- 1State Key Laboratory of Genetic Engineering and Collaborative Innovation Center for Genetics and Development, School of Life Sciences, Fudan University, Shanghai, China
- 2Human Phenome Institute, Fudan University, Shanghai, China
- 3Ministry of Education Key Laboratory of Contemporary Anthropology, Department of Anthropology and Human Genetics, School of Life Sciences, Fudan University, Shanghai, China
Mitochondria are the main producers of energy in eukaryotic cells. Mitochondrial dysfunction is associated with specific mitochondrial DNA (mtDNA) variations (haplogroups), and these variations can contribute to human disease. East Asian populations show enrichment of many mitochondrial haplogroups, including A, B, D, G, M7, M8, M9, N9, R9, and exhibit half of the known haplogroups of worldwide. In this review, we summarize the current research in the field of mtDNA variation and associated disease in East Asian populations and discuss the physiological and pathological relevance of mitochondrial biology. mtDNA haplogroups are associated with various metabolic disorders ascribed to altered oxidative phosphorylation. The same mitochondrial haplogroup can show either a negative or positive association with different diseases. Mitochondrial dynamics, mitophagy, and mitochondrial oxidative stress, ultimately influence susceptibility to various diseases. In addition, mitochondrial retrograde signaling pathways may have profound effects on nuclear-mitochondrial interactions, affecting cellular morphology, and function. Other complex networks including proteostasis, mitochondrial unfolded protein response and reactive oxygen species signaling may also play pivotal roles in metabolic performance.
Introduction
Mitochondria are cytoplasmic organelles of eukaryotic cells that provide more than 90% of the cell's adenosine triphosphate (ATP) through oxidative phosphorylation (OXPHOS) and the mitochondrial electron transport chain (ETC). OXPHOS enzymes include five complexes: complex I (NADH: ubiquinone oxidoreductase), complex II (FADH2: succinate dehydrogenase), complex III (coenzyme Q- Cytochrome C [Cyt-C] reductase), complex IV (Cyt-C oxidase), and complex V (F1F0-ATP synthase). These are embed in the inner mitochondrial membrane. There are about 1,500 proteins that maintain the normal structure and function of mitochondria. Thirteen of these proteins are mtDNA-encoded proteins. The remainder are encoded by nuclear genes and are synthesized in the cytoplasm and transported into mitochondria (Wallace, 2005). Electrons from NADH and FADH2 are transferred to complexes I and II, respectively, and are donated to complex III via ubiquinone. Complex IV then receives electrons from complex III via Cyt-C to reduce molecular oxygen to water. Electrons transferred between these complexes generate a proton gradient across the inner mitochondrial membrane, which is then used by complex V to synthesize ATP from ADP (Wallace, 2013).
mtDNA is continuously synthesized throughout the cell cycle in early human embryonic development. mtDNA has an extremely high mutation rate, presumably due to the lack of histone protection and chronic exposure to mitochondrial reactive oxygen species (ROS). Pathogenic mtDNA mutations include rearrangement mutations (Holt et al., 1988), polypeptide gene missense mutations (Wallace et al., 1988a), and gene mutations (rRNA and tRNA) related to protein synthesis (Wallace et al., 1988b; Shoffner et al., 1990). These mismatches, designated single nucleotide polymorphisms (SNPs) by the Cambridge reference sequence for human mtDNA, determine mitochondrial haplogroups. A mitochondrial haplogroup is a combination of variants that are phylogenetically related (PhyloTree.org-mtDNA tree). A Sub-haplogroup is a sub-branch of haplogroup characterized by new variants, based on the major haplogroup branches. Numerous pathogenic variations in mtDNA or in nuclear DNA (nDNA) encoding mitochondrial proteins may lead to clinically and genetically heterogeneous disorders due to mitochondrial ETC dysfunction (Fang H. et al., 2015; van Rahden et al., 2015; Piekutowska-Abramczuk et al., 2018). Insufficient energy for cardiac and skeletal muscles, brain, liver, and kidney, may lead to metabolic disorders (Lu et al., 2010; Nishigaki et al., 2010; Wang et al., 2010; Liou et al., 2016). Tools for studies of mutant mtDNA have been established by fusing enucleated cells with mitochondria donors, which are called cybrids. Cybrids are useful for studying alterations of mitochondrial function at the cellular level without the influence of the nuclear background (King and Attardi, 1989; Wilkins et al., 2014).
As a multi-ethnic region, East Asia contains nearly half of the known mitochondrial haplogroups, most of which are associated with metabolic and degenerative diseases (Kong et al., 2006; Takasaki, 2008; van Oven and Kayser, 2009). No review has been published discussing the relationship between mtDNA variations and diseases in East Asia. It is important to correlate the East Asian mtDNA variations with different disease to determine their role in pathogenesis. Herein, we review the current research on mtDNA variations and haplogroups in various metabolic diseases and discuss the physiological and pathological relevance of mitochondrial biology in East Asia.
Mitochondrial Haplogroups Associated With Disease in East Asia
Figure 1 and Table 1 summarize previous studies of the East Asia mitochondrial haplogroups and their association with disease Multi-ethnic East Asia populations (Figure 1) account for more than half of the known haplogroups worldwide. Haplogroups M and N are essentially equally represented. Haplogroup M includes sub-haplogroups D4, D5, M7, and G, Haplogroup N includes B4, B5, N9, A, and F. mtDNA variation is not uniform from south to north in East Asia. In the north, more haplogroup M is present, which includes the sub-haplogroups A, C, D4, D5, G, M8, M9, N9, and Z. In the south, more haplogroup N is found, specifically sub-haplogroups B4a,B5a, F, M7, and R9 (Xue et al., 2008). Most of these haplogroups are associated with metabolic and degenerative diseases (Kong et al., 2006; Takasaki, 2008; van Oven and Kayser, 2009). Table 1 shows mitochondrial haplogroups which are closely related to several diseases. Understanding the mechanisms of mitochondrial dysfunction is critical for clinical diagnosis and for development of therapies for patients with mitochondrial diseases.
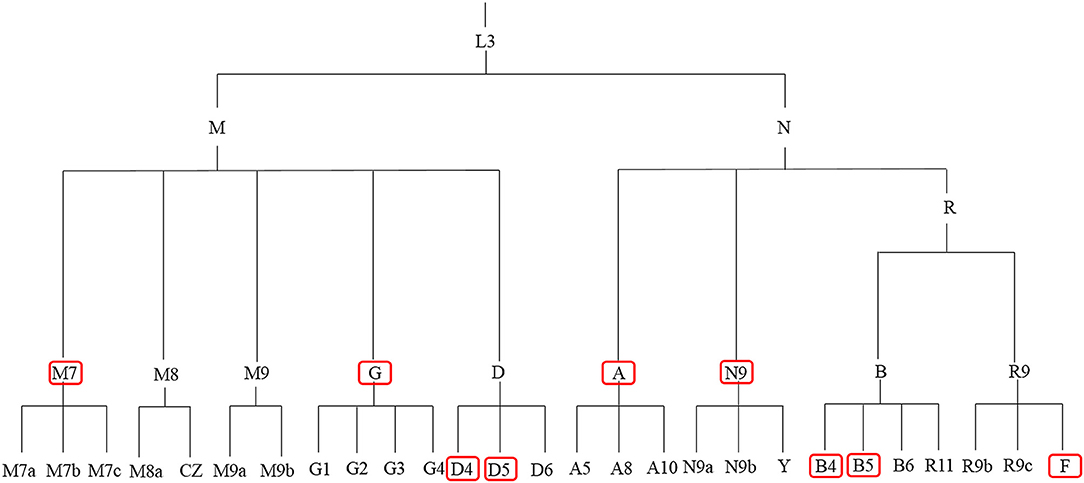
Figure 1. mtDNA tree of the mitochondrial haplogroups in East Asia. The red boxes denote the high frequency mitochondrial haplogroups in East Asia.
Nervous System Diseases
Neurons need most energy produced from mitochondria to maintain neuron cellular function. In addition, normal mitophagy to eliminate misfolded and aggregated proteins is necessary for neuron cellular function. Mitophagy is a specific process that degrade damaged mitochondria to maintain cellular homeostasis based on the mechanism of autophagy (Youle and Narendra, 2011). Autophagy is dependent on enclosing ubiquitinated proteins in vesicles termed “autophagosomes,” and subsequently lysosomal fusion (Levine and Kroemer, 2008). mtDNA mutation can lead to mitophagy (Dombi et al., 2016) and mitochondrial dysfunction (Dolle et al., 2016; Pera et al., 2017; Ehrnhoefer et al., 2018; Lindqvist et al., 2018; Pereira et al., 2018; Puthumana and Regenold, 2019) have long been reported as pathogenic in psychiatric and neurodegenerative diseases.
Alzheimer Disease and Parkinson's Disease
Mitochondrial abnormalities are associated with high levels of amyloid-beta protein in Alzheimer disease (AD) (Wang et al., 2007). Studies found increased tricarboxylic acid (TCA) cycle metabolism in AD patients due to an increased levels of ROS from deficiencies in OXPHOS (Bubber et al., 2005; Carvalho et al., 2009). Other papers suggest that mitochondrial dysfunction may start 3 months before extracellular deposition of amyloid-beta protein, and that progression accelerates with aging (Hauptmann et al., 2009). The mitophagy-related-protein-PINK1, which plays a pivotal role in Parkinson's disease (PD). PINK1 regulates mitochondrial stress through indirect interaction with mitochondrial proteases and the fission protein-Drp1 to further recruit Parkin by depolarized mitochondria (Chu, 2010). In addition, mitochondrial respiration complex I and IV deficiency may also contribute to the occurrence of AD and PD (Holper et al., 2019).
Comprehensive epidemiological analyses of mtDNA variations in Japanese patients with AD (n = 96) or PD (n = 96) showed that AD is uniquely associated with haplogroups G2a, B4c1, and N9b1, and PD with haplogroups M7a, M7b2, B4e, and B5b (Takasaki, 2008, 2009). In Han Chinese populations, haplogroup B5 is significantly associated with AD (n = 341) in patients from Southwest China (Bi et al., 2015). Cells with the B5 haplogroup had higher levels of ROS, decreased mitochondrial mass, lower ATP generation, and lower respiration when compared with non-B5 haplogroup cells (Bi et al., 2015). A study of the distribution of mtDNA haplogroups of the Han population with sporadic PD (n = 279) indicated that haplogroup B may confer a lower risk for PD, while haplogroup D may lead to a higher risk of PD in people younger than 50 years of age (Chen et al., 2015). Consistent with these findings, Liou et al. determined the association of mtDNA haplogroups with PD patients (n = 725) in Taiwan. They also found that mitochondrial haplogroup B5 confers resistance to PD. In cybrid cellular models, the B5 cybrid showed lower ROS generation and a lower rate of apoptosis compared with the B4 cybrid (Liou et al., 2016).
Psychiatric Disorders
Mitochondrial abnormalities may be involved in the pathophysiology of psychiatric disorders, such as schizophrenia, bipolar disorder, and attention deficit hyperactivity disorder (ADHD). Studies showed decreased protein and transcript levels of mitochondrial complex I and IV, decreased mitochondrial fusion levels, increased fission levels, and impaired OXPHOS in patients with schizophrenia or with bipolar disorder (Bubber et al., 2004; Hjelm et al., 2015; Flippo and Strack, 2017; Haghighatfard et al., 2017; Rollins et al., 2018; Holper et al., 2019).
A recent study of 11 families with schizophrenia demonstrated that mtDNA A15395G and A8536G were deleterious (Bi et al., 2016). Functional characterization further confirmed the potential pathogenicity of the two variants which includes lower mitomass, mtDNA copy number, respiration, ATP, and higher ROS (Bi et al., 2016). The T3644C mutation was found in Japanese patients with bipolar disorder (n = 199) but not in healthy controls. This mutation converts a well-conserved valine, to alanine in the complex I ND1 subunit, and may impair assembly of complex I. The m.3644T>C (MT-ND1) variant alters mitochondrial function by decreasing mitochondrial membrane potential (MMP) and complex I activity in 3644C cybrids compared with 3644T cybrids (Munakata et al., 2004). An epidemiologic study of Korean ADHD children (n = 150) revealed that haplogroup B4 increases the occurrence of ADHD, and haplogroup B5 and D4b are significantly associated with ADHD boys and girls, respectively. These results suggest that mtDNA plays an important role in the genetic etiology of ADHD in Korean children (Hwang et al., 2018). Cybrids of the SH-SY5Y neuroblastoma cell line showed decreased complex V activity and MMP, but elevated oxidative stress (Verma et al., 2016).
