- 1Research Group Neuroprotection, German Center for Neurodegenerative Diseases (DZNE), Magdeburg, Germany
- 2Department of Sport Science, Institute III, Otto von Guericke University Magdeburg, Magdeburg, Germany
- 3Center for Behavioral Brain Sciences (CBBS), Magdeburg, Germany
- 4Department of Neurology, Medical Faculty, Otto von Guericke University Magdeburg, Magdeburg, Germany
The demographic change in industrial countries, with increasingly sedentary lifestyles, has a negative impact on mental health. Normal and pathological aging leads to cognitive deficits. This development poses major challenges on national health systems. Therefore, it is necessary to develop efficient cognitive enhancement strategies. The combination of regular physical exercise with cognitive stimulation seems especially suited to increase an individual’s cognitive reserve, i.e., his/her resistance to degenerative processes of the brain. Here, we outline insufficiently explored fields in exercise-cognition research and provide a classification approach for different motor-cognitive training regimens. We suggest to classify motor-cognitive training in two categories, (I) sequential motor-cognitive training (the motor and cognitive training are conducted time separated) and (II) simultaneous motor-cognitive training (motor and cognitive training are conducted sequentially). In addition, simultaneous motor-cognitive training may be distinguished based on the specific characteristics of the cognitive task. If successfully solving the cognitive task is not a relevant prerequisite to complete the motor-cognitive task, we would consider this type of training as (IIa) motor-cognitive training with additional cognitive task. In contrast, in ecologically more valid (IIb) motor cognitive training with incorporated cognitive task, the cognitive tasks are a relevant prerequisite to solve the motor-cognitive task. We speculate that incorporating cognitive tasks into motor tasks, rather than separate training of mental and physical functions, is the most promising approach to efficiently enhance cognitive reserve. Further research investigating the influence of motor(-cognitive) exercises with different quantitative and qualitative characteristics on cognitive performance is urgently needed.
Introduction
A crucial aspect of human living is motion. While the control of movements requires cognitive processes, moving probably influences cognition and its underlying processes (structures), too (Hamacher et al., 2015c). Cognition is a term covering a wide range of mental abilities that are necessary to percept, process, and interact with our environment (Bostrom and Sandberg, 2009; Borson, 2010). Therefore, intact cognitive processes are fundamental for human living. Across the life span, cognitive performance is influenced and changed by many factors. Normal aging is associated with a decline of cognitive functions such as processing speed and memory (Albert, 1997; Park et al., 2002; Buckner, 2004; Hedden and Gabrieli, 2004; Fjell and Walhovd, 2010). Moreover, old age is also a risk factor for developing neurological diseases like dementia (Fiest et al., 2016). Dementia has a negative impact on the cognitive performance of an individual and reduces the autonomy as well as the quality of life (Andersen et al., 2004; Scherder et al., 2011; Fiest et al., 2016). Remarkably, dementia is a major contributor to health care costs (Hurd et al., 2013; Wimo et al., 2013), and it is expected that the number of those affected by dementia will almost double every 20 years (Prince et al., 2013). Furthermore, the neuropathological signs of dementia aggravate with physical inactivity (Scherder et al., 2010). Physical inactivity and sedentary behavior are associated with reduced cognitive functions (Falck et al., 2016; Ku et al., 2017) and increased welfare costs (Janssen, 2012; Peeters et al., 2014), too. Unfortunately, the average time people are physically inactive in daily life has increased substantially in the last decades in western countries (Owen et al., 2010; Church et al., 2011). Taken together, the proportion of individuals with poorer cognitive capacities will increase in the next years, which makes it necessary to develop effective cognitive enhancement strategies (Colzato, 2016) that serve to enhance the cognitive reserve [defined as individual differences in how people process tasks; detailed description of term and concept could be found in Stern (2002, 2009)] and the resilience against neurodegeneration (Nithianantharajah and Hannan, 2009; Stern, 2012, 2013).
Physical Activity and Cognition
Apart from a healthy diet and cognitive stimulation, (I) physical activity which is defined as any bodily, muscle-produced movement that increases the energy expenditure above ∼1.5 metabolic equivalent of task [1 MET = 1 kcal (4,184 kJ) × kg-1 × h-1] (Caspersen et al., 1985; Ainsworth et al., 2000; Mansoubi et al., 2015) and (II) physical exercise (training), which is defined as a planned, structured (repetitive) form of distinct physical activities (Caspersen et al., 1985; Howley, 2001; Budde et al., 2016) are proposed crucial for the preservation of cognitive functions and the enhancement of the cognitive reserve. For instance, physical activity and/or exercise were shown to improve cognition in children (Hillman and Schott, 2013; Chaddock-Heyman et al., 2014; Khan and Hillman, 2014; Verburgh et al., 2014; Ludyga et al., 2016), in adolescents (Verburgh et al., 2014; Esteban-Cornejo et al., 2015; Greeff et al., 2017; Li et al., 2017), in young and middle-aged adults (Verburgh et al., 2014; Cox et al., 2016), in older adults (Colcombe and Kramer, 2003; Bherer et al., 2013; Erickson et al., 2013; Carvalho et al., 2014; Northey et al., 2017), and in persons suffering from neurocognitive disorders such as dementia or mild cognitive impairment (Heyn et al., 2004; Smith et al., 2013; Groot et al., 2016; Ahn et al., 2017; Song et al., 2018). Moreover, regardless of whether physical exercise is conducted in a single (acute) exercise bout (Chang et al., 2012; Roig et al., 2013) and/or in form of multiple (chronic) exercise bouts (Bherer et al., 2013; Hötting and Röder, 2013; Roig et al., 2013; Esteban-Cornejo et al., 2015), it has been demonstrated to enhance cognitive performance. Furthermore, it is speculated that regular physical activity and physical exercising prevent cognitive decline and neurological diseases (Ahlskog, 2011; Sofi et al., 2011; Blondell et al., 2014; Paillard, 2015). However, which exact prerequisites (e.g., intensity, duration, frequency, type of exercise) make an exercise optimal for effectively enhancing cognition are largely unknown (Hillman et al., 2008; Lustig et al., 2009; Rolland et al., 2010; Bherer et al., 2013; Voelcker-Rehage and Niemann, 2013; Cai and Abrahamson, 2015; Esteban-Cornejo et al., 2015; Lauenroth et al., 2016; Batouli and Saba, 2017; Tait et al., 2017). Often, aerobic (cardiovascular) exercises like cycling, walking, or running are used in interventions to enhance cognitive fitness, especially in the elderly (Roig et al., 2013; Voelcker-Rehage and Niemann, 2013). For instance, a 6-month walking intervention leads to an increased hippocampal volume and improved memory performance in seniors (Erickson et al., 2011). Remarkably, preceding aerobic exercises boost performance in an alter reaction-time test (Pontifex et al., 2009) but not in executive functions when compared to preceding resistance exercises (Alves et al., 2012).
Interestingly, physical and motor fitness (coordinative abilities) are both related to cognitive performance in older individuals (Voelcker-Rehage et al., 2010) but do evoke differential structural adaptions (Voelcker-Rehage et al., 2011; Voelcker-Rehage and Niemann, 2013; Paillard, 2015) or correlate only with distinct cognitive functions (Marchetti et al., 2015). For instance, a 12-month aerobic exercise intervention led to increased activation in sensori-motor networks while coordinative training increased activation in the visual–spatial network during an executive function test (Voelcker-Rehage et al., 2011).
An exercise type that demands a high level of coordinative abilities is dancing (Dhami et al., 2014; Hamacher et al., 2015a,b). Participating in a 6-month dancing intervention enhanced attentional performance to a higher degree than participating in a fall prevention or Tai Chi Chuan program (Coubard et al., 2011). Additionally, in Parkinson disease dancing led to higher improvements in physical and cognitive functions than aerobic exercise (Hashimoto et al., 2015). Regular dancing has been associated with a lower risk of dementia (Verghese et al., 2003) presumably because it induces a larger increase in gray matter volume in frontal regions (Müller et al., 2016, 2017) and the hippocampus (Rehfeld et al., 2017).
Similar to chronic exercise, acute bouts of different exercise types have divergent effects on cognitive performance. Acute physical exercise with a high coordinative demand leads, in comparison to a purely aerobic exercise, to higher scores in attention tests (Budde et al., 2008), in working memory tests (Koutsandreou et al., 2016; Zach and Shalom, 2016), and in cognitive flexibility measures (Benzing et al., 2016). Taken together, acute and chronic physical exercises with high cognitive (coordinative) demands enhance cognitive performance. The beneficial effect of cognitively demanding exercises supports the recommendation of Pesce (2012) who proposes to shift the focus of exercise–cognition research from quantitative (e.g., exercise duration or intensity) to qualitative aspects (e.g., type of exercise).
Remarkably, it has been speculated that the combination of physical and cognitive exercise (also known as motor-cognitive training) could evoke a higher cognitive enhancement than cognitive or physical exercise alone (Kraft, 2012; Fissler et al., 2013; Bamidis et al., 2014; Lauenroth et al., 2016; Levin et al., 2017). The reasons why combined physical and cognitive exercise may be more effective than physical or cognitive training alone as well as the underlying neurophysiological mechanism are elucidated in the next section.
