- 1Unidad de Investigación Médica en Enfermedades Infecciosas y Parasitarias, Hospital de Pediatría, Centro Médico Nacional Siglo XXI, Instituto Mexicano del Seguro Social, Mexico City, Mexico
- 2Departamento de Microbiología, Escuela Nacional de Ciencias Biológicas, Instituto Politécnico Nacional, Mexico City, Mexico
- 3Instituto de Ciencias Básicas e Ingeniería, Universidad Autónoma del Estado de Hidalgo, Carretera Pachuca-Tulancingo Km 4.5 Mineral de la Reforma, Hidalgo, Mexico
- 4Centro de Detección Biomolecular, Benemérita Universidad Autónoma de Puebla, Puebla, Mexico
Enterobacter cloacae has emerged as an opportunistic pathogen in healthcare-associated infections. Analysis of the genomic sequences of several E. cloacae strains revealed the presence of genes that code for expression of at least one type VI secretion system (T6SS). Here, we report that E. cloacae strain ATCC 13047 codes for two functional T6SS named T6SS-1 and T6SS-2. T6SS-1 and T6SS-2 were preferentially expressed in tryptic soy broth and tissue culture medium (DMEM), respectively. Mutants in T6SS-1-associated genes clpV1 and hcp1 significantly affected their ability of inter- and intra-bacterial killing indicating that T6SS-1 is required for bacterial competition. In addition, the Hcp effector protein was detected in supernatants of E. cloacae cultures and a functional T6SS-1 was required for the secretion of this protein. A clpV2 mutant was impaired in both biofilm formation and adherence to epithelial cells, supporting the notion that these phenotypes are T6SS-2 dependent. In vivo data strongly suggest that both T6SSs are required for intestinal colonization because single and double mutants in clpV1 and clpV2 genes were defective in gut colonization in mice. We conclude that the two T6SSs are involved in the pathogenesis scheme of E. cloacae with specialized functions in the interaction with other bacteria and with host cells.
Introduction
Enterobacter cloacae are Gram-negative, facultative anaerobic and rod-shaped bacteria belonging to the order Enterobacterales (McAdam, 2020). These bacteria are saprophytic in the environment and are also part of the human gut microbiota (Mezzatesta et al., 2012). Currently, six species of the Enterobacter cloacae complex have been described: E. asbuariae, E. cloacae, E. hormaechei, E. kobei, E. ludwigii, and E. nimipressuralis (Paauw et al., 2008). E. cloacae is a human opportunistic pathogen that is frequently associated with hospital-acquired infections of the lower respiratory tract, urinary tract, and meninges (Liu et al., 2013). Despite the relevance of E. cloacae as a nosocomial pathogen, its pathogenicity mechanisms are not yet fully understood, but biofilm formation is a virulence feature of this opportunistic microorganism (Zhou et al., 2014; Zurob et al., 2019). In addition, some E. cloacae strains possess cytotoxic activity, suggesting the secretion of bacterial toxins to the cell host (Barnes et al., 1997; Krzyminska et al., 2009).
Successful host colonization by a bacterial pathogen depends on functional secretory systems, which translocate and secrete several effectors proteins into host cells or to the extracellular environment, respectively, with the purpose of competing with the host microbiota (Pukatzki et al., 2006). The type 6 secretion system (T6SS) is a multi-proteinaceous complex encoded by large gene regions found in 25% of all sequenced Gram-negative bacteria (Boyer et al., 2009). Interestingly, the T6SS core proteins share structural homology with proteins that form the T4 bacteriophage tail (Bingle et al., 2008; Boyer et al., 2009; Ho et al., 2014).
At least 13 core components (tssA to tssM) are required for the assembly of a functional T6SS (Cherrak et al., 2019; Navarro-Garcia et al., 2019). The tail-like structure is formed of Hcp (hemolysin-coregulated protein) homo-hexamers, which are heaped and enveloped by heterodimers of TssB/TssC proteins forming a sheath-type structure (Mougous et al., 2006; Zoued et al., 2014). Located at the tip of the inner tube formed by the Hcp homo-hexamers, the VgrG/PAAR complex functions as a cell-puncturing device for injection of multiple effectors into target cells (Shneider et al., 2013; Douzi et al., 2016). The ClpV protein is an AAA+ ATPase that disassembles the contracted tubule, recycling different components of the tail-like structure, maintaining the membrane complex stability, which can be reused for multiple sheath-like assemblies (Kube et al., 2014).
Different studies have described the role of T6SS in virulence, immunomodulation, persistence, adherence, and invasion to epithelial cells, biofilm formation, and inter-bacterial competition (Ma et al., 2009; Hood et al., 2010; de Pace et al., 2011; Lertpiriyapong et al., 2012; Weyrich et al., 2012; Russell et al., 2014; Repizo et al., 2015; Gallique et al., 2017). The role of T6SS in E. cloacae remains unknown and so far has not been implicated in the virulence of this opportunistic pathogen. Recent comparative genome analysis of different E. cloacae strains showed that E. cloacae ATCC 13047 possesses two different T6SS-like clusters (Liu et al., 2013) that we named T6SS-1 and T6SS-2 (Figure 1), suggesting that the presence of two T6SS could provide adaptive advantages for E. cloacae in bacterial competition and virulence, as it has been reported for other bacteria that possess two or more T6SS clusters (Journet and Cascales, 2016; Chen et al., 2019).
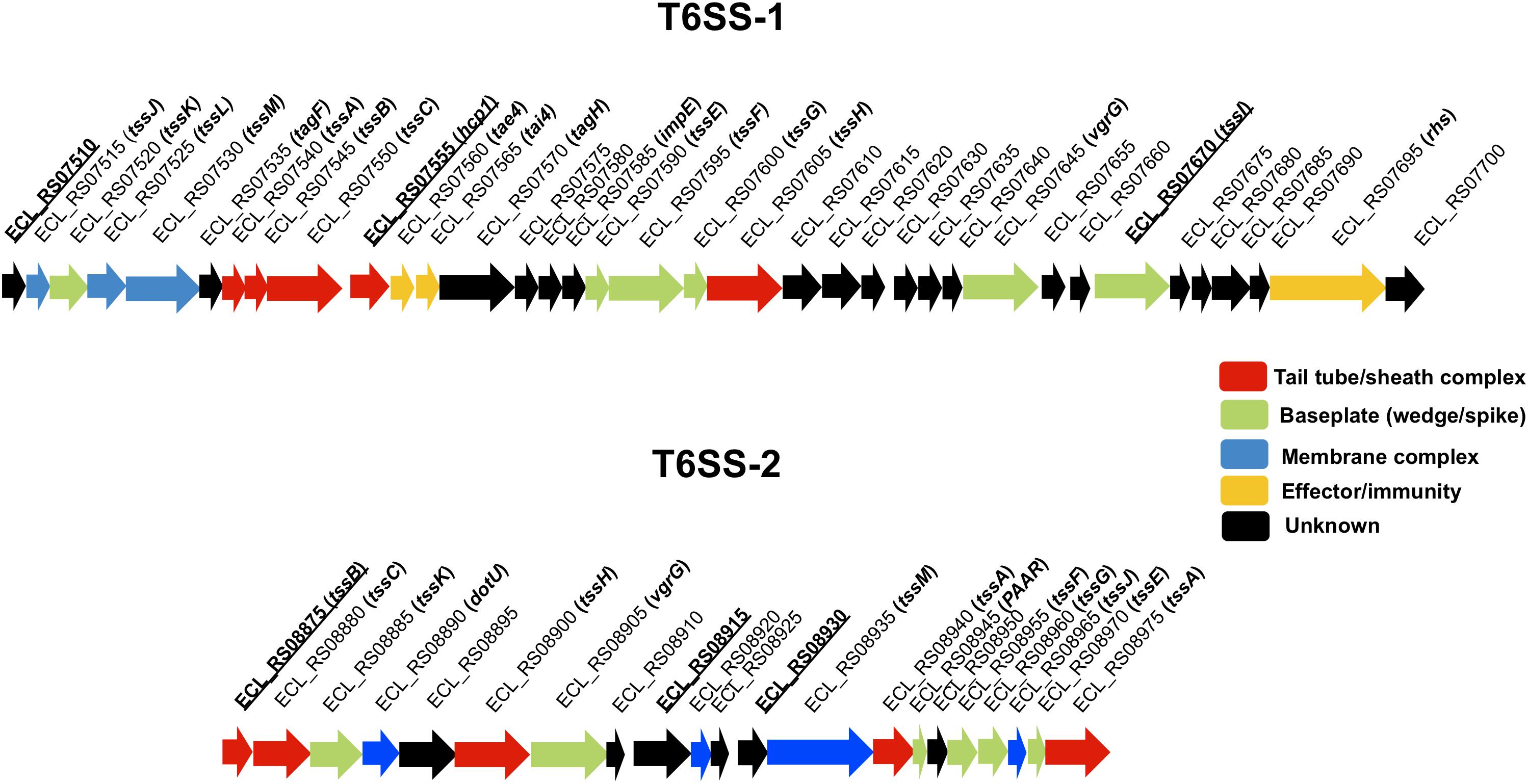
Figure 1. Genetic organization of T6SS-1 and T6SS-2 of E. cloacae ATCC 13047. Genes are plotted as arrows in order according to their genomic positions. The underline text represents the genes whose expression was quantified by RT-qPCR and are the first genes of putative polycistronic operons. Tail tube/sheath complex, baseplate, membrane complex, effector/immunity, and unknown functions are colored in red, green, blue, yellow, and black, respectively.
