- 1Laboratory of Biochemistry and Protein Chemistry, Department of Cell Biology, Institute of Biology, University of Brasilia, Brasília, Brazil
- 2Department of Biochemistry and Molecular Biology, University of Southern Denmark, Odense, Denmark
- 3Laboratory of Surgical Physiopathology (LIM-62), Faculty of Medicine, University of São Paulo, São Paulo, Brazil
Intestinal ischemia and reperfusion injury is a model system of possible consequences of severe trauma and surgery, which might result into tissue dysfunction and organ failure. Neutrophils contribute to the injuries preceded by ischemia and reperfusion. However, the mechanisms by which intestinal ischemia and reperfusion stimulate and activate circulating neutrophils is still not clear. In this work, we used proteomics approach to explore the underlying regulated mechanisms in Wistar rat neutrophils after ischemia and reperfusion. We isolated neutrophils from three different biological groups; control, sham laparotomy, and intestinal ischemia/reperfusion. In the workflow, we included iTRAQ-labeling quantification and peptide fractionation using HILIC prior to LC-MS/MS analysis. From proteomic analysis, we identified 2,045 proteins in total that were grouped into five different clusters based on their regulation trend between the experimental groups. A total of 417 proteins were found as significantly regulated in at least one of the analyzed conditions. Interestingly, the enzyme prediction analysis revealed that ischemia/reperfusion significantly reduced the relative abundance of most of the antioxidant and pro-survival molecules to cause more tissue damage and ROS production whereas some of the significantly up regulated enzymes were involved in cytoskeletal rearrangement, adhesion and migration. Clusters based KEGG pathways analysis revealed high motility, phagocytosis, directional migration, and activation of the cytoskeletal machinery in neutrophils after ischemia and reperfusion. Increased ROS production and decreased phagocytosis were experimentally validated by microscopy assays. Taken together, our findings provide a characterization of the rat neutrophil response to intestinal ischemia and reperfusion and the possible mechanisms involved in the tissue injury by neutrophils after intestinal ischemia and reperfusion.
Introduction
The intestine is the most sensitive organ to ischemia and reperfusion (IR) injury. This injury can result from various clinical situations, such as intestinal obstruction, acute mesenteric ischemia, incarcerated hernia, small intestine transplantation, neonatal necrotizing enterocolitis, trauma, and shock, taking the patient to relentless clinical syndromes, and even death (Mojzis et al., 2001; Mallick et al., 2004; Guneli et al., 2007). It is clear now that the reperfusion following ischemia leads to significantly greater mucosal intestinal injury as compared to the ischemia alone (Crissinger and Granger, 1989) whereas development of the systemic inflammatory response syndrome (SIRS) and multiple organ failure (MOF) can be the final consequences of IR (Ceppa et al., 2003).
Among the polymorphonuclear leukocytes (PMNs), neutrophils are the first line of defense against bacterial and fungal infections (Kaufmann, 2008). However, a large number of studies showed that IR injury is mainly because of PMN and endothelial cell (EC) interactions in reperfused tissues (Massberg et al., 1998; Kumar et al., 2009; Kvietys and Granger, 2012). Normally, a multistep process of neutrophil recruitment to the site of infection requires three types of adhesion receptors, like integrins, selectins, and adhesion receptors of the immunoglobulin superfamily (Rao et al., 2007).
It has been shown that P- and E-selectins are over expressed in a mouse model after intestinal ischemia/reperfusion and that inhibiting P-selectin decreased neutrophil rolling and adhesion, reducing the injury (Riaz et al., 2002). Another IR model showed decreased neutrophil rolling and adherence by blocking of P- and L-selectins (Kubes et al., 1995). The up-regulation of adhesion molecules on the endothelial surface was observed following ischemia/reperfusion injury (IRI) that can result in diapedesis of neutrophils, further contributing to muscle dysfunction (Hierholzer et al., 1999). Similarly, in small intestine, an increase in expression of inflammatory mediators like tumor necrosis factor-α (TNF-α), cyclooxygenase-2 (COX-2) and intercellular adhesion molecule-1 (ICAM-1), have been observed after IR with increase in neutrophil infiltration (Watanabe et al., 2014). Another study demonstrated systemic serum level elevation of the CC chemokines along with XC chemokines in a model of intestinal ischemia, hence leading to greater PMNs activation and tissue injury (Jawa et al., 2013). Each of these signals interact with specific receptors expressed on the plasma membrane of PMNs with an overlapping array of signal transduction pathways leading to functional responses such as rearrangement of the actin cytoskeleton (Luerman et al., 2010).
A gradient of chemoattractant signals arising from the dying tissues helps in the recruitment of PMNs (McDonald et al., 2010) that secrete a large number of factors like reactive oxygen species, chemokines, cytokines, lipid mediators, and proteases (Rodrigues and Granger, 2010). However, molecular mechanisms, enzymes, and pathways by which PMNs participate in the IR injury are not fully understood. The current understanding of the interconnections among the many signal transduction pathways that regulate neutrophil activation is incomplete. Proteomics research has improved the understanding of the neutrophil biology in the past, and few publications describe the effect of the inflammatory response on the PMNs proteome (Morris et al., 2008). There is one proteomic study on the response to intestinal ischemia and reperfusion, however limited on molecules expressed by the intestinal epithelium, and there is lack of understanding regarding the PMNs response against IR. The main objective of this study is to explore the effect of IR on the PMNs in rats using mass spectrometry based proteomics (Hurst et al., 1998). We analyzed the neutrophil proteome from three different biological conditions, including control, sham laparotomy and intestinal ischemia/reperfusion. Proteomic analysis of neutrophils after ischemia and reperfusion revealed that neutrophils down regulate the expression of different antioxidant, pro-survival molecules. Significantly up regulated oxidoreductases and down regulated transferases can interfere in the integrin signaling pathway, lipid metabolism and reactive oxygen species (ROS) generation, leading to the local and remote tissues injury. Furthermore, our analysis shows the regulation of different proteins and pathways required for neutrophil adhesion, directional migration, and phagocytosis after intestinal ischemia and reperfusion. Down regulation of important enzymes from LTB4 synthesis pathway opens some questions that need further analysis. Functional assays revealing increased ROS production and decreased phagocytosis after ischemia/reperfusion are coherent with the proteomics findings. We anticipate that these findings will provide trustworthy basis for further deep analysis in neutrophil biology.
Results
Protein Identification and Relative Abundance Profile
For the large-scale proteomics analysis of rat neutrophils, samples were collected from three experimental groups (Figure 1) including control, laparotomy and intestinal ischemia/reperfusion groups. Proteins from each experimental group with five biological replicates were iTRAQ labeled for relative quantification analysis. To find the significant changes in protein abundance among three groups, unique peptides with high confidence identification and their respective iTRAQ reporter ion intensity values were analyzed by using R. For a detailed description of the methods, see the Supplementary Methods section. Mass spectrometry analysis resulted in the identification of 2045 proteins (Supplementary Table S1).