Neurophysiological Mechanisms of Motor-Cognitive Training
To understand the beneficial effects of combined physical and cognitive exercises, it is advisable to consider our evolutionary past. From an evolutionary perspective, the human body and its organic systems exhibit a high adaptability to environmental constraints (Raichlen and Alexander, 2017). Moreover, over centuries the human physiology was adapted to an active lifestyle which ensured human survival, for example, through foraging, hunting, and fishing (Raichlen and Alexander, 2017). In industrialized nations, an increasing sedentary lifestyle (Owen et al., 2010; Church et al., 2011) probably promotes negative adaptations which minimize energy consumption, but also cause a decline of the motor and cognitive systems (Raichlen and Alexander, 2017). In contrast, the foraging activities (e.g., hunting) of our ancestors required a simultaneous use of cognitive and sensori-motor resources (e.g., walking and simultaneously scanning the environment for food) (Raichlen and Alexander, 2017). Such cognitive processes (e.g., detecting a deer in the landscape) were intertwined with related actions of the body (e.g., throwing a spear on a moving deer). The idea expressing that cognitive processes, bodily movements and interaction with the environment are interrelated is close to those theories known as “embodied cognition” and “embedded cognition” (Wilson, 2002; Pouw et al., 2014a,b). These considerations suggest that the combination of physical and cognitive challenges are essential to preserve or enhance the neural capacity which, in turn, ensures that cognitive processes function well (Kempermann et al., 2010; Fissler et al., 2013; Bamidis et al., 2014; Raichlen and Alexander, 2017).
According to the “guided plasticity facilitation” framework (see Figure 1), the combination of physical and cognitive activities has positive synergistic effects that exceed the pure addition of the positive effects of cognitive and physical exercises (Kraft, 2012; Fissler et al., 2013). These super additive synergistic effects emerge from (I) the “facilitation effects” of physical exercises and (II) the “guidance effects” of cognitive exercises (see Figure 1). The “facilitation effect” of physical exercises triggers neurophysiological mechanisms, which promote neuroplasticity (Fissler et al., 2013). A possible physical exercise-induced mechanism, which promotes neuroplasticity is the enhanced release of neurotrophic factors such as the brain-derived neurotrophic factor (BDNF) (Knaepen et al., 2010; Coelho et al., 2013; Szuhany et al., 2015; Assis and Almondes, 2017; Dinoff et al., 2017). BDNF is associated with synaptogenesis and neurogenesis which may foster improved cognition (Cotman et al., 2007; Lu et al., 2013; Brigadski and Leßmann, 2014; Borror, 2017). Notably, an increased level of BDNF was observed during physical exercises and up to 60 min after cessation of the acute bout of physical exercises (Knaepen et al., 2010; Piepmeier and Etnier, 2015; Dinoff et al., 2017). Based on this observation, the “facilitation effects” of acute physical exercises seem transient and time-constrained (Fissler et al., 2013). While physical exercises induce neurophysiological processes that are fundamental for transient neuroplasticity (e.g., neurogenesis (Geibig et al., 2012), cognitive stimulation is assumed to “guide” these neuroplastic processes (Fissler et al., 2013; Bamidis et al., 2014). The “guidance effects” of cognitive exercises probably initiate distinct survival mechanisms of newborn cells (Fabel et al., 2009). These survival mechanisms are presumably part of a complex, multi-step mechanism that depends on the activation/stimulation of the newly generated synapses or neurons. The activation/stimulation of synapses and neurons occurs due to the execution of cognitive tasks and enables the functional integration of new neuronal structures in the respective brain circuits (Trachtenberg et al., 2002; Bruel-Jungerman et al., 2007; Bergami and Berninger, 2012; Bamidis et al., 2014). The integration in functional brain circuits seems to be crucial in order to stabilize the (by motor-cognitive training) induced neuroplastic changes. In addition to neuroplasticity, the stabilization of central nervous structures is likewise important to ensure good brain function (Kasai et al., 2003; Koleske, 2013).
Types of Motor-Cognitive Training
As shown in the framework in Figure 2, motor and cognitive exercises can be combined in several ways. In the first stage, we categorize motor and cognitive exercises based on their temporal order, which is a key factor for the effectiveness of the intervention (Roig et al., 2012; Fissler et al., 2013; Frith et al., 2017) in (I) sequential (or subsequent) motor-cognitive training and (II) simultaneous motor-cognitive training (see Figure 2).
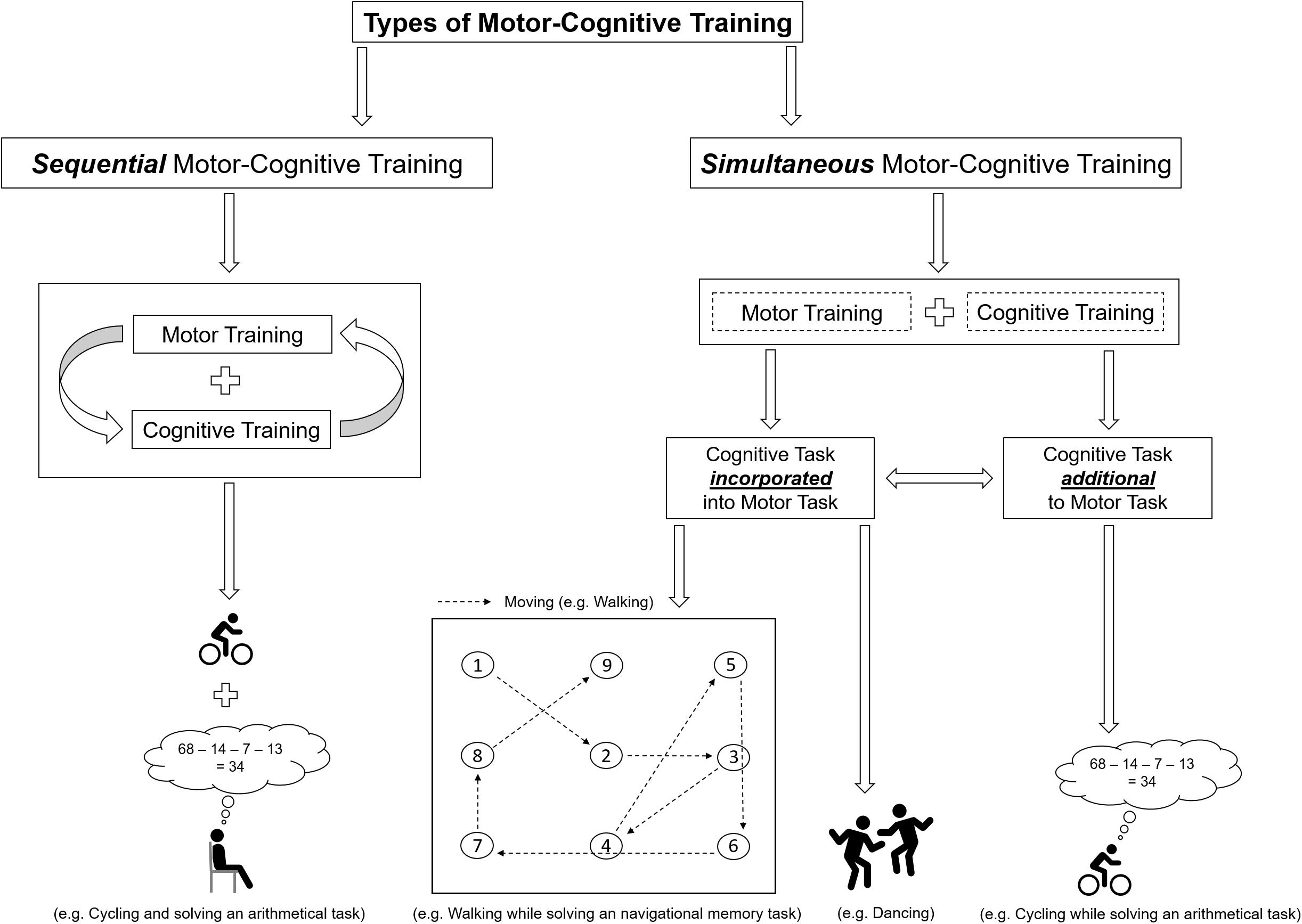
FIGURE 2. Schematic illustration of the classification of motor-cognitive training and a exemplifying illustrations of the differences between “additional” and “incorporated” cognitive training.
Sequential Motor-Cognitive Training
In sequential (or subsequent) motor-cognitive training, both the motor training and the cognitive training are each conducted at separate time points on the same day (prior to or after a bout of physical exercises) or at separate days (Tait et al., 2017). Commonly, the motor component of simultaneous motor-cognitive exercises compromises aerobic, resistance, balance, flexibility training, or a combination of them (for review see Lauenroth et al., 2016; Tait et al., 2017). In sequential motor-cognitive training interventions, the cognitive training was mainly focused on attention, memory, or multiple cognitive domains (for review see Lauenroth et al., 2016; Tait et al., 2017). An advantage of sequential motor-cognitive training (compared to simultaneous motor-cognitive training) is the absence of (1) possible dual-task costs [performance decrements in the motor task, the cognitive task, or in both tasks that may occur when two tasks are solved simultaneously (Hamacher et al., 2017)] and (2) prioritization effects (given a priority either to the motor task or the cognitive task), which vary individually and which might influence the results of the intervention. However, drawbacks of a sequential motor-cognitive training are the unknown appropriate load characteristics (frequency, length, duration, type of exercise, and the temporal order of the cognitive intervention and the motor intervention) for favorable cognitive outcomes (Lauenroth et al., 2016). For instance, the best benefit of an acute bout of motor exercises on cognitive performance was observed 11–20 min after cessation of the motor exercises (Chang et al., 2012). In contrast, another study reported that performing motor exercises 4 h after learning resulted in higher cognitive performance as compared to learning immediately afterward (van Dongen et al., 2016). Hence, it remains unclear whether cognitive training should be performed prior to or after motor exercises.
