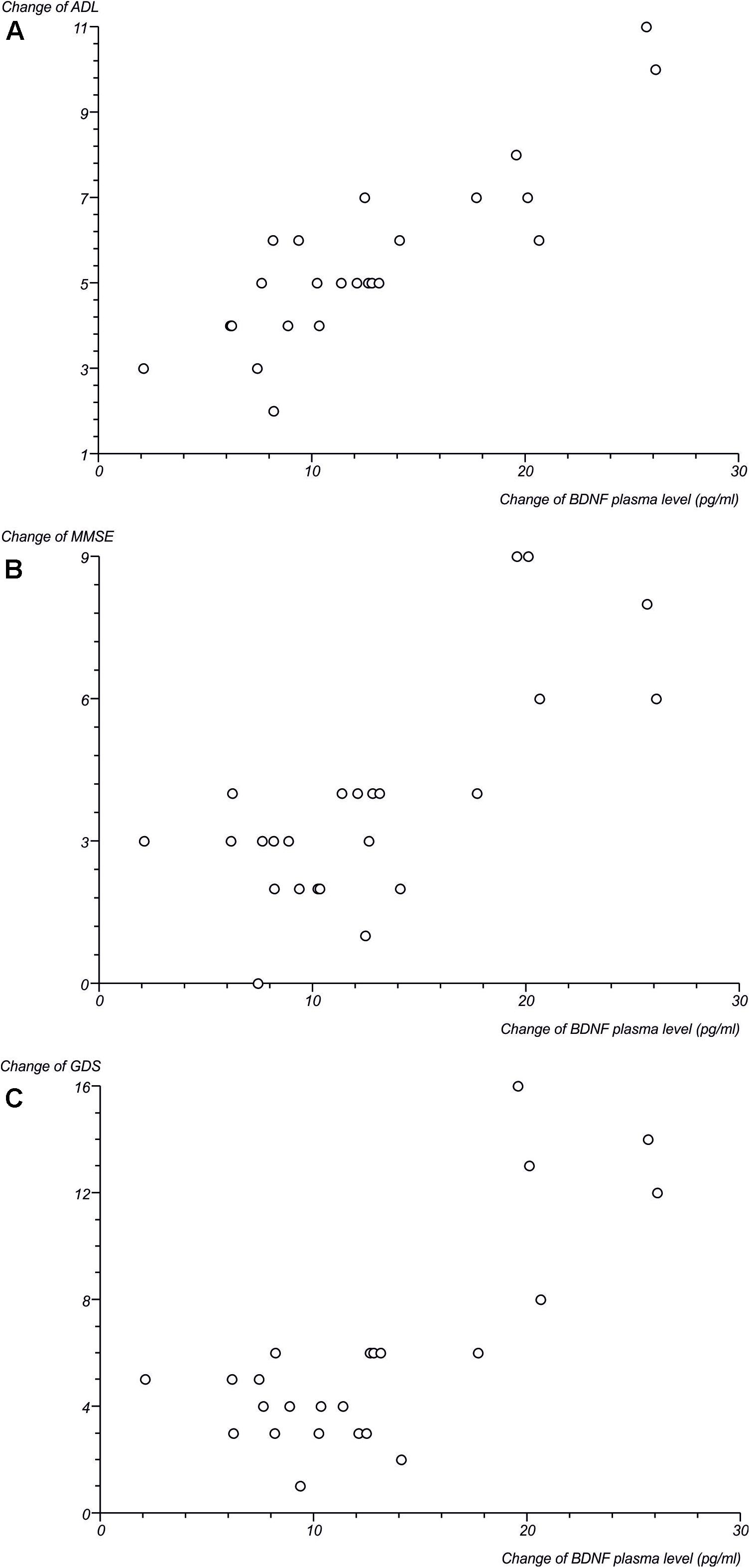
FIGURE 5. Scatterplots presenting correlation between changes of BDNF plasma level (in pg/ml) in ELF-EMF group and (A) changes of ADL; (B) changes of MMSE; (C) changes of GDS.
Discussion
Post-stroke rehabilitation is intended to restore the patient to a healthy condition and return them to a functional state, as prior to their illness, or allow them to adapt and achieve an optimal level of independence. Active post-stroke therapy should be begun as soon as possible, immediately upon stabilization of the general medical state. The rehabilitation process should be continued until obtaining a good result on an actual improvement index, which should also include cognitive disorders and behavioral changes (Jia et al., 2017).
Ischaemic stroke and other acute CNS injury indicates an increase of neuronal progenitor’s proliferation in the SVZ zone identifying the lesion area and neuroblast migration to the ischaemic area within the striatum and cortex (Hu et al., 2013; Liu et al., 2015b; Choi et al., 2017).