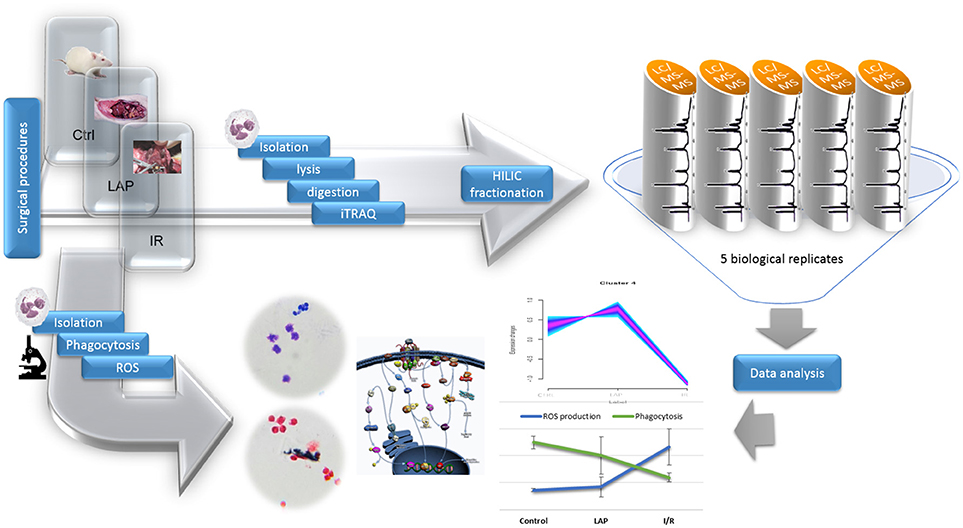
Figure 1. Experimental design. Surgical procedures were followed by isolation of neutrophils from the three conditions. Protein extraction was performed by using FASP protocol. After Hilic fractionations, LTQ Orbitrap MS analysis was performed and the data obtained was analyzed by using bioinformatics tools. Another set of isolated neutrophils was used to determine the phagocytosis rate and ROS production by optical microscopy.
After the data analysis, proteins were grouped into clusters according to their abundance profile among the three conditions. To assign the proteins according to their abundance in the best number of clusters, two validation indices, Xie-Beni index (Xie and Beni, 1991) and minimal centroid distance (Schwammle and Jensen, 2010), were applied and proteins were assigned to five different clusters based on their regulation trend (Figure 2, Supplementary Table S2). Expression changes are shown as z scores (i.e., relative abundances normalized by mean and standardized by dividing by the standard deviation). The colors correspond to the so-called membership values (values in the range [0.1]), representing the degree of how much a protein belongs to the nearest cluster. Only proteins with memberships above 0.5 are shown. A subset of 417 proteins was significantly regulated (Limma and rank products q-value < 0.05) in at least one of the analyzed conditions within the five clusters. A standard PCA analysis was performed to check the similarity among different samples.
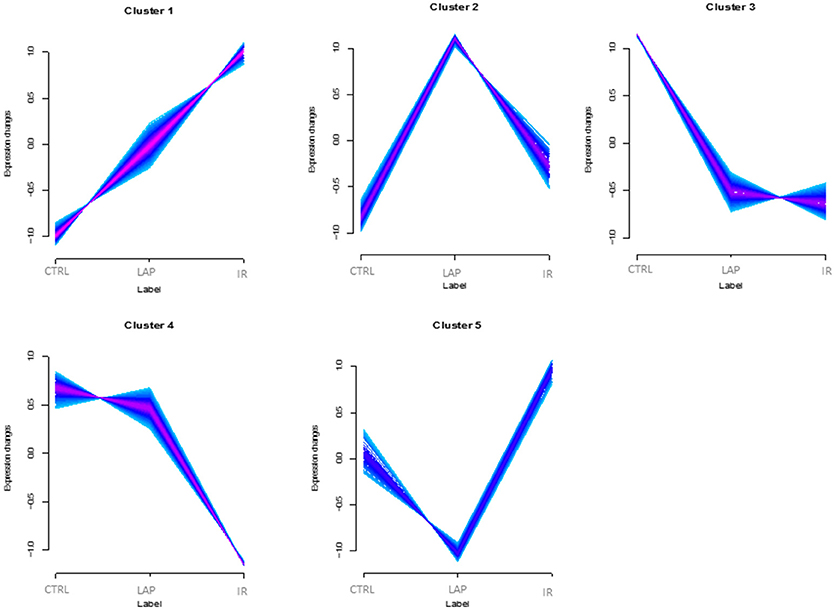
Figure 2. Abundance profiles of rat neutrophils proteins. Abundance profile of the regulated proteins in control (Ctrl), laparotomy (LAP), and ischemia/reperfusion (IR) experimental groups. The x-axis shows the different conditions and y-axis represents expression change (z-scores). The colors correspond to the membership values, representing the degree of how much a protein belongs to the nearest cluster.
Go slim Analysis and Enzyme Activity Prediction for Total Rat Neutrophil Proteome
Protein classification was performed by GO slim analysis using ProteinCenter (Thermo Scientific) as a platform. The resultant cellular component, biological process and molecular function terms for the five clusters are shown in Supplementary Figure S1. Molecular function analysis revealed that about 33% of the neutrophil proteome has predicted enzymatic activity. It is composed of 18% oxidoreductases (EC:1), 27% transferases (EC:2), 38% hydrolases (EC:3), 3% lyases (EC:4), 5% isomerases (EC:5) and 9% ligases (EC:6) as predicted activity, as shown in Figure 3A. The overall distribution of the enzymes across five clusters is illustrated in Figure 3B whereas Table 1 represents the significantly regulated proteins in at least two conditions among 5 clusters.
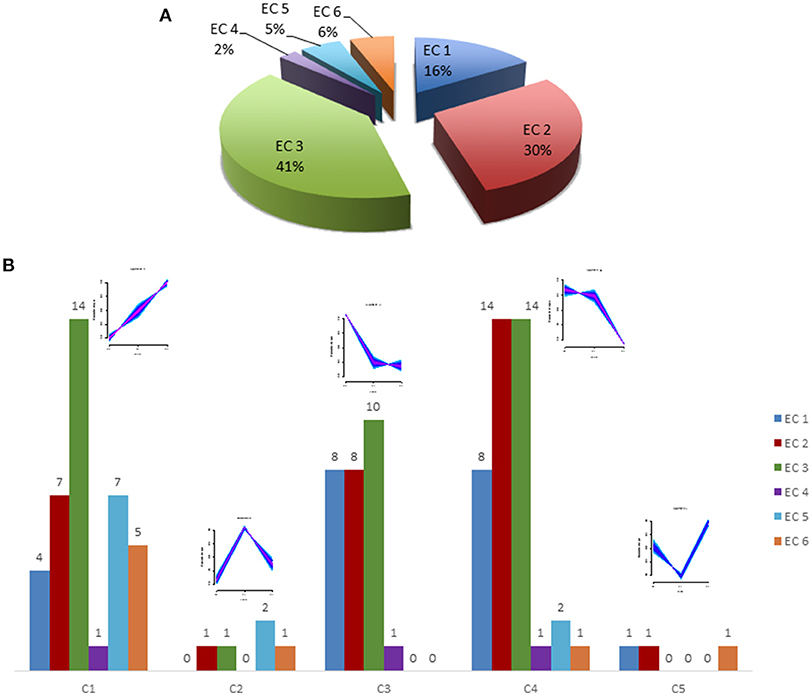
Figure 3. Enzyme activity prediction. (A) A pie chart shows the predicted enzyme activity of six classes of enzymes for total rat neutrophil proteome. (B) A bar chart shows predicted enzyme activity for regulated proteins from all 5 clusters. EC 1 represents Oxidoreductases, EC 2–Transferases, EC 3–Hydrolases, EC 4–Lyases, EC 5–Isomerases, and EC 6–Ligases. A detail of the predicted enzymatic activities of these proteins is listed in Table 1. The small images above each cluster show the general cluster pattern. For details on this see Figure 2.
Major Functional Classes of the Neutrophil Proteome Regulated by IR
For the functional classification of the significantly regulated identified proteins, KEGG and Wiki pathways analyses were done and the enriched pathways are listed in Table 2. Most of the enriched pathways found were immune-related indicating the effect of intestinal ischemia and reperfusion on the neutrophil function. Phagocytosis was found significantly down regulated in IR as shown in Table 2, Figure 4.