Interestingly, a recent review that compared the effects of sequential with simultaneous motor-cognitive training, reported that the simultaneous training significantly improved cognitive performance in various populations, whereas the sequential training yield inconclusive results (Tait et al., 2017). The latter finding may be explained by the fact that up to day it is unknown what time interval between motor and cognitive exercises is optimal (see Figure 1). Different time intervals may activate different neurobiological pathways (Tait et al., 2017). Based on these findings, simultaneous motor-cognitive training seems a more promising and time-efficient approach to foster cognitive functions than sequential training regimens.
Simultaneous Motor-Cognitive Training
Simultaneous motor-cognitive training or motor-cognitive dual-task training is defined as training where both motor training and cognitive training are performed at the same time (Lauenroth et al., 2016). Simultaneous motor-cognitive training can be further classified regarding the supposed demands of the cognitive task (see Figure 2). We suggest to differentiate the two types of the supposed demands of cognitive tasks in simultaneous motor-cognitive training, which are presented in the following.
Motor-Cognitive Training With Additional Cognitive Tasks
A motor-cognitive training with additional cognitive task is similar to the “classical” dual-task approaches where, the secondary cognitive task is typically used as a distractor of the motor task (Schott, 2015). Distractor means that the additional cognitive task is not a relevant prerequisite to successfully complete the motor-cognitive task (task nonrelevant; e.g., walking and solving an arithmetic task or stationary cycling while citing alternate letters) and can be described as Thinking while Moving.
Motor-Cognitive Training With Incorporated Cognitive Tasks
When the cognitive task is “incorporated” into the motor task, the cognitive task is a relevant prerequisite to successfully solve the motor-cognitive task (e.g., walking to certain cones in a predefined order or dancing; see Figure 2) (Schott, 2015). Hence, this form of motor cognitive training can be described as Moving while Thinking.
We argue that incorporating the cognitive task(s) into the motor task(s) is more beneficial in terms of stabilizing neuroplasticity effects than using the cognition task as a distractor. Below, we will outline several reasons why this might be the case:
(1) Several studies suggest that the incorporation of a cognitive task into the motor task combines the advantageous effects of cognitive and motor training and leads to greater (motor-) cognitive improvements (Paas et al., 2003; Paas and Sweller, 2012; Moreau and Conway, 2013; Moreau, 2015; Ruiter et al., 2015). For example, it was observed that the integration of the cognitive task into physical activity enhanced cognitive learning in children more than performing physical activity unrelated to the cognitive task (Toumpaniari et al., 2015; Mavilidi et al., 2016, 2017, 2018).
(2) A training form that incorporates the cognitive task(s) into the motor task(s) is closer to daily life situations. For example, it is unlikely that an older person habitually solves an arithmetic task during walking, but it is likely that he/she walks through the supermarket while remembering what goods to buy and where to find those.
(2a) Exercise benefits are moderated by expectations (beliefs toward the effectiveness of the intervention) and preferences (Crum and Langer, 2007; Helfer et al., 2015). Given that even neurophysiological parameters are affected by individual preferences and expectations regarding the exercise intervention (Crabbe and Dishman, 2004; Schneider et al., 2009; Mothes et al., 2016), it can be assumed that the exercise-induced neural adaption processes and, in turn, the cognitive outcomes are influenced by those factors, too. For example, for an intervention to be effective, the adherence rate (compliance) to the training must be high. The latter is largely influenced by the subjectively experienced meaningfulness (perceived importance of the intervention with respect to individual situation) of the training (Trombly, 1995; Carlson et al., 2008; Fissler et al., 2013; Lautenschlager and Cox, 2013). As a cognitive task that is incorporated into a motor task closely resembles real-life situations, it is likely that such a combination is regarded as meaningful and, therefore, results in a high degree of adherence and in training success.
(2b) Older adults (Park et al., 2002) and persons with dementia (Pal et al., 2016), for instance, exhibit impaired visuospatial abilities (e.g., remembering the location of a certain product in the supermarket). As physical fitness effectively enhances cognitive functions (Bherer et al., 2013; Carvalho et al., 2014; Groot et al., 2016), an intervention, that targets the deficit in visuospatial memory, should, in our opinion, not only include a training of cognitive functions but also one of physical fitness. Moreover, given that the adaptions of the brain are task-specific (Dahlin et al., 2008; Green and Bavelier, 2012; Schlaffke et al., 2014), we propose that the ideal (motor-) cognitive training should also be specific (e.g., related to spatial memory) and should induce substantial transfer effects to everyday cognitive functions (e.g., remembering shopping list) as well as to activities of daily living (e.g., walking) (Jobe et al., 2001; Zelinski, 2009). These considerations suggest that the cognitive task should be incorporated into the motor task. In the context of our supermarket example remembering and walking to the respective goods can be combined. This approach is schematically depicted in Figure 2.
(3) If the cognitive task is incorporated into the motor task, no prioritization effects (which can be observed in motor-cognitive tasks with an additional cognitive task) would occur (Yogev-Seligmann et al., 2012a; Plummer et al., 2013, 2014; Plummer and Eskes, 2015). Such prioritization effects (giving priority either to the cognitive or the motor task) are known to influence motor and cognitive performance (Yogev-Seligmann et al., 2012b; Plummer et al., 2015a,b) as well as the activation of prefrontal structures (Lague-Beauvais et al., 2015). Theoretically, such prioritization-related effects could evoke distinct (undesired) adaptation processes.
(4) A further advantage of motor-cognitive training with incorporated cognitive tasks could be that multiple sensory systems are stimulated (due to the execution and control of the cognitive task and the motor task at the same point of time). Also, it is assumed that multisensory training environments better approximate all-day settings (which are ecologically more valid) and therefore provide an optimal basis for cognitive processes such as learning (Shams and Seitz, 2008).
Apart from a real-world setting as outlined for the proposed supermarket task, virtual reality environments offer the opportunity to combine cognitive and motor tasks without the need to construct a complex scenery (Tarr and Warren, 2002; Bruin et al., 2010). Respective approaches are the so-called “exergames” (Skjæret et al., 2016; Boissieu et al., 2017). Those video games, that are also a type of physical exercise task, can be classified within our proposed framework as simultaneous motor-cognitive training because they pose motor and cognitive demands simultaneously (Pichierri et al., 2012; Monteiro-Junior et al., 2016). However, as expectations always prove the rule, motor-cognitive tasks with an additional cognitive task are sometimes also common in a modern world [e.g., walking while texting or phoning (Hamacher et al., 2016)]. Nevertheless, we argue that this circumstance does not challenge our proposed framework; however, one would have to adapt the training characteristics if the aim is to improve the use of a smartphone while walking.
Suggestions for Further Research Directions
We conclude that further exercise-cognition research is strongly needed, and we recommend that the major goals of this research should be:
(I) The identification of optimal qualitative (e.g., type of exercise) and quantitative training characteristics (e.g., frequency, duration, intensity, and temporal proximity of cognitive and motor training), which effectively and sustainably enhance the individual cognitive reserve and show transfer to daily life activities. Thereto, future research should consider the concept of “personalized training (or personalized medicine, if exercise is seen as a “medicament”), which aims to understand the heterogeneity in (motor-cognitive) exercise responsiveness (Buford and Pahor, 2012; Buford et al., 2013; Barha et al., 2017). “Personalized training” goes behind the “one-size-fits-all approach,” and the overall goal is to deliver tailored exercise prescriptions for the individual subject. Hence, participating subjects in future studies should be intensively screened and stratified into subgroups based on measures of physical fitness (e.g., cardiorespiratory fitness level or physical activity level assessed by questionnaires); motor fitness (e.g., coordinative abilities such as fine motor skills or hand/foot tapping speed); cognitive fitness (e.g., level of individual working memory performance); age, gender health status, and socioemotional status (e.g., measures of motivation, mood or stress). Furthermore, other potential moderators such as genotypic factors should be assessed if possible (Buford and Pahor, 2012; Barha et al., 2017). In order to achieve a “personalized training,” the programming of (motor-cognitive) exercise training should not solely be based on external training load characteristics (work performed by the subject, e.g., 70 % of maximal oxygen uptake) but also on internal training load characteristics (physiological and/or psychological effects in response to the performed task; e.g., individual level of blood lactate) (Bourdon et al., 2017; Burgess, 2017), because the same external training loads could lead to varying internal training loads (e.g., metabolic response such as blood lactate level) (Meyer et al., 1999; Scharhag-Rosenberger et al., 2010). For more detailed information on exercise prescriptions, we refer to the articles of Hofmann and Tschakert (2010), Mann et al. (2013), Bourdon et al. (2017), Burgess (2017), and Vanrenterghem et al. (2017).