In current research on brain plasticity processes, particular attention has been focused on BDNF an activator of various signaling pathways involved in regulation of neurogenesis and survival of neurons. The BDNF function may also be related to the formation and maintenance of dendritic spines and dendrites, as well as regulation of synaptic function during long-term potentiation, learning, and memory process (Greenberg et al., 2009). The source of BDNF is an active microglial, as well as the endothelium and neurons (Gomes et al., 2012). BDNF is involved in the regulation of neurogenesis in the SVZ zone and the migration of progenitor cells from the SVZ to the damaged striatum (Bathina and Das, 2015). BDNF infusions into lateral ventricles cause duplication of a number of neurons in the olfactory bulb region and production of synaptic connections (Bath and Lee, 2010).
In this study, we showed for the first time that ELF-EMF increased BDNF concentration (Figure 1A), as well as BDNF mRNA expression in vivo in humans (Figure 1B). Our results coincide with those of studies conducted by Di Loreto et al. (2009). They investigated changes in the expression profile of cytokine and the growth factor profile in rat cortical neurons with exposure to ELF-EMF (50 Hz, 0.1 and 1 mT) during maturation of cells. They observed that ELF-EMF exposure induced an increased mRNA and protein expression of BNDF and its receptor. As such, they found that ELF-EMF caused up-regulation in neurons (Di Loreto et al., 2009).
The regenerative ability of ELF-EMF has been confirmed by Hei et al. (2016) They observed that pulsating electromagnetic fields (50 Hz, 1 mT) caused increased BDNF gene expression in immortalized rat Schwann cells. They suggested that the electromagnetic field improved regeneration of peripheral nerves by enhancing proliferation of cells, and BDNF and S100 gene expression (Hei et al., 2016). Similar evidence was provided by Urnukhsaikhan et al. (2017). The increase of BDNF, protein level after EMF treatment, confirmed the neuroprotective action of EMF in mice during recovery process after stroke (Urnukhsaikhan et al., 2017).
The probable mechanism of increase of BDNF mRNA expression after EMF treatment was explained by Li et al. (2014). They investigated L-type voltage-gated calcium channels-and Erk-dependent signaling pathways and sampled BNDF mRNA expression in cultured dorsal root ganglion neurons (Li et al., 2014). Sun et al. (2016) investigated membrane capacitance and calcium influx in the calyx of Held. They certified the significance of the effect of ELF-EMF on plasticity and synaptic transmission by facilitation of synaptic plasticity in a calcium-dependent manner, and vesicle endocytosis. Exposition of ELF-EMF causes increases in the impact of calcium influx on the enhancement of calcium channel expression at the presynaptic nerve terminal (Sun et al., 2016).
The primary function of VEGF is a pro-angiogenic action, but there is much evidence of its neurotrophic and neuroprotective effect, both on the central and peripheral nervous system (Saban et al., 2011; Yao et al., 2016; Shahhoseini and Bigdeli, 2017). VEGF generated by ependymal cells activates and enhances neuronal precursor proliferation and growth in the SCZ and SGZ zones (Chodobski et al., 2003). Moreover, VEGF enhances astrocytes proliferation and migration (Lenzer-Fanara et al., 2017), as well as stimulates growth and survival of Schwann cells after hypoxia (Cattin et al., 2015). Its neurogenesis effect is assessed by stimulation of the endothelium to release neurotrophic factors (Feng et al., 2012).
In the current study, we observed that the VEGF plasma level increased in the group exposed to ELF-EMF (Figure 2). However, our results are compatible with studies conducted by Delle Monache et al. (2008), who suggested ELF-EMF’s impact on in vitro modulation of endothelial functions through VEGF-dependent signal transduction pathways. Liu et al. (2015a) estimated the effect of a pulsating electromagnetic field on neurotrophic genes’ expression and proliferation in Schwann cells in rats. They observed that EMF increased both protein levels and the gene expression of VEGF, BDNF, and GDNF. They thereby confirm that EMF therapy improves nerve regeneration (Liu et al., 2015a).
We also measured the cytokine plasma levels involved in neuroplasticity processes: HGF, SCF, SDF-1α, β-NGF, and LIF. We observed that after the application of ELF-EMF, hepatocyte growth factor increased (Table 3 and Figure 3A). HGF is expressed in many different tissues, including the brain (Sharma, 2010). HGF by cMet receptor impacts morphogenesis, cell motility, and proliferation of neuronal and non-neuronal tissues. It also activates the migration and proliferation of the progenitors of oligodendrocyte, Schwann cells, as well as intensifying the differentiation and survival of hippocampal, cortical, and midbrain dopaminergic neurons (Wang et al., 2011). Shang et al. (2011) found that in Wistar rats, after transient middle cerebral artery occlusion (tMCAO), exogenous administration of HGF caused a decrease in infarct size, intensification of synaptogenesis and angiogenesis, and a reduction of scar thickness of the pia mater and glial scar formation (Shang et al., 2011). Similarly, Doeppner et al. (2011) investigated the impact of intrastriatal HGF treatment on the long-term effects of and neurologic recovery and brain injury. They suggested that long-term neuroprotection caused by HGF was associated with enhanced neurovascular remodeling, and maintained recruitment of proliferating cells (Doeppner et al., 2011).