In this work, we described the role of both E. cloacae T6SS-1 and T6SS-2 in inter-bacterial competition, biofilm formation, adherence to cells, and intestinal colonization. We found that T6SS-1 is required for inter-bacterial competition because mutants of E. cloacae deficient in T6SS-1 were not able to kill E. coli and another Gram-negative enterobacteria as the parental strain did. Virulence phenotypes such as biofilm formation and cell adherence were found to be T6SS-2 dependent. Finally, in vivo studies showed that both T6SSs are required for the colonization of mice gut. Our results show that both T6SSs are virulence factors that confer E. cloacae the ability to survive in different environments and ecological niches and colonize different hosts.
Materials and Methods
Bacterial Strains and Culture Conditions
Bacterial strains and plasmids used in this study are listed in Table 1. To analyze the expression of T6SS genes, several bacteriological broths such as lysogenic broth (LB), trypticase soy broth (TSB), Dulbecco’s Modified Eagle’s Medium (DMEM) with high glucose (4.5 g/l), pleuropneumoniae-like organisms (PPLO) broth, and colonization factor antigen (CFA) broth were used. When necessary, media were supplemented with antibiotics: ampicillin (200 μg/ml), kanamycin (50 μg/ml), and tetracycline (10 μg/ml).
Construction of E. cloacae Mutants
Enterobacter cloacae was targeted for mutagenesis of clpV1, hcp1, and clpV2, following the procedure previously reported (Datsenko and Wanner, 2000) with some modifications. Each purified PCR product was electroporated into competent E. cloacae carrying the lambda-Red recombinase helper plasmid pKD119, whose expression was induced by adding L-(+)-arabinose (Sigma) at a final concentration of 1.0%. PCR fragments containing clpV1, clpV2, and hcp1 sequences flanking a kanamycin cassette were generated using gene-specific primer pairs (Table 2), and the pKD4 plasmid was used as template. The resistance genes were eliminated by using FLP recombinase from pCP20 plasmid. All mutations were verified by PCR and sequencing.
Construction of Plasmids
Plasmids pT3-Hcp1, pT3-ClpV1, and pT3-ClpV2 were generated by cloning of a PCR product containing the corresponding hcp1, clpV1, and clpV2 regions of E. cloacae, respectively, into the pMPM-T3 plasmid (see primers in Table 2). The PCR products were digested with HindIII/BamHI for hcp1, and KpnI/BamHI for clpV1 and clpV2. The digested PCR products were ligated into pMPM-T3, which was also previously digested with the same enzymes. The identity of the insert was confirmed by DNA sequencing.
Quantitative RT-PCR
Total RNA extraction was performed using the hot phenol method as described (Jahn et al., 2008). DNA was removed with TURBO DNA-free (Ambion) and the quality of RNA was assessed using a NanoDrop (ND-1000; Thermo Scientific) and an Agilent 2100 bioanalyzer with a Picochip (Agilent Technologies). The absence of contaminating DNA was controlled by lack of amplification products after 35 qPCR cycles. cDNA was prepared using 1 μg of RNA, random hexamer primers (0.2 μg/μl), and a M-MulV-RT (20 U/μl, reverse transcriptase of Moloney Murine Leukemia Virus; Thermo Fisher Scientific). Specific primers were designed with the Primer3Plus software1 and are listed in Table 2. Quantitative RT-PCR was performed in a Lightcycler 480 instrument (Roche). Control reactions with no template (water) and minus-reverse transcriptase (RNA) were run with all reactions. 16S rRNA was used as a reference gene for normalization and the relative gene expression was calculated using the 2−ΔΔCt method (Livak and Schmittgen, 2001). Expression of 16S rRNA remained unaffected in all conditions tested (Supplementary Figure S1). These experiments were performed in triplicate on three independent times.
Bacterial Competition
Experiments were done essentially as previously described (Repizo et al., 2015), with some modifications. Briefly, the different E. cloacae, E. coli, Klebsiella, and Salmonella strains were grown overnight with aeration in 5 ml of LB broth containing the appropriate antibiotics, and overnight cultures were then adjusted up to OD600 nm ∼1.0 and they were mixed in 4:1 ratio (predator/prey). Aliquots of 20 μl of the mixed bacterial culture were spotted onto LB agar and incubated at 37°C for 4 h. The bacterial spot on the agar surface was subsequently removed, vigorously resuspended in PBS, and the colony-forming units (CFU) per milliliter of surviving prey strains were measured by plating serial dilutions on solid selective media. The selective medium contained 50 μg/ml of kanamycin for prey strains previously transformed with pMPM-K6 plasmid. The output/input ratio of the prey to predator strains was interpreted as survival and includes a minimum of three independent assays.
Hcp Secretion Analysis
E. cloacae strains were grown in TSB with aeration in 35 ml of LB broth at 37°C (containing the appropriate antibiotics) until OD600 nm ∼1.0. Supernatants of culture (25 ml) were centrifuged at 4000 × g followed by filtration (0.22 μm) and concentration using Amicon Ultra-15 centrifuge filters. Concentrated supernatants were resuspended in Laemmli sample buffer (Bio-Rad) and analyzed by 16% SDS-PAGE. Polyacrylamide gels were stained using Coomassie Brilliant Blue.