(II) In order to prove whether the incorporation of cognitive tasks into acute or chronic exercise interventions (as described, for example, in Figure 2) is more effective regarding the enhancement of cognitive performance than (I) a sole motor training, (II) a sole cognitive training, (III) a sequential motor-cognitive training, or (IV) simultaneous motor-cognitive training with a non-task-relevant secondary cognitive task and in order to verify (or identify further) underlying, causal mechanisms, future work should elaborate on detailed modeling of neurobehavioral effects of (motor-cognitive) exercise interventions. Since physical exercises influence cognitive performance on multiple levels (Stillman et al., 2016) and also trough diverse short- and long-term mechanisms (Stimpson et al., 2018), different behavioral proxies (e.g., neuropsychological clinical tests and/or gait analysis), neuroimaging modalities (e.g., functional magnetic resonance imaging and/or functional near-infrared spectroscopy) as well as molecular and cellular measures (e.g., BDNF and/or lactate) should be used to validate such a neurobehavioral model.
Author Contributions
FH and DH wrote the manuscript. LS and NM reviewed the drafted versions. All authors have read and approved the final version.
Conflict of Interest Statement
The authors declare that the research was conducted in the absence of any commercial or financial relationships that could be construed as a potential conflict of interest.
References
Ahlskog, J. E. (2011). Does vigorous exercise have a neuroprotective effect in Parkinson disease? Neurology 77, 288–294. doi: 10.1212/WNL.0b013e318225ab66
Ahn, S., Salisbury, D., and Yu, F. (2017). Effects of physical exercise on cognition in persons with subjective cognitive decline or mild cognitive impairment: a review. J. Parkinsons Dis. Alzheimers Dis. 4, 1–11. doi: 10.13188/2376-922X.1000023
Ainsworth, B. E., Haskell, W. L., Whitt, M. C., Irwin, M. L., Swartz, A. M., Strath, S. J., et al. (2000). Compendium of physical activities: an update of activity codes and MET intensities. Med. Sci. Sports Exerc. 32, S498–S504. doi: 10.1097/00005768-200009001-00009
Albert, M. S. (1997). The ageing brain: normal and abnormal memory. Philos. Trans. R. Soc. Lond. B Biol. Sci. 352, 1703–1709. doi: 10.1098/rstb.1997.0152
Alves, C. R., Gualano, B., Takao, P. P., Avakian, P., Fernandes, R. M., Morine, D., et al. (2012). Effects of acute physical exercise on executive functions: a comparison between aerobic and strength exercise. J. Sport Exerc. Psychol. 34, 539–549. doi: 10.1123/jsep.34.4.539
Andersen, C. K., Wittrup-Jensen, K. U., Lolk, A., Andersen, K., and Kragh-Sorensen, P. (2004). Ability to perform activities of daily living is the main factor affecting quality of life in patients with dementia. Health Qual. Life Outcomes 2:52. doi: 10.1186/1477-7525-2-52
Assis, G. G., and Almondes, K. M. (2017). Exercise-dependent BDNF as a modulatory factor for the executive processing of individuals in course of cognitive decline. A systematic review. Front. Psychol. 8:584. doi: 10.3389/fpsyg.2017.00584
Bamidis, P. D., Vivas, A. B., Styliadis, C., Frantzidis, C., Klados, M., Schlee, W., et al. (2014). A review of physical and cognitive interventions in aging. Neurosci. Biobehav. Rev. 44, 206–220. doi: 10.1016/j.neubiorev.2014.03.019
Barha, C. K., Galea, L. A., Nagamatsu, L. S., Erickson, K. I., and Liu-Ambrose, T. (2017). Personalising exercise recommendations for brain health: considerations and future directions. Br. J. Sports Med. 51, 636–639. doi: 10.1136/bjsports-2016-096710
Batouli, S. A., and Saba, V. (2017). At least eighty percent of brain grey matter is modifiable by physical activity: a review study. Behav. Brain Res. 332, 204–217. doi: 10.1016/j.bbr.2017.06.002
Benzing, V., Heinks, T., Eggenberger, N., and Schmidt, M. (2016). Acute cognitively engaging exergame-based physical activity enhances executive functions in adolescents. PLoS One 11:e0167501. doi: 10.1371/journal.pone.0167501
Bergami, M., and Berninger, B. (2012). A fight for survival: the challenges faced by a newborn neuron integrating in the adult hippocampus. Dev. Neurobiol. 72, 1016–1031. doi: 10.1002/dneu.22025
Bherer, L., Erickson, K. I., and Liu-Ambrose, T. (2013). A review of the effects of physical activity and exercise on cognitive and brain functions in older adults. J. Aging Res. 2013:657508. doi: 10.1155/2013/657508
Blondell, S. J., Hammersley-Mather, R., and Veerman, J. L. (2014). Does physical activity prevent cognitive decline and dementia? A systematic review and meta-analysis of longitudinal studies. BMC Public Health 14:510. doi: 10.1186/1471-2458-14-510
Boissieu, P. de, Denormandie, P., Armaingaud, D., Sanchez, S., and Jeandel, C. (2017). Exergames and elderly: a non-systematic review of the literature. Eur. Geriatr. Med. 8, 111–116. doi: 10.1016/j.eurger.2017.02.003
Borror, A. (2017). Brain-derived neurotrophic factor mediates cognitive improvements following acute exercise. Med. Hypotheses 106, 1–5. doi: 10.1016/j.mehy.2017.06.024
Borson, S. (2010). Cognition, aging, and disabilities: conceptual issues. Phys. Med. Rehabil. Clin. N. Am. 21, 375–382. doi: 10.1016/j.pmr.2010.01.001
Bostrom, N., and Sandberg, A. (2009). Cognitive enhancement: methods, ethics, regulatory challenges. Sci. Eng. Ethics 15, 311–341. doi: 10.1007/s11948-009-9142-5
Bourdon, P. C., Cardinale, M., Murray, A., Gastin, P., Kellmann, M., Varley, M. C., et al. (2017). Monitoring athlete training loads: consensus statement. Int. J. Sports Physiol. Perform. 12, S2161–S2170 doi: 10.1123/IJSPP.2017-0208
Brigadski, T., and Leßmann, V. (2014). BDNF: a regulator of learning and memory processes with clinical potential. e-Neuroforum 5, 1–11. doi: 10.1007/s13295-014-0053-9
Bruel-Jungerman, E., Rampon, C., and Laroche, S. (2007). Adult hippocampal neurogenesis, synaptic plasticity and memory: facts and hypotheses. Rev. Neurosci. 18, 93–114. doi: 10.1515/REVNEURO.2007.18.2.93
Bruin, E. D. de, Schoene, D., Pichierri, G., and Smith, S. T. (2010). Use of virtual reality technique for the training of motor control in the elderly. Some theoretical considerations. Z. Gerontol. Geriatr. 43, 229–234. doi: 10.1007/s00391-010-0124-7
Buckner, R. L. (2004). Memory and executive function in aging and AD: multiple factors that cause decline and reserve factors that compensate. Neuron 44, 195–208. doi: 10.1016/j.neuron.2004.09.006
Budde, H., Schwarz, R., Velasques, B., Ribeiro, P., Holzweg, M., Machado, S., et al. (2016). The need for differentiating between exercise, physical activity, and training. Autoimmun. Rev. 15, 110–111. doi: 10.1016/j.autrev.2015.09.004
Budde, H., Voelcker-Rehage, C., Pietrabyk-Kendziorra, S., Ribeiro, P., and Tidow, G. (2008). Acute coordinative exercise improves attentional performance in adolescents. Neurosci. Lett. 441, 219–223. doi: 10.1016/j.neulet.2008.06.024
Buford, T. W., and Pahor, M. (2012). Making preventive medicine more personalized: implications for exercise-related research. Prev. Med. 55, 34–36. doi: 10.1016/j.ypmed.2012.05.001
Buford, T. W., Roberts, M. D., and Church, T. S. (2013). Toward exercise as personalized medicine. Sports Med. 43, 157–165. doi: 10.1007/s40279-013-0018-0
Burgess, D. J. (2017). The research doesn’t always apply: practical solutions to evidence-based training-load monitoring in elite team sports. Int. J. Sports Physiol. Perform. 12, S2136–S2141. doi: 10.1123/ijspp.2016-0608
Cai, Y., and Abrahamson, K. (2015). Does exercise impact cognitive performance research article op in community-dwelling older adults with mild cognitive impairment? A systematic review. Qual. Prim. Care 23, 214–222.
Carlson, M. C., Saczynski, J. S., Rebok, G. W., Seeman, T., Glass, T. A., McGill, S., et al. (2008). Exploring the effects of an “everyday” activity program on executive function and memory in older adults: experience Corps. Gerontologist 48, 793–801. doi: 10.1093/geront/48.6.793
Carvalho, A., Rea, I. M., Parimon, T., and Cusack, B. J. (2014). Physical activity and cognitive function in individuals over 60 years of age: a systematic review. Clin. Interv. Aging 9, 661–682. doi: 10.2147/CIA.S55520
Caspersen, C. J., Powell, K. E., and Christenson, G. M. (1985). Physical activity, exercise, and physical fitness: definitions and distinctions for health-related research. Public Health Rep. 100, 126–131.