Stem cell factor (SCF) also has an impact on neuroprotection and neurogenesis. It is involved in the development of the cortex, and migration and proliferation of neural progenitor cells. SCF in microglia enhances BNDF and NGF expression and reduces pro-inflammatory cytokine expression. Furthermore, SCF participates in neuron-glia, and neuron-neuron interaction (Benedetti et al., 2016). Liu et al. (2016) estimated the effect of administration of SCF and granulocyte-colony stimulating factor (G-CSF) on the effectiveness of recovery in aged mice after stroke. A similar study was conducted by Cui et al., who investigated the effect of the SCF and G-CSF combination on brain repair, 6 months after cortical injury in transgenic mice. They also suggested that SCF + G-SCF treatment enhanced motor function through vascular and synaptic regeneration (Cui et al., 2016). In this study, we showed that the plasma concentration of SCF increased about 25% in the ELF-EMF group (Table 3 and Figure 3B), and this is consistent with Fan et al. whose evaluated the effect of ELF-EMF (50 Hz, 1 mT) on cytokine production and proliferation of mesenchymal stem cells (MSC) in rats. They found that SCF mRNA expression after ELF-EMF increased in comparison to their control group. They suggested that ELF-EMF enhanced the proliferation of MSC, as well as up-regulated haematopoietic growth factor expression (Fan et al., 2015).
Stromal derived factor-1α (SDF-1α) is a chemokine secreted from the endothelium which can induce neuroblast migration from SVZ to the ischemic area in rats (Zhao et al., 2015). Luo et al. (2014) demonstrated the impact of physical exercise on functional recovery by improving migration, differentiation, and proliferation of NSCs in SDF-1α rats after MCAO. In our study, we observed no change in SDF-1α, in both the ELF-EMF and non-ELF-EMF groups (Table 3 and Figure 3C).
Despite nerve growth factor (NGF) being a neurotrophic factor predominantly involved in neuroplasticity (Isaev et al., 2017), in our study, the βNGF level in both groups before and after treatment was out of range (Table 3). Similarly, leukemia inhibitory factor (LIF), which is an anti-inflammatory cytokine involved in brain plasticity (Vidal et al., 2013), was out of range (Table 3). In research by Sarchielli et al. (2013) the level of these molecules was also below the detection limit.
In our study we suggested that ELF-EMF improved neuroplasticity, which idea is compatible with data from the existing literature (Oda and Koike, 2004; Cuccurazzu et al., 2010; Balassa et al., 2013; Cheng et al., 2015). Cuccurazzu et al. (2010) investigated the effect of ELF-EMF (50Hz, 1mT) on hippocampal neurogenesis in adult mice. They observed that ELF-EMF exposition increased the expression of Mash1, NeuroD2, and Hes1 (pro-neuronal transcription genes), and genes encoding Ca(v)1.2 channel α(1C) subunits, thus promoted neurogenesis in the dentate gyrus (Cuccurazzu et al., 2010). On the other hand, Balassa et al. estimated the impact of long-term ELF-EMF (50 Hz, 3mT) synaptic functions in the developing brain. They found that exposure to ELF-EMF enhanced synaptic plasticity and basic neuronal functions in the brain, both in newborn and fetal rats (Balassa et al., 2013). Oda and Koike examined the effect of ELF-EMF (50 Hz, 0.3 mT) on neuronal apoptosis. They observed that application of ELF-EMF inhibited apoptosis and enhanced the survival of immature cerebellar granule neurons (Oda and Koike, 2004). Moreover, Cheng et al. investigated the impact of ELF-EMF (50 Hz, 0.4 mT) on hippocampal neural progenitor cells from both ischaemic and embryonic brains. They observed that application of ELF-EMF intensified the ability of neural progenitor cells to proliferate in both kinds of the brain (Cheng et al., 2015).
In our study, we also found a relationship between ELF-EMF and the enhancement of the clinical parameters of tested subjects, as well as a correlation between BDNF level and clinical parameters (Figure 5). The results we obtained show that the ADL value was comparable in both groups of patients (Figure 4) and that there was a significant positive correlation between an increase of BDNF level and ΔADL in the ELF-EMF group (Figure 5 and Table 4). Our findings are compatible with results obtained by Zhang et al. (2017). They evaluated functional recovery and serum BDNF level in post-stroke patients and the relationship between the two. They observed a positive correlation between BNDF level and Barhel Index, and between BNDF level and mRS, which indicated functional status (Zhang et al., 2017). The increase in the MMSE parameter before and after ELF-EMF treatment was about 15% (Figure 4), and we proved a significantly positive correlation between ΔBDNF level and ΔMMSE in our study group (Figure 5 and Table 4). A positive correlation between BDNF level and the MMSE scale was previously shown by Belviranli et al. (2016) who investigated the dependence of cognitive parameters (assessed by MMSE) and BDNF plasma level in endurance athletes. Similarity, Levada et al. (2016) observed that after BDNF administration in patients with mild neurocognitive disorders, their MMSE level increased. The decline in parameters on the GDS scale was about 50% greater in the ELF-EMF group, in comparison to the non-ELF-EMF group. We also demonstrated a significant positive correlation between changes of BDNF level and ΔGDS (Figure 5 and Table 4). Importantly, a low BDNF level is associated with post-stroke depression and acute stroke (Yang et al., 2011), and over-expression of BDNF in the hippocampus moderates depression behaviors in post-stroke depressive rats (Chen et al., 2015).