Liquid Chromatography–Tandem Mass Spectrometry (LC-MS/MS)
In-gel digestion and mass spectrometry (LC-MS/MS) were performed as previously described (Garcia-Morales et al., 2017). Protein bands were cut and treated with 10 mM DTT in 50 mM NH4HCO3 at 56°C for 45 min. After incubation, DTT was replaced by 55 mM iodoacetamide. The dried gel fragments were incubated at 50°C for 1 h with 50 mM NH4HCO3 containing 0.01% of ProteaseMAX Surfactant (Promega, United States). The resulting peptides were tested on an LC-MS system consisting of a fluid flow micro-chromatograph Accela with “spliter” (1/20) and a mass spectrometer LTQ-Orbitrap Velos (Thermo-Fisher, San Jose, CA, United States) with an electrospray ionization system. Fragmentation data were captured in a dependent manner according to predetermined loads with an isolation width of 3.0 (m/z), normalized collision energy of 35 arbitrary units, Q activation of 0.250, activation time of 40 ms, and a maximum injection time of 10 ms per micro-scan. The resultant MS/MS data were searched against the NCBI non-redundant database2, and the identification of proteins was determined by using Protein Prospector version 5.10.17 (San Francisco, CA, United States3). Mass tolerances for precursor ions and fragment ions were set to 20 ppm and 0.2 Da, respectively. In all cases, match punctuations were less than 5 ppm. A discriminant score was carried out for each analyzed peptide; this value is the combination of two measurements of the search result. One is the expectation value for the peptide match (“FDR,” a measure of the likelihood that a match is random) and the other is a “best peptide score,” which takes into account the fact that if a protein has been confidently identified in a sample, it is more likely that other peptides from the same protein will be identified (San Francisco, CA, United States see text footnote 3).
Hemolysis Quantification
The quantification of bacterial hemolysis was performed as described (Soto et al., 2017). E. cloacae strains were grown in TSB until the OD600 nm = 1.0, and 0.5 ml culture was added to 0.5 ml of a 4% (v/v) red blood cells (RBC)/saline solution (0.9% NaCl), and centrifuged at 2500 × g for 1 min. After 4 h of incubation at 37°C, the bacteria/RBC mix was resuspended, and cells were centrifuged at 12,000 × g for 1 min, and the hemoglobin released into the supernatant was determined by measuring the OD at 450 nm. The complete hemolysis was expressed in percentage with respect to the lysis obtained with wild-type E. cloacae strain. These experiments were performed in triplicate on three independent times.
Biofilm Formation
Adhesion to abiotic surface (polystyrene) was analyzed using 96-well plates as described previously (Ares et al., 2016). Overnight cultures of bacteria grown in LB broth (10 μl) were added to 1 ml of DMEM. This volume was distributed in quintuples (100 μl per well) into a 96-well plate and incubated at room temperature for 24 h. To remove loosely attached bacteria, the culture was removed from the wells and gently rinsed three times with PBS and bound bacteria were stained with 1% crystal violet (CV) and incubated for 20 min at room temperature. After incubation, the wells were rinsed three times with phosphate buffered saline (PBS), and the dye was solubilized in 100 μl of ethanol 70%. Finally, the amount of extracted crystal violet was determined by measuring the OD600 using an ELISA Multiskan Plate reader (Thermo Scientific). These experiments were performed in triplicate on three independent times.
Bacterial Adherence
Monolayers of HeLa (ATCC CCL-2) cell line (7 × 105 cells/well) were infected with the indicated strains of an LB broth overnight culture at a multiplicity of infection (MOI) of 100. Epithelial cells were grown in DMEM with 10% fetal bovine serum (FBS). After infection, eukaryotic cells were incubated in DMEM with no FBS for 2 h at 37°C under an atmosphere of 5% CO2. After 2 h of incubation period, cells were washed three times with PBS and then lysed with a solution of 0.1% Triton X-100 for 15 min. After homogenization, the lysates containing total cell-associated bacteria were diluted serially in PBS and plated onto LB agar plates to enumerate adherent bacteria. The results shown are the mean of at least three experiments performed in triplicate on different days.
Phagocytosis of Bacteria by Macrophages
THP-1 (ATCC TIB-202) human monocytes differentiated to macrophages with 200 nM of phorbol 12-myristate 13-acetate for 24 h (6 × 105) were seeded into 24-well tissue culture plates. Bacteria were grown in 5 ml of LB broth overnight at 37°C. Macrophages were infected with a MOI of 100 in a final volume of 1 ml RPMI 1640 tissue culture medium supplemented with 10% heat-activated FBS. Plates were incubated at 37°C under a humidified 5% CO2 atmosphere. After 2 h, cells were rinsed three times with sterile PBS and incubated for an additional 60 min with 1 ml of RPMI 1640 containing 10% FBS and gentamycin (100 μg/ml) to eliminate extracellular bacteria. Cells were then rinsed three times with sterile PBS and lysed with 0.1% Triton X-100. After homogenization, serial dilutions were plated onto LB agar to enumerate total CFUs.
Ethics Statement
Animal experimentation was conducted in strict accordance with good animal practice as defined by the use of laboratory animals and quality requirements, in agreement with animal welfare bodies from Mexico (SAGARPA NOM-062-ZOO-1999: “Technical Specifications for the Production, Care and Use of Laboratory Animals”). All animal work was approved by the Internal Ethics Committee of the Animal Resource Facility of the Universidad Autónoma del Estado de Hidalgo (approval number: CICUAL/016/2019R to Carlos A. Gómez-Aldapa).
Mouse Inoculation Experiments
Mice infection experiments were performed using BALB/c strain. Mice groups (n = 6) were pretreated with 50 mg of streptomycin 24 h before infection with E. cloacae strains. Mice were infected by intragastric (i.g.) inoculation with 1 × 109 CFU/ml of bacteria under sterile conditions. Fresh fecal pellets were collected directly into microtubes at 3 and 6 days post-infection (p.i.). Pellets were resuspended vigorously in sterile PBS 1×, and CFUs per gram of feces were determined by plating serial dilutions on LB agar plates with ampicillin (200 μg/ml).
Statistical Analysis
Prism 5 (GraphPad) was used for statistical differences. One-way ANOVA followed by Tukey’s multiple comparison test and unpaired Student’s t test was performed. A p-value ≤ 0.05 was considered statistically significant.
Results
Identification and Expression Analysis of the T6SSs in E. cloacae
The genome of E. cloacae ATCC 13047 codes for two putative T6SS loci (Liu et al., 2013), which we named T6SS-1 and T6SS-2 (Figure 1). T6SS-1 appears to be complete as it contains hcp (named hcp1) secreted protein-encoding gene as well as the clpV ATPase-encoding gene (named clpV1), among other core and accessory gene components (Figure 1; Supplementary Table S1), suggesting that T6SS-1 could be functional in E. cloacae. In the T6SS-2 loci, the hcp core gene is missing; however, it contains genes such as clpV (named clpV2), vgrG, PAAR, tssM, and other core components (Figure 1; Supplementary Table S1). In both clusters, proteins homologous to T6SS components were found in enterobacteria such as Salmonella enterica serovar Typhimurium and enteroaggregative Escherichia coli (Supplementary Table S1).
We evaluated gene expression of both T6SS-1 and T6SS-2 on 6 h of growth of E. cloacae in different culture media by RT-qPCR, determining the mRNA levels of three different genes of each genetic cluster, which are the first genes of putative operons. Transcription of ECL_RS07510, ECL_RS07555, and ECL_RS07670 genes, which encompass T6SS-1, was enhanced when E. cloacae was grown in TSB (Figure 2A). In contrast, ECL_RS08875, ECL_RS08915, and ECL_RS08930 genes, belonging to T6SS-2, were highly expressed in DMEM (Figure 2B). Differences observed in genetic organization and expression in both gene clusters could suggest different roles of these secretion systems in the pathogenesis of E. cloacae ATCC 13047.