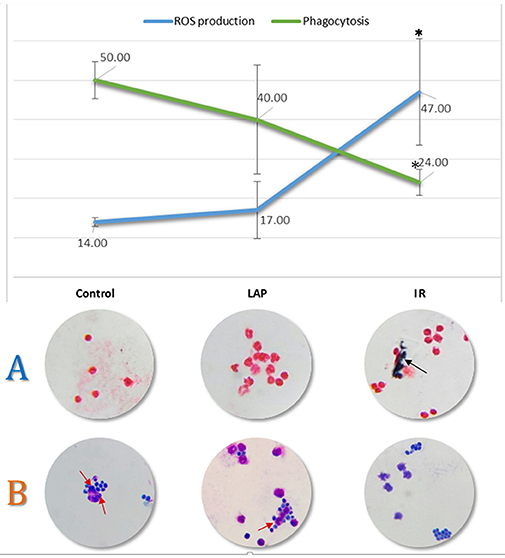
Figure 4. Evaluation of ROS production and phagocytosis. The line graph shows the results of neutrophil function assays obtained by optical microscopy analysis—Phagocytosis in green and ROS production in blue. The asterisk represents significant differences (p < 0.05) when compared to control or to LAP groups. Cell counts were normalized to one hundred cells. Typical cells are shown below their respective condition in the graph for ROS evaluation (A) where the black arrow points to formazan crystals and phagocytosis (B) of Saccharomyces cerevisiae pointed by red arrows.
Verification of ROS Production and Phagocytosis
To validate such findings we performed a ROS production and phagocytosis assays by incubating neutrophils from the three groups with Saccharomyces cerevisiae yeast cells. Phagocytic activity was significantly decreased in IR group (p-value < 0.05) compared to control and LAP (Figure 4). Only about 23.90% of cells phagocytosed in IR group while control and LAP presented 50 and 40.7% phagocytosis rates respectively.
Discussion
Speculating about correlations between cluster profiles and physiological conditions, cluster 1 suggests possible markers that would correlate to the injury severity, since protein abundances progressively increase with the severity of the surgical event. Clusters 2 and 5 suggest proteins that change the sense of regulation when the condition changes from mild to more intense surgical procedure and, in similar ways, cluster 3 detected proteins that respond similarly to any intensity of trauma, and proteins regulated only by intestinal ischemia and reperfusion were clustered in 4.
The five clusters where our quantified proteins were grouped did not enrich for specific cellular localization. According to Supplementary Figure S1A, most of the significantly regulated proteins from clusters 1, 3, and 4 were annotated to cytoplasm, extracellular, and nucleus. Cytosol and organelle lumen had proteins from cluster 1 whereas ribosome proteins were exclusively annotated to cluster 1. Mostly membrane proteins were grouped in cluster 4 that is down regulated in IR as compared to the control and laparotomy groups.
Proteins related to metabolic process from cluster 1 and 4 showed enrichment (Supplementary Figure S1B) whereas, the highest number of proteins related to metabolic processes belong to cluster 1 which is continuously up regulated in laparotomy and IR as compared to the control group. Gene Ontology (GO) terms distribution for the molecular functions (Supplementary Figure S1C) of all the regulated proteins from clusters 1, 3, and 4 showed annotation to catalytic activity, binding proteins whereas cluster 1 represented a high amount of protein enrichment for RNA binding and structural molecule activity. Proteins from cluster 1 and 4 showed highest diversity in molecular functions among the clusters.
After identifying a large number of proteins, the data were analyzed in Uniprot to predict the enzyme activity for the identified neutrophil proteome. It has been clear from the literature that different enzymes play an important role in neutrophils. The enzyme activities encountered varies for each cluster (Figure 3B).
Predicted Enzyme Activity for Cluster 1 Proteins
These enzymes are found progressively up regulated in neutrophils from the control to the laparotomy to the ischemia/reperfusion group suggesting that the intensity of regulation may be proportional to the severity of the inflammatory process.
Oxidoreductases
The quantitative analysis revealed significantly up regulated oxidoreductases in cluster 1, among which we found superoxide dismutase (SOD). Normally during inflammation, SOD regulates ROS concentration and reactive nitrogen species however high levels of extracellular SOD activity resulted in reduced innate immune response of neutrophils (Break et al., 2012). Increased level of DHFR was observed in our quantitative analysis whereas in peripheral blood leucocytes, mainly neutrophils, of cancer patients' higher expression of DHFR has been associated with leukocytosis (Iqbal et al., 2000). Other significantly up regulated oxidoreductases of cluster 1 include Prostagladin reductase 1 (PtGR-1), and fatty acyl-CoA reductase 1 (Table 1). PtGR-1 is a nitroalkene reductase and its overexpression in HEK293T cells promoted inactivation of nitroalkane, inhibition of hemeoxygenase HO-1 and finally abrogated tissue protection (Vitturi et al., 2013). It has been shown that inducing HO-1 prior to IRI results in a significant decrease of intestinal tissue injury (Wasserberg et al., 2007).
Transferases
N-myristoyltransferase (NMT1) catalyzes protein myristoylation process that is essential for leukocyte growth and development, found elevated in activated neutrophils along with increase in lifespan (Kumar et al., 2011). The majority of significantly regulated transferases are oligosaccharyltransferases involved in N-Linked protein glycosylation. Since pro-inflammatory stimuli modify glycan profiles on cell surfaces, the overexpression of such enzymes can lead to an increased adhesion of activated neutrophils to endothelial cells (Sriramarao et al., 1993). S-adenosylmethionine (SAM) acts as a donor for methyltransferases that is involved in methylation of DNA, RNA, proteins, and lipids. SAM has important implications in antigen-induced immune responses (Wu et al., 2005).
Hydrolases
Most of the regulated hydrolases are annotated to DNA helicases and one RNA helicase. Other statistically regulated hydrolases, relevant to the inflammation were Caspase-1, involved in the inflamasome pathway, and Cathepsin D. Increased activity of cathepsin D in myocardial ischemic neutrophils was found to be associated with increased ROS production (Miriyala et al., 2013). Both the Caspase-1 and Cathepsin D were significantly found up regulated in this study showing high inflammasome pathway activation and ROS production.
Predicted Enzyme Activity for Cluster 4 Proteins
Another group of transferases, oxidoreductases, and hydrolases present predominant regulation in neutrophils from intestinal ischemia/reperfusion in cluster 4 as shown in Figure 3B.
Oxidoreductases
There are some important oxidoreductases regulated in this cluster. Arachidonate 15-lipoxygenase was found significantly down regulated. It has been known that arachidonate 12/15-lipoxygenases (12/15-LOX) forms hydroperoxy eicosatetraenoic acids (HPETEs) from arachidonic acid that subsequently produces eicosanoids. Leukocytes highly expresses 15-LOX but little is known about its role in neutrophils (Nadel et al., 1991). Xanthine oxidase (Xdh) and thioredoxin reductase 1 (TXNRD1) are cellular defense enzymes against oxidative stress. Significantly down regulation of these antioxidants after IR can be an important step in modulating the neutrophil response to oxidative stress (Vorbach et al., 2003; Biterova et al., 2005). The most extensively studied primary granule enzyme of neutrophils is myeloperoxidase (MPO), found significantly regulated in cluster 4 by our quantitative analysis (Table 1). Recently an increase in MPO along with ICAM-1 and P-selectin expressions in neutrophils has been found in a model of small intestinal ischemia in rats (Gan et al., 2015).