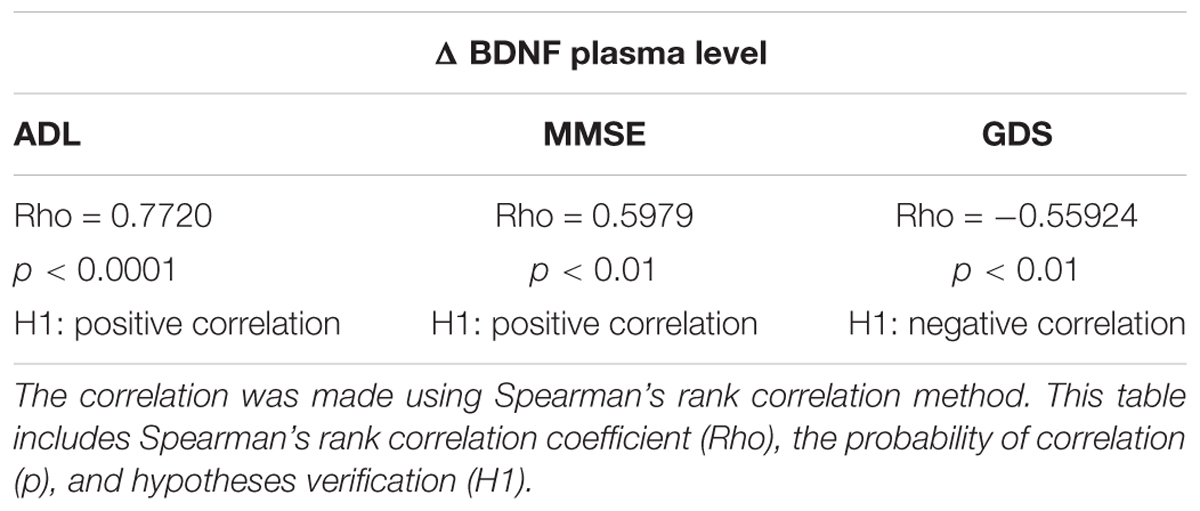
TABLE 4. Correlation coefficient values obtained for the change in BDNF plasma level (ΔBDNF) and parameters of functional status (ADL, MMSE, and GDS) after ELF-EMF treatment.
Conclusion
ELF-EMF improves functional recovery in stroke patients by improving neuroplasticity processes. Intensifying brain plasticity using ELF-EMF therapy is associated with an increased level of neurotrophic factors, which could be caused by the impact of ELF-EMF on gene expression. We also suggested that the inclusion of ELF-EMF treatment in post-stroke therapy could enhance the effectiveness of the therapy.
Ethics Statement
This study was approved by the Bioethics Committee of the Faculty of Biology and Environmental Protection of University of Lodz, Poland with Resolution No. 13/KBBN-UŁ/II/2016. All participants gave their written informed consent before participation. The study was executed according to the principles of the Helsinki Declaration.
Author Contributions
NC was involved in the preparation of the research project, preparation, collection and interpretation of data, as well as preparation of the manuscript. MB was involved in the interpretation of data, statistical analysis, and preparation of the manuscript. PC was involved in the preparation of samples (isolation of RNA) and determination of VEGF. EM was responsible for patient recruitment and was involved in the analysis and interpretation of clinical data. ES was involved in the evaluation of the quality of RNA and preparation of cDNA. TS analyzed and interpreted of obtained data and was responsible for language correction. JS-B was involved in the preparation of the research project, critically revision, analysis and interpretation of the data, and preparation of the manuscript. All authors read and approved the final manuscript.
Funding
This study was supported by the Department of General Biochemistry, Faculty of Biology and Environmental Protection, University of Lodz (No. 506/1136) and Grants for Young Scientists and PhD Students (B1611000001145.02).
Conflict of Interest Statement
The authors declare that the research was conducted in the absence of any commercial or financial relationships that could be construed as a potential conflict of interest.
The reviewer EC-J and handling Editor declared their shared affiliation at the time of the review.