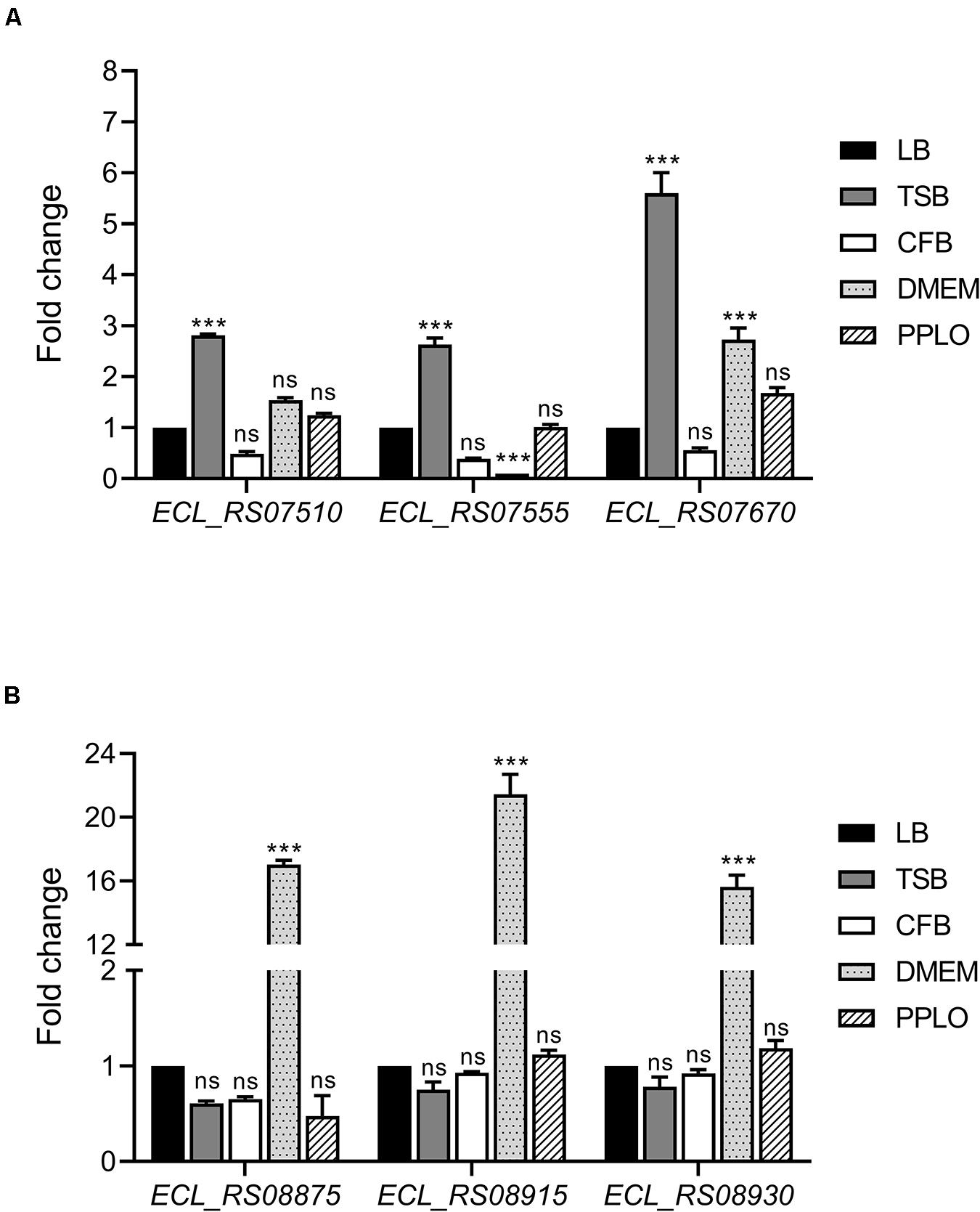
Figure 2. E. cloacae T6SS-1 and T6SS-2 are differentially expressed in TSB and DMEM. Fold change expression (RT-qPCR) of T6SS-1 (A) and T6SS-2 genes (B), compared with LB medium. E. cloacae strains were grown in different culture media: lysogenic broth (LB), trypticase soy broth (TSB), Dulbecco’s Modified Eagle’s Medium (DMEM) with high glucose (4.5 g/l), pleuropneumoniae-like organisms (PPLO) broth, and colonization factor antigen (CFA) broth. Bacterial cultures were grown at 37°C for 6 h. 16S rRNA was used as a reference gene for normalization. Data represent the mean of three independent experiments performed in triplicate. Statistically significant with respect to the WT bacteria grown in LB medium; ns: not significant; ***p < 0.001.
T6SS-1 Is Required for Bacterial Competition
Several findings reveal that T6SSs have bactericidal activity against different bacterial species (MacIntyre et al., 2010; Murdoch et al., 2011; Repizo et al., 2015). To investigate the role of both T6SSs in this phenotype, we initially focused in the deletion of clpV1 and clpV2 genes, which code for ATPases of each T6SS, and they are required for a functional system. E. coli DH5α was used as a target strain in the antibacterial competition assay. While wild-type E. cloacae strain was able to kill E. coli, the ability of ΔclpV1 mutant to outcompete against E. coli was abolished (Figure 3A). In contrast, the absence of a functional T6SS-2, observed in the ΔclpV2 mutant, did not affect the E. cloacae competition against E. coli (Figure 3A). As further confirmation of the contribution of the T6SS-1 to the killing of E. coli, the phenotype of the Δhcp1 mutant was also analyzed. The absence of the Hcp1 protein impaired the ability of E. cloacae to kill E. coli, showing a similar phenotype to the absence of ClpV1 ATPase (Figure 3A). Complementation of the Δhcp1 mutant restored E. coli killing to wild-type E. cloacae levels.
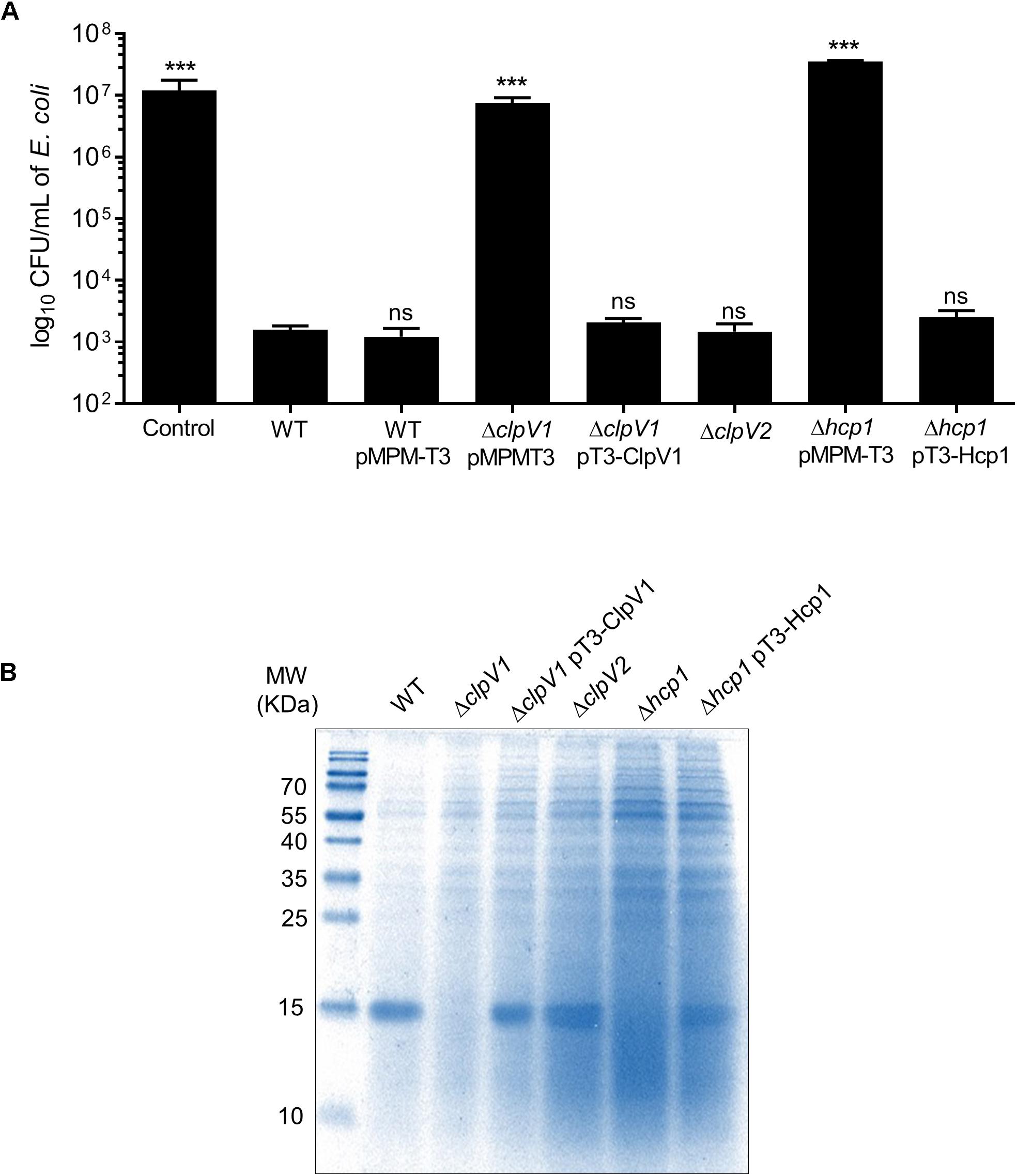
Figure 3. E. cloacae T6SS-1 is required for the bacterial competition. (A) Comparison of the survival of E. coli DH5α against wild-type E. cloacae and ΔclpV1, ΔclpV2, and Δhcp1 mutants. Survival rates are expressed in CFU/ml. Control: E. coli DH5α in LB with no E. cloacae strains. (B) Hcp1 protein was detected by 16% SDS-PAGE and Coomasie Blue staining, from concentrated supernatants of E. cloacae strains grown at 6 h in TSB medium. Statistically significant with respect to the wild-type strain; ns: not significant; ***p < 0.001.