Chaddock-Heyman, L., Hillman, C. H., Cohen, N. J., and Kramer, A. F. (2014). III. The importance of physical activity and aerobic fitness for cognitive control and memory in children. Monogr. Soc. Res. Child Dev. 79, 25–50. doi: 10.1111/mono.12129
Chang, Y. K., Labban, J. D., Gapin, J. I., and Etnier, J. L. (2012). The effects of acute exercise on cognitive performance: a meta-analysis. Brain Res. 1453, 87–101. doi: 10.1016/j.brainres.2012.02.068
Church, T. S., Thomas, D. M., Tudor-Locke, C., Katzmarzyk, P. T., Earnest, C. P., Rodarte, R. Q., et al. (2011). Trends over 5 decades in U.S. occupation-related physical activity and their associations with obesity. PLoS One 6:e19657. doi: 10.1371/journal.pone.0019657
Coelho, F G., de Melo, Gobbi, S., Andreatto, C. A. A., Corazza, D. I., Pedroso, R. V., and Santos-Galduroz, R. F. (2013). Physical exercise modulates peripheral levels of brain-derived neurotrophic factor (BDNF): a systematic review of experimental studies in the elderly. Arch. Gerontol. Geriatr. 56, 10–15. doi: 10.1016/j.archger.2012.06.003
Colcombe, S., and Kramer, A. F. (2003). Fitness effects on the cognitive function of older adults: a meta-analytic study. Psychol. Sci. 14, 125–130. doi: 10.1111/1467-9280.t01-1-01430
Cotman, C. W., Berchtold, N. C., and Christie, L. -A. (2007). Exercise builds brain health: key roles of growth factor cascades and inflammation. Trends Neurosci. 30, 464–472. doi: 10.1016/j.tins.2007.06.011
Coubard, O. A., Duretz, S., Lefebvre, V., Lapalus, P., and Ferrufino, L. (2011). Practice of contemporary dance improves cognitive flexibility in aging. Front. Aging Neurosci. 3:13. doi: 10.3389/fnagi.2011.00013
Cox, E. P., O’Dwyer, N., Cook, R., Vetter, M., Cheng, H. L., Rooney, K., et al. (2016). Relationship between physical activity and cognitive function in apparently healthy young to middle-aged adults: a systematic review. J. Sci. Med. Sport 19, 616–628. doi: 10.1016/j.jsams.2015.09.003
Crabbe, J. B., and Dishman, R. K. (2004). Brain electrocortical activity during and after exercise: a quantitative synthesis. Psychophysiology 41, 563–574. doi: 10.1111/j.1469-8986.2004.00176.x
Crum, A. J., and Langer, E. J. (2007). Mind-set matters: exercise and the placebo effect. Psychol. Sci. 18, 165–171. doi: 10.1111/j.1467-9280.2007.01867.x
Dahlin, E., Neely, A. S., Larsson, A., Bäckman, L., and Nyberg, L. (2008). Transfer of learning after updating training mediated by the striatum. Science 320, 1510–1512. doi: 10.1126/science.1155466
Dhami, P., Moreno, S., and DeSouza, J. F. (2014). New framework for rehabilitation - fusion of cognitive and physical rehabilitation: the hope for dancing. Front. Psychol. 5:1478. doi: 10.3389/fpsyg.2014.01478
Dinoff, A., Herrmann, N., Swardfager, W., and Lanctôt, K. L. (2017). The effect of acute exercise on blood concentrations of brain-derived neurotrophic factor in healthy adults: a meta-analysis. Eur. J. Neurosci. 46, 1635–1646. doi: 10.1111/ejn.13603
Erickson, K. I., Gildengers, A. G., and Butters, M. A. (2013). Physical activity and brain plasticity in late adulthood. Dialogues Clin. Neurosci. 15, 99–108.
Erickson, K. I., Voss, M. W., Prakash, R. S., Basak, C., Szabo, A., Chaddock, L., et al. (2011). Exercise training increases size of hippocampus and improves memory. Proc. Natl. Acad. Sci. U.S.A. 108, 3017–3022. doi: 10.1073/pnas.1015950108
Esteban-Cornejo, I., Tejero-Gonzalez, C. M., Sallis, J. F., and Veiga, O. L. (2015). Physical activity and cognition in adolescents: a systematic review. J. Sci. Med. Sport 18, 534–539. doi: 10.1016/j.jsams.2014.07.007
Fabel, K., Wolf, S. A., Ehninger, D., Babu, H., Leal-Galicia, P., and Kempermann, G. (2009). Additive effects of physical exercise and environmental enrichment on adult hippocampal neurogenesis in mice. Front. Neurosci. 3:50. doi: 10.3389/neuro.22.002.2009
Falck, R. S., Davis, J. C., and Liu-Ambrose, T. (2016). What is the association between sedentary behaviour and cognitive function? A systematic review. Br. J. Sports Med. 51, 800–811. doi: 10.1136/bjsports-2015-095551
Fiest, K. M., Jette, N., Roberts, J. I., Maxwell, C. J., Smith, E. E., Black, S. E., et al. (2016). The prevalence and incidence of dementia: a systematic review and meta-analysis. Can. J. Neurol. Sci. 43(Suppl. 1), S3–S50. doi: 10.1017/cjn.2016.18
Fissler, P., Küster, O., Schlee, W., and Kolassa, I. -T. (2013). Novelty interventions to enhance broad cognitive abilities and prevent dementia: synergistic approaches for the facilitation of positive plastic change. Prog. Brain Res. 207, 403–434. doi: 10.1016/B978-0-444-63327-9.00017-5
Fjell, A. M., and Walhovd, K. B. (2010). Structural brain changes in aging: courses, causes and cognitive consequences. Rev. Neurosci. 21, 187–221. doi: 10.1515/REVNEURO.2010.21.3.187
Frith, E., Sng, E., and Loprinzi, P. D. (2017). Randomized controlled trial evaluating the temporal effects of high-intensity exercise on learning, short-term and long-term memory, and prospective memory. Eur. J. Neurosci. 46, 2557–2564. doi: 10.1111/ejn.13719
Geibig, C. S., Keiner, S., and Redecker, C. (2012). Functional recruitment of newborn hippocampal neurons after experimental stroke. Neurobiol. Dis. 46, 431–439. doi: 10.1016/j.nbd.2012.02.007
Greeff, J. W. de, Bosker, R. J., Oosterlaan, J., Visscher, C., and Hartman, E. (2017). Effects of physical activity on executive functions, attention and academic performance in preadolescent children: a meta-analysis. J. Sci. Med. Sport 21, 501–507. doi: 10.1016/j.jsams.2017.09.595
Green, C. S., and Bavelier, D. (2012). Learning, attentional control, and action video games. Curr. Biol. 22, R197–R206. doi: 10.1016/j.cub.2012.02.012
Groot, C., Hooghiemstra, A. M., Raijmakers, P. H., van Berckel, B. N., Scheltens, P., Scherder, E. J., et al. (2016). The effect of physical activity on cognitive function in patients with dementia: a meta-analysis of randomized control trials. Ageing Res. Rev. 25, 13–23. doi: 10.1016/j.arr.2015.11.005
Hamacher, D., Brennicke, M., Behrendt, T., Alt, P., Törpel, A., and Schega, L. (2017). Motor-cognitive dual-tasking under hypoxia. Exp. Brain Res. 235, 2997–3001. doi: 10.1007/s00221-017-5036-y
Hamacher, D., Hamacher, D., Rehfeld, K., Hökelmann, A., and Schega, L. (2015a). The effect of a six-month dancing program on motor-cognitive dual-task performance in older adults. JAPA 23, 647–652. doi: 10.1123/japa.2014-0067
Hamacher, D., Hamacher, D., Rehfeld, K., and Schega, L. (2015b). Motor-cognitive dual-task training improves local dynamic stability of normal walking in older individuals. Clin. Biomech. 32, 138–141. doi: 10.1016/j.clinbiomech.2015.11.021
Hamacher, D., Hamacher, D., Törpel, A., Krowicki, M., Herold, F., and Schega, L. (2016). The reliability of local dynamic stability in walking while texting and performing an arithmetical problem. Gait Posture 44, 200–203. doi: 10.1016/j.gaitpost.2015.12.021
Hamacher, D., Herold, F., Wiegel, P., Hamacher, D., and Schega, L. (2015c). Brain activity during walking: a systematic review. Neurosci. Biobehav. Rev. 57, 310–327. doi: 10.1016/j.neubiorev.2015.08.002
Hashimoto, H., Takabatake, S., Miyaguchi, H., Nakanishi, H., and Naitou, Y. (2015). Effects of dance on motor functions, cognitive functions, and mental symptoms of Parkinson’s disease: a quasi-randomized pilot trial. Complement. Ther. Med 23, 210–219. doi: 10.1016/j.ctim.2015.01.010
Hedden, T., and Gabrieli, J. D. (2004). Insights into the ageing mind: a view from cognitive neuroscience. Nat. Rev. Neurosci. 5, 87–96. doi: 10.1038/nrn1323
Helfer, S. G., Elhai, J. D., and Geers, A. L. (2015). Affect and exercise: positive affective expectations can increase post-exercise mood and exercise intentions. Ann. Behav. Med. 49, 269–279. doi: 10.1007/s12160-014-9656-1