Transferases
Most down regulated transferases have function in phospholipid metabolism, carbohydrate degradation (glycolysis) and nucleotide metabolism. The tyrosine-protein kinases Lyn/Fgr belong to the Src family of kinases that negatively regulate integrin-signaling pathway. Neutrophils deficient of these kinases had enhanced respiratory burst, secondary granule release, and a hyper-adhesive phenotype due to reduced mobilization of SHP-1 (Pereira and Lowell, 2003). Many metabolic enzymes including hexokinase, phosphofructokinase and pyruvate kinase were found significantly down regulated in cluster 4 in this study, changes that have also been previously observed during neutrophil activation (Huang et al., 2002). Pyruvate kinase down regulation can lead to partial inhibition of glycolysis at the last step and enhance the synthesis of lipids and nucleic acids, however neutrophils deficient in PK also showed immunodeficiency (Burge et al., 1976). At the first step of glycolysis, Hexokinase (HK3) has 70–80% activity in granulocytes while its down regulation impairs neutrophil differentiation (Federzoni et al., 2012). Although significantly regulated, the transposition of these results to functional inferences needs validation due to the presence of multiple proteoforms of such enzymes.
Hydrolases
Some of the important hydrolases were found significantly down regulated like P97573, Phosphatidylinositol 3,4,5-trisphosphate 5-phosphatase (SHIP). Neutrophil with loss of SHIP showed defective cell migration, loss of polarity upon cell adhesion and increased adhesion due to Akt activation and higher PtdIns(3,4,5)P3 (Mondal et al., 2012). Leukotrienes (LTs) are implicated in a wide variety of inflammatory disorders and are produced in arachidonic acid (Kutmon et al., 2015) cascade in immune cells. Two bifunctional hydrolases of AA cascade were found regulated in cluster 4. One is leukotriene A-4 hydrolase (LTA4H) involved in leukotriene B4 biosynthesis from LTB4 (Liu and Yokomizo, 2015) and in aminopeptidase activity by breakdown and clearance of Proline-Glycine-Proline (PGP), a neutrophil chemoattractant (Snelgrove et al., 2010). The other bifunctional hydrolase found down regulated, Gamma-glutamyltransferase 5 (GGT5), participates in glutathione metabolism and in leukotriene D4 biosynthesis from LTC4 and down regulation of these enzymes can lead to accumulation of leukotriene products (Liu and Yokomizo, 2015) along with PGP and affect neutrophil biology with influx of neutrophils into the tissue and air spaces (Paige et al., 2014). Another interesting hydrolase is Dynamin-2\Dynamin GTPase (DNM), which is involved in microtubules production, binding and hydrolyzation of GTP. Recently, a study showed that inhibition of dynamin impaired the membrane fusion/fission events and resulted in production of highly adhesive cellular secretory protrusions called cytonemes that help neutrophils in long-range contacts with other cells or bacteria after adhesion. Down regulation of dynamin can be an important step in cytoneme production leading to increase in adhesion (Galkina et al., 2005).
An overall evaluation of the enzymes regulated in cluster 1 suggests that neutrophils would progressively increase the ROS production and self-protection against ROS (like SOD, DHFR, Cathepsin-D), decrease tissue protection e.g. PtGR1, increase neutrophil lifespan such as NMT1 and promote adhesion (oligosaccharyl transferases). Such progression appears to be proportional to the injury severity. The regulated enzymes in cluster 4 also suggest an intense effect of IR on the oxidative stress mechanism (including XO, TXNRD1, MPO, Lyn, Fgr, and GGt5) and leukotriene metabolism (as LTA4H and GGT5).
To validate the results regarding ROS production, we performed an NBT test that allows the visualization of blue formazan crystals inside cells that produced ROS. We compared the formazan crystals formation in control, LAP and IR surgical groups. Yellow and water-soluble NBT is reduced to blue formazan crystals by ROS produced by activated neutrophils (Baehner and Nathan, 1968). The exposure of neutrophils to intestinal IR induced a significantly higher (p < 0.05) amount of formazan (Figure 4), used as a marker of NADPH oxidase activity: 46.5% of the cells contained extensive formazan formation while LAP and control showed 16.9% and 14.4% cells respectively, probably due to baseline production. These results support the hypothesis that neutrophils contribute to the ischemic oxidative stress as shown in (Jaeschke et al., 1992; Arumugam et al., 2004) and may be related to the regulation of antioxidant molecules after IR found in cluster 4, as well as the regulation of SOD, DHFR, and Cathepsin-D found in cluster 1.
Major Functional Classes of Neutrophil Proteome
For the functional classification of the significantly regulated identified proteins, KEGG pathways, and Wikipathways databases were used as a reference knowledge base to understand various signaling mechanisms and pathways (Zhang and Wiemann, 2009; Kutmon et al., 2015). Differentially regulated proteins were mapped to the Rattus norvegicus genome as reference set for enrichment analysis using the online analysis WebGestalt (Wang et al., 2013). Most of the enriched pathways are immune-related indicating the effect of intestinal ischemia and reperfusion on the neutrophil function.
Five proteins from cluster 1 were found to be involved in antigen processing and presentation. They are Hspa8 (heat shock 70kDa protein 8), Hspa5 (heat shock protein 5), Hsp90aa1/Hsp90ab1 [heat shock protein 90, alpha (cytosolic), class A member 1/class B member 1] and Pdia3 (protein disulfide isomerase family A, member 3). Antigen processing and presentation is a well-known phenomenon performed by antigen-presenting cells, including dendritic cells, B cells, macrophages, and thymic epithelial cells, signaling to antigen-specific T cells in order to generate effective adaptive immune responses (Roche and Furuta, 2015). It has been found recently that mouse neutrophils express MHC class II and co-stimulatory molecules like CD80 and CD86, and can prime antigen-specific T cells in an MHC class II-dependent manner (Abi Abdallah et al., 2011). Interestingly the identification of up regulation of the identified proteins in our data set suggests that intestinal ischemia and reperfusion increases antigen processing and presentation process in neutrophils.
TNF-alpha/NF-kB signaling pathway was significantly enriched with eight proteins from cluster 1. TNF-alpha is a multifunctional proinflammatory cytokine, which can induce a wide range of apoptosis and cell survival genes as well as inflammation and immunity-related genes by the NF-kB signaling pathway (Barnes and Karin, 1997; Blackwell and Christman, 1997). It has been shown that up-regulation of TNF-alpha/NF-kB signaling pathway after ischemia and reperfusion induces inflammation and tissue injury by the activated neutrophils (Kin et al., 2006). The identification of up-regulation of eight proteins in this pathway expands the previous results that showed up-regulation of this pathway in activated neutrophils after intestinal ischemia and reperfusion causing more injury to the bystander tissues by ROS production (Tang et al., 2011).
Three proteins with significant up-regulation from cluster 1 were grouped in IL-2 signaling pathway with significant enrichment. They include Gnb2l1 [Guanine nucleotide binding protein (G protein), beta polypeptide 2 like 1], Hsp90aa1 [Heat shock protein 90, alpha (cytosolic), class A member 1], and Stat3 (Signal transducer and activator of transcription 3). Jablons et al. (1990) demonstrated that in vivo administration of IL-2 in humans with advanced cancers suppresses FcγR expression (CD16) and chemotaxis in neutrophils whereas another study had opposite results and was performed in vitro to check the direct effect of IL-2 on the neutrophils functions (Girard et al., 1996). Our results are in accordance with the in vivo analysis as our results show up-regulated IL-2 signaling pathway proteins concomitantly with a down regulation of FcγR signaling proteins after IR. Fc gamma R-mediated phagocytosis down regulation was found in cluster 4 (Table 2) and is further discussed below.