To demonstrate if T6SS-1 was functional, we performed protein secretion assays to detect the Hcp1 protein from supernatants recovered of bacterial culture grown at 6 h in TSB. A clear band was observed in the wild-type strain that it was absent in the ΔclpV1 and the Δhcp1 mutants (Figure 3B). We detected the band in the complemented ΔclpV1 and Δhcp1 mutants and the ΔclpV2 single mutant. These 17-kDa bands were excised from Coomassie-stained gel and verified by LC-MS/MS analysis, corroborating the identity of the Hcp protein (Supplementary Table S2). These results demonstrate that killing of E. coli by E. cloacae and Hcp1 protein secretion are dependent on a functional T6SS-1.
E. cloacae T6SS-1 Is Involved in the Bacterial Competition Against Different E. coli Pathotypes and Other Gram-Negative Pathogens
We wanted to explore the impact of the antibacterial activity of E. cloacae on diarrheagenic and non-diarrheagenic pathotypes of E. coli, such as enteropathogenic (EPEC), enterohemorrhagic (EHEC), enterotoxigenic (ETEC), enteroaggregative (EAEC), uropathogenic (UPEC), and avian-pathogenic (APEC) E. coli. As shown in Figure 4A, wild-type E. cloacae strain caused a reduction between 102- and 103-fold in killing of the EPEC, EHEC, ETEC, EAEC, APEC, and UPEC strains. Interestingly, this loss of viability of E. coli pathotypes was caused by the E. cloacae T6SS-1 because the absence of Hcp1 resulted in the lack of killing activity of E. cloacae (Figure 4A). The complemented Δhcp1 mutant restored the antibacterial activity of E. cloacae against E. coli pathotypes.
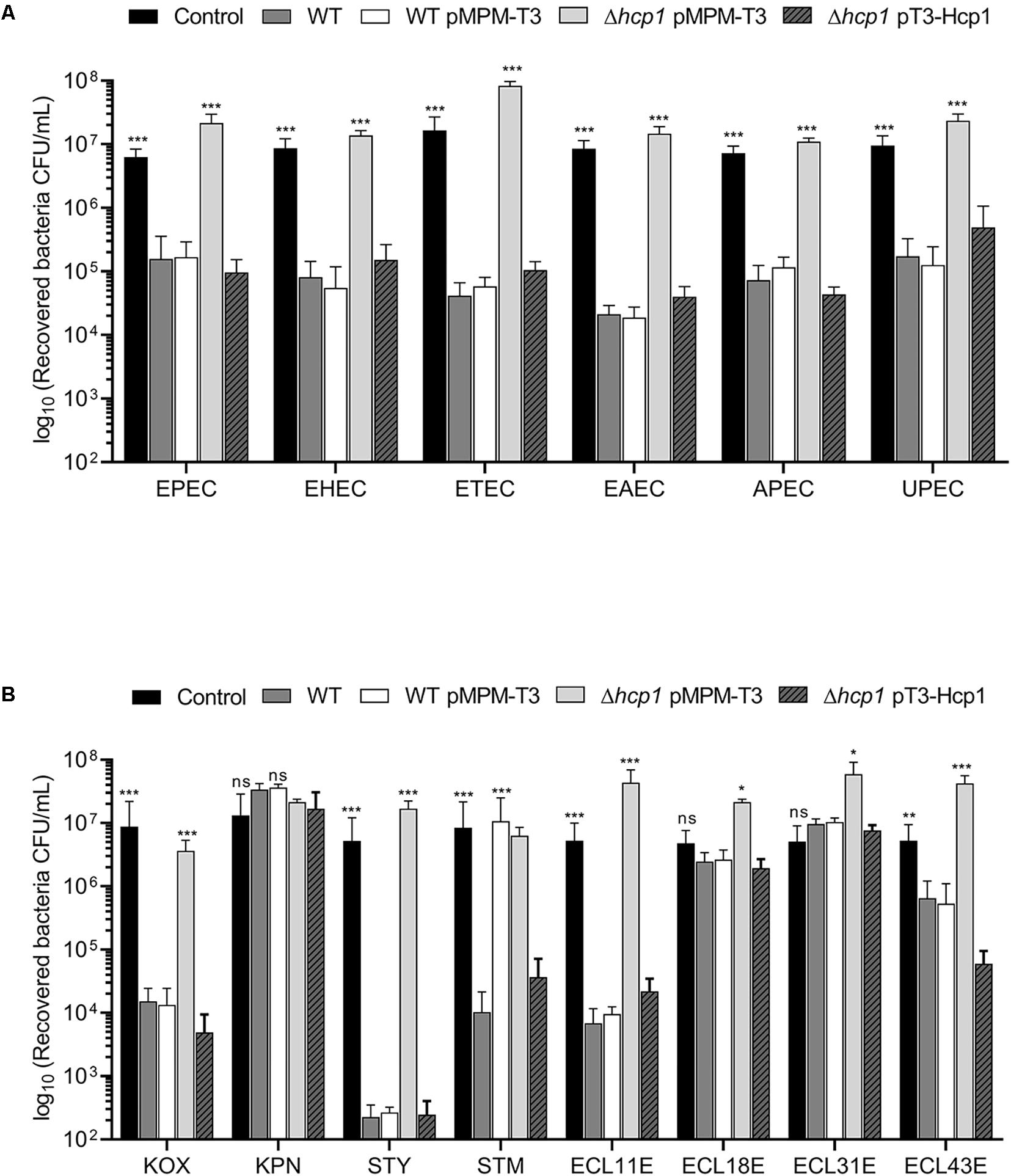
Figure 4. E. cloacae bacterial competition against different enteric bacteria. Comparison of the survival of the different E. coli pathotypes (A) and other Gram-negative pathogens (B) against wild-type E. cloacae (−/+pMPMT-3), Δhcp1 pMPM-T3, and Δhcp1 pT3-Hcp1. EPEC: enteropathogenic E. coli. EHEC: enterohemorragic E. coli. ETEC: enterotoxigenic E. coli. EAEC: enteroaggregative E. coli. APEC: avian-pathogenic E. coli. UPEC: uropathogenic E. coli. KOX: K. oxytoca. KPN: K. pneumoniae. STY: S. Typhi. STM: S. Typhimurium. ECL11E, ECL18E, ECL31E, and ECL43E: clinical isolates of E. cloacae. Survival levels are expressed in CFU/ml. Control: prey bacteria in LB with no E. cloacae strains. Statistically significant with respect to the wild-type strain; ns: not significant; *p < 0.05; **p < 0.01; ***p < 0.001.