Optic Neuropathy
Mitochondrial dysfunction can also cause optic neuropathy. The relationship between several mtDNA variants (G11778A, G3460A, T14484C, G11696A, G13708A, G10680A, and T12338C) and Leber's hereditary optic neuropathy (LHON) have been reported (Yoneda et al., 1989; Hotta et al., 1995; Brown et al., 2000; Ji et al., 2008). The typical LHON-related G11778A mutation in different families belongs to the Chinese haplogroups B5b, G2a, C4a1, M7b102, and M8a; the Thai urban population haplogroups M and B; and European haplogroups J, K, and H, respectively. Several groups constructed cybrids of LHON probands carrying the G3460A, G11778A, and T14484C LHON primary mutations to confirm that mitochondrial dysfunction is caused by these mtDNA variants. They detected complex I-dependent defects in respiration, decreased ATP synthesis, increased ROS production, disrupted glutamate transport, increased mitochondrial-dependent apoptotic death, delayed complex I assembly kinetics, and instability of complexes III and IV (Ghelli et al., 2003; Baracca et al., 2005; Floreani et al., 2005; Pello et al., 2008). The mtDNA G13051A leaded to variable neurology and activated mitophagy in LHON patients (Dombi et al., 2016). Mitophagy activation can also repair LHON-associated mitochondrial dysfunction and improve cell survival (Sharma et al., 2019).
These results point to promising targets for predicting the probabilities and initial diagnosis of nervous system diseases, although the etiology of these diseases remains unclear. Targeting mitochondrial function and oxidative stress, with antioxidants, coenzyme Q10, and vitamin C may be effective strategies to ameliorate the progress of nervous system diseases.
Endocrine System Diseases
Steroid hormone biosynthesis is carried out in mitochondria. The ATP produced by the mitochondria also provides energy for hormone generation and trafficking. mtDNA variants may result in endocrine organ defects due to impaired OXPHOS (Chow et al., 2017).
Diabetes Mellitus
Diabetes mellitus (DM) is a major global health problem. It is a challenge to understand the physiological and pathological conditions that lead to the development of this disease (Zimmet et al., 2001; Roglic and Unwin, 2010). Mitochondria are essential for providing energy to maintain insulin metabolism; mtDNA mutations of OXPHOS complexes can lead to pancreatic islet dysfunction. Increased mitochondrial fission may impair endothelial function via increased ROS in DM (Shenouda et al., 2011). Decreased OXPHOS and fatty acid oxidation in insulin-sensitive tissues has been reported (Kwak and Park, 2016). Mitochondrial uncoupling may protect the mitochondrial matrix against lipid-induced mitochondrial damage (Schrauwen and Hesselink, 2004).
The association between mtDNA haplogroups and the risk of DM is controversial. Some studies found diabetes susceptibility genes located in mitochondria-encoded genomes, such as G3316A and C3310T in a Japanese family (Nakano et al., 1998; Hattori et al., 2005), and G3316A, C3310T, A3243G T3394C, G4491A, T16189C, and T16519C in a Chinese population (n = 826) (Liao et al., 2008; Li M. Z. et al., 2008; Wang et al., 2013; Zhong et al., 2014). The N9a, M8a, B4 and D4 haplogroups appeared to be related to DM in East Asia (n = 1289) (Fuku et al., 2007; Loo et al., 2014; Li et al., 2015). A cybrid with a C3310T mutation showed that mitochondrial complex I activity, ATP generation, oxygen consumption were significantly decreased (Chen et al., 2006). Fuku et al. found that mitochondrial haplogroup N9a was a significant protective factor for DM in a Korean study (n = 732) (Fuku et al., 2007). In contrast, Niu et al. found that N9a was a risk factor for diabetic nephropathy (n = 235) (Niu et al., 2015). Subsequently, Fang et al. confirmed that the N9a haplogroup increased the risk of DM in the Chinese population by altering mitochondrial function and intracellular mitochondrial signals. The N9a haplogroup cybrids exhibited lower respiratory chain complex activity, ATP, MMP and oxygen consumption; however, they contained more ROS and fragmented mitochondria than non-N9a haplogroup cybrids. Insulin-stimulated glucose uptake was partially inhibited through enhanced stimulation of ERK1/2 phosphorylation and subsequent TLR4 activation in N9a haplogroup cybrids (Fang et al., 2018). Taken together, these studies show that mitochondrial haplogroups profoundly affect the occurrence and development of diabetes. Some antioxidants, including vitamin C and vitamin E, can ameliorate the oxidative stress associated with diabetes (Victor et al., 2011).
Diabetic Kidney Disease
Diabetic kidney disease (DKD) is the most common cause of end-stage kidney disease worldwide. Mitochondrial dysfunction plays a role in the pathophysiology of diabetes (Susztak et al., 2006; Fakhruddin et al., 2017; Forbes and Thorburn, 2018). Overproduction of ROS, activation of apoptosis, and defective mitophagy have been shown to contribute to the progression of the disease (Wei and Szeto, 2019). A study of mitochondria-targeted metabolic tubular injury in diabetic kidney disease, which included healthy controls (n = 65), diabetes patients without kidney disease (n = 48), and DKD patients (n = 60) was carried out in China. The accumulation of damaged mtDNA, fragmented mitochondria, activated apoptosis, loss of MMP, and perturbations in glycolysis and TCA cycle were detected in tubules and PBMCs from the patients. These results indicate that mitochondrial damage may be the hallmark of DKD patients (Jiang et al., 2019).
Salidroside, an active component from the traditional Chinese medicine Rhodiola rosea L, stimulated the Sirt1/PGC-1alpha axis and ameliorated diabetic nephropathy through enhanced mitochondrial DNA copy number and ETC protein expression in mice (Xue et al., 2019). SIRT3 overexpression also inhibited kidney tumor cells and improved mitochondrial biogenesis rather than exhibiting the Warburg effect (Liu et al., 2018). Overexpression of MnSOD can abrogate mitochondrial dysfunction and effectively prevent the development of diabetic retinopathy (Madsen-Bouterse et al., 2010). Pyruvate kinase M2 activation may protect against the progression of diabetic glomerular pathology and mitochondrial dysfunction by increasing glucose metabolic flux, and inhibiting the production of toxic glucose metabolites (Qi et al., 2017).
Obesity
Obesity is one of the most important pathogenic factors of DM. Impaired mitochondrial lipid oxidation, increased inflammation, and increased mitochondrial OXPHOS in the liver have been observed in obese subjects (Khasawneh et al., 2009; Rogge, 2009; Buchner et al., 2011). Guo et al. found that the C8684T transition of haplogroup M8a, and the C3497T and T1119C transitions of haplogroup B4c caused increased susceptibility to DM.
Asthenozoospermia
Asthenozoospermia is a multi-factor disorder that affects approximately half of males with infertility, and nearly 15% of cases result from genetic abnormalities (Moore and Reijo-Pera, 2000; Ferlin et al., 2007). Mitochondrial ATP, appropriate MMP levels, respiration activity, and low ROS levels are necessary to sustain normal sperm motility (Kasai et al., 2002; Marchetti et al., 2002; Ferramosca et al., 2012). The first study of human mtDNA haplogroups associated with asthenozoospermia was performed in Spain. The authors showed that haplogroups H and T conferred susceptibility to non-asthenozoospermic and asthenozoospermic, respectively. More importantly, complex IV activity was significantly decreased in haplogroup T cybrids (Ruiz-Pesini et al., 2000). In the Han population, men with haplogroup R exhibited decreased frequency of asthenozoospermia (n = 312) (Feng et al., 2013). Recently, Wang's group reported that haplogroup M8a played a critical role in the penetrance of variant C8684T in the pathogenesis of non-obstructive azoospermia due to increased mtDNA damage and impaired normal spermatogenesis (Ji et al., 2018). These studies indicated that mitochondria play a pivotal role of human fertility.
Cancer
Cancer is a chronic non-communicable disease affecting most of the people worldwide. Cancer cells attack the immune system of the human body. Mitochondria play a critical role in providing energy for T cells, B cells, and marcrophages, albeit the metabolism of the various cells are diverse. For example, M1 macrophages utilize a modified TCA cycle to drive inflammation and are characterized by increased lactate production. However, M2 macrophages require both mitochondrial TCA metabolism and glycolysis; they use acetyl-CoA to drive forward flux through the ETC, but also require glycolysis, in part to support hexosamine biosynthesis (Vats et al., 2006; Haschemi et al., 2012; Huang et al., 2014; Jin et al., 2014). Each cell type possesses its own unique immune status, it can be pro-inflammatory or anti-inflammatory, depending on the different metabolic pathways. Additionally, new mitochondrial DNA synthesis enables NLRP3 inflammasome activation (Zhong et al., 2018). Therefore, mtDNA mutations profoundly affect the human immune system. Here, we focus on some common diseases associated with cancer.
The “Warburg effect” is a feature of most cancer cells, they prefer aerobic glycolysis from glucose to lactate in the presence of oxygen, rather than complete oxidation of glucose and mitochondrial respiration (Warburg, 1956; Wallace, 2005). Mitochondrial DNA mutation would affect mitochondrial biogenesis and turnover, mitophage, fission and fusion dynamics, oxidative stress, and cell death (Smith et al., 2012). The balance of mitochondrial metabolism plays a crucial role in the pathogenesis and progression of human malignancies, and mtDNA mutations play bidirectional roles in tumorigenesis; some are protective factors, while others are risk factors (Xu et al., 2006; Li et al., 2007; Ma L. et al., 2018).
Endometrial Cancer
A PGC1α-dependent pathway increases mitochondrial biogenesis, mitochondrial fission, mitophagy, proteolysis, and antioxidant response in endometrial cancer (Cormio et al., 2017), and haplogroup D shows significant correlation with the incidence of endometrial cancer in the Yunnan province in China (Xu et al., 2006). Treatment of 1, 1-bis (3′-indolyl)-1-(p-substituted phenyl) methane has been reported to decrease mitochondrial membrane potential and induce apoptosis in endometrial cancer cell lines (Hong et al., 2008).
Breast Cancer
Mitochondrial dysfunction and abnormal intracellular mitochondrial signaling has been detected in breast cancer cells (Santidrian et al., 2013; Pelicano et al., 2014). Haplogroup M may be a risk factor for breast cancer. Mutations in the D-loop region are more likely to be detected in benign breast tumors (n = 104) (Fang et al., 2010). The frequency of haplogroup D5 is significantly increased in patients with breast cancer. It was found that mitochondrial respiration, ATP content, and MMP levels were decreased in D5 haplogroup cybrids compared to those with non-D5 haplogroups. The D5 cybrids were also more susceptible to tumorigenesis through activation of the AKT pathway, mediated by ROS generation (Ma L. et al., 2018). A potential therapeutic strategy for breast cancer may depend on improving the NAD+/NADH balance through treatment with NAD+ precursors, which can inhibit metastasis and prevent progression of breast cancer (Santidrian et al., 2013).
Cervical Cancer
mtDNA variations located in the D-loop, coding region, and tRNA and rRNA genes are potential biomarkers in cervical carcinogenesis (Kabekkodu et al., 2014). Two groups investigated mtDNA mutations in cervical cancers of Chinese women. Zhai et al. reported that an mtDNA C150T polymorphism in HPV-positive cervical cancer patients was significantly increased compared to HPV-negative controls (Zhai et al., 2011). Li's group found that mitochondrial haplogroup D4b1 enhanced the risk of cervical cancer initiation in Chinese women (n = 150) (Li et al., 2016).
There are many therapeutic drugs targeted to mitochondrial dysfunction available today: benzimidazolethiol induces apoptosis by regulating the PI3K/Akt signaling pathway, interferon alpha activates both the intrinsic mitochondrial pathway and endoplasmic reticulum stress-induced pathway, mefloquine impairs mitochondrial function and inhibits mTOR pathway, nicotinamide induces mitochondrial-mediated apoptosis through oxidative stress, tocotrienol inhibits proliferation and inducing apoptosis, betulinic acid induces apoptosis by regulating PI3K/Akt signaling, and pterostilbene targets m-TOR/PI3K/Akt signaling pathway via disruption of MMP (Hoti et al., 2003; Shi et al., 2016; Feng et al., 2017; Li et al., 2017; Xu T. et al., 2017; Xu W. et al., 2017; Hong Bin et al., 2018; Tian et al., 2018).