References
Aimone, J. B., Li, Y., Lee, S. W., Clemenson, G. D., Deng, W., and Gage, F. H. (2014). Regulation and function of adult neurogenesis: from genes to cognition. Physiol. Rev. 94, 991–1026. doi: 10.1152/physrev.00004.2014
Balassa, T., Varró, P., Elek, S., Drozdovszky, O., Szemerszky, R., Világi, I., et al. (2013). Changes in synaptic efficacy in rat brain slices following extremely low-frequency magnetic field exposure at embryonic and early postnatal age. Int. J. Dev. Neurosci. 31, 724–730. doi: 10.1016/j.ijdevneu.2013.08.004
Bath, K. G., and Lee, F. S. (2010). Neurotrophic factor control of adult SVZ neurogenesis. Dev. Neurobiol. 70, 339–349. doi: 10.1002/dneu.20781
Bathina, S., and Das, U. N. (2015). Brain-derived neurotrophic factor and its clinical implications. Arch. Med. Sci. 11, 1164–1178. doi: 10.5114/aoms.2015.56342
Belviranli, M., Okudan, N., Kabak, B., Erdoğan, M., and Karanfilci, M. (2016). The relationship between brain-derived neurotrophic factor, irisin and cognitive skills of endurance athletes. Phys. Sportsmed. 44, 290–296. doi: 10.1080/00913847.2016.1196125
Benedetti, F., Poletti, S., Hoogenboezem, T. A., Locatelli, C., Ambrée, O., de Wit, H., et al. (2016). Stem cell factor (SCF) is a putative biomarker of antidepressant response. J. Neuroimmune Pharmacol. 11, 248–258. doi: 10.1007/s11481-016-9672-y
Bijak, M., Kolodziejczyk-Czepas, J., Ponczek, M. B., Saluk, J., and Nowak, P. (2012). Protective effects of grape seed extract against oxidative and nitrative damage of plasma proteins. Int. J. Biol. Macromol. 51, 183–187. doi: 10.1016/j.ijbiomac.2012.05.009
Bijak, M., Saluk, J., Antosik, A., Ponczek, M. B., Zbikowska, H. M., Borowiecka, M., et al. (2013). Aronia melanocarpa as a protector against nitration of fibrinogen. Int. J. Biol. Macromol. 55, 264–268. doi: 10.1016/j.ijbiomac.2013.01.019
Cattin, A. L., Burden, J. J., Van Emmenis, L., Mackenzie, F. E., Hoving, J. J., Garcia Calavia, N., et al. (2015). Macrophage-induced blood vessels guide schwann cell-mediated regeneration of peripheral nerves. Cell 162, 1127–1139. doi: 10.1016/j.cell.2015.07.021
Chen, H. H., Zhang, N., Li, W. Y., Fang, M. R., Zhang, H., Fang, Y. S., et al. (2015). Overexpression of brain-derived neurotrophic factor in the hippocampus protects against post-stroke depression. Neural Regen. Res. 10, 1427–1432. doi: 10.4103/1673-5374.165510
Cheng, Y., Dai, Y., Zhu, X., Xu, H., Cai, P., Xia, R., et al. (2015). Extremely low-frequency electromagnetic fields enhance the proliferation and differentiation of neural progenitor cells cultured from ischemic brains. Neuroreport 26, 896–902. doi: 10.1097/WNR.0000000000000450
Chodobski, A., Chung, I., Koźniewska, E., Ivanenko, T., Chang, W., Harrington, J. F., et al. (2003). Early neutrophilic expression of vascular endothelial growth factor after traumatic brain injury. Neuroscience 122, 853–867. doi: 10.1016/j.neuroscience.2003.08.055
Choi, J. Y., Kim, J. Y., Park, J., Lee, W. T., and Lee, J. E. (2017). M2 phenotype microglia-derived cytokine stimulates proliferation and neuronal differentiation of endogenous stem cells in ischemic brain. Exp. Neurobiol. 26, 33–41. doi: 10.5607/en.2017.26.1.33
Cichoń, N., Bijak, M., Miller, E., and Saluk, J. (2017a). Extremely low frequency electromagnetic field (ELF-EMF) reduces oxidative stress and improves functional and psychological status in ischemic stroke patients. Bioelectromagnetics 38, 386–396. doi: 10.1002/bem.22055
Cichoń, N., Czarny, P., Bijak, M., Miller, E., Śliwinñski, T., Szemraj, J., et al. (2017b). Benign effect of extremely low-frequency electromagnetic field on brain plasticity assessed by nitric oxide metabolism during poststroke rehabilitation. Oxid. Med. Cell. Longev. 2017:2181942. doi: 10.1155/2017/2181942
Cichoń, N., Rzeźnicka, P., Bijak, M., Miller, E., Miller, S., and Saluk, J. (2018). Extremely low frequency electromagnetic field reduces oxidative stress during the rehabilitation of post-acute stroke patients. Adv. Clin. Exp. Med. doi: 10.17219/acem/73699 [Epub ahead of print].