Here Fc gamma R mediated phagocytosis, regulation of actin cytoskeleton and chemokine signaling pathways were found down regulated in IR, as described by the cluster 4 profile. All the down regulated proteins in these pathways could lead to dramatically slower cell migration, improper polarization, transendothelial migration (TEM), and phagocytosis. Studies have reported that WASF requirement for initial spike in actin polymerization correlates with directional sensing. Zhang et al. (2006) observed reduced adhesion followed by reduced migration toward fMLP along with reduced TEM in WASF-deficient neutrophils associated with defective β2-integrin clustering. In addition, WASF may play multiple roles in chemotaxis (Kumar et al., 2012).
Disruption of SHIP1\INPP5D, a primary inositol phosphatase causes accumulation of [a PtdIns(3,4,5)P3 probe] and F-actin polymerization across the cell membrane in neutrophils and as a result the neutrophils become flattened and display irregular polarization and less cell migration (Nishio et al., 2007).
We observed down regulation (cluster 4) of Leukotriene synthesis pathways represented by important enzymes like 15 lipoxygenase, 5 lipoxygenase activating protein (FLAP), and LTA4 hydrolase. Leukotrienes (LTs) are inflammatory mediators causing, for example, phagocyte chemotaxis and increased vascular permeability. LTB4 is a potent chemotactic agent produced by almost all types of immune cells, especially by neutrophils, and its overproduction leads to certain pathological conditions such as lung edema (Pace et al., 2004) and inflammatory bowel disease (Singh et al., 2003). Few studies have shown the effect of down regulation of 5-LO however, one study reported an increased neutrophil infiltration and TNF expression within the myocardial infarction area of 5-LO deficient mice. However inhibition of 5-lipoxygenase did not affect IR related injury of the wild type mice (Adamek et al., 2007). We have also found down regulation of important enzymes of LTB4 synthesis pathway in PMNs after intestinal IR. Similarly, platelets could naturally inhibit the LTB4 synthesis in neutrophils through their spontaneous interactions with these cells. The inhibitory factor can be adenosine as identified in ligand-operated interactions of platelets with neutrophils, but the platelet-derived product responsible for down regulation of neutrophil lipid mediators release and generation remains to be identified. This could have a significant impact on the homeostatic process of inflammation (Chabannes et al., 2003).
It is clear that intestinal obstruction (Sagar et al., 1995) and ischemia (Meddah et al., 2001) cause mucosal injury with a subsequent increase of mucosal permeability and bacterial translocation. Therefore, the up regulation of antimicrobial proteins in cluster 5 like CAMP and Lcn2 observed after IR could be related to protection against bacterial infection and modulation of oxidative stress (Chakraborty et al., 2012).
To validate such findings we performed a phagocytosis assay by incubating neutrophils from the three groups with Saccharomyces cerevisiae yeast cells. Phagocytic activity was significantly decreased in the IR group (p-value < 0.05) compared to control and LAP (Figure 4). Only about 23.90% of cells phagocytosed in IR group while control and LAP presented 50 and 40.7% phagocytosis rates, respectively. The mechanisms for the decreased neutrophils phagocytosis have not been explained yet, but might be related to the down regulation (cluster 4) of SHIP and the five proteins that belong to the Fc gamma R-mediated phagocytosis pathway (Detmers et al., 1987; Ravetch and Kinet, 1991).
As a result of oxidative stress, most of the tissues undergo various anti-oxidative defense mechanisms. In our study, we found various anti-oxidant proteins in neutrophils that have been significantly regulated after IR. It includes glutathione reductase and glutathione S-transferase (GST). GSTs and its isoenzyme activities increase in response to oxidative stress due to lipid peroxidation resulting from superoxide production (Vasieva, 2011). Glutathione S transferase 1 (GSTP1), is a detoxification enzyme and regulator of cell signaling in response to growth factors, hypoxia, stress, and other stimuli in human hepatocellular carcinoma (HCC) (Kou et al., 2013) but its role in neutrophils is not clear. Down regulation of the response to reactive oxygen species was observed in cluster 3 after IR in neutrophil. Their major role in inflammatory and immune responses has long been thought to be phagocytosis and killing of bacteria via the generation of ROS and release of lytic enzymes stored in granules (Root and Cohen, 1981). Especially ROS, since they are potentially toxic essential molecules in the killing of bacteria. PMNs are thought to be exposed to ROS produced by themselves and by other inflammatory cells, and to suffer from resulting damage, such as DNA cleavage, protein modifications, and lipid peroxidation. The ROS-mediated damage to intracellular molecules is considered to be limited by cellular antioxidant enzymes, such as SODs (superoxide dismutase), Glutathione-s-transferase (Hurst et al., 1998) and GPx (glutathione peroxidase) (Arai et al., 1999). Down regulation of Glutathione-s-transferase and peroxidase could limit PMNs role in inflammatory and immune responses such as phagocytosis and killing of bacteria, as confirmed by our functional assays and previously mentioned in the literature (Hattori et al., 2005).
Concluding Remarks
Conclusively, our proteomic approach revealed that intestinal ischemia/reperfusion causes the down regulation of important antioxidants together with the up regulation of enzymes involved in ROS production. This could result in reactive oxygen species accumulation observed by many researchers and confirmed herein. From enzyme classification, cluster based KEGG pathways analysis and phagocytosis assays, we observed the changes in neutrophils motility, phagocytosis, directional migration, and cytoskeletal machinery activation after ischemia and reperfusion. Moreover, regulated pathways and enzymes suggest the influence of IR on the carbohydrate and lipid metabolism and a possible correlation to bacterial translocation, but such findings need further studies.
Collectively, our MS-based quantitative proteomic analysis illustrates the significance of comparative proteomic strategies applied to the neutrophils in different surgical groups, showing results supported by functional assays and consistent with the literature, and lay the basis for further profound studies in neutrophil biology.
Ethics Statement
This study was carried out in accordance with the recommendations of the Ethics committee at the Faculty of Medicine, University of São Paulo. The protocol was approved by the Ethics committee at the Faculty of Medicine, University of São Paulo, registered under protocol number 8186.
Author Contributions
WF and PR: Study idea and design, data analysis, manuscript writing. MT, SA: sample prep, proteomics analyses, manuscript writing. BF: surgical assays. IL, KB, and MC: functional assays, manuscript writing. SS, VS: data analysis, manuscript writing.
Conflict of Interest Statement
The authors declare that the research was conducted in absence of any commercial or financial relationships that could be construed as a potential conflict of interest.
The handling editor declared a shared affiliation, though no other collaboration, with several of the authors, MT, SA, MC, IL, KB, and WF, at time of review.
Acknowledgments
The authors acknowledge TWAS, CNPq, CAPES, FAPESP, FAP-DF, and FUB-UnB for financial support.
Supplementary Material
The Supplementary Material for this article can be found online at: https://www.frontiersin.org/articles/10.3389/fmolb.2018.00089/full#supplementary-material
Abbreviations
CAMP Cathelicidin antimicrobial peptide DTT Dithiothreitol EC number Enzyme commission number FASP Filter aided sample prep FDR False discovery rate fMLP N-Formyl-Met-Leu-Phe GFP-PH-Akt Green fluorescent protein probe for the PH domain of protein kinase B HCD higher energy collisional dissociation HILIC Hydrophilic interaction chromatography IR Ischemia and reperfusion iTRAQ Isobaric tag for relative and absolute quantitation KEGG Kyoto Encyclopedia of Genes and Genomes LC-MS/MS Tandem mass spectrometry liquid chromatography Lcn2 Lipocalin-2 LTB4 Leukotriene B4 LTQ Linear trap quadrupole MOF multiple organ failure PCA Principal Component Analysis PMNs polymorphonuclear leukocytes PtdIns(3, 4, 5)P3, Phosphatidylinositol (3, 4 5)-trisphosphate ROS Reactive oxygen species SDS Sodium dodecyl sulfate SHP-1 SH2-containing tyrosine-specific protein phosphatase SIRS systemic inflammatory response syndrome TEAB Triethylammonium bicarbonate WASF Wiskott-Aldrich syndrome protein XC and CC families of chemokines.