Prostate Cancer
Prostate cancer is associated with dysregulation of OXPHOS. A multiethnic cohort epidemiological study of 4,086 prostate cancer cases and 3,698 controls from African, Asian, American, European, Latino, and Native Hawaiian patients was performed in order to examine the association of mtDNA and prostate cancer. This study revealed that haplogroup N contributed to overall prostate cancer, however, the mtDNA-encoded OXPHOS genes were not associated with prostate cancer risk in this cohort (Giorgi et al., 2016). A study of a Korean population also revealed no association of the mtDNA-encoded OXPHOS genes with prostate cancer (n = 139) (Kim et al., 2008). However, several studies of prostate cancer demonstrated that mitochondrial dysfunction and altered intermediary metabolism, especially high ROS levels, occurred in prostate cancer cells (Dakubo et al., 2006; Mizumachi et al., 2008; Altieri, 2010; Chaudhary et al., 2017). ROS production accelerated mtDNA mutations in prostate cancer and further stimulated malignant transformation of prostate through increased ETC activity (Dakubo et al., 2006).
Lung Cancer
Cell migration and invasion, which occurs through the induction of AKT and AMPK pathways in lung cancer cells, has been associated with mitochondrial dysfunction (Han et al., 2018). Two case-control cohort studies found an association between mtDNA variation and lung cancer risk in a Han Chinese population from southwestern China (n = 422). Zheng et al. revealed that haplogroups D and F were protective factors for lung cancer, while haplogroups G and M7 increased susceptibility (Zheng et al., 2012). However, Fang's group demonstrated that haplogroups F and G predisposed people to lung cancer (n = 237). Although the results varied, both studies suggest that haplogroup G is a risk factor for lung cancer due to excess ROS generated by the impaired mitochondrial respiration chain (Fang Y. et al., 2015). In lung cancer, altered rates of mitochondrial fission and fusion were seen, which can influence metabolic function, proliferation, and cell survival. Therefore, altering mitochondrial dynamics may be a therapeutic strategy, for example, inhibiting mitochondrial fission can prevent cell cycle progression in lung cancer (Rehman et al., 2012; Lennon and Salgia, 2014).
Hepatocellular Carcinoma
Mutations in the mitochondrial D-loop region have been reported in hepatocellular carcinoma (HCC), which may partly contribute to cancer development (Zhang et al., 2010). Mitochondrial pyruvate carrier (MPC1/2) protein expression was significantly downregulated in HCC, and may serve as a biomarker for the identification of patients with this disease (Ma X. et al., 2018). Mitochondrial fission significantly promoted the reprogramming of focal-adhesion dynamics and lamellipodia formation in HCC cells, mainly by activating Ca2+/CaMKII/ERK/FAK pathway (Sun et al., 2018). In addition, Drp1-mediated mitochondrial fission promoted cell proliferation through crosstalk between the p53 and NF-kappaB pathways in HCC (Zhan et al., 2016). Therefore, treatment with mitochondrial division inhibitor-1 may decrease proliferation in HCC cells.
Esophageal Cancer
Esophageal cancer (EC) has a very high mortality rate in China. Casticin treatment plays a pivotal role in inhibiting proliferation and inducing apoptosis of EC cells through activation of JNK signaling pathway, and hesperetin induces apoptosis via increased intracellular reactive oxygen species (Wu et al., 2016; Qiao et al., 2019). A study of mitochondrial haplogroups and esophageal cancer (n = 30) in the Taihang Mountain and Chaoshan areas of China has shown that haplogroups D4a and D5 in Taihang Mountain, and haplogroups D and D5 in Chaoshan areas, were related to higher susceptibility to esophageal cancer (Li et al., 2007). Overall, haplogroup D, specifically sub-haplogroups D4a and D5a, can serve as potential biomarkers for esophageal cancer, at least in these two areas. Other papers showed that haplogroup D4a was associated with an increased risk of thyroid cancer (n = 100) in China (Fang et al., 2010). As previously mentioned, haplogroup D5 was also a risk factor of breast cancer. It appears that haplogroup D is a risk factor for a diverse group of diseases because it impairs mitochondrial OXPHOS.
Cardiovascular and Cerebrovascular Diseases
Cardiovascular and cerebrovascular diseases are major health problems worldwide, but Asian countries have higher mortality rates for stroke than Western countries, although these rates have recently decreased in Japan and urban areas in China. South Asian, but not East Asian countries have a higher mortality rate for coronary heart disease than Western countries (Zhang et al., 2007; Ueshima et al., 2008). There are about 290 million patients, or one in five adults, with cardiovascular or cerebrovascular diseases in China (Wallace, 2000).
Hypertension
Hypertension is one of the most common risk factors of cardiovascular disease, affecting ~168.1 million in China (Wang et al., 2019). It can be caused by both hereditary and genetic factors. Mutations in the mitochondrial genome are associated with essential hypertension; several mtDNA mutations associated with hypertension are found in haplogroup D4j (A4295G) (Li Z. et al., 2008), haplogroup G2a1 (A4435G) (Lu et al., 2011), and haplogroup B5b1 (T16189C) (Zhu et al., 2016). Mitochondrial dysfunction associated with increased ROS production may be involved in the pathogenesis of hypertension (Dikalov and Ungvari, 2013; Lahera et al., 2017). Lymphocyte cell lines with a tRNA(Met) C4467A mutation showed oxidative stress and mitochondrial biogenesis dysfunction, including lower ATP generation, MMP activity, and oxygen consumption, and increased ROS levels (Liu et al., 2017).
Myocardial and Cerebral Infarction
Myocardial and cerebral infarction are multifactorial disorders affected by both genetic and environmental conditions, such as coronary atherosclerosis (Sawabe et al., 2011), carotid artery stenosis (Iizuka et al., 2009), hypertrophic cardiomyopathy (Wei et al., 2009), and left ventricular non-compaction (Tang et al., 2010). Mitochondria-derived ROS plays a role in myocardial and cerebral infarction due to the fact that mitochondria in vascular endothelial cells are the major source of superoxide (Guzik et al., 2006). Therefore, it is important to determine the variants of mtDNA associated with myocardial and cerebral infarction. In the Japanese population, mtDNA C5178A transversion causes leucine to methionine substitution in ND2, resulting in anti-atherosclerotic effects in diabetic subjects and a lower prevalence of myocardial infarction (Takagi et al., 2004). The study found that mitochondrial haplogroup A contributes to atherothrombotic cerebral infarction (n = 1,181), but only in females. Haplogroup N9b protects against myocardial infarction; however, haplogroup G1 is a risk factor for this disease in Japanese males (Nishigaki et al., 2007a,b). Mitochondrial haplogroups A and M7a increase the risk for coronary atherosclerosis in a Japanese population (n = 1,536). Surprisingly, a haplogroup associated with extreme longevity, D4a, conferred a risk of myocardial infarction (Alexe et al., 2007; Cai et al., 2009; Sawabe et al., 2011). Inhibition of mitochondrial permeability transition improved functional recovery and reduced mortality following acute myocardial infarction in mice (Gomez et al., 2007). Therefore, targeting mitochondrial calcium transport and inhibiting mitochondrial fission may be effective strategies for myocardial infarction (Cooper and Eguchi, 2018; Frangogiannis, 2018).
Hypertrophic Cardiomyopathy
Hypertrophic cardiomyopathy (HCM) is a common genetic disorder, affecting 1 in 500 individuals worldwide (Maron et al., 2006). Mitochondrial haplogroup M10 may be a risk factor for HCM, specifically, three mutations - G7967A in the COX II of complex IV, and T12477C and G13135A in the ND5 of complex I. Mitochondrial complex I activity was markedly decreased in the HCM individuals, resulting in disrupted mitochondrial respiratory function (Wei et al., 2009). The mitochondrial ND5 T12338C variant which belongs to haplogroup F2a was associated with hypertrophic cardiomyopathy in a Chinese pedigree (Liu et al., 2012). A characteristic T2336C homoplasmic mutation in the mitochondrial 16S rRNA gene of HCM has also been found in a Chinese family. Reduced ATP and MMP levels, and increased ROS generation in the mutant 2336C cybrids may lead to deterioration of mitochondrial function (Li et al., 2018).
Left Ventricular Non-compaction
Left ventricular non-compaction (LVNC) is a genetically heterogeneous pathological disorder that is affected by both the nuclear and mitochondrial genomes, leading to congenital heart disease (Digilio et al., 1999; Xing et al., 2006). Tang et al. found that mtDNA A3397G and T3398C of the complex I ND1 subunit may disrupt mitochondrial function to initiate LVNC (Tang et al., 2010).
Stroke
Stroke is a complex multifactorial disorder caused by both genetics and environment in the Chinese population (Della-Morte et al., 2012). One study shows that mtDNA C5178A belongs to haplogroup D4b and is a protective factor for IS in the Chinese Han population (n = 200) (Yang et al., 2014). Other studies reported that m.5178C, but not m.5178A, is a risk factor for several diseases including myocardial infarction (n = 517), cerebrovascular diseases (n = 127), and diabetes (n = 270) (Wang et al., 2001; Ohkubo et al., 2002; Takagi et al., 2004). One explanation may be that the production of ROS in complex I disrupts the structure and respiratory chain function, and further damages the cardiovascular system. Rapamycin treatment attenuated mitochondrial dysfunction following cerebral ischemia, possibly through enhancement of mitophagy (Li et al., 2014).
Conclusions
In summary, the studies described in this review shed light on the pathogenesis of diseases associated with mitochondrial dysfunction. They provide biological plausibility for the observed epidemiological surveys, although a number of limitations must also be considered; notably, the specific mechanisms of disease require further investigation. An intriguing finding is that the same mitochondrial haplogroup can have negative or positive association with different diseases. These associations remain unclear, although haplogroups defined by using common SNPs in each ethnic group may be a potential explanation. The distribution of mtDNA allele frequency varies considerably with the populations studied, which implies that haplogroup analysis is insufficient across populations. The results suggest that there are mechanisms other than mitochondrial pathways that affect susceptibility to these diseases. Mitochondrial retrograde signaling pathways may have profound effects on nuclear-mitochondrial interactions in cellular morphology and functionality. A possible explanation is that the nucleus attempts to make more mitochondria compensate for the energy deficiency. Other complex networks including proteostasis, mitochondrial unfolded protein response (UPR) and ROS signaling may also play pivotal roles in the organism's metabolism and deserve future investigation.
Author Contributions
DS and H-XZ designed this review. DS searched literature and wrote the initial manuscript. YW made the table. JW and LJ supervised and provided critical comments on the manuscript. DS, YW, H-XZ, LJ, and JW read, amended, and discussed the article.
Funding
This study was supported by the grants from the National Science Foundation of China (31871436) and Shanghai Municipal Science and Technology Major Project (2017SHZDZX01).
Conflict of Interest
The authors declare that the research was conducted in the absence of any commercial or financial relationships that could be construed as a potential conflict of interest.