Cuccurazzu, B., Leone, L., Podda, M. V., Piacentini, R., Riccardi, E., Ripoli, C., et al. (2010). Exposure to extremely low-frequency (50 Hz) electromagnetic fields enhances adult hippocampal neurogenesis in C57BL/6 mice. Exp. Neurol 226, 173–182. doi: 10.1016/j.expneurol.2010.08.022
Cui, L., Wang, D., McGillis, S., Kyle, M., and Zhao, L. R. (2016). Repairing the brain by SCF+G-CSF Treatment at 6 months postexperimental stroke: mechanistic determination of the causal link between neurovascular regeneration and motor functional recovery. ASN Neuro 8:1759091416655010. doi: 10.1177/1759091416655010
Delle Monache, S., Alessandro, R., Iorio, R., Gualtieri, G., and Colonna, R. (2008). Extremely low frequency electromagnetic fields (ELF-EMFs) induce in vitro angiogenesis process in human endothelial cells. Bioelectromagnetics 29, 640–648. doi: 10.1002/bem.20430
Di Loreto, S., Falone, S., Caracciolo, V., Sebastiani, P., D’Alessandro, A., Mirabilio, A., et al. (2009). Fifty hertz extremely low-frequency magnetic field exposure elicits redox and trophic response in rat-cortical neurons. J. Cell. Physiol. 219, 334–343. doi: 10.1002/jcp.21674
Doeppner, T. R., Kaltwasser, B., ElAli, A., Zechariah, A., Hermann, D. M., and Bähr, M. (2011). Acute hepatocyte growth factor treatment induces long-term neuroprotection and stroke recovery via mechanisms involving neural precursor cell proliferation and differentiation. J. Cereb. Blood Flow Metab. 31, 1251–1262. doi: 10.1038/jcbfm.2010.211
Fan, W., Qian, F., Ma, Q., Zhang, P., Chen, T., Chen, C., et al. (2015). 50 Hz electromagnetic field exposure promotes proliferation and cytokine production of bone marrow mesenchymal stem cells. Int. J. Clin. Exp Med. 8, 7394–7404.
Feng, S., Zhuang, M., and Wu, R. (2012). Secretion of nerve growth factor, brain-derived neurotrophic factor, and glial cell-line derived neurotrophic factor in co-culture of four cell types in cerebrospinal fluid-containing medium. Neural Regen. Res. 7, 2907–2914. doi: 10.3969/j.issn.1673-5374.2012.36.008
Gomes, J. R., Costa, J. T., Melo, C. V., Felizzi, F., Monteiro, P., Pinto, M. J., et al. (2012). Excitotoxicity downregulates TrkB.FL signaling and upregulates the neuroprotective truncated TrkB receptors in cultured hippocampal and striatal neurons. J. Neurosci. 32, 4610–4622. doi: 10.1523/JNEUROSCI.0374-12.2012
Greenberg, M. E., Xu, B., Lu, B., and Hempstead, B. L. (2009). New insights in the biology of BDNF synthesis and release: implications in CNS function. J. Neurosci. 29, 12764–12767. doi: 10.1523/JNEUROSCI.3566-09.2009
Hagg, T. (2009). From neurotransmitters to neurotrophic factors to neurogenesis. Neuroscientist 15, 20–27. doi: 10.1177/1073858408324789
Hei, W. H., Byun, S. H., Kim, J. S., Kim, S., Seo, Y. K., Park, J. C., et al. (2016). Effects of electromagnetic field (PEMF) exposure at different frequency and duration on the peripheral nerve regeneration: in vitro and in vivo study. Int. J. Neurosci. 126, 739–748. doi: 10.3109/00207454.2015.1054032
Hu, X., Zhang, F., Leak, R. K., Zhang, W., Iwai, M., Stetler, R. A., et al. (2013). Transgenic overproduction of omega-3 polyunsaturated fatty acids provides neuroprotection and enhances endogenous neurogenesis after stroke. Curr. Mol. Med. 13, 1465–1473. doi: 10.2174/15665240113139990075
Hylin, M. J., Kerr, A. L., and Holden, R. (2017). Understanding the mechanisms of recovery and/or compensation following injury. Neural Plast. 2017:7125057. doi: 10.1155/2017/7125057
Isaev, N. K., Stelmashook, E. V., and Genrikhs, E. E. (2017). Role of nerve growth factor in plasticity of forebrain cholinergic neurons. Biochemistry 82, 291–300. doi: 10.1134/S0006297917030075
Jia, H., Pei, Q., Sullivan, C. T., Cowper Ripley, D. C., Wu, S. S., Vogel, W. B., et al. (2017). Regional variation in post-stroke multidisciplinary rehabilitation care among veteran residents in community nursing homes. J. Multidiscip. Healthc. 10, 75–85. doi: 10.2147/JMDH.S123905
Klein, R., Mahlberg, N., Ohren, M., Ladwig, A., Neumaier, B., Graf, R., et al. (2016). The neural cell adhesion molecule-derived (NCAM)-peptide FG loop (FGL) mobilizes endogenous neural stem cells and promotes endogenous regenerative capacity after stroke. J. Neuroimmune Pharmacol. 11, 708–720. doi: 10.1007/s11481-016-9694-5
Law, H. C., Szeto, S. S., Quan, Q., Zhao, Y., Zhang, Z., Krakovska, O., et al. (2017). Characterization of the molecular mechanisms underlying the chronic phase of stroke in a cynomolgus monkey model of induced cerebral ischemia. J. Proteome. Res. 16, 1150–1166. doi: 10.1021/acs.jproteome.6b00651