Heyn, P., Abreu, B. C., and Ottenbacher, K. J. (2004). The effects of exercise training on elderly persons with cognitive impairment and dementia: a meta-analysis11No commercial party having a direct financial interest in the results of the research supporting this article has or will confer a benefit upon the author(s) or upon any organization with which the author(s) is/are associated. Arch. Phys. Med. Rehabil. 85, 1694–1704. doi: 10.1016/j.apmr.2004.03.019
Hillman, C. H., Erickson, K. I., and Kramer, A. F. (2008). Be smart, exercise your heart: exercise effects on brain and cognition. Nat. Rev. Neurosci. 9, 58–65. doi: 10.1038/nrn2298
Hillman, C. H., and Schott, N. (2013). Der Zusammenhang von Fitness, kognitiver Leistungsfähigkeit und Gehirnzustand im Schulkindalter. Z. Sportpsychol. 20, 33–41. doi: 10.1026/1612-5010/a000085
Hofmann, P., and Tschakert, G. (2010). Special needs to prescribe exercise intensity for scientific studies. Cardiol. Res. Pract. 2011:209302. doi: 10.4061/2011/209302
Hötting, K., and Röder, B. (2013). Beneficial effects of physical exercise on neuroplasticity and cognition. Neurosci. Biobehav. Rev. 37, 2243–2257. doi: 10.1016/j.neubiorev.2013.04.005
Howley, E. T. (2001). Type of activity: resistance, aerobic and leisure versus occupational physical activity. Med. Sci. Sports Exerc. 33, S364–S369. doi: 10.1097/00005768-200106001-00005
Hurd, M. D., Martorell, P., Delavande, A., Mullen, K. J., and Langa, K. M. (2013). Monetary costs of dementia in the United States. N. Engl. J. Med. 368, 1326–1334. doi: 10.1056/NEJMsa1204629
Janssen, I. (2012). Health care costs of physical inactivity in Canadian adults. Appl. Physiol. Nutr. Metab. 37, 803–806. doi: 10.1139/h2012-061
Jobe, J. B., Smith, D. M., Ball, K., Tennstedt, S. L., Marsiske, M., Willis, S. L., et al. (2001). Active: a cognitive intervention trial to promote independence in older adults. Control. Clin. Trials 22, 453–479. doi: 10.1016/S0197-2456(01)00139-8
Kasai, H., Matsuzaki, M., Noguchi, J., Yasumatsu, N., and Nakahara, H. (2003). Structure–stability–function relationships of dendritic spines. Trends Neurosci. 26, 360–368. doi: 10.1016/S0166-2236(03)00162-0
Kempermann, G., Fabel, K., Ehninger, D., Babu, H., Leal-Galicia, P., Garthe, A., et al. (2010). Why and how physical activity promotes experience-induced brain plasticity. Front. Neurosci. 4:189. doi: 10.3389/fnins.2010.00189
Khan, N. A., and Hillman, C. H. (2014). The relation of childhood physical activity and aerobic fitness to brain function and cognition: a review. Pediatr. Exerc. Sci. 26, 138–146. doi: 10.1123/pes.2013-0125
Knaepen, K., Goekint, M., Heyman, E. M., and Meeusen, R. (2010). Neuroplasticity - exercise-induced response of peripheral brain-derived neurotrophic factor: a systematic review of experimental studies in human subjects. Sports Med. 40, 765–801. doi: 10.2165/11534530-000000000-00000
Koleske, A. J. (2013). Molecular mechanisms of dendrite stability. Nat. Rev. Neurosci. 14, 536–550. doi: 10.1038/nrn3486
Koutsandreou, F., Wegner, M., Niemann, C., and Budde, H. (2016). Effects of motor versus cardiovascular exercise training on children’s working memory. Med. Sci. Sports Exerc. 48, 1144–1152. doi: 10.1249/MSS.0000000000000869
Kraft, E. (2012). Cognitive function, physical activity, and aging: possible biological links and implications for multimodal interventions. Neuropsychol. Dev. Cogn. B Aging Neuropsychol. Cogn. 19, 248–263. doi: 10.1080/13825585.2011.645010
Ku, P. -W., Liu, Y. -T., Lo, M. -K., Chen, L. -J., and Stubbs, B. (2017). Higher levels of objectively measured sedentary behavior is associated with worse cognitive ability: two-year follow-up study in community-dwelling older adults. Exp. Gerontol. 99, 110–114. doi: 10.1016/j.exger.2017.09.014
Lague-Beauvais, M., Fraser, S. A., Desjardins-Crepeau, L., Castonguay, N., Desjardins, M., Lesage, F., et al. (2015). Shedding light on the effect of priority instructions during dual-task performance in younger and older adults: a fNIRS study. Brain Cogn. 98, 1–14. doi: 10.1016/j.bandc.2015.05.001
Lauenroth, A., Ioannidis, A. E., and Teichmann, B. (2016). Influence of combined physical and cognitive training on cognition: a systematic review. BMC Geriatr. 16:141. doi: 10.1186/s12877-016-0315-1
Lautenschlager, N. T., and Cox, K. L. (2013). Can participation in mental and physical activity protect cognition in old age? Comment on “The Mental Activity and eXercise (MAX) trial: a randomized controlled trial to enhance cognitive function in older adults”. JAMA Intern. Med. 173, 805–806. doi: 10.1001/jamainternmed.2013.206
Levin, O., Netz, Y., and Ziv, G. (2017). The beneficial effects of different types of exercise interventions on motor and cognitive functions in older age: a systematic review. Eur. Rev. Aging Phys. Act. 14:20. doi: 10.1186/s11556-017-0189-z
Li, J. W., O’Connor, H., O’Dwyer, N., and Orr, R. (2017). The effect of acute and chronic exercise on cognitive function and academic performance in adolescents: a systematic review. J. Sci. Med. Sport 20, 841–848. doi: 10.1016/j.jsams.2016.11.025
Lu, B., Nagappan, G., Guan, X., Nathan, P. J., and Wren, P. (2013). BDNF-based synaptic repair as a disease-modifying strategy for neurodegenerative diseases. Nat. Rev. Neurosci. 14, 401–416. doi: 10.1038/nrn3505
Ludyga, S., Gerber, M., Brand, S., Holsboer-Trachsler, E., and Puhse, U. (2016). Acute effects of moderate aerobic exercise on specific aspects of executive function in different age and fitness groups: a meta-analysis. Psychophysiology 53, 1611–1626. doi: 10.1111/psyp.12736
Lustig, C., Shah, P., Seidler, R., and Reuter-Lorenz, P. A. (2009). Aging, training, and the brain: a review and future directions. Neuropsychol. Rev. 19, 504–522. doi: 10.1007/s11065-009-9119-9
Mann, T., Lamberts, R. P., and Lambert, M. I. (2013). Methods of prescribing relative exercise intensity: physiological and practical considerations. Sports Med. 43, 613–625. doi: 10.1007/s40279-013-0045-x
Mansoubi, M., Pearson, N., Clemes, S. A., Biddle, S. J., Bodicoat, D. H., Tolfrey, K., et al. (2015). Energy expenditure during common sitting and standing tasks: examining the 1.5 MET definition of sedentary behaviour. BMC Public Health 15:516. doi: 10.1186/s12889-015-1851-x
Marchetti, R., Forte, R., Borzacchini, M., Vazou, S., Tomporowski, P. D., and Pesce, C. (2015). Physical and motor fitness, sport skills and executive function in adolescents: a moderated prediction model. Psychology 6, 1915–1929. doi: 10.4236/psych.2015.614189
Mavilidi, M. -F., Okely, A., Chandler, P., Louise Domazet, S., and Paas, F. (2018). Immediate and delayed effects of integrating physical activity into preschool children’s learning of numeracy skills. J. Exp. Child Psychol. 166, 502–519. doi: 10.1016/j.jecp.2017.09.009
Mavilidi, M. -F., Okely, A. D., Chandler, P., and Paas, F. (2016). Infusing physical activities into the classroom: effects on preschool children’s geography learning. Mind Brain Educ. 10, 256–263. doi: 10.1111/mbe.12131
Mavilidi, M. -F., Okely, A. D., Chandler, P., and Paas, F. (2017). Effects of integrating physical activities into a science lesson on preschool children’s learning and enjoyment. Appl. Cogn. Psychol. 31, 281–290. doi: 10.1002/acp.3325
Meyer, T., Gabriel, H. H., and Kindermann, W. (1999). Is determination of exercise intensities as percentages of VO2max or HRmax adequate? Med. Sci. Sports Exerc. 31, 1342–1345. doi: 10.1097/00005768-199909000-00017
Monteiro-Junior, R. S., Vaghetti, C. A. O., Nascimento, O. J. M., Laks, J., and Deslandes, A. C. (2016). Exergames: neuroplastic hypothesis about cognitive improvement and biological effects on physical function of institutionalized older persons. Neural Regen. Res. 11, 201–204. doi: 10.4103/1673-5374.177709
Moreau, D. (2015). Brains and brawn: complex motor activities to maximize cognitive enhancement. Educ. Psychol. Rev. 27, 475–482. doi: 10.1007/s10648-015-9323-5