References
Alexe, G., Fuku, N., Bilal, E., Ueno, H., Nishigaki, Y., Fujita, Y., et al. (2007). Enrichment of longevity phenotype in mtDNA haplogroups D4b2b, D4a, and D5 in the Japanese population. Hum. Genet. 121, 347–356. doi: 10.1007/s00439-007-0330-6
Altieri, D. C. (2010). Mitochondrial Hsp90 chaperones as novel molecular targets in prostate cancer. Fut. Oncol. 6, 487–489. doi: 10.2217/fon.10.26
Baracca, A., Solaini, G., Sgarbi, G., Lenaz, G., Baruzzi, A., Schapira, A. H., et al. (2005). Severe impairment of complex I-driven adenosine triphosphate synthesis in leber hereditary optic neuropathy cybrids. Arch. Neurol. 62, 730–736. doi: 10.1001/archneur.62.5.730
Bi, R., Li, W. L., Chen, M. Q., Zhu, Z., and Yao, Y. G. (2011). Rapid identification of mtDNA somatic mutations in gastric cancer tissues based on the mtDNA phylogeny. Mutat. Res. 709–710, 15–20. doi: 10.1016/j.mrfmmm.2011.02.016
Bi, R., Tang, J., Zhang, W., Li, X., Chen, S. Y., Yu, D., et al. (2016). Mitochondrial genome variations and functional characterization in Han Chinese families with schizophrenia. Schizophr. Res. 171, 200–206. doi: 10.1016/j.schres.2016.01.011
Bi, R., Zhang, W., Yu, D., Li, X., Wang, H. Z., Hu, Q. X., et al. (2015). Mitochondrial DNA haplogroup B5 confers genetic susceptibility to Alzheimer's disease in Han Chinese. Neurobiol. Aging 36, 1604.e1607–1616. doi: 10.1016/j.neurobiolaging.2014.10.009
Brown, M. D., Trounce, I. A., Jun, A. S., Allen, J. C., and Wallace, D. C. (2000). Functional analysis of lymphoblast and cybrid mitochondria containing the 3460, 11778, or 14484 Leber's hereditary optic neuropathy mitochondrial DNA mutation. J. Biol. Chem. 275, 39831–39836. doi: 10.1074/jbc.M006476200
Bubber, P., Haroutunian, V., Fisch, G., Blass, J. P., and Gibson, G. E. (2005). Mitochondrial abnormalities in Alzheimer brain: mechanistic implications. Ann. Neurol. 57, 695–703. doi: 10.1002/ana.20474
Bubber, P., Tang, J., Haroutunian, V., Xu, H., Davis, K. L., Blass, J. P., et al. (2004). Mitochondrial enzymes in schizophrenia. J. Mol. Neurosci. 24, 315–321. doi: 10.1385/JMN:24:2:315
Buchner, D. A., Yazbek, S. N., Solinas, P., Burrage, L. C., Morgan, M. G., Hoppel, C. L., et al. (2011). Increased mitochondrial oxidative phosphorylation in the liver is associated with obesity and insulin resistance. Obesity 19, 917–924. doi: 10.1038/oby.2010.214
Cai, X. Y., Wang, X. F., Li, S. L., Qian, J., Qian, D. G., Chen, F., et al. (2009). Association of mitochondrial DNA haplogroups with exceptional longevity in a Chinese population. PLoS ONE 4:e6423. doi: 10.1371/journal.pone.0006423
Carvalho, C., Correia, S. C., Santos, R. X., Cardoso, S., Moreira, P. I., Clark, T. A., et al. (2009). Role of mitochondrial-mediated signaling pathways in Alzheimer disease and hypoxia. J. Bioenerg. Biomembr. 41, 433–440. doi: 10.1007/s10863-009-9247-1
Chaudhary, A. K., O'Malley, J., Kumar, S., Inigo, J. R., Kumar, R., Yadav, N., et al. (2017). Mitochondrial dysfunction and prostate cancer racial disparities among American men. Front. Biosci. 9, 154–164. doi: 10.2741/s479
Chen, J., Hattori, Y., Nakajima, K., Eizawa, T., Ehara, T., Koyama, M., et al. (2006). Mitochondrial complex I activity is significantly decreased in a patient with maternally inherited type 2 diabetes mellitus and hypertrophic cardiomyopathy associated with mitochondrial DNA C3310T mutation: a cybrid study. Diabetes Res. Clin. Pract. 74, 148–153. doi: 10.1016/j.diabres.2006.03.024
Chen, Y. F., Chen, W. J., Lin, X. Z., Zhang, Q. J., Cai, J. P., Liou, C. W., et al. (2015). Mitochondrial DNA haplogroups and the risk of sporadic parkinson's disease in han Chinese. Chin. Med. J. 128, 1748–1754. doi: 10.4103/0366-6999.159348
Chow, J., Rahman, J., Achermann, J. C., Dattani, M. T., and Rahman, S. (2017). Mitochondrial disease and endocrine dysfunction. Nat. Rev. Endocrinol. 13, 92–104. doi: 10.1038/nrendo.2016.151
Chu, C. T. (2010). A pivotal role for PINK1 and autophagy in mitochondrial quality control: implications for Parkinson disease. Hum. Mol. Genet. 19, R28–R37. doi: 10.1093/hmg/ddq143
Cooper, H. A., and Eguchi, S. (2018). Inhibition of mitochondrial fission as a novel therapeutic strategy to reduce mortality upon myocardial infarction. Clin. Sci. 132, 2163–2167. doi: 10.1042/CS20180671
Cormio, A., Musicco, C., Gasparre, G., Cormio, G., Pesce, V., Sardanelli, A. M., et al. (2017). Increase in proteins involved in mitochondrial fission, mitophagy, proteolysis and antioxidant response in type I endometrial cancer as an adaptive response to respiratory complex I deficiency. Biochem. Biophys. Res. Commun. 491, 85–90. doi: 10.1016/j.bbrc.2017.07.047
Dakubo, G. D., Parr, R. L., Costello, L. C., Franklin, R. B., and Thayer, R. E. (2006). Altered metabolism and mitochondrial genome in prostate cancer. J. Clin. Pathol. 59, 10–16. doi: 10.1136/jcp.2005.027664
Della-Morte, D., Guadagni, F., Palmirotta, R., Testa, G., Caso, V., Paciaroni, M., et al. (2012). Genetics of ischemic stroke, stroke-related risk factors, stroke precursors and treatments. Pharmacogenomics 13, 595–613. doi: 10.2217/pgs.12.14
Digilio, M. C., Marino, B., Bevilacqua, M., Musolino, A. M., Giannotti, A., and Dallapiccola, B. (1999). Genetic heterogeneity of isolated noncompaction of the left ventricular myocardium. Am. J. Med. Genet. 85, 90–91. doi: 10.1002/(SICI)1096-8628(19990702)85:1<90::AID-AJMG19>3.0.CO;2-U
Dikalov, S. I., and Ungvari, Z. (2013). Role of mitochondrial oxidative stress in hypertension. Am. J. Physiol. Heart Circ. Physiol. 305, H1417–H1427. doi: 10.1152/ajpheart.00089.2013
Ding, Y., Li, Y., You, J., Yang, L., Chen, B., Lu, J., et al. (2009). Mitochondrial tRNA(Glu) A14693G variant may modulate the phenotypic manifestation of deafness-associated 12S rRNA A1555G mutation in a Han Chinese family. J. Genet. Genomics 36, 241–250. doi: 10.1016/S1673-8527(08)60111-3
Dolle, C., Flones, I., Nido, G. S., Miletic, H., Osuagwu, N., Kristoffersen, S., et al. (2016). Defective mitochondrial DNA homeostasis in the substantia nigra in Parkinson disease. Nat. Commun. 7:13548. doi: 10.1038/ncomms13548
Dombi, E., Diot, A., Morten, K., Carver, J., Lodge, T., Fratter, C., et al. (2016). The m.13051G>A mitochondrial DNA mutation results in variable neurology and activated mitophagy. Neurology 86, 1921–1923. doi: 10.1212/WNL.0000000000002688
Ehrnhoefer, D. E., Southwell, A. L., Sivasubramanian, M., Qiu, X., Villanueva, E. B., Xie, Y., et al. (2018). HACE1 is essential for astrocyte mitochondrial function and influences Huntington disease phenotypes in vivo. Hum. Mol. Genet. 27, 239–253. doi: 10.1093/hmg/ddx394
Fakhruddin, S., Alanazi, W., and Jackson, K. E. (2017). Diabetes-induced reactive oxygen species: mechanism of their generation and role in renal injury. J. Diabetes Res. 2017:8379327. doi: 10.1155/2017/8379327
Fang, H., Hu, N., Zhao, Q., Wang, B., Zhou, H., Fu, Q., et al. (2018). mtDNA haplogroup N9a increases the risk of type 2 diabetes by altering mitochondrial function and intracellular mitochondrial signals. Diabetes 67, 1441–1453. doi: 10.2337/db17-0974
Fang, H., Liu, X., Shen, L., Li, F., Liu, Y., Chi, H., et al. (2014). Role of mtDNA haplogroups in the prevalence of knee osteoarthritis in a southern Chinese population. Int. J. Mol. Sci. 15, 2646–2659. doi: 10.3390/ijms15022646
Fang, H., Shen, L., Chen, T., He, J., Ding, Z., Wei, J., et al. (2010). Cancer type-specific modulation of mitochondrial haplogroups in breast, colorectal and thyroid cancer. BMC Cancer 10:421. doi: 10.1186/1471-2407-10-421
Fang, H., Shi, H., Li, X., Sun, D., Li, F., Li, B., et al. (2015). Exercise intolerance and developmental delay associated with a novel mitochondrial ND5 mutation. Sci. Rep. 5:10480. doi: 10.1038/srep10480
Fang, Y., Yang, H. Y., Shi, Y. H., Cui, J. H., Li, L. Y., Xu, Y. C., et al. (2015). Mitochondrial DNA haplogroups and somatic mutations are associated with lung cancer in patients from Southwest China. Genet. Mol. Res. 14, 5031–5043. doi: 10.4238/2015.May.12.6
Feng, G. F., Zhang, J., Feng, L. M., Shen, N. X., Li, L. J., and Zhu, Y. M. (2013). Mitochondrial DNA haplogroup associated with sperm motility in the Han population. Asian J. Androl. 15, 630–633. doi: 10.1038/aja.2013.83
Feng, J., Zhang, J., Liu, M., Wan, G., Qi, K., Zheng, C., et al. (2011). Association of mtDNA haplogroup F with healthy longevity in the female Chuang population, China. Exp. Gerontol. 46, 987–993. doi: 10.1016/j.exger.2011.09.001
Feng, Y., Wang, Y., Jiang, C., Fang, Z., Zhang, Z., Lin, X., et al. (2017). Nicotinamide induces mitochondrial-mediated apoptosis through oxidative stress in human cervical cancer HeLa cells. Life Sci. 181, 62–69. doi: 10.1016/j.lfs.2017.06.003
Ferlin, A., Raicu, F., Gatta, V., Zuccarello, D., Palka, G., and Foresta, C. (2007). Male infertility: role of genetic background. Reprod. Biomed. Online 14, 734–745. doi: 10.1016/S1472-6483(10)60677-3