References
Abi Abdallah, D. S., Egan, C. E., Butcher, B. A., and Denkers, E. Y. (2011). Mouse neutrophils are professional antigen-presenting cells programmed to instruct Th1 and Th17 T-cell differentiation. Int. Immunol. 23, 317–326. doi: 10.1093/intimm/dxr007
Adamek, A., Jung, S., Dienesch, C., Laser, M., Ertl, G., Bauersachs, J., et al. (2007). Role of 5-lipoxygenase in myocardial ischemia-reperfusion injury in mice. Eur. J. Pharmacol. 571, 51–54. doi: 10.1016/j.ejphar.2007.05.040
Arai, M., Imai, H., Koumura, T., Yoshida, M., Emoto, K., Umeda, M., et al. (1999). Mitochondrial phospholipid hydroperoxide glutathione peroxidase plays a major role in preventing oxidative injury to cells. J. Biol. Chem. 274, 4924–4933. doi: 10.1074/jbc.274.8.4924
Arumugam, T. V., Shiels, I. A., Woodruff, T. M., Granger, D. N., and Taylor, S. M. (2004). The role of the complement system in ischemia-reperfusion injury. Shock 21, 401–409. doi: 10.1097/00024382-200405000-00002
Baehner, R. L., and Nathan, D. G. (1968). Quantitative nitroblue tetrazolium test in chronic granulomatous disease. N. Engl. J. Med. 278, 971–976. doi: 10.1056/NEJM196805022781801
Barnes, P. J., and Karin, M. (1997). Nuclear factor-kappaB: a pivotal transcription factor in chronic inflammatory diseases. N. Engl. J. Med. 336, 1066–1071. doi: 10.1056/NEJM199704103361506
Biterova, E. I., Turanov, A. A., Gladyshev, V. N., and Barycki, J. J. (2005). Crystal structures of oxidized and reduced mitochondrial thioredoxin reductase provide molecular details of the reaction mechanism. Proc. Natl. Acad. Sci. U.S.A. 102, 15018–15023. doi: 10.1073/pnas.0504218102
Blackwell, T. S., and Christman, J. W. (1997). The role of nuclear factor-kappa B in cytokine gene regulation. Am. J. Respir. Cell Mol. Biol. 17, 3–9. doi: 10.1165/ajrcmb.17.1.f132
Break, T. J., Jun, S., Indramohan, M., Carr, K. D., Sieve, A. N., Dory, L., et al. (2012). Extracellular superoxide dismutase inhibits innate immune responses and clearance of an intracellular bacterial infection. J. Immunol. 188, 3342–3350. doi: 10.4049/jimmunol.1102341
Burge, P. S., Johnson, W. S., and Hayward, A. R. (1976). Neutrophil pyruvate kinase deficiency with recurrent staphylococcal infections: first reported case. Br. Med. J. 1, 742–745. doi: 10.1136/bmj.1.6012.742
Ceppa, E. P., Fuh, K. C., and Bulkley, G. B. (2003). Mesenteric hemodynamic response to circulatory shock. Curr. Opin. Crit. Care 9, 127–132. doi: 10.1097/00075198-200304000-00008
Chabannes, B., Poubelle, P. E., Moliere, P., De Medicis, R., Lussier, A., and Lagarde, M. (2003). Platelets abrogate leukotriene B(4) generation by human blood neutrophils stimulated with monosodium urate monohydrate or f-Met-Leu-Phe in vitro. Lab. Invest. 83, 491–499. doi: 10.1097/01.LAB.0000062855.90029.D8
Chakraborty, S., Kaur, S., Guha, S., and Batra, S. K. (2012). The multifaceted roles of neutrophil gelatinase associated lipocalin (NGAL) in inflammation and cancer. Biochim. Biophys. Acta 1826, 129–169. doi: 10.1016/j.bbcan.2012.03.008
Crissinger, K. D., and Granger, D. N. (1989). Mucosal injury induced by ischemia and reperfusion in the piglet intestine: influences of age and feeding. Gastroenterology 97, 920–926. doi: 10.1016/0016-5085(89)91498-4
Detmers, P. A., Wright, S. D., Olsen, E., Kimball, B., and Cohn, Z. A. (1987). Aggregation of complement receptors on human neutrophils in the absence of ligand. J. Cell Biol. 105, 1137–1145. doi: 10.1083/jcb.105.3.1137
Federzoni, E. A., Valk, P. J. M., Torbett, B. E., Haferlach, T., Löwenberg, B., Fey, M. F., et al. (2012). PU.1 is linking the glycolytic enzyme HK3 in neutrophil differentiation and survival of APL cells. Blood 119, 4963–4970. doi: 10.1182/blood-2011-09-378117
Galkina, S. I., Molotkovsky, J. G., Ullrich, V., and Sud'ina, G. F. (2005). Scanning electron microscopy study of neutrophil membrane tubulovesicular extensions (cytonemes) and their role in anchoring, aggregation and phagocytosis. The effect of nitric oxide. Exp. Cell Res. 304, 620–629. doi: 10.1016/j.yexcr.2004.12.005
Gan, X., Xing, D., Su, G., Li, S., Luo, C., Irwin, M. G., et al. (2015). Propofol attenuates small intestinal ischemia reperfusion injury through inhibiting NADPH oxidase mediated mast cell activation. Oxidative Med. Cell. Longevity 2015:167014. doi: 10.1155/2015/167014
Girard, D., Paquet, M. E., Paquin, R., and Beaulieu, A. D. (1996). Differential effects of interleukin-15 (IL-15) and IL-2 on human neutrophils: modulation of phagocytosis, cytoskeleton rearrangement, gene expression, and apoptosis by IL-15. Blood 88, 3176–3184.
Guneli, E., Cavdar, Z., Islekel, H., Sarioglu, S., Erbayraktar, S., Kiray, M., et al. (2007). Erythropoietin protects the intestine against ischemia/reperfusion injury in rats. Mol. Med. 13, 509–517. doi: 10.2119/2007-00032.Guneli
Hattori, H., Imai, H., Hanamoto, A., Furuhama, K., and Nakagawa, Y. (2005). Up-regulation of phospholipid hydroperoxide glutathione peroxidase in rat casein-induced polymorphonuclear neutrophils. Biochem. J. 389(Pt 2), 279–287. doi: 10.1042/BJ20050006
Hierholzer, C., Kalff, J. C., Audolfsson, G., Billiar, T. R., Tweardy, D. J., and Bauer, A. J. (1999). Molecular and functional contractile sequelae of rat intestinal ischemia/reperfusion injury. Transplantation 68, 1244–1254. doi: 10.1097/00007890-199911150-00006
Huang, J. B., Kindzelskii, A. L., and Petty, H. R. (2002). Hexokinase translocation during neutrophil activation, chemotaxis, and phagocytosis: disruption by cytochalasin D, dexamethasone, and indomethacin. Cell. Immunol. 218, 95–106. doi: 10.1016/S0008-8749(02)00582-8
Hurst, R., Bao, Y., Jemth, P., Mannervik, B., and Williamson, G. (1998). Phospholipid hydroperoxide glutathione peroxidase activity of human glutathione transferases. Biochem. J. 332(Pt 1), 97–100. doi: 10.1042/bj3320097
Iqbal, M. P., Burney, I. A., Sultana, F., Mehboobali, N., and Siddiqui, T. (2000). Increased levels of multiple forms of dihydrofolate reductase in peripheral blood leucocytes of cancer patients receiving haematopoietic colony-stimulating factors: interim analysis. Exp. Mol. Med. 32, 84–87. doi: 10.1038/emm.2000.15
Jablons, D., Bolton, E., Mertins, S., Rubin, M., Pizzo, P., Rosenberg, S. A., et al. (1990). IL-2-based immunotherapy alters circulating neutrophil Fc receptor expression and chemotaxis. J. Immunol. 144, 3630–3636.