Lenzer-Fanara, J. R., Li, T., Salerni, E. A., Payen, F., and Croll, S. D. (2017). VEGF treatment during status epilepticus attenuates long-term seizure-associated alterations in astrocyte morphology. Epilepsy Behav. 70(Pt A), 33–44. doi: 10.1016/j.yebeh.2017.02.019
Levada, O. A., Cherednichenko, N. V., Trailin, A. V., and Troyan, A. S. (2016). Plasma brain-derived neurotrophic factor as a biomarker for the main types of mild neurocognitive disorders and treatment efficacy: a preliminary study. Dis. Mark. 2016:4095723. doi: 10.1155/2016/4095723
Li, S. (2017). Spasticity, motor recovery, and neural plasticity after stroke. Front. Neurol. 8:120. doi: 10.3389/fneur.2017.00120
Li, Y., Yan, X., Liu, J., Li, L., Hu, X., Sun, H., et al. (2014). Pulsed electromagnetic field enhances brain-derived neurotrophic factor expression through L-type voltage-gated calcium channel- and Erk-dependent signaling pathways in neonatal rat dorsal root ganglion neurons. Neurochem. Int. 75, 96–104. doi: 10.1016/j.neuint.2014.06.004
Liguz-Lecznar, M., and Kossut, M. (2013). Influence of inflammation on poststroke plasticity. Neural Plast. 2013, 258582. doi: 10.1155/2013/258582
Lindholm, P., and Saarma, M. (2010). Novel CDNF/MANF family of neurotrophic factors. Dev. Neurobiol. 70, 360–371. doi: 10.1002/dneu.20760
Ling, G. Q., Liu, Y. J., Ke, Y. Q., Chen, L., Jiang, X. D., Jiang, C. L., et al. (2015). All-trans retinoic acid impairs the vasculogenic mimicry formation ability of U87 stem-like cells through promoting differentiation. Mol. Med. Rep. 12, 165–172. doi: 10.3892/mmr.2015.3449
Liu, L., Liu, Z., Huang, L., Sun, Z., Ma, T., Zhu, S., et al. (2015a). Pulsed magnetic field promotes proliferation and neurotrophic genes expression in Schwann cells in vitro. Int. J. Clin. Exp. Pathol. 8, 2343–2353.
Liu, X. Y., Zhou, X. Y., Hou, J. C., Zhu, H., Wang, Z., Liu, J. X., et al. (2015b). Ginsenoside Rd promotes neurogenesis in rat brain after transient focal cerebral ischemia via activation of PI3K/Akt pathway. Acta Pharmacol. Sin. 36, 421–428. doi: 10.1038/aps.2014.156
Liu, Y., Popescu, M., Longo, S., Gao, M., Wang, D., McGillis, S., et al. (2016). Fibrinogen reduction and motor function improvement by hematopoietic growth factor treatment in chronic stroke in aged mice: a treatment frequency study. Cell Transplant. 25, 729–734. doi: 10.3727/096368916X690791
Luo, J., Hu, X., Zhang, L., Li, L., Zheng, H., Li, M., et al. (2014). Physical exercise regulates neural stem cells proliferation and migration via SDF-1α/CXCR4 pathway in rats after ischemic stroke. Neurosci. Lett. 578, 203–208. doi: 10.1016/j.neulet.2014.06.059
Martin, B., Wang, R., Cong, W. N., Daimon, C. M., Wu, W. W., Ni, B., et al. (2017). Altered learning, memory, and social behavior in type 1 taste receptor subunit 3 knock-out mice are associated with neuronal dysfunction. J. Biol. Chem. 292, 11508–11530. doi: 10.1074/jbc.M116.773820
Oda, T., and Koike, T. (2004). Magnetic field exposure saves rat cerebellar granule neurons from apoptosis in vitro. Neurosci. Lett. 365, 83–86. doi: 10.1016/j.neulet.2004.04.068
Qiao, H. J., Li, Z. Z., Wang, L. M., Sun, W., Yu, J. C., and Wang, B. (2017). Association of lower serum brain-derived neurotrophic factor levels with larger infarct volumes in acute ischemic stroke. J. Neuroimmunol. 307, 69–73. doi: 10.1016/j.jneuroim.2017.04.002
Rosenstein, J. M., Krum, J. M., and Ruhrberg, C. (2010). VEGF in the nervous system. Organogenesis 6, 107–114. doi: 10.4161/org.6.2.11687
Saban, M. R., Davis, C. A., Avelino, A., Cruz, F., Maier, J., Bjorling, D. E., et al. (2011). VEGF signaling mediates bladder neuroplasticity and inflammation in response to BCG. BMC Physiol. 11:16. doi: 10.1186/1472-6793-11-16
Sarchielli, P., Nardi, K., Chiasserini, D., Eusebi, P., Tantucci, M., Di Piero, V., et al. (2013). Immunological profile of silent brain infarction and lacunar stroke. PLoS One 8:e68428. doi: 10.1371/journal.pone.0068428
Schiavone, S., Tucci, P., Mhillaj, E., Bove, M., Trabace, L., and Morgese, M. G. (2017). Antidepressant drugs for beta amyloid-induced depression: a new standpoint? Prog. Neuropsychopharmacol. Biol. Psychiatry 78, 114–122. doi: 10.1016/j.pnpbp.2017.05.004
Shahhoseini, M., and Bigdeli, M. R. (2017). Time course of neuroprotection induced by. Iran J. Basic Med. Sci. 20, 67–74.