Ferramosca, A., Provenzano, S. P., Coppola, L., and Zara, V. (2012). Mitochondrial respiratory efficiency is positively correlated with human sperm motility. Urology 79, 809–814. doi: 10.1016/j.urology.2011.12.042
Flippo, K. H., and Strack, S. (2017). An emerging role for mitochondrial dynamics in schizophrenia. Schizophr. Res. 187, 26–32. doi: 10.1016/j.schres.2017.05.003
Floreani, M., Napoli, E., Martinuzzi, A., Pantano, G., De Riva, V., Trevisan, R., et al. (2005). Antioxidant defences in cybrids harboring mtDNA mutations associated with Leber's hereditary optic neuropathy. FEBS J. 272, 1124–1135. doi: 10.1111/j.1742-4658.2004.04542.x
Forbes, J. M., and Thorburn, D. R. (2018). Mitochondrial dysfunction in diabetic kidney disease. Nat. Rev. Nephrol. 14, 291–312. doi: 10.1038/nrneph.2018.9
Frangogiannis, N. G. (2018). Targeting mitochondrial calcium transport in myocardial infarction. Hellenic J. Cardiol. 59, 223–225. doi: 10.1016/j.hjc.2018.06.010
Fuku, N., Park, K. S., Yamada, Y., Nishigaki, Y., Cho, Y. M., Matsuo, H., et al. (2007). Mitochondrial haplogroup N9a confers resistance against type 2 diabetes in Asians. Am. J. Hum. Genet. 80, 407–415. doi: 10.1086/512202
Ghelli, A., Zanna, C., Porcelli, A. M., Schapira, A. H., Martinuzzi, A., Carelli, V., et al. (2003). Leber's hereditary optic neuropathy (LHON) pathogenic mutations induce mitochondrial-dependent apoptotic death in transmitochondrial cells incubated with galactose medium. J. Biol. Chem. 278, 4145–4150. doi: 10.1074/jbc.M210285200
Giorgi, E. E., Li, Y., Caberto, C. P., Beckman, K. B., Lum-Jones, A., Haiman, C. A., et al. (2016). No association between the mitochondrial genome and prostate cancer risk: the multiethnic cohort. Cancer Epidemiol. Biomarkers Prev. 25, 1001–1003. doi: 10.1158/1055-9965.EPI-16-0111
Gomez, L., Thibault, H., Gharib, A., Dumont, J. M., Vuagniaux, G., Scalfaro, P., et al. (2007). Inhibition of mitochondrial permeability transition improves functional recovery and reduces mortality following acute myocardial infarction in mice. Am. J. Physiol. Heart Circ. Physiol. 293, H1654–H1661. doi: 10.1152/ajpheart.01378.2006
Guzik, T. J., Sadowski, J., Guzik, B., Jopek, A., Kapelak, B., Przybylowski, P., et al. (2006). Coronary artery superoxide production and nox isoform expression in human coronary artery disease. Arterioscler. Thromb. Vasc. Biol. 26, 333–339. doi: 10.1161/01.ATV.0000196651.64776.51
Haghighatfard, A., Andalib, S., Amini Faskhodi, M., Sadeghi, S., Ghaderi, A. H., Moradkhani, S., et al. (2017). Gene expression study of mitochondrial complex I in schizophrenia and paranoid personality disorder. World J. Biol. Psychiatry 19(Sup3):S133–S146. doi: 10.1080/15622975.2017.1282171
Han, S. Y., Jeong, Y. J., Choi, Y., Hwang, S. K., Bae, Y. S., and Chang, Y. C. (2018). Mitochondrial dysfunction induces the invasive phenotype, and cell migration and invasion, through the induction of AKT and AMPK pathways in lung cancer cells. Int. J. Mol. Med. 42, 1644–1652. doi: 10.3892/ijmm.2018.3733
Hao, X. D., Yang, Y. L., Tang, N. L., Kong, Q. P., Wu, S. F., and Zhang, Y. P. (2013). Mitochondrial DNA haplogroup Y is associated to Leigh syndrome in Chinese population. Gene 512, 460–463. doi: 10.1016/j.gene.2012.10.054
Haschemi, A., Kosma, P., Gille, L., Evans, C. R., Burant, C. F., Starkl, P., et al. (2012). The sedoheptulose kinase CARKL directs macrophage polarization through control of glucose metabolism. Cell Metab. 15, 813–826. doi: 10.1016/j.cmet.2012.04.023
Hattori, Y., Takeoka, M., Nakajima, K., Ehara, T., and Koyama, M. (2005). A heteroplasmic mitochondrial DNA 3310 mutation in the ND1 gene in a patient with type 2 diabetes, hypertrophic cardiomyopathy, and mental retardation. Exp. Clin. Endocrinol. Diabetes 113, 318–323. doi: 10.1055/s-2005-865646
Hauptmann, S., Scherping, I., Drose, S., Brandt, U., Schulz, K. L., Jendrach, M., et al. (2009). Mitochondrial dysfunction: an early event in Alzheimer pathology accumulates with age in AD transgenic mice. Neurobiol. Aging 30, 1574–1586. doi: 10.1016/j.neurobiolaging.2007.12.005
Hjelm, B. E., Rollins, B., Mamdani, F., Lauterborn, J. C., Kirov, G., Lynch, G., et al. (2015). Evidence of mitochondrial dysfunction within the complex genetic etiology of schizophrenia. Mol. Neuropsychiatr. 1, 201–219. doi: 10.1159/000441252
Holper, L., Ben-Shachar, D., and Mann, J. J. (2019). Multivariate meta-analyses of mitochondrial complex I and IV in major depressive disorder, bipolar disorder, schizophrenia, Alzheimer disease, and Parkinson disease. Neuropsychopharmacology 44, 837–849. doi: 10.1038/s41386-018-0090-0
Holt, I. J., Harding, A. E., and Morgan-Hughes, J. A. (1988). Deletions of muscle mitochondrial DNA in patients with mitochondrial myopathies. Nature 331, 717–719. doi: 10.1038/331717a0
Hong Bin, W., Da, L. H., Xue, Y., and Jing, B. (2018). Pterostilbene (3′,5′-dimethoxy-resveratrol) exerts potent antitumor effects in HeLa human cervical cancer cells via disruption of mitochondrial membrane potential, apoptosis induction and targeting m-TOR/PI3K/Akt signalling pathway. J. Buon. 23, 1384–1389.
Hong, J., Samudio, I., Chintharlapalli, S., and Safe, S. (2008). 1,1-bis(3′-indolyl)-1-(p-substituted phenyl)methanes decrease mitochondrial membrane potential and induce apoptosis in endometrial and other cancer cell lines. Mol. Carcinog. 47, 492–507. doi: 10.1002/mc.20407
Hoti, N., Ma, J., Tabassum, S., Wang, Y., and Wu, M. (2003). Triphenyl tin benzimidazolethiol, a novel antitumor agent, induces mitochondrial-mediated apoptosis in human cervical cancer cells via suppression of HPV-18 encoded E6. J. Biochem. 134, 521–528. doi: 10.1093/jb/mvg169
Hotta, Y., Fujiki, K., Hayakawa, M., Nakajima, A., Kanai, A., Mashima, Y., et al. (1995). Clinical features of Japanese Leber's hereditary optic neuropathy with 11778 mutation of mitochondrial DNA. Jpn. J. Ophthalmol. 39, 96–108.
Hu, S. P., Du, J. P., Li, D. R., and Yao, Y. G. (2014). Mitochondrial DNA haplogroup confers genetic susceptibility to nasopharyngeal carcinoma in Chaoshanese from Guangdong, China. PLoS ONE 9:e87795. doi: 10.1371/journal.pone.0087795
Huang, S. C., Everts, B., Ivanova, Y., O'Sullivan, D., Nascimento, M., Smith, A. M., et al. (2014). Cell-intrinsic lysosomal lipolysis is essential for alternative activation of macrophages. Nat. Immunol. 15, 846–855. doi: 10.1038/ni.2956
Hwang, I. W., Kwon, B. N., Kim, H. J., Han, S. H., Lee, N. R., Lim, M. H., et al. (2018). Assessment of associations between mitochondrial DNA haplogroups and attention deficit and hyperactivity disorder in Korean children. Mitochondrion 47, 174–178. doi: 10.1016/j.mito.2018.11.003
Iizuka, T., Goto, Y., Miyakawa, S., Sato, M., Wang, Z., Suzuki, K., et al. (2009). Progressive carotid artery stenosis with a novel tRNA phenylalanine mitochondrial DNA mutation. J. Neurol. Sci. 278, 35–40. doi: 10.1016/j.jns.2008.11.016
Ji, J., Xu, M., Wang, R., Wang, Y., Qin, Y., Li, L., et al. (2018). Human mitochondrial DNA haplogroup M8a influences the penetrance of m.8684C>T in Han Chinese men with non-obstructive azoospermia. Reprod. Biomed. Online 37, 480–488. doi: 10.1016/j.rbmo.2018.08.004
Ji, Y., Liang, M., Zhang, J., Zhang, M., Zhu, J., Meng, X., et al. (2014). Mitochondrial haplotypes may modulate the phenotypic manifestation of the LHON-associated ND1 G3460A mutation in Chinese families. J. Hum. Genet. 59, 134–140. doi: 10.1038/jhg.2013.134
Ji, Y., Zhang, A. M., Jia, X., Zhang, Y. P., Xiao, X., Li, S., et al. (2008). Mitochondrial DNA haplogroups M7b1'2 and M8a affect clinical expression of leber hereditary optic neuropathy in Chinese families with the m.11778G–>a mutation. Am. J. Hum. Genet. 83, 760–768. doi: 10.1016/j.ajhg.2008.11.002
Jiang, H., Shao, X., Jia, S., Qu, L., Weng, C., Shen, X., et al. (2019). The mitochondria-targeted metabolic tubular injury in diabetic kidney disease. Cell. Physiol. Biochem. 52, 156–171. doi: 10.33594/000000011
Jiang, W., Li, R., Zhang, Y., Wang, P., Wu, T., Lin, J., et al. (2017). Mitochondrial DNA mutations associated with type 2 diabetes mellitus in Chinese Uyghur population. Sci. Rep. 7:16989. doi: 10.1038/s41598-017-17086-7
Jin, Z., Wei, W., Yang, M., Du, Y., and Wan, Y. (2014). Mitochondrial complex I activity suppresses inflammation and enhances bone resorption by shifting macrophage-osteoclast polarization. Cell Metab. 20, 483–498. doi: 10.1016/j.cmet.2014.07.011
Kabekkodu, S. P., Bhat, S., Mascarenhas, R., Mallya, S., Bhat, M., Pandey, D., et al. (2014). Mitochondrial DNA variation analysis in cervical cancer. Mitochondrion 16, 73–82. doi: 10.1016/j.mito.2013.07.001
Kasai, T., Ogawa, K., Mizuno, K., Nagai, S., Uchida, Y., Ohta, S., et al. (2002). Relationship between sperm mitochondrial membrane potential, sperm motility, and fertility potential. Asian J. Androl. 4, 97–103.
Kato, T., Fuku, N., Noguchi, Y., Murakami, H., Miyachi, M., Kimura, Y., et al. (2012). Mitochondrial DNA haplogroup associated with hereditary hearing loss in a Japanese population. Acta. Otolaryngol. 132, 1178–1182. doi: 10.3109/00016489.2012.693624.