In addition to E. coli, we analyzed the ability of E. cloacae to kill different Gram-negative enteropathogens such as Salmonella, Klebsiella, and other E. cloacae strains isolated from blood culture (Figure 4B). We found that wild-type E. cloacae was able to decrease the growth of S. Typhi (∼104-fold). For S. Typhimurium and K. oxytoca, E. cloacae decreased the bacterial viability by 103-fold. Interestingly, E. cloacae ATCC 13047 killed two E. cloacae clinical isolates around 101- and 103-fold. Surprisingly, K. pneumoniae and two E. cloacae clinical isolates did not show a reduction in the recovery of viable cells in presence of wild-type E. cloacae (Figure 4B). Our data show that T6SS-1 confers an advantage to E. cloacae in an inter- and intra-bacterial competition against other bacterial pathogens.
T6SS-1 Confers Hemolytic Activity to E. cloacae
Since T6SS confers hemolytic activity in some bacteria (Bleumink-Pluym et al., 2013; Zong et al., 2019), we analyzed this phenotype incubating red blood cells with wild-type E. cloacae, ΔclpV1 pMPM-T3, ΔclpV1 pT3-ClpV1, ΔclpV2 Δhcp1 pMPM-T3, and Δhcp1 pT3-Hcp1 mutants. The lack of a functional T6SS-1 impaired the hemolytic activity of E. cloacae (Figure 5). Likewise, the absence of Hcp1 protein diminished to 43% the bacterial hemolytic activity compared with the wild-type E. cloacae (Figure 5). The complemented ΔclpV1 and Δhcp mutants restored the hemolysis levels. These results demonstrate that Hcp1 protein secretion, which is T6SS-1 dependent, confers hemolytic activity to E. cloacae.
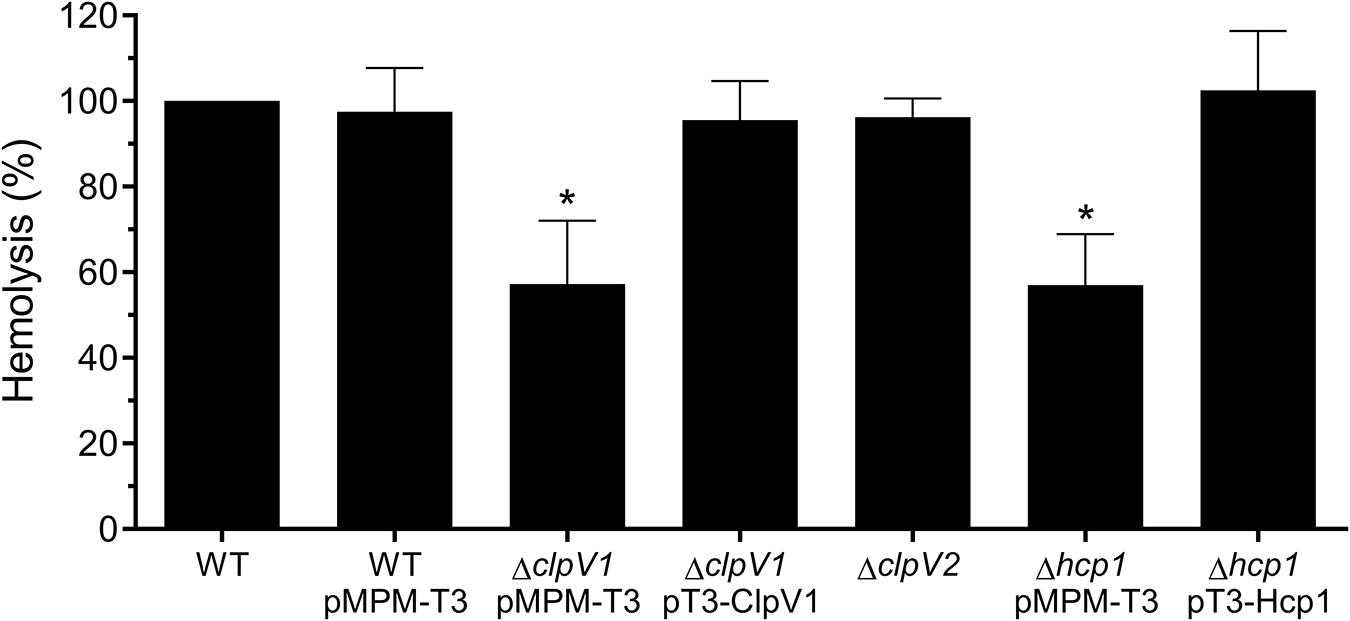
Figure 5. Hemolysis assay describing T6SS-1-mediated hemolytic activity of E. cloacae. Hemolysis of wild-type E. cloacae (−/+pMPM-T3), ΔclpV1 pMPM-T3, ΔclpV1 pT3-ClpV1, ΔclpV2, Δhcp1 pMPM-T3, and Δhcp1 pT3-Hcp backgrounds was quantified by measuring absorbance at 450 nm and expressed in percentage with respect to the lysis obtained with wild-type E. cloacae strain. Statistically significant with respect to the wild-type strain; ns: not significant; *p < 0.05.
T6SS-2 Is Implicated in Biofilm Formation and Adherence to Epithelial Cells
A virulence hallmark of Proteobacteria is their ability to interact to both abiotic and biotic surfaces, resulting mainly in the phenotypes of biofilm formation and adherence to epithelial cells, respectively, which are crucial for pathogenicity. In the case of E. cloacae, it was suggested that adherence to epithelial cells and biofilm formation are traits associated with pathogenicity (Krzyminska et al., 2010; Mezzatesta et al., 2012). To explore the roles of both E. cloacae T6SSs in these phenotypes, we evaluated both cell adherence and biofilm formation of the wild-type E. cloacae and T6SS-ATPases mutant strains (ΔclpV1 and ΔclpV2). We observed that only ΔclpV2 mutant strain exhibited a significant decrease in the biofilm formation compared with wild-type strain (Figure 6A). The ΔclpV2 complemented mutant, which expresses clpV2 gene from a lac promoter, was able to restore biofilm formation to levels similar to the wild-type strain (Figure 4A). Furthermore, the adherence of ΔclpV2 single mutant to HeLa cells was also reduced (11.3-fold) compared to wild-type strain (Figure 6B). While a ΔclpV1 ΔclpV2 double mutant showed the same phenotype that a ΔclpV2 single mutant, the ΔclpV1 mutant was similar to the wild-type strain indicating that T6SS-1 was neither required for adherence to HeLa cells nor for biofilm formation (Figure 6). We evaluated the probable role of T6SS on macrophage-mediated phagocytosis. Neither T6SS-1 nor T6SS-2 participated in resistance to phagocytosis (Supplementary Figure S2). The data compellingly show that the E. cloacae T6SS-2 contributes to adherence to both abiotic and biotic surfaces.