Moreau, D., and Conway, A. R. A. (2013). Cognitive enhancement: a comparative review of computerized and athletic training programs. Int. Rev. Sport Exerc. Psychol. 6, 155–183. doi: 10.1080/1750984X.2012.758763
Mothes, H., Leukel, C., Jo, H. -G., Seelig, H., Schmidt, S., and Fuchs, R. (2016). Expectations affect psychological and neurophysiological benefits even after a single bout of exercise. J. Behav. Med. 40, 293–306. doi: 10.1007/s10865-016-9781-3
Müller, P., Rehfeld, K., Lüders, A., Schmicker, M., Hökelmann, A., Kaufman, J., et al. (2016). Effekte eines Tanz- und eines Gesundheitssporttrainings auf die graue Hirnsubstanz gesunder Senioren. Sportwissenschaft 46, 213–222. doi: 10.1007/s12662-016-0411-6
Müller, P., Rehfeld, K., Schmicker, M., Hökelmann, A., Dordevic, M., Lessmann, V., et al. (2017). Evolution of neuroplasticity in response to physical activity in old age: the case for dancing. Front. Aging Neurosci. 9:56. doi: 10.3389/fnagi.2017.00056
Nithianantharajah, J., and Hannan, A. J. (2009). The neurobiology of brain and cognitive reserve: mental and physical activity as modulators of brain disorders. Prog. Neurobiol. 89, 369–382. doi: 10.1016/j.pneurobio.2009.10.001
Northey, J. M., Cherbuin, N., Pumpa, K. L., Smee, D. J., and Rattray, B. (2017). Exercise interventions for cognitive function in adults older than 50: a systematic review with meta-analysis. Br. J. Sports Med. 52, 154–160. doi: 10.1136/bjsports-2016-096587
Owen, N., Sparling, P. B., Healy, G. N., Dunstan, D. W., and Matthews, C. E. (2010). Sedentary behavior: emerging evidence for a new health risk. Mayo Clin. Proc. 85, 1138–1141. doi: 10.4065/mcp.2010.0444
Paas, F., Renkl, A., and Sweller, J. (2003). Cognitive load theory and instructional design: recent developments. Educ. Psychol. 38, 1–4. doi: 10.1207/S15326985EP3801_1
Paas, F., and Sweller, J. (2012). An evolutionary upgrade of cognitive load theory: using the human motor system and collaboration to support the learning of complex cognitive tasks. Educ. Psychol. Rev. 24, 27–45. doi: 10.1007/s10648-011-9179-2
Paillard, T. (2015). Preventive effects of regular physical exercise against cognitive decline and the risk of dementia with age advancement. Sports Med. Open 1:20. doi: 10.1186/s40798-015-0016-x
Pal, A., Biswas, A., Pandit, A., Roy, A., Guin, D., Gangopadhyay, G., et al. (2016). Study of visuospatial skill in patients with dementia. Ann. Indian Acad. Neurol. 19, 83–88. doi: 10.4103/0972-2327.168636
Park, D. C., Lautenschlager, G., Hedden, T., Davidson, N. S., Smith, A. D., and Smith, P. K. (2002). Models of visuospatial and verbal memory across the adult life span. Psychol. Aging 17, 299–320. doi: 10.1037/0882-7974.17.2.299
Peeters, G. M., Mishra, G. D., Dobson, A. J., and Brown, W. J. (2014). Health care costs associated with prolonged sitting and inactivity. Am. J. Prev. Med. 46, 265–272. doi: 10.1016/j.amepre.2013.11.014
Pesce, C. (2012). Shifting the focus from quantitative to qualitative exercise characteristics in exercise and cognition research. J. Sport Exerc. Psychol. 34, 766–786. doi: 10.1123/jsep.34.6.766
Pichierri, G., Murer, K., and Bruin, E. D. de (2012). A cognitive-motor intervention using a dance video game to enhance foot placement accuracy and gait under dual task conditions in older adults: a randomized controlled trial. BMC Geriatr. 12:74. doi: 10.1186/1471-2318-12-74
Piepmeier, A. T., and Etnier, J. L. (2015). Brain-derived neurotrophic factor (BDNF) as a potential mechanism of the effects of acute exercise on cognitive performance. J. Sport Health Sci. 4, 14–23. doi: 10.1016/j.jshs.2014.11.001
Plummer, P., Apple, S., Dowd, C., and Keith, E. (2015a). Texting and walking: effect of environmental setting and task prioritization on dual-task interference in healthy young adults. Gait Posture 41, 46–51. doi: 10.1016/j.gaitpost.2014.08.007
Plummer, P., and Eskes, G. (2015). Measuring treatment effects on dual-task performance: a framework for research and clinical practice. Front. Hum. Neurosci. 9:225. doi: 10.3389/fnhum.2015.00225
Plummer, P., Eskes, G., Wallace, S., Giuffrida, C., Fraas, M., Campbell, G., et al. (2013). Cognitive-motor interference during functional mobility after stroke: state of the science and implications for future research. Arch. Phys. Med. Rehabil. 94, 2565–2574.e6. doi: 10.1016/j.apmr.2013.08.002
Plummer, P., Grewal, G., Najafi, B., and Ballard, A. (2015b). Instructions and skill level influence reliability of dual-task performance in young adults. Gait Posture 41, 964–967. doi: 10.1016/j.gaitpost.2015.03.348
Plummer, P., Villalobos, R. M., Vayda, M. S., Moser, M., and Johnson, E. (2014). Feasibility of dual-task gait training for community-dwelling adults after stroke: a case series. Stroke Res. Treat. 2014:538602. doi: 10.1155/2014/538602
Pontifex, M. B., Hillman, C. H., Fernhall, B., Thompson, K. M., and Valentini, T. A. (2009). The effect of acute aerobic and resistance exercise on working memory. Med. Sci. Sports Exerc. 41, 927–934. doi: 10.1249/MSS.0b013e3181907d69
Pouw, W. T. J. L., Nooijer, J. A. de, van Gog, T., Zwaan, R. A., and Paas, F. (2014a). Toward a more embedded/extended perspective on the cognitive function of gestures. Front. Psychol. 5:359. doi: 10.3389/fpsyg.2014.00359
Pouw, W. T. J. L., van Gog, T., and Paas, F. (2014b). An embedded and embodied cognition review of instructional manipulatives. Educ. Psychol. Rev. 26, 51–72. doi: 10.1007/s10648-014-9255-5
Prince, M., Bryce, R., Albanese, E., Wimo, A., Ribeiro, W., and Ferri, C. P. (2013). The global prevalence of dementia: a systematic review and metaanalysis. Alzheimers Dement. 9, 63.e2–75.e2. doi: 10.1016/j.jalz.2012.11.007
Raichlen, D. A., and Alexander, G. E. (2017). Adaptive capacity: an evolutionary neuroscience model linking exercise, cognition, and brain health. Trends Neurosci. 40, 408–421. doi: 10.1016/j.tins.2017.05.001
Rehfeld, K., Müller, P., Aye, N., Schmicker, M., Dordevic, M., Kaufmann, J., et al. (2017). Dancing or fitness sport? The effects of two training programs on hippocampal plasticity and balance abilities in healthy seniors. Front. Hum. Neurosci. 11:261. doi: 10.3389/fnhum.2017.00305
Roig, M., Nordbrandt, S., Geertsen, S. S., and Nielsen, J. B. (2013). The effects of cardiovascular exercise on human memory: a review with meta-analysis. Neurosci. Biobehav. Rev. 37, 1645–1666. doi: 10.1016/j.neubiorev.2013.06.012
Roig, M., Skriver, K., Lundbye-Jensen, J., Kiens, B., and Nielsen, J. B. (2012). A single bout of exercise improves motor memory. PLoS One 7:e44594. doi: 10.1371/journal.pone.0044594
Rolland, Y., van Abellan Kan, G., and Vellas, B. (2010). Healthy brain aging: role of exercise and physical activity. Clin. Geriatr. Med. 26, 75–87. doi: 10.1016/j.cger.2009.11.002
Ruiter, M., Loyens, S., and Paas, F. (2015). Watch your step children! learning two-digit numbers through mirror-based observation of self-initiated body movements. Educ. Psychol. Rev. 27, 457–474. doi: 10.1007/s10648-015-9324-4
Scharhag-Rosenberger, F., Meyer, T., Gässler, N., Faude, O., and Kindermann, W. (2010). Exercise at given percentages of VO2max: heterogeneous metabolic responses between individuals. J. Sci. Med. Sport 13, 74–79. doi: 10.1016/j.jsams.2008.12.626
Scherder, E., Eggermont, L., Visscher, C., Scheltens, P., and Swaab, D. (2011). Understanding higher level gait disturbances in mild dementia in order to improve rehabilitation: ‘Last in–first out’. Neurosci. Biobehav. Rev. 35, 699–714. doi: 10.1016/j.neubiorev.2010.08.009
Scherder, E. J.A., Bogen, T., Eggermont, L. P., Hamers, J. P., and Swaab, D. F. (2010). The more physical inactivity, the more agitation in dementia. Int. Psychogeriatr. 22, 1203–1208. doi: 10.1017/S1041610210001493