Khasawneh, J., Schulz, M. D., Walch, A., Rozman, J., Hrabe de Angelis, M., Klingenspor, M., et al. (2009). Inflammation and mitochondrial fatty acid beta-oxidation link obesity to early tumor promotion. Proc. Natl. Acad. Sci. U.S.A. 106, 3354–3359. doi: 10.1073/pnas.0802864106
Kim, H. R., Kang, M. G., Lee, Y. E., Na, B. R., Noh, M. S., Yang, S. H., et al. (2018). Spectrum of mitochondrial genome instability and implication of mitochondrial haplogroups in Korean patients with acute myeloid leukemia. Blood Res. 53, 240–249. doi: 10.5045/br.2018.53.3.240
Kim, W., Yoo, T. K., Shin, D. J., Rho, H. W., Jin, H. J., Kim, E. T., et al. (2008). Mitochondrial DNA haplogroup analysis reveals no association between the common genetic lineages and prostate cancer in the Korean population. PLoS ONE 3:e2211. doi: 10.1371/journal.pone.0002211
King, M. P., and Attardi, G. (1989). Human cells lacking mtDNA: repopulation with exogenous mitochondria by complementation. Science 246, 500–503. doi: 10.1126/science.2814477
Kong, Q. P., Bandelt, H. J., Sun, C., Yao, Y. G., Salas, A., Achilli, A., et al. (2006). Updating the East Asian mtDNA phylogeny: a prerequisite for the identification of pathogenic mutations. Hum. Mol. Genet. 15, 2076–2086. doi: 10.1093/hmg/ddl130
Koo, B. S., Song, Y., Lee, S., Sung, Y. K., Shin, K. J., Cho, N. H., et al. (2019). Association of Asian mitochondrial DNA haplogroup B with new development of knee osteoarthritis in Koreans. Int. J. Rheum. Dis. 22, 411–416. doi: 10.1111/1756-185X.13453
Kwak, S. H., and Park, K. S. (2016). Role of mitochondrial DNA variation in the pathogenesis of diabetes mellitus. Front. Biosci. 21, 1151–1167. doi: 10.2741/4447
Lahera, V., de Las Heras, N., Lopez-Farre, A., Manucha, W., and Ferder, L. (2017). Role of mitochondrial dysfunction in hypertension and obesity. Curr. Hypertens. Rep. 19:11. doi: 10.1007/s11906-017-0710-9
Lai, C. H., Huang, S. F., Chen, I. H., Liao, C. T., Wang, H. M., and Hsieh, L. L. (2012). The mitochondrial DNA Northeast Asia CZD haplogroup is associated with good disease-free survival among male oral squamous cell carcinoma patients. PLoS ONE 7:e49684. doi: 10.1371/journal.pone.0049684
Lennon, F. E., and Salgia, R. (2014). Mitochondrial dynamics: biology and therapy in lung cancer. Exp. Opin. Investig. Drugs 23, 675–692. doi: 10.1517/13543784.2014.899350
Levine, B., and Kroemer, G. (2008). Autophagy in the pathogenesis of disease. Cell 132, 27–42. doi: 10.1016/j.cell.2007.12.018
Li, D., Sun, Y., Zhuang, Q., Song, Y., Wu, B., Jia, Z., et al. (2018). Mitochondrial dysfunction caused by m.2336T>C mutation with hypertrophic cardiomyopathy in cybrid cell lines. Mitochondrion 46, 313–320. doi: 10.1016/j.mito.2018.08.005
Li, F. X., Ji, F. Y., Zheng, S. Z., Yao, W., Xiao, Z. L., and Qian, G. S. (2011). MtDNA haplogroups M7 and B in southwestern Han Chinese at risk for acute mountain sickness. Mitochondrion 11, 553–558. doi: 10.1016/j.mito.2011.02.003
Li, H., Jiao, S., Li, X., Banu, H., Hamal, S., and Wang, X. (2017). Therapeutic effects of antibiotic drug mefloquine against cervical cancer through impairing mitochondrial function and inhibiting mTOR pathway. Can. J. Physiol. Pharmacol. 95, 43–50. doi: 10.1139/cjpp-2016-0124
Li, M. Z., Yu, D. M., Yu, P., Liu, D. M., Wang, K., and Tang, X. Z. (2008). Mitochondrial gene mutations and type 2 diabetes in Chinese families. Chin. Med. J. 121, 682–686. doi: 10.1097/00029330-200804020-00004
Li, Q., Zhang, T., Wang, J., Zhang, Z., Zhai, Y., Yang, G. Y., et al. (2014). Rapamycin attenuates mitochondrial dysfunction via activation of mitophagy in experimental ischemic stroke. Biochem. Biophys. Res. Commun. 444, 182–188. doi: 10.1016/j.bbrc.2014.01.032
Li, W., Wen, C., Li, W., Wang, H., Guan, X., Zhang, W., et al. (2015). The tRNA(Gly) T10003C mutation in mitochondrial haplogroup M11b in a Chinese family with diabetes decreases the steady-state level of tRNA(Gly), increases aberrant reactive oxygen species production, and reduces mitochondrial membrane potential. Mol. Cell. Biochem. 408, 171–179. doi: 10.1007/s11010-015-2493-0
Li, X. Y., Su, M., Huang, H. H., Li, H., Tian, D. P., and Gao, Y. X. (2007). mtDNA evidence: genetic background associated with related populations at high risk for esophageal cancer between Chaoshan and Taihang Mountain areas in China. Genomics 90, 474–481. doi: 10.1016/j.ygeno.2007.06.006
Li, Y., Li, X., Wang, Z., Feng, Z., Li, L., and Ke, X. (2016). Subhaplogroup D4b1 enhances the risk of cervical cancer initiation: a case-control study in southern China. J. Obstet. Gynaecol. Res. 42, 325–330. doi: 10.1111/jog.12879
Li, Z., Liu, Y., Yang, L., Wang, S., and Guan, M. X. (2008). Maternally inherited hypertension is associated with the mitochondrial tRNA(Ile) A4295G mutation in a Chinese family. Biochem. Biophys. Res. Commun. 367, 906–911. doi: 10.1016/j.bbrc.2007.12.150
Liao, W. Q., Pang, Y., Yu, C. A., Wen, J. Y., Zhang, Y. G., and Li, X. H. (2008). Novel mutations of mitochondrial DNA associated with type 2 diabetes in Chinese Han population. Tohoku J. Exp. Med. 215, 377–384. doi: 10.1620/tjem.215.377
Liao, Z., Zhao, J., Zhu, Y., Yang, L., Yang, A., Sun, D., et al. (2007). The ND4 G11696A mutation may influence the phenotypic manifestation of the deafness-associated 12S rRNA A1555G mutation in a four-generation Chinese family. Biochem. Biophys. Res. Commun. 362, 670–676. doi: 10.1016/j.bbrc.2007.08.034
Lindqvist, D., Wolkowitz, O. M., Picard, M., Ohlsson, L., Bersani, F. S., Fernstrom, J., et al. (2018). Circulating cell-free mitochondrial DNA, but not leukocyte mitochondrial DNA copy number, is elevated in major depressive disorder. Neuropsychopharmacology 43, 1557–1564. doi: 10.1038/s41386-017-0001-9
Liou, C. W., Chen, J. B., Tiao, M. M., Weng, S. W., Huang, T. L., Chuang, J. H., et al. (2012). Mitochondrial DNA coding and control region variants as genetic risk factors for type 2 diabetes. Diabetes 61, 2642–2651. doi: 10.2337/db11-1369
Liou, C. W., Chuang, J. H., Chen, J. B., Tiao, M. M., Wang, P. W., Huang, S. T., et al. (2016). Mitochondrial DNA variants as genetic risk factors for Parkinson disease. Eur. J. Neurol. 23, 1289–1300. doi: 10.1111/ene.13020
Liu, H., Li, S., Liu, X., Chen, Y., and Deng, H. (2018). SIRT3 overexpression inhibits growth of kidney tumor cells and enhances mitochondrial biogenesis. J. Proteome Res. 17, 3143–3152. doi: 10.1021/acs.jproteome.8b00260
Liu, X. L., Zhou, X., Zhou, J., Zhao, F., Zhang, J., Li, C., et al. (2011). Leber's hereditary optic neuropathy is associated with the T12338C mutation in mitochondrial ND5 gene in six Han Chinese families. Ophthalmology 118, 978–985. doi: 10.1016/j.ophtha.2010.09.003
Liu, Y., Gao, L., Li, Y., Li, Z., Xu, H., Wang, L., et al. (2009). Voltage-dependent anion channel (VDAC) is involved in apoptosis of cell lines carrying the mitochondrial DNA mutation. BMC Med. Genet. 10:114. doi: 10.1186/1471-2350-10-114
Liu, Y., Li, Y., Zhu, C., Tian, L., Guan, M., and Chen, Y. (2017). Mitochondrial biogenesis dysfunction and metabolic dysfunction from a novel mitochondrial tRNA(Met) 4467 C>A mutation in a Han Chinese family with maternally inherited hypertension. Sci. Rep. 7:3034. doi: 10.1038/s41598-017-03303-w
Liu, Z., Song, Y., Gu, S., He, X., Zhu, X., Shen, Y., et al. (2012). Mitochondrial ND5 12338T>C variant is associated with maternally inherited hypertrophic cardiomyopathy in a Chinese pedigree. Gene 506, 339–343. doi: 10.1016/j.gene.2012.06.071
Loo, J. H., Trejaut, J. A., Yen, J. C., Chen, Z. S., Ng, W. M., Huang, C. Y., et al. (2014). Mitochondrial DNA association study of type 2 diabetes with or without ischemic stroke in Taiwan. BMC Res. 7:223. doi: 10.1186/1756-0500-7-223
Lu, J., Qian, Y., Li, Z., Yang, A., Zhu, Y., Li, R., et al. (2010). Mitochondrial haplotypes may modulate the phenotypic manifestation of the deafness-associated 12S rRNA 1555A>G mutation. Mitochondrion 10, 69–81. doi: 10.1016/j.mito.2009.09.007
Lu, M. Y., Huang, J. F., Liao, Y. C., Bai, R. K., Trieu, R. B., Chuang, W. L., et al. (2012). Mitochondrial polymorphism 12361A>G is associated with nonalcoholic fatty liver disease. Transl. Res. 159, 58–59. doi: 10.1016/j.trsl.2011.10.011.
Lu, Z., Chen, H., Meng, Y., Wang, Y., Xue, L., Zhi, S., et al. (2011). The tRNAMet 4435A>G mutation in the mitochondrial haplogroup G2a1 is responsible for maternally inherited hypertension in a Chinese pedigree. Eur. J. Hum. Genet. 19, 1181–1186. doi: 10.1038/ejhg.2011.111
Lyu, L., Wang, Q., Song, S., Li, L., Zhou, H., Li, M., et al. (2019). Oncocytic tumors are marked by enhanced mitochondrial content and mtDNA mutations of complex I in Chinese patients. Mitochondrion 45, 1–6. doi: 10.1016/j.mito.2018.01.008
Ma, L., Fu, Q., Xu, B., Zhou, H., Gao, J., Shao, X., et al. (2018). Breast cancer-associated mitochondrial DNA haplogroup promotes neoplastic growth via ROS-mediated AKT activation. Int. J. Cancer 142, 1786–1796. doi: 10.1002/ijc.31207
Ma, X., Cui, Y., Zhou, H., and Li, Q. (2018). Function of mitochondrial pyruvate carriers in hepatocellular carcinoma patients. Oncol. Lett. 15, 9110–9116. doi: 10.3892/ol.2018.8466
Madsen-Bouterse, S. A., Zhong, Q., Mohammad, G., Ho, Y. S., and Kowluru, R. A. (2010). Oxidative damage of mitochondrial DNA in diabetes and its protection by manganese superoxide dismutase. Free Radic. Res. 44, 313–321. doi: 10.3109/10715760903494168
Marchetti, C., Obert, G., Deffosez, A., Formstecher, P., and Marchetti, P. (2002). Study of mitochondrial membrane potential, reactive oxygen species, DNA fragmentation and cell viability by flow cytometry in human sperm. Hum. Reprod. 17, 1257–1265. doi: 10.1093/humrep/17.5.1257
Maron, B. J., Towbin, J. A., Thiene, G., Antzelevitch, C., Corrado, D., Arnett, D., et al. (2006). Contemporary definitions and classification of the cardiomyopathies: an American Heart Association Scientific Statement from the Council on Clinical Cardiology, Heart Failure and Transplantation Committee; Quality of Care and Outcomes Research and Functional Genomics and Translational Biology Interdisciplinary Working Groups; and Council on Epidemiology and Prevention. Circulation 113, 1807–1816. doi: 10.1161/CIRCULATIONAHA.106.174287
Mizumachi, T., Muskhelishvili, L., Naito, A., Furusawa, J., Fan, C. Y., Siegel, E. R., et al. (2008). Increased distributional variance of mitochondrial DNA content associated with prostate cancer cells as compared with normal prostate cells. Prostate 68, 408–417. doi: 10.1002/pros.20697
Moore, F. L., and Reijo-Pera, R. A. (2000). Male sperm motility dictated by mother's mtDNA. Am. J. Hum. Genet. 67, 543–548. doi: 10.1086/303061