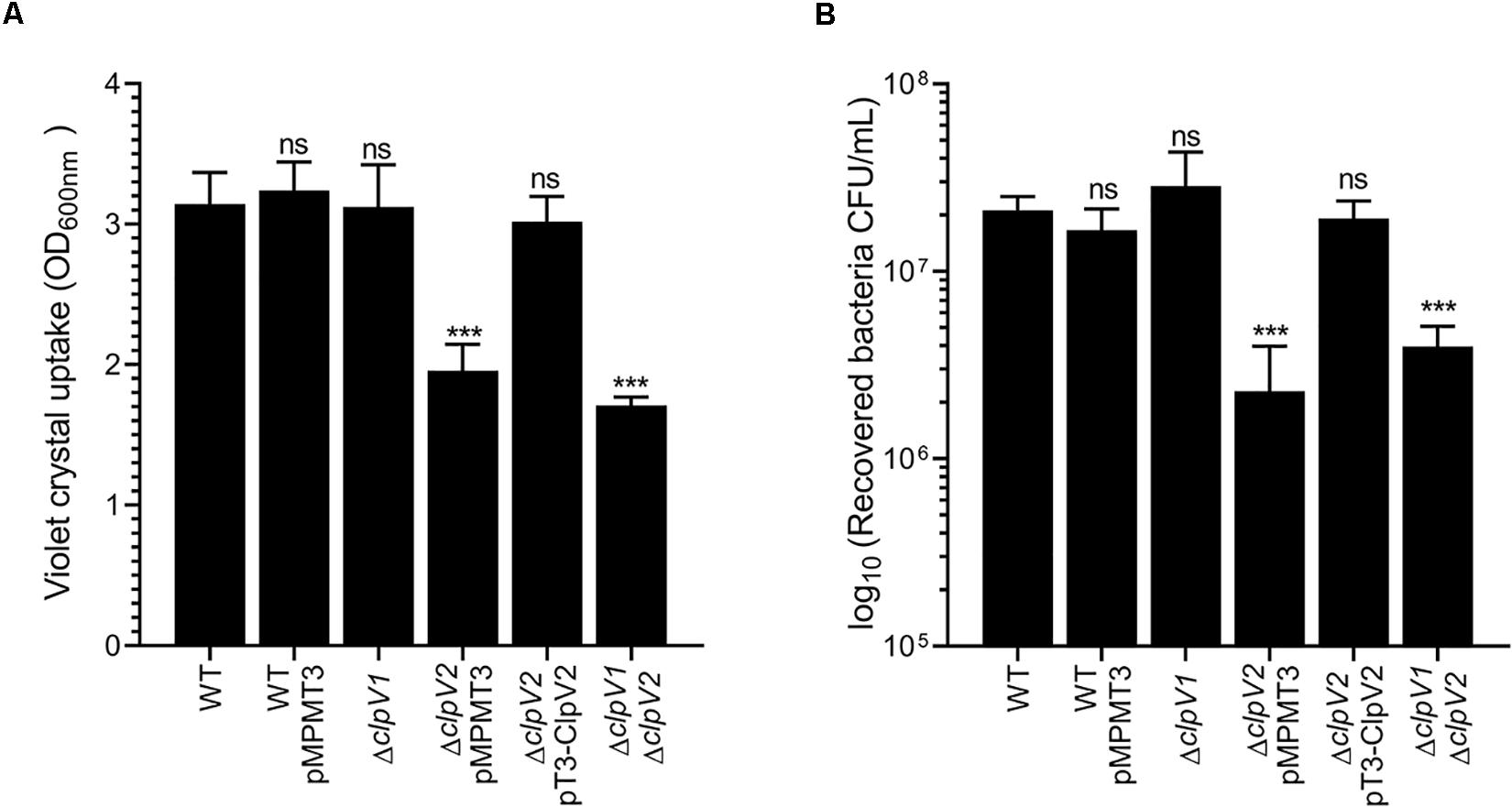
Figure 6. Role of E. cloacae T6SSs on the biofilm formation and cell adherence. (A) Quantification of biofilm formation by measuring crystal violet uptake. Wild-type E. cloacae and T6SS mutants were grown for 24 h in DMEM. (B) Adherence of wild-type E. cloacae ΔclpV1, ΔclpV2, ΔclpV2 pT3-ClpV2, and ΔclpV1 ΔclpV2 backgrounds, after 2 h of infection of HeLa cell monolayers. Statistically significant differences between wild-type E. cloacae and their respective T6SS isogenic mutants; ns: not significant; ***p < 0.001.
Both T6SSs of E. cloacae Contribute for the Bacterial Colonization in vivo
Given that the T6SS is associated with bacterial pathogenesis, we investigated the in vivo contribution of both E. cloacae T6SSs in colonization of the mouse gut. For this purpose, BALB/c mice were infected with wild-type E. cloacae strain and the ΔclpV1, ΔclpV2 and ΔclpV1 ΔclpV2 mutants and bacteria were recovered 3 and 6 days post-infection (Figure 7). After 3 days post-infection, the ΔclpV1 and ΔclpV2 strains showed a decrease in colonization by 74.62- and 9.68-fold, respectively, compared with the wild-type strain. The Δhcp1 mutant resulted in a similar phenotype than the ΔclpV1 single mutant. However, very low CFU numbers were recovered in the ΔclpV1 ΔclpV2 double mutant compared with ΔclpV1, ΔclpV2, and Δhcp1 single mutants (Figure 7).
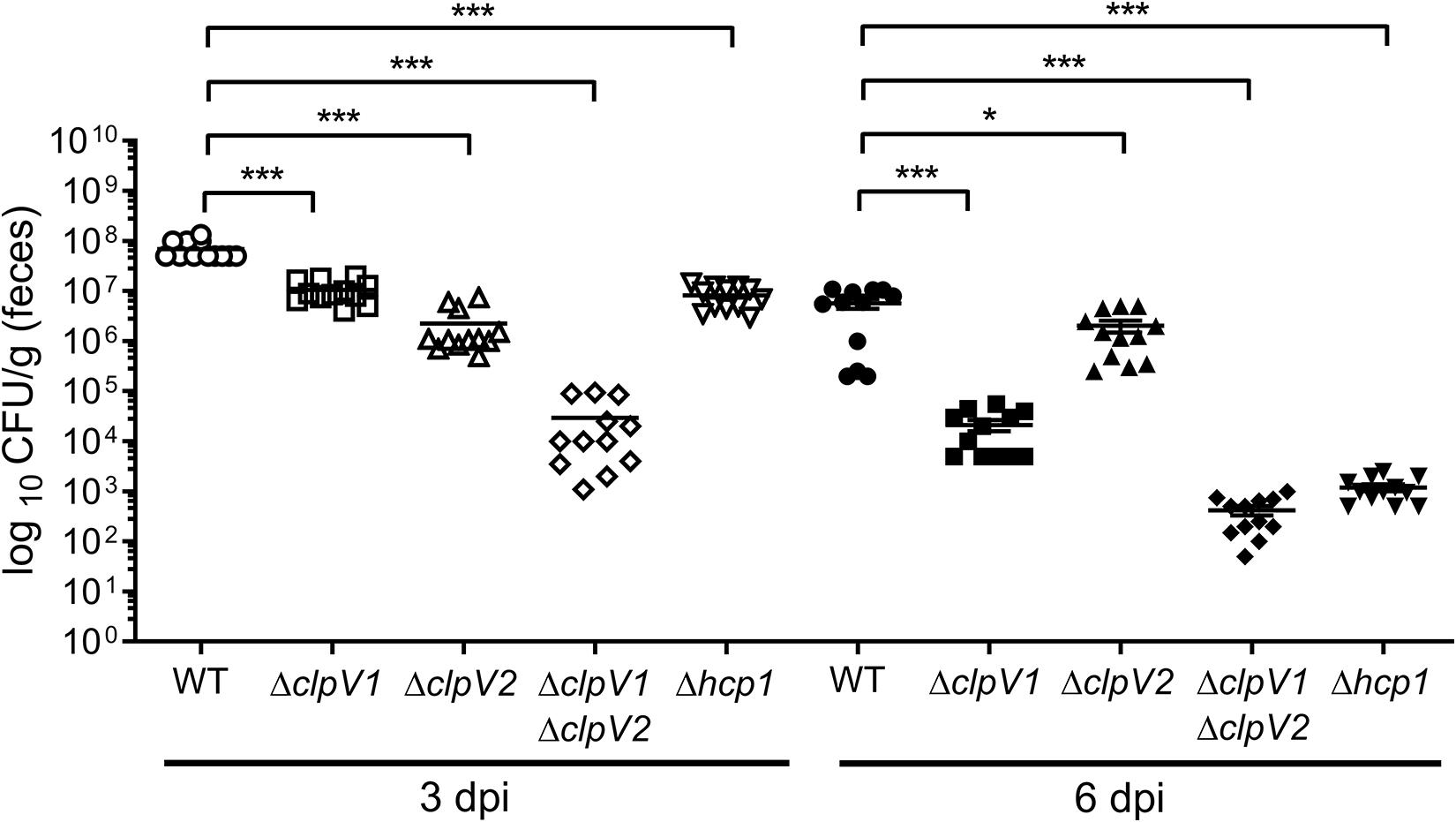
Figure 7. Both T6SSs of E. cloacae are required for the gut colonization. BALB/c mice were infected by intragastric inoculation with 109 CFU/ml with the WT strain and their respective isogenic mutants in the ATPases of both T6SSs. Bacterial colonization was assessed after 3 and 6 days post-infection. Statistically significant differences between wild-type E. cloacae and their respective T6SS isogenic mutants; *p < 0.05; ***p < 0.001.