Jaeschke, H., Bautista, A. P., Spolarics, Z., and Spitzer, J. J. (1992). Superoxide generation by neutrophils and Kupffer cells during in vivo reperfusion after hepatic ischemia in rats. J. Leukocyte Biol. 52, 377–382. doi: 10.1002/jlb.52.4.377
Jawa, R. S., Quist, E., Boyer, C. W., Shostrom, V. K., and Mercer, D. W. (2013). Mesenteric ischemia-reperfusion injury up-regulates certain CC, CXC, and XC chemokines and results in multi-organ injury in a time-dependent manner. Eur. Cytokine Netw. 24, 148–156. doi: 10.1684/ecn.2014.0345
Kaufmann, S. H. (2008). Immunology's foundation: the 100-year anniversary of the Nobel Prize to Paul Ehrlich and Elie Metchnikoff. Nat. Immunol. 9, 705–712. doi: 10.1038/ni0708-705
Kin, H., Wang, N. P., Halkos, M. E., Kerendi, F., Guyton, R. A., and Zhao, Z. Q. (2006). Neutrophil depletion reduces myocardial apoptosis and attenuates NFkappaB activation/TNFalpha release after ischemia and reperfusion. J. Surg. Res. 135, 170–178. doi: 10.1016/j.jss.2006.02.019
Kou, X., Chen, N., Feng, Z., Luo, L., and Yin, Z. (2013). GSTP1 negatively regulates Stat3 activation in epidermal growth factor signaling. Oncol. Lett. 5, 1053–1057. doi: 10.3892/ol.2012.1098
Kubes, P., Jutila, M., and Payne, D. (1995). Therapeutic potential of inhibiting leukocyte rolling in ischemia/reperfusion. J. Clin. Invest. 95, 2510–2519. doi: 10.1172/JCI117952
Kumar, P., Shen, Q., Pivetti, C. D., Lee, E. S., Wu, M. H., and Yuan, S. Y. (2009). Molecular mechanisms of endothelial hyperpermeability: implications in inflammation. Expert Rev. Mol. Med. 11:e19. doi: 10.1017/S1462399409001112
Kumar, S., Singh, B., Dimmock, J. R., and Sharma, R. K. (2011). N-myristoyltransferase in the leukocytic development processes. Cell Tissue Res. 345, 203–211. doi: 10.1007/s00441-011-1202-x
Kumar, S., Xu, J., Perkins, C., Guo, F., Snapper, S., Finkelman, F. D., et al. (2012). Cdc42 regulates neutrophil migration via crosstalk between WASp, CD11b, and microtubules. Blood 120, 3563–3574. doi: 10.1182/blood-2012-04-426981
Kutmon, M., Riutta, A., Nunes, N., Hanspers, K., Willighagen, E. L., Bohler, A., et al. (2015). WikiPathways: capturing the full diversity of pathway knowledge. Nucleic Acids Res. 44, D488–D494. doi: 10.1093/nar/gkv1024
Kvietys, P. R., and Granger, D. N. (2012). Role of reactive oxygen and nitrogen species in the vascular responses to inflammation. Free Radic. Biol. Med. 52, 556–592. doi: 10.1016/j.freeradbiomed.2011.11.002
Liu, M., and Yokomizo, T. (2015). The role of leukotrienes in allergic diseases. Allergol. Int. 64, 17–26. doi: 10.1016/j.alit.2014.09.001
Luerman, G. C., Uriarte, S. M., Rane, M. J., and McLeish, K. R. (2010). Application of proteomics to neutrophil biology. J. Proteomics 73, 552–561. doi: 10.1016/j.jprot.2009.06.013
Mallick, I. H., Yang, W., Winslet, M. C., and Seifalian, A. M. (2004). Ischemia-reperfusion injury of the intestine and protective strategies against injury. Dig. Dis. Sci. 49, 1359–1377. doi: 10.1023/B:DDAS.0000042232.98927.91
Massberg, S., Gonzalez, A. P., Leiderer, R., Menger, M. D., and Messmer, K. (1998). In vivo assessment of the influence of cold preservation time on microvascular reperfusion injury after experimental small bowel transplantation. Br. J. Surg. 85, 127–133. doi: 10.1046/j.1365-2168.1998.00534.x
McDonald, B., Pittman, K., Menezes, G. B., Hirota, S. A., Slaba, I., Waterhouse, C. C., et al. (2010). Intravascular danger signals guide neutrophils to sites of sterile inflammation. Science 330, 362–366. doi: 10.1126/science.1195491
Meddah, A. T., Leke, L., Romond, M. B., Grenier, E., Cordonnier, C., Risbourg, B., et al. (2001). The effects of mesenteric ischemia on ileal colonization, intestinal integrity, and bacterial translocation in newborn piglets. Pediatr. Surg. Int. 17, 515–520. doi: 10.1007/s003830100582
Miriyala, S., Panchatcharam, M., Ramanujam, M., and Puvanakrishnan, R. (2013). A novel tetrapeptide derivative exhibits in vitro inhibition of neutrophil-derived reactive oxygen species and lysosomal enzymes release. Oxid. Med. Cell. Longev. 2013:853210. doi: 10.1155/2013/853210
Mojzis, J., Hviscova, K., Germanova, D., Bukovicova, D., and Mirossay, L. (2001). Protective effect of quercetin on ischemia/reperfusion-induced gastric mucosal injury in rats. Physiol. Res. Academia Scientiarum Bohemoslovaca 50, 501–506.