Munakata, K., Tanaka, M., Mori, K., Washizuka, S., Yoneda, M., Tajima, O., et al. (2004). Mitochondrial DNA 3644T–>C mutation associated with bipolar disorder. Genomics 84, 1041–1050. doi: 10.1016/j.ygeno.2004.08.015
Nakano, S., Fukuda, M., Hotta, F., Ito, T., Ishii, T., Kitazawa, M., et al. (1998). Mitochondrial DNA point mutation at nucleotide pair 3316 in a Japanese family with heterogeneous phenotypes of diabetes. Endocr. J. 45, 625–630. doi: 10.1507/endocrj.45.625
Nishigaki, Y., Fuku, N., and Tanaka, M. (2010). Mitochondrial haplogroups associated with lifestyle-related diseases and longevity in the Japanese population. Geriatr. Gerontol. Int. 10 (Suppl 1), S221–235. doi: 10.1111/j.1447-0594.2010.00599.x
Nishigaki, Y., Yamada, Y., Fuku, N., Matsuo, H., Segawa, T., Watanabe, S., et al. (2007a). Mitochondrial haplogroup A is a genetic risk factor for atherothrombotic cerebral infarction in Japanese females. Mitochondrion 7, 72–79. doi: 10.1016/j.mito.2006.11.002
Nishigaki, Y., Yamada, Y., Fuku, N., Matsuo, H., Segawa, T., Watanabe, S., et al. (2007b). Mitochondrial haplogroup N9b is protective against myocardial infarction in Japanese males. Hum. Genet. 120, 827–836. doi: 10.1007/s00439-006-0269-z
Niu, Q., Zhang, W., Wang, H., Guan, X., Lu, J., and Li, W. (2015). Effects of mitochondrial haplogroup N9a on type 2 diabetes mellitus and its associated complications. Exp. Ther. Med. 10, 1918–1924. doi: 10.3892/etm.2015.2751
Ohkubo, R., Nakagawa, M., Ikeda, K., Kodama, T., Arimura, K., Akiba, S., et al. (2002). Cerebrovascular disorders and genetic polymorphisms: mitochondrial DNA5178C is predominant in cerebrovascular disorders. J. Neurol. Sci. 198, 31–35. doi: 10.1016/S0022-510X(02)00055-2
Pelicano, H., Zhang, W., Liu, J., Hammoudi, N., Dai, J., Xu, R. H., et al. (2014). Mitochondrial dysfunction in some triple-negative breast cancer cell lines: role of mTOR pathway and therapeutic potential. Breast Cancer Res. 16:434. doi: 10.1186/s13058-014-0434-6
Pello, R., Martin, M. A., Carelli, V., Nijtmans, L. G., Achilli, A., Pala, M., et al. (2008). Mitochondrial DNA background modulates the assembly kinetics of OXPHOS complexes in a cellular model of mitochondrial disease. Hum. Mol. Genet. 17, 4001–4011. doi: 10.1093/hmg/ddn303
Pera, M., Larrea, D., Guardia-Laguarta, C., Montesinos, J., Velasco, K. R., Agrawal, R. R., et al. (2017). Increased localization of APP-C99 in mitochondria-associated ER membranes causes mitochondrial dysfunction in Alzheimer disease. EMBO J. 36, 3356–3371. doi: 10.15252/embj.201796797
Pereira, C., Chavarria, V., Vian, J., Ashton, M. M., Berk, M., Marx, W., et al. (2018). Mitochondrial agents for bipolar disorder. Int. J. Neuropsychopharmacol. 21, 550–569. doi: 10.1093/ijnp/pyy018
Piekutowska-Abramczuk, D., Assouline, Z., Matakovic, L., Feichtinger, R. G., Konarikova, E., Jurkiewicz, E., et al. (2018). NDUFB8 mutations cause mitochondrial complex I deficiency in Individuals with leigh-like encephalomyopathy. Am. J. Hum. Genet. 102, 460–467. doi: 10.1016/j.ajhg.2018.01.008
Puthumana, J. S., and Regenold, W. T. (2019). Glucose-6-phosphate dehydrogenase activity in bipolar disorder and schizophrenia: relationship to mitochondrial impairment. J. Psychiatr. Res. 112, 99–103. doi: 10.1016/j.jpsychires.2019.03.004
Qi, W., Keenan, H. A., Li, Q., Ishikado, A., Kannt, A., Sadowski, T., et al. (2017). Pyruvate kinase M2 activation may protect against the progression of diabetic glomerular pathology and mitochondrial dysfunction. Nat. Med. 23, 753–762. doi: 10.1038/nm.4328
Qian, Y., Zhou, X., Hu, Y., Tong, Y., Li, R., Lu, F., et al. (2005). Clinical evaluation and mitochondrial DNA sequence analysis in three Chinese families with Leber's hereditary optic neuropathy. Biochem. Biophys. Res. Commun. 332, 614–621. doi: 10.1016/j.bbrc.2005.05.003
Qiao, Z., Cheng, Y., Liu, S., Ma, Z., Li, S., and Zhang, W. (2019). Casticin inhibits esophageal cancer cell proliferation and promotes apoptosis by regulating mitochondrial apoptotic and JNK signaling pathways. Naunyn Schmiedebergs. Arch. Pharmacol. 392, 177–187. doi: 10.1007/s00210-018-1574-5
Qu, J., Li, R., Zhou, X., Tong, Y., Lu, F., Qian, Y., et al. (2006). The novel A4435G mutation in the mitochondrial tRNAMet may modulate the phenotypic expression of the LHON-associated ND4 G11778A mutation. Invest. Ophthalmol. Vis. Sci. 47, 475–483. doi: 10.1167/iovs.05-0665
Qu, J., Wang, Y., Tong, Y., Zhou, X., Zhao, F., Yang, L., et al. (2010). Leber's hereditary optic neuropathy affects only female matrilineal relatives in two Chinese families. Invest. Ophthalmol. Vis. Sci. 51, 4906–4912. doi: 10.1167/iovs.09-5027
Rehman, J., Zhang, H. J., Toth, P. T., Zhang, Y., Marsboom, G., Hong, Z., et al. (2012). Inhibition of mitochondrial fission prevents cell cycle progression in lung cancer. FASEB J. 26, 2175–2186. doi: 10.1096/fj.11-196543
Rogge, M. M. (2009). The role of impaired mitochondrial lipid oxidation in obesity. Biol. Res. Nurs. 10, 356–373. doi: 10.1177/1099800408329408
Roglic, G., and Unwin, N. (2010). Mortality attributable to diabetes: estimates for the year 2010. Diabetes Res. Clin. Pract. 87, 15–19. doi: 10.1016/j.diabres.2009.10.006
Rollins, B. L., Morgan, L., Hjelm, B. E., Sequeira, A., Schatzberg, A. F., Barchas, J. D., et al. (2018). Mitochondrial complex I deficiency in Schizophrenia and bipolar disorder and medication influence. Mol. Neuropsychiatr. 3, 157–169. doi: 10.1159/000484348
Ruiz-Pesini, E., Lapena, A. C., Diez-Sanchez, C., Perez-Martos, A., Montoya, J., Alvarez, E., et al. (2000). Human mtDNA haplogroups associated with high or reduced spermatozoa motility. Am. J. Hum. Genet. 67, 682–696. doi: 10.1086/303040
Santidrian, A. F., Matsuno-Yagi, A., Ritland, M., Seo, B. B., LeBoeuf, S. E., Gay, L. J., et al. (2013). Mitochondrial complex I activity and NAD+/NADH balance regulate breast cancer progression. J. Clin. Invest. 123, 1068–1081. doi: 10.1172/JCI64264
Sawabe, M., Tanaka, M., Chida, K., Arai, T., Nishigaki, Y., Fuku, N., et al. (2011). Mitochondrial haplogroups A and M7a confer a genetic risk for coronary atherosclerosis in the Japanese elderly: an autopsy study of 1,536 patients. J. Atheroscler. Thromb. 18, 166–175. doi: 10.5551/jat.6742
Schrauwen, P., and Hesselink, M. K. (2004). Oxidative capacity, lipotoxicity, and mitochondrial damage in type 2 diabetes. Diabetes 53, 1412–1417. doi: 10.2337/diabetes.53.6.1412
Sharma, L. K., Tiwari, M., Rai, N. K., and Bai, Y. (2019). Mitophagy activation repairs Leber's hereditary optic neuropathy-associated mitochondrial dysfunction and improves cell survival. Hum. Mol. Genet. 28, 422–433. doi: 10.1093/hmg/ddy354
Shenouda, S. M., Widlansky, M. E., Chen, K., Xu, G., Holbrook, M., Tabit, C. E., et al. (2011). Altered mitochondrial dynamics contributes to endothelial dysfunction in diabetes mellitus. Circulation 124, 444–453. doi: 10.1161/CIRCULATIONAHA.110.014506
Shi, W. Y., Cao, C., and Liu, L. (2016). Interferon α induces the apoptosis of cervical cancer HeLa cells by activating both the intrinsic mitochondrial pathway and endoplasmic reticulum stress-induced pathway. Int. J. Mol. Sci. 17:1832. doi: 10.3390/ijms17111832
Shoffner, J. M., Lott, M. T., Lezza, A. M., Seibel, P., Ballinger, S. W., and Wallace, D. C. (1990). Myoclonic epilepsy and ragged-red fiber disease (MERRF) is associated with a mitochondrial DNA tRNA(Lys) mutation. Cell 61, 931–937. doi: 10.1016/0092-8674(90)90059-N
Smith, R. A., Hartley, R. C., Cocheme, H. M., and Murphy, M. P. (2012). Mitochondrial pharmacology. Trends Pharmacol. Sci. 33, 341–352. doi: 10.1016/j.tips.2012.03.010
Sun, X., Cao, H., Zhan, L., Yin, C., Wang, G., Liang, P., et al. (2018). Mitochondrial fission promotes cell migration by Ca(2+) /CaMKII/ERK/FAK pathway in hepatocellular carcinoma. Liver Int. 38, 1263–1272. doi: 10.1111/liv.13660
Susztak, K., Raff, A. C., Schiffer, M., and Bottinger, E. P. (2006). Glucose-induced reactive oxygen species cause apoptosis of podocytes and podocyte depletion at the onset of diabetic nephropathy. Diabetes 55, 225–233. doi: 10.2337/diabetes.55.01.06.db05-0894
Takagi, K., Yamada, Y., Gong, J. S., Sone, T., Yokota, M., and Tanaka, M. (2004). Association of a 5178C–>A (Leu237Met) polymorphism in the mitochondrial DNA with a low prevalence of myocardial infarction in Japanese individuals. Atherosclerosis 175, 281–286. doi: 10.1016/j.atherosclerosis.2004.03.008
Takasaki, S. (2008). Mitochondrial SNPs associated with Japanese centenarians, Alzheimer's patients, and Parkinson's patients. Comput. Biol. Chem. 32, 332–337. doi: 10.1016/j.compbiolchem.2008.03.014
Takasaki, S. (2009). Mitochondrial haplogroups associated with Japanese Alzheimer's patients. J. Bioenerg. Biomembr. 41, 407–410. doi: 10.1007/s10863-009-9240-8
Tanaka, M., Fuku, N., Nishigaki, Y., Matsuo, H., Segawa, T., Watanabe, S., et al. (2007). Women with mitochondrial haplogroup N9a are protected against metabolic syndrome. Diabetes 56, 518–521. doi: 10.2337/db06-1105
Tang, S., Batra, A., Zhang, Y., Ebenroth, E. S., and Huang, T. (2010). Left ventricular noncompaction is associated with mutations in the mitochondrial genome. Mitochondrion 10, 350–357. doi: 10.1016/j.mito.2010.02.003
Tian, S., Chen, H., and Tan, W. (2018). Targeting mitochondrial respiration as a therapeutic strategy for cervical cancer. Biochem. Biophys. Res. Commun. 499, 1019–1024. doi: 10.1016/j.bbrc.2018.04.042
Ueshima, H., Sekikawa, A., Miura, K., Turin, T. C., Takashima, N., Kita, Y., et al. (2008). Cardiovascular disease and risk factors in Asia: a selected review. Circulation 118, 2702–2709. doi: 10.1161/CIRCULATIONAHA.108.790048
van Oven, M., and Kayser, M. (2009). Updated comprehensive phylogenetic tree of global human mitochondrial DNA variation. Hum. Mutat. 30, E386–394. doi: 10.1002/humu.20921
van Rahden, V. A., Fernandez-Vizarra, E., Alawi, M., Brand, K., Fellmann, F., Horn, D., et al. (2015). Mutations in NDUFB11, encoding a complex I component of the mitochondrial respiratory chain, cause microphthalmia with linear skin defects syndrome. Am. J. Hum. Genet. 96, 640–650. doi: 10.1016/j.ajhg.2015.02.002
Vats, D., Mukundan, L., Odegaard, J. I., Zhang, L., Smith, K. L., Morel, C. R., et al. (2006). Oxidative metabolism and PGC-1beta attenuate macrophage-mediated inflammation. Cell Metab. 4, 13–24. doi: 10.1016/j.cmet.2006.05.011
Verma, P., Singh, A., Nthenge-Ngumbau, D. N., Rajamma, U., Sinha, S., Mukhopadhyay, K., et al. (2016). Attention deficit-hyperactivity disorder suffers from mitochondrial dysfunction. BBA Clin. 6, 153–158. doi: 10.1016/j.bbacli.2016.10.003
Victor, V. M., Rocha, M., Herance, R., and Hernandez-Mijares, A. (2011). Oxidative stress and mitochondrial dysfunction in type 2 diabetes. Curr. Pharm. Des. 17, 3947–3958. doi: 10.2174/138161211798764915