Schlaffke, L., Lissek, S., Lenz, M., Brune, M., Juckel, G., Hinrichs, T., et al. (2014). Sports and brain morphology - a voxel-based morphometry study with endurance athletes and martial artists. Neuroscience 259, 35–42. doi: 10.1016/j.neuroscience.2013.11.046
Schneider, S., Brummer, V., Abel, T., Askew, C. D., and Struder, H. K. (2009). Changes in brain cortical activity measured by EEG are related to individual exercise preferences. Physiol. Behav. 98, 447–452. doi: 10.1016/j.physbeh.2009.07.010
Schott, N. (2015). Trail Walking Test zur Erfassung der motorisch-kognitiven Interferenz bei alteren Erwachsenen. Entwicklung und Uberprufung der psychometrischen Eigenschaften des Verfahrens. Z. Gerontol. Geriatr. 48, 722–733. doi: 10.1007/s00391-015-0866-3
Shams, L., and Seitz, A. R. (2008). Benefits of multisensory learning. Trends Cogn. Sci. 12, 411–417. doi: 10.1016/j.tics.2008.07.006
Skjæret, N., Nawaz, A., Morat, T., Schoene, D., Helbostad, J. L., and Vereijken, B. (2016). Exercise and rehabilitation delivered through exergames in older adults: an integrative review of technologies, safety and efficacy. Int. J. Med. Inf. 85, 1–16. doi: 10.1016/j.ijmedinf.2015.10.008
Smith, J. C., Nielson, K. A., Woodard, J. L., Seidenberg, M., and Rao, S. M. (2013). Physical activity and brain function in older adults at increased risk for Alzheimer’s disease. Brain Sci. 3, 54–83. doi: 10.3390/brainsci3010054
Sofi, F., Valecchi, D., Bacci, D., Abbate, R., Gensini, G. F., Casini, A., et al. (2011). Physical activity and risk of cognitive decline: a meta-analysis of prospective studies. J. Intern. Med. 269, 107–117. doi: 10.1111/j.1365-2796.2010.02281.x
Song, D., Yu, D. S. F., Li, P. W. C., and Lei, Y. (2018). The effectiveness of physical exercise on cognitive and psychological outcomes in individuals with mild cognitive impairment: a systematic review and meta-analysis. Int. J. Nurs. Stud. 79, 155–164. doi: 10.1016/j.ijnurstu.2018.01.002
Stern, Y. (2002). What is cognitive reserve? Theory and research application of the reserve concept. J. Int. Neuropsychol. Soc. 8, 448–460. doi: 10.1017/S1355617702813248
Stern, Y. (2009). Cognitive reserve. Neuropsychologia 47, 2015–2028. doi: 10.1016/j.neuropsychologia.2009.03.004
Stern, Y. (2012). Cognitive reserve in ageing and Alzheimer’s disease. Lancet Neurol. 11, 1006–1012. doi: 10.1016/S1474-4422(12)70191-6
Stern, Y. (2013). Cognitive reserve: implications for assessment and intervention. Folia Phoniatr. Logop. 65, 49–54. doi: 10.1159/000353443
Stillman, C. M., Cohen, J., Lehman, M. E., and Erickson, K. I. (2016). Mediators of physical activity on neurocognitive function: a review at multiple levels of analysis. Front. Hum. Neurosci. 10:626. doi: 10.3389/fnhum.2016.00626
Stimpson, N. J., Davison, G., and Javadi, A. -H. (2018). Joggin’ the noggin: towards a physiological understanding of exercise-induced cognitive benefits. Neurosci. Biobehav. Rev. 88, 177–186. doi: 10.1016/j.neubiorev.2018.03.018
Szuhany, K. L., Bugatti, M., and Otto, M. W. (2015). A meta-analytic review of the effects of exercise on brain-derived neurotrophic factor. J. Psychiatr. Res. 60, 56–64. doi: 10.1016/j.jpsychires.2014.10.003
Tait, J. L., Duckham, R. L., Milte, C. M., Main, L. C., and Daly, R. M. (2017). Influence of sequential vs. simultaneous dual-task exercise training on cognitive function in older adults. Front. Aging Neurosci. 9:368. doi: 10.3389/fnagi.2017.00368
Tarr, M. J., and Warren, W. H. (2002). Virtual reality in behavioral neuroscience and beyond. Nat. Neurosci. 5, 1089–1092. doi: 10.1038/nn948
Toumpaniari, K., Loyens, S., Mavilidi, M. -F., and Paas, F. (2015). Preschool children’s foreign language vocabulary learning by embodying words through physical activity and gesturing. Educ. Psychol. Rev. 27, 445–456. doi: 10.1007/s10648-015-9316-4
Trachtenberg, J. T., Chen, B. E., Knott, G. W., Feng, G., Sanes, J. R., Welker, E., et al. (2002). Long-term in vivo imaging of experience-dependent synaptic plasticity in adult cortex. Nature 420, 788–794. doi: 10.1038/nature01273
Trombly, C. A. (1995). Occupation: purposefulness and meaningfulness as therapeutic mechanisms. 1995 Eleanor Clarke Slagle Lecture. Am. J. Occup. Ther. 49, 960–972. doi: 10.5014/ajot.49.10.960
van Dongen, E. V., Kersten, I. H. P., Wagner, I. C., Morris, R. G. M., and Fernandez, G. (2016). Physical exercise performed four hours after learning improves memory retention and increases hippocampal pattern similarity during retrieval. Curr. Biol. 26, 1722–1727. doi: 10.1016/j.cub.2016.04.071
Vanrenterghem, J., Nedergaard, N. J., Robinson, M. A., and Drust, B. (2017). Training load monitoring in team sports: a novel framework separating physiological and biomechanical load-adaptation pathways. Sports Med. 47, 2135–2142. doi: 10.1007/s40279-017-0714-2
Verburgh, L., Konigs, M., Scherder, E. J. A., and Oosterlaan, J. (2014). Physical exercise and executive functions in preadolescent children, adolescents and young adults: a meta-analysis. Br. J. Sports Med. 48, 973–979. doi: 10.1136/bjsports-2012-091441
Verghese, J., Lipton, R. B., Katz, M. J., Hall, C. B., Derby, C. A., Kuslansky, G., et al. (2003). Leisure activities and the risk of dementia in the elderly. N. Engl. J. Med. 348, 2508–2516. doi: 10.1056/NEJMoa022252
Voelcker-Rehage, C., Godde, B., and Staudinger, U. M. (2010). Physical and motor fitness are both related to cognition in old age. Eur. J. Neurosci. 31, 167–176. doi: 10.1111/j.1460-9568.2009.07014.x
Voelcker-Rehage, C., Godde, B., and Staudinger, U. M. (2011). Cardiovascular and coordination training differentially improve cognitive performance and neural processing in older adults. Front. Hum. Neurosci. 5:26. doi: 10.3389/fnhum.2011.00026
Voelcker-Rehage, C., and Niemann, C. (2013). Structural and functional brain changes related to different types of physical activity across the life span. Neurosci. Biobehav. Rev. 37, 2268–2295. doi: 10.1016/j.neubiorev.2013.01.028
Wilson, M. (2002). Six views of embodied cognition. Psychon. Bull. Rev. 9, 625–636. doi: 10.3758/BF03196322
Wimo, A., Jonsson, L., Bond, J., Prince, M., and Winblad, B. (2013). The worldwide economic impact of dementia 2010. Alzheimers Dement. 9, 1.e3–11.e3. doi: 10.1016/j.jalz.2012.11.006
Yogev-Seligmann, G., Hausdorff, J. M., and Giladi, N. (2012a). Do we always prioritize balance when walking? Towards an integrated model of task prioritization. Mov. Disord. 27, 765–770. doi: 10.1002/mds.24963
Yogev-Seligmann, G., Rotem-Galili, Y., Dickstein, R., Giladi, N., and Hausdorff, J. M. (2012b). Effects of explicit prioritization on dual task walking in patients with Parkinson’s disease. Gait Posture 35, 641–646. doi: 10.1016/j.gaitpost.2011.12.016
Zach, S., and Shalom, E. (2016). The influence of acute physical activity on working memory. Percept. Mot. Skills 122, 365–374. doi: 10.1177/0031512516631066
Keywords: exercise, cognition, dementia, dual task, cognitive enhancement
Citation: Herold F, Hamacher D, Schega L and Müller NG (2018) Thinking While Moving or Moving While Thinking – Concepts of Motor-Cognitive Training for Cognitive Performance Enhancement. Front. Aging Neurosci. 10:228. doi: 10.3389/fnagi.2018.00228
Received: 16 February 2018; Accepted: 09 July 2018;
Published: 06 August 2018.
Edited by:
Ashok Kumar, University of Florida, United StatesCopyright © 2018 Herold, Hamacher, Schega and Müller. This is an open-access article distributed under the terms of the Creative Commons Attribution License (CC BY). The use, distribution or reproduction in other forums is permitted, provided the original author(s) and the copyright owner(s) are credited and that the original publication in this journal is cited, in accordance with accepted academic practice. No use, distribution or reproduction is permitted which does not comply with these terms.
*Correspondence: Fabian Herold, fabian.herold@dzne.de