Shang, J., Deguchi, K., Ohta, Y., Liu, N., Zhang, X., Tian, F., et al. (2011). Strong neurogenesis, angiogenesis, synaptogenesis, and antifibrosis of hepatocyte growth factor in rats brain after transient middle cerebral artery occlusion. J. Neurosci. Res. 89, 86–95. doi: 10.1002/jnr.22524
Sharma, S. (2010). Hepatocyte growth factor in synaptic plasticity and Alzheimer’s disease. Sci. World J. 10, 457–461. doi: 10.1100/tsw.2010.49
Sun, Z. C., Ge, J. L., Guo, B., Guo, J., Hao, M., Wu, Y. C., et al. (2016). Extremely low frequency electromagnetic fields facilitate vesicle endocytosis by increasing presynaptic calcium channel expression at a central synapse. Sci. Rep. 6:21774. doi: 10.1038/srep21774
Urnukhsaikhan, E., Mishig-Ochir, T., Kim, S. C., Park, J. K., and Seo, Y. K. (2017). Neuroprotective effect of low frequency-pulsed electromagnetic fields in ischemic stroke. Appl. Biochem. Biotechnol. 181, 1360–1371. doi: 10.1007/s12010-016-2289-z
Vidal, P. M., Lemmens, E., Dooley, D., and Hendrix, S. (2013). The role of “anti-inflammatory” cytokines in axon regeneration. Cytokine Growth Factor Rev. 24, 1–12. doi: 10.1016/j.cytogfr.2012.08.008
Wang, T. W., Zhang, H., Gyetko, M. R., and Parent, J. M. (2011). Hepatocyte growth factor acts as a mitogen and chemoattractant for postnatal subventricular zone-olfactory bulb neurogenesis. Mol. Cell. Neurosci. 48, 38–50. doi: 10.1016/j.mcn.2011.06.003
Yang, L., Zhang, Z., Sun, D., Xu, Z., Yuan, Y., Zhang, X., et al. (2011). Low serum BDNF may indicate the development of PSD in patients with acute ischemic stroke. Int. J. Geriatr. Psychiatry 26, 495–502. doi: 10.1002/gps.2552
Yao, Y., Zheng, X. R., Zhang, S. S., Wang, X., Yu, X. H., Tan, J. L., et al. (2016). Transplantation of vascular endothelial growth factor-modified neural stem/progenitor cells promotes the recovery of neurological function following hypoxic-ischemic brain damage. Neural Regen. Res. 11, 1456–1463. doi: 10.4103/1673-5374.191220
Zanotta, N., Tornesello, M. L., Annunziata, C., Stellato, G., Buonaguro, F. M., and Comar, M. (2016). Candidate soluble immune mediators in young women with high-risk human papillomavirus infection: high expression of chemokines promoting angiogenesis and cell proliferation. PLoS One 11:e0151851. doi: 10.1371/journal.pone.0151851
Zelentsova-Levytskyi, K., Talmi, Z., Abboud-Jarrous, G., Capucha, T., Sapir, T., Burstyn-Cohen, T., et al. (2017). Negatively regulates neural stem cell self-renewal through Bmi-1 signaling. Front. Mol. Neurosci. 10:124. doi: 10.3389/fnmol.2017.00124
Zhang, J., Mu, X., Breker, D. A., Li, Y., Gao, Z., and Huang, Y. (2017). Atorvastatin treatment is associated with increased BDNF level and improved functional recovery after atherothrombotic stroke. Int. J. Neurosci. 127, 92–97. doi: 10.3109/00207454.2016.1146882
Keywords: extremely low-frequency electromagnetic field, neuroplasticity, brain-derived neurotrophic factor, stroke, rehabilitation
Citation: Cichoń N, Bijak M, Czarny P, Miller E, Synowiec E, Sliwinski T and Saluk-Bijak J (2018) Increase in Blood Levels of Growth Factors Involved in the Neuroplasticity Process by Using an Extremely Low Frequency Electromagnetic Field in Post-stroke Patients. Front. Aging Neurosci. 10:294. doi: 10.3389/fnagi.2018.00294
Received: 13 April 2018; Accepted: 06 September 2018;
Published: 26 September 2018.
Edited by:
Ashok Kumar, University of Florida, United StatesReviewed by:
Eduardo Candelario-Jalil, University of Florida, United StatesHyacinth Idu Hyacinth, Emory University, United States
Copyright © 2018 Cichoń, Bijak, Czarny, Miller, Synowiec, Sliwinski and Saluk-Bijak. This is an open-access article distributed under the terms of the Creative Commons Attribution License (CC BY). The use, distribution or reproduction in other forums is permitted, provided the original author(s) and the copyright owner(s) are credited and that the original publication in this journal is cited, in accordance with accepted academic practice. No use, distribution or reproduction is permitted which does not comply with these terms.
*Correspondence: Natalia Cichoń, bmF0YWxpYS5jaWNob25AYmlvbC51bmkubG9kei5wbA==