Wallace, D. C. (2000). Mitochondrial defects in cardiomyopathy and neuromuscular disease. Am. Heart J. 139(2 Pt 3), S70–S85. doi: 10.1067/mhj.2000.103934
Wallace, D. C. (2005). Mitochondria and cancer: warburg addressed. Cold Spring Harb. Symp. Quant. Biol. 70, 363–374. doi: 10.1101/sqb.2005.70.035
Wallace, D. C. (2013). A mitochondrial bioenergetic etiology of disease. J. Clin. Invest. 123, 1405–1412. doi: 10.1172/JCI61398
Wallace, D. C., Singh, G., Lott, M. T., Hodge, J. A., Schurr, T. G., Lezza, A. M., et al. (1988a). Mitochondrial DNA mutation associated with Leber's hereditary optic neuropathy. Science 242, 1427–1430. doi: 10.1126/science.3201231
Wallace, D. C., Zheng, X. X., Lott, M. T., Shoffner, J. M., Hodge, J. A., Kelley, R. I., et al. (1988b). Familial mitochondrial encephalomyopathy (MERRF): genetic, pathophysiological, and biochemical characterization of a mitochondrial DNA disease. Cell 55, 601–610. doi: 10.1016/0092-8674(88)90218-8
Wang, C. Y., Kong, Q. P., Yao, Y. G., and Zhang, Y. P. (2006). mtDNA mutation C1494T, haplogroup A, and hearing loss in Chinese. Biochem. Biophys. Res. Commun. 348, 712–715. doi: 10.1016/j.bbrc.2006.07.119
Wang, D., Taniyama, M., Suzuki, Y., Katagiri, T., and Ban, Y. (2001). Association of the mitochondrial DNA 5178A/C polymorphism with maternal inheritance and onset of type 2 diabetes in Japanese patients. Exp. Clin. Endocrinol. Diabetes 109, 361–364. doi: 10.1055/s-2001-17407
Wang, S., Wu, S., Zheng, T., Yang, Z., Ma, X., Jia, W., et al. (2013). Mitochondrial DNA mutations in diabetes mellitus patients in Chinese Han population. Gene 531, 472–475. doi: 10.1016/j.gene.2013.09.019
Wang, X., Guo, Y., and Luan, Q. (2015). Association of mitochondrial DNA displacement loop polymorphisms and aggressive periodontitis in a Chinese population: a pilot study. Mitochondrial DNA 26, 389–395. doi: 10.3109/19401736.2013.840589
Wang, X., Su, B., Perry, G., Smith, M. A., and Zhu, X. (2007). Insights into amyloid-beta-induced mitochondrial dysfunction in Alzheimer disease. Free Radic. Biol. Med. 43, 1569–1573. doi: 10.1016/j.freeradbiomed.2007.09.007
Wang, Z., Hao, G., Wang, X., Chen, Z., Zhang, L., Zhang, Z., et al. (2019). Clinical outcomes and economic impact of the 2017 ACC/AHA guidelines on hypertension in China. J. Clin. Hypertens. 21, 1212–1220. doi: 10.1111/jch.13609
Wang, Z., Ying, Z., Bosy-Westphal, A., Zhang, J., Schautz, B., Later, W., et al. (2010). Specific metabolic rates of major organs and tissues across adulthood: evaluation by mechanistic model of resting energy expenditure. Am. J. Clin. Nutr. 92, 1369–1377. doi: 10.3945/ajcn.2010.29885
Warburg, O. (1956). On the origin of cancer cells. Science 123, 309–314. doi: 10.1126/science.123.3191.309
Wei, P. Z., and Szeto, C. C. (2019). Mitochondrial dysfunction in diabetic kidney disease. Clin. Chim. Acta 496, 108–116. doi: 10.1016/j.cca.2019.07.005
Wei, Y. L., Yu, C. A., Yang, P., Li, A. L., Wen, J. Y., Zhao, S. M., et al. (2009). Novel mitochondrial DNA mutations associated with Chinese familial hypertrophic cardiomyopathy. Clin. Exp. Pharmacol. Physiol. 36, 933–939. doi: 10.1111/j.1440-1681.2009.05183.x
Wilkins, H. M., Carl, S. M., and Swerdlow, R. H. (2014). Cytoplasmic hybrid (cybrid) cell lines as a practical model for mitochondriopathies. Redox Biol. 2, 619–631. doi: 10.1016/j.redox.2014.03.006
Wu, D., Zhang, J., Wang, J., Li, J., Liao, F., and Dong, W. (2016). Hesperetin induces apoptosis of esophageal cancer cells via mitochondrial pathway mediated by the increased intracellular reactive oxygen species. Tumour Biol. 37, 3451–3459. doi: 10.1007/s13277-015-4176-6
Xing, Y., Ichida, F., Matsuoka, T., Isobe, T., Ikemoto, Y., Higaki, T., et al. (2006). Genetic analysis in patients with left ventricular noncompaction and evidence for genetic heterogeneity. Mol. Genet. Metab. 88, 71–77. doi: 10.1016/j.ymgme.2005.11.009
Xu, L., Hu, Y., Chen, B., Tang, W., Han, X., Yu, H., et al. (2006). Mitochondrial polymorphisms as risk factors for endometrial cancer in southwest China. Int. J. Gynecol. Cancer 16, 1661–1667. doi: 10.1111/j.1525-1438.2006.00641.x
Xu, T., Pang, Q., Wang, Y., and Yan, X. (2017). Betulinic acid induces apoptosis by regulating PI3K/Akt signaling and mitochondrial pathways in human cervical cancer cells. Int. J. Mol. Med. 40, 1669–1678. doi: 10.3892/ijmm.2017.3163
Xu, W., Mi, Y., He, P., He, S., and Niu, L. (2017). γ-tocotrienol inhibits proliferation and induces apoptosis via the mitochondrial pathway in human cervical cancer HeLa cells. Molecules 22:E1299. doi: 10.3390/molecules22081299
Xue, F., Wang, Y., Xu, S., Zhang, F., Wen, B., Wu, X., et al. (2008). A spatial analysis of genetic structure of human populations in China reveals distinct difference between maternal and paternal lineages. Eur. J. Hum. Genet. 16, 705–717. doi: 10.1038/sj.ejhg.5201998
Xue, H., Li, P., Luo, Y., Wu, C., Liu, Y., Qin, X., et al. (2019). Salidroside stimulates the Sirt1/PGC-1α axis and ameliorates diabetic nephropathy in mice. Phytomedicine 54, 240–247. doi: 10.1016/j.phymed.2018.10.031
Yang, D., Wang, Q., Shi, Y., Fan, Y., Zheng, H. X., Song, G., et al. (2014). Mitochondrial DNA haplogroup D4b is a protective factor for ischemic stroke in Chinese Han population. Mol. Genet. Genomics 289, 1241–1246. doi: 10.1007/s00438-014-0884-7
Ying, Z., Zheng, J., Cai, Z., Liu, L., Dai, Y., Yao, J., et al. (2015). Mitochondrial haplogroup B increases the risk for hearing loss among the Eastern Asian pedigrees carrying 12S rRNA 1555A>G mutation. Protein Cell 6, 844–848. doi: 10.1007/s13238-015-0203-z
Yoneda, M., Tsuji, S., Yamauchi, T., Inuzuka, T., Miyatake, T., Horai, S., et al. (1989). Mitochondrial DNA mutation in family with Leber's hereditary optic neuropathy. Lancet 1, 1076–1077. doi: 10.1016/S0140-6736(89)92470-7
Youle, R. J., and Narendra, D. P. (2011). Mechanisms of mitophagy. Nat. Rev. Mol. Cell Biol. 12, 9–14. doi: 10.1038/nrm3028
Young, W. Y., Zhao, L., Qian, Y., Wang, Q., Li, N., Greinwald, J. H. Jr., et al. (2005). Extremely low penetrance of hearing loss in four Chinese families with the mitochondrial 12S rRNA A1555G mutation. Biochem. Biophys. Res. Commun. 328, 1244–1251. doi: 10.1016/j.bbrc.2005.01.085
Yu, D., Jia, X., Zhang, A. M., Li, S., Zou, Y., Zhang, Q., et al. (2010). Mitochondrial DNA sequence variation and haplogroup distribution in Chinese patients with LHON and m.14484T>C. PLoS ONE 5:e13426. doi: 10.1371/journal.pone.0013426
Yuan, H., Chen, J., Liu, X., Cheng, J., Wang, X., Yang, L., et al. (2007). Coexistence of mitochondrial 12S rRNA C1494T and CO1/tRNA(Ser(UCN)) G7444A mutations in two Han Chinese pedigrees with aminoglycoside-induced and non-syndromic hearing loss. Biochem. Biophys. Res. Commun. 362, 94–100. doi: 10.1016/j.bbrc.2007.07.161
Zhai, K., Chang, L., Zhang, Q., Liu, B., and Wu, Y. (2011). Mitochondrial C150T polymorphism increases the risk of cervical cancer and HPV infection. Mitochondrion 11, 559–563. doi: 10.1016/j.mito.2011.02.005
Zhan, L., Cao, H., Wang, G., Lyu, Y., Sun, X., An, J., et al. (2016). Drp1-mediated mitochondrial fission promotes cell proliferation through crosstalk of p53 and NF-kappaB pathways in hepatocellular carcinoma. Oncotarget 7, 65001–65011. doi: 10.18632/oncotarget.11339
Zhang, J., Zhang, Z. X., Du, P. C., Zhou, W., Wu, S. D., Wang, Q. L., et al. (2015). Analyses of the mitochondrial mutations in the Chinese patients with sporadic Creutzfeldt-Jakob disease. Eur. J. Hum. Genet. 23, 86–91. doi: 10.1038/ejhg.2014.52
Zhang, R., Zhang, F., Wang, C., Wang, S., Shiao, Y. H., and Guo, Z. (2010). Identification of sequence polymorphism in the D-Loop region of mitochondrial DNA as a risk factor for hepatocellular carcinoma with distinct etiology. J. Exp. Clin. Cancer Res. 29:130. doi: 10.1186/1756-9966-29-130
Zhang, X. H., Guan, T. R., Mao, J. W., and Liu, L. S. (2007). Disparity and its time trends in stroke mortality between urban and rural populations in China 1987 to 2001 - Changing patterns and their implications for public health policy. Stroke 38, 3139–3144. doi: 10.1161/STROKEAHA.107.494336
Zhang, Y., Zhao, Y., Wen, S., Yan, R., Yang, Q., and Chen, H. (2017). Associations of mitochondrial haplogroups and mitochondrial DNA copy numbers with end-stage renal disease in a Han population. Mitochondrial DNA A DNA Mapp. Seq. Anal. 28, 725–731. doi: 10.1080/24701394.2016.1177038
Zheng, S., Qian, P., Li, F., Qian, G., Wang, C., Wu, G., et al. (2012). Association of mitochondrial DNA variations with lung cancer risk in a Han Chinese population from southwestern China. PLoS ONE 7:e31322. doi: 10.1371/journal.pone.0031322
Zhong, L., Tang, J., Kong, Q. P., Sun, C., Zhou, W. P., Yang, M., et al. (2014). Reappraising the relationship between mitochondrial DNA variant m.16189T>C and type 2 diabetes mellitus in East Asian populations. Curr. Mol. Med. 14, 1273–1278. doi: 10.2174/1566524014666141202161326
Zhong, Z., Liang, S., Sanchez-Lopez, E., He, F., Shalapour, S., Lin, X. J., et al. (2018). New mitochondrial DNA synthesis enables NLRP3 inflammasome activation. Nature 560, 198–203. doi: 10.1038/s41586-018-0372-z
Zhou, X., Zhang, H., Zhao, F., Ji, Y., Tong, Y., Zhang, J., et al. (2010). Very high penetrance and occurrence of Leber's hereditary optic neuropathy in a large Han Chinese pedigree carrying the ND4 G11778A mutation. Mol. Genet. Metab. 100, 379–384. doi: 10.1016/j.ymgme.2010.04.013
Zhu, J. F., Zhang, X., and Ling, L. (2016). Molecular characterization of a Han Chinese family with essential hypertension. Genet. Mol. Res. 15:gmr.15028084. doi: 10.4238/gmr.15028084
Keywords: mitochondrial DNA, variation, haplogroup, chronic disease, East Asian
Citation: Sun D, Wei Y, Zheng H-X, Jin L and Wang J (2019) Contribution of Mitochondrial DNA Variation to Chronic Disease in East Asian Populations. Front. Mol. Biosci. 6:128. doi: 10.3389/fmolb.2019.00128
Received: 18 July 2019; Accepted: 29 October 2019;
Published: 15 November 2019.
Edited by:
Eleonora Napoli, University of California, Davis, United StatesReviewed by:
Aurora Gomez-Duran, University of Cambridge, United KingdomPrasun K. Datta, Temple University, United States
Copyright © 2019 Sun, Wei, Zheng, Jin and Wang. This is an open-access article distributed under the terms of the Creative Commons Attribution License (CC BY). The use, distribution or reproduction in other forums is permitted, provided the original author(s) and the copyright owner(s) are credited and that the original publication in this journal is cited, in accordance with accepted academic practice. No use, distribution or reproduction is permitted which does not comply with these terms.
*Correspondence: Li Jin, bGlqaW4mI3gwMDA0MDtmdWRhbi5lZHUuY24=; Jiucun Wang, amN3YW5nJiN4MDAwNDA7ZnVkYW4uZWR1LmNu