Mondal, S., Subramanian, K. K., Sakai, J., Bajrami, B., and Luo, H. R. (2012). Phosphoinositide lipid phosphatase SHIP1 and PTEN coordinate to regulate cell migration and adhesion. Mol. Biol. Cell 23, 1219–1230. doi: 10.1091/mbc.e11-10-0889
Morris, C. F., Castro, M. S., and Fontes, W. (2008). Neutrophil proteome: lessons from different standpoints. Protein Pept. Lett. 15, 995–1001. doi: 10.2174/092986608785849371
Nadel, J. A., Conrad, D. J., Ueki, I. F., Schuster, A., and Sigal, E. (1991). Immunocytochemical localization of arachidonate 15-lipoxygenase in erythrocytes, leukocytes, and airway cells. J. Clin. Investig. 87, 1139–1145. doi: 10.1172/JCI115110
Nishio, M., Watanabe, K., Sasaki, J., Taya, C., Takasuga, S., Iizuka, R., et al. (2007). Control of cell polarity and motility by the PtdIns(3,4,5)P3 phosphatase SHIP1. Nat. Cell Biol. 9, 36–44. doi: 10.1038/ncb1515
Pace, E., Profita, M., Melis, M., Bonanno, A., Paterno, A., Mody, C. H., et al. (2004). LTB4 is present in exudative pleural effusions and contributes actively to neutrophil recruitment in the inflamed pleural space. Clin. Exp. Immunol. 135, 519–527. doi: 10.1111/j.1365-2249.2003.02387.x
Paige, M., Wang, K., Burdick, M., Park, S., Cha, J., Jeffery, E., et al. (2014). Role of leukotriene A4 hydrolase aminopeptidase in the pathogenesis of emphysema. J. Immunol. 192, 5059–5068. doi: 10.4049/jimmunol.1400452
Pereira, S., and Lowell, C. (2003). The Lyn tyrosine kinase negatively regulates neutrophil integrin signaling. J. Immunol. 171, 1319–1327. doi: 10.4049/jimmunol.171.3.1319
Rao, R. M., Yang, L., Garcia-Cardena, G., and Luscinskas, F. W. (2007). Endothelial-dependent mechanisms of leukocyte recruitment to the vascular wall. Circ. Res. 101, 234–247. doi: 10.1161/CIRCRESAHA.107.151860b
Riaz, A. A., Wan, M. X., Schaefer, T., Schramm, R., Ekberg, H., Menger, M. D., et al. (2002). Fundamental and distinct roles of P-selectin and LFA-1 in ischemia/reperfusion-induced leukocyte-endothelium interactions in the mouse colon. Ann. Surg. 236, 777–784; discussion 84. doi: 10.1097/00000658-200212000-00010
Roche, P. A., and Furuta, K. (2015). The ins and outs of MHC class II-mediated antigen processing and presentation. Nat. Rev. Immunol. 15, 203–216. doi: 10.1038/nri3818
Rodrigues, S. F., and Granger, D. N. (2010). Role of blood cells in ischaemia-reperfusion induced endothelial barrier failure. Cardiovasc. Res. 87, 291–299. doi: 10.1093/cvr/cvq090
Root, R. K., and Cohen, M. S. (1981). The microbicidal mechanisms of human neutrophils and eosinophils. Rev. Infect. Dis. 3, 565–598. doi: 10.1093/clinids/3.3.565
Sagar, P. M., MacFie, J., Sedman, P., May, J., Mancey-Jones, B., and Johnstone, D. (1995). Intestinal obstruction promotes gut translocation of bacteria. Dis. Colon Rectum 38, 640–644. doi: 10.1007/BF02054126
Schwammle, V., and Jensen, O. N. (2010). A simple and fast method to determine the parameters for fuzzy c-means cluster analysis. Bioinformatics 26, 2841–2848. doi: 10.1093/bioinformatics/btq534
Singh, V. P., Patil, C. S., Jain, N. K., Singh, A., and Kulkarni, S. K. (2003). Effect of nimesulide on acetic acid- and leukotriene-induced inflammatory bowel disease in rats. Prostaglandins Other Lipid Mediat. 71, 163–175. doi: 10.1016/S1098-8823(03)00038-8
Snelgrove, R. J., Jackson, P. L., Hardison, M. T., Noerager, B. D., Kinloch, A., Gaggar, A., et al. (2010). A critical role for LTA4H in limiting chronic pulmonary neutrophilic inflammation. Science 330, 90–94. doi: 10.1126/science.1190594
Sriramarao, P., Berger, E., Chambers, J. D., Arfors, K. E., and Gehlsen, K. R. (1993). High mannose type N-linked oligosaccharides on endothelial cells may influence beta 2 integrin mediated neutrophil adherence in vitro. J. Cell. Biochem. 51, 360–368. doi: 10.1002/jcb.240510316
Tang, C., Xue, H. L., Bai, C. L., and Fu, R. (2011). Regulation of adhesion molecules expression in TNF-alpha-stimulated brain microvascular endothelial cells by tanshinone IIA: involvement of NF-kappaB and ROS generation. Phytothe. Res. PTR 25, 376–380. doi: 10.1002/ptr.3278
Vasieva, O. (2011). The many faces of glutathione transferase pi. Curr. Mol. Med. 11, 129–139. doi: 10.2174/156652411794859278
Vitturi, D. A., Chen, C. S., Woodcock, S. R., Salvatore, S. R., Bonacci, G., Koenitzer, J. R., et al. (2013). Modulation of nitro-fatty acid signaling: prostaglandin reductase-1 is a nitroalkene reductase. J. Biol. Chem. 288, 25626–25637. doi: 10.1074/jbc.M113.486282
Vorbach, C., Harrison, R., and Capecchi, M. R. (2003). Xanthine oxidoreductase is central to the evolution and function of the innate immune system. Trends Immunol. 24, 512–517. doi: 10.1016/S1471-4906(03)00237-0
Wang, J., Duncan, D., Shi, Z., and Zhang, B. (2013). WEB-based GEne SeT AnaLysis Toolkit (WebGestalt): update 2013. Nucleic Acids Res. 41(Web Server issue), W77–83. doi: 10.1093/nar/gkt439
Wasserberg, N., Pileggi, A., Salgar, S. K., Ruiz, P., Ricordi, C., Inverardi, L., et al. (2007). Heme oxygenase-1 upregulation protects against intestinal ischemia/reperfusion injury: a laboratory based study. Int. J. Surg. 5, 216–224. doi: 10.1016/j.ijsu.2006.06.001
Watanabe, T., Tanigawa, T., Kobata, A., Takeda, S., Nadatani, Y., Otani, K., et al. (2014). Toll-like receptor 2 mediates ischemia-reperfusion injury of the small intestine in adult mice. PLoS ONE 9:e110441. doi: 10.1371/journal.pone.0110441
Wu, Q. L., Fu, Y. F., Zhou, W. L., Wang, J. X., Feng, Y. H., Liu, J., et al. (2005). Inhibition of S-adenosyl-L-homocysteine hydrolase induces immunosuppression. J. Pharmacol. Exp. Ther. 313, 705–711. doi: 10.1124/jpet.104.080416
Xie, X. L. L., and Beni, G. (1991). A validity measure for fuzzy clustering. IEEE Trans Pattern Anal. 13, 841–847. doi: 10.1109/34.85677
Zhang, H., Schaff, U. Y., Green, C. E., Chen, H., Sarantos, M. R., Hu, Y., et al. (2006). Impaired integrin-dependent function in Wiskott-Aldrich syndrome protein-deficient murine and human neutrophils. Immunity 25, 285–295. doi: 10.1016/j.immuni.2006.06.014
Keywords: ischemia reperfusion, neutrophils, proteomics, systemic inflammatory response, LC-MS/MS
Citation: Tahir M, Arshid S, Fontes B, Castro MS, Luz IS, Botelho KLR, Sidoli S, Schwämmle V, Roepstorff P and Fontes W (2018) Analysis of the Effect of Intestinal Ischemia and Reperfusion on the Rat Neutrophils Proteome. Front. Mol. Biosci. 5:89. doi: 10.3389/fmolb.2018.00089
Received: 10 December 2017; Accepted: 04 October 2018;
Published: 29 November 2018.
Edited by:
Aline Maria Araujo Martins, Universidade de Brasília, BrazilReviewed by:
Francisco Solano, Universidad de Murcia, SpainEdouard Nice, Monash University, Australia
Copyright © 2018 Tahir, Arshid, Fontes, Castro, Luz, Botelho, Sidoli, Schwämmle, Roepstorff and Fontes. This is an open-access article distributed under the terms of the Creative Commons Attribution License (CC BY). The use, distribution or reproduction in other forums is permitted, provided the original author(s) and the copyright owner(s) are credited and that the original publication in this journal is cited, in accordance with accepted academic practice. No use, distribution or reproduction is permitted which does not comply with these terms.
*Correspondence: Wagner Fontes, d2FnbmVyZkB1bmIuYnI=
†These authors have contributed equally to this work
‡Present Address: Simone Sidoli, Epigenetics Program, Department of Biochemistry and Biophysics, Perelman School of Medicine, University of Pennsylvania, Philadelphia, PA, United States