At day 6 post-infection, the absence of ClpV1 and Hcp1 also reduced colonization levels (272.38-fold) of E. clocace (Figure 7). The CFU numbers of ΔclpV2 single mutant had a slight decrease (2.82-fold) compared with the wild-type strain. Interestingly, the ΔclpV1 ΔclpV2 double mutant was strongly affected in the colonization of mice gut compared with ΔclpV1 single mutant (Figure 7). These data strongly indicate that both T6SSs are required for intestinal colonization.
Discussion
The type VI secretion system was initially described in Vibrio cholerae (Pukatzki et al., 2006); however, these genes have been found in more than 25% of all sequenced Gram-negative bacteria (Boyer et al., 2009). Recently, it was reported that E. cloacae strains possess gene sequences that code for at least one T6SS (Liu et al., 2013). This work represents the first investigation of the T6SS function in the Enterobacter genus and it describes the different roles of two T6SS in the pathogenicity of E. cloacae ATCC 13047. Several studies have demonstrated that environmental conditions are important for the differential expression of genes involved in the bacterial virulence (Dong and Mekalanos, 2012; Blair et al., 2013; De La Cruz et al., 2017; Ares et al., 2019). We showed that TSB and DMEM growth media stimulated the expression of E. cloacae T6SS-1 and T6SS-2, respectively, indicating that gene expression in both T6SSs is regulated by specific environmental cues, most likely compounds present in the culture medium. Interestingly, the expression of ECL_RS07670 was slightly increased in DMEM. ECL_RS07670-ECL_RS7700 genes form a putative polycistronic operon, and ECL_RS07695 codes for an Rhs toxin, which could be involved in bacteria–bacteria and bacteria–eukaryotic cell interactions, as it was reported for Dickeya dadantii and S. Typhimurium, respectively (Koskiniemi et al., 2013; Starsta et al., 2020). Hence, DMEM components might mimic conditions that occur during the bacteria–cell host interaction and it would stimulate the transcription of ECL_RS07670-ECL_RS7700 operon in such conditions.
T6SS core structural constituents play an important role in bacteria–bacteria interactions (Wexler et al., 2016). One crucial component of the T6SS is the Hcp protein, which constitutes the ∼600-nm-long inner tube wrapped into a sheath-like structure and is essential for the translocation of effector proteins (Basler et al., 2012; Brunet et al., 2014; Zoued et al., 2014). When the tail sheath is contracted, the components are recycled by the ClpV ATPase for a new cycle of T6SS tail elongation (Douzi et al., 2016). The absence of Hcp1 or ClpV1 decreased the ability of E. cloacae to outcompete against pathogenic and non-pathogenic E. coli and other Gram-negative bacteria as well (including other E. cloacae clinical isolates), supporting the notion that T6SS-1 functions as an antibacterial weapon that could be used by E. cloacae to compete against other bacteria. This inter- and intra-bacterial competition that is T6SS dependent has been described for Acinetobacter baumannii, another Gram-negative opportunistic pathogen (Repizo et al., 2015). Interestingly, E. cloacae was not able to kill K. pneumoniae or the two E. cloacae clinical isolates. Ongoing work by our group shows that the capsule polysaccharide protects K. pneumoniae against the T6SS of other bacteria, acting as a shield (Soria-Bustos et al., in preparation; Bleumink-Pluym et al., 2013; Toska et al., 2018). One explanation for the resistance of the two E. cloacae clinical isolates is that these bacteria possess homologous antitoxin proteins encoded in the T6SS clusters, which neutralize the poison proteins of E. cloacae ATCC 13047.
An interesting structural feature of the T6SS-2 is that it lacks the canonical Hcp protein. Besides, Hcp1 protein was not required for the T6SS-2 activity because the cell adherence and biofilm formation in Δhcp1 were similar to wild-type strain (Supplementary Figure S3). However, four Hcp1 homologous proteins were found in the genome of E. cloacae ATCC 13047: Hcp2 (ECL_RS00165), Hcp3 (ECL_RS07685), Hcp4 (ECL_RS10590), and Hcp5 (ECL_RS19875), which showed 26 and 45% amino acid identity and similarity, respectively. The role of those Hcp1 homologs in the function of E. cloacae T6SSs remains unknown. It is reasonable to speculate that any of these proteins could be associated with the T6SS-2 needle structure. Therefore, the mutations of such hcp1 homologous genes and the resulting phenotypic effects are currently researched in our group.
Our results show that the E. cloacae T6SS-2 plays an essential role in biofilm formation and adherence to eukaryotic cells. These observations are in agreement to the function of T6SS of other pathogens on the bacterial adherence on abiotic and biotic surfaces (de Pace et al., 2011; Lertpiriyapong et al., 2012; Sheng et al., 2013; Tang et al., 2015; Gallique et al., 2017). Enteroaggregative E. coli strain 17-2 codes for two functional T6SS named Sci-1 and Sci-2, which are both involved in bacterial competition (Brunet et al., 2013; Flaugnatti et al., 2016), but Sci-1 also confers the ability to form biofilms (Aschtgen et al., 2008). Recently, it was reported that the absence of two functional T6SSs in K. pneumoniae affected the expression of type-1 fimbriae and subsequently the adherence to epithelial cells was impaired (Hsieh et al., 2019). Ongoing experiments in our laboratory will analyze if T6SS-2 controls the expression of type-1 fimbriae or any other adherence factor in E. cloacae. In terms of macrophage interaction, T6SS confers phagocytosis resistance in some bacteria (Suarez et al., 2008; Wan et al., 2017). Our data showed that neither T6SS-1 nor T6SS-2 are involved in this phenotype.
The T6SS has been shown to be relevant for virulence in vivo in many pathogenic bacteria (Lertpiriyapong et al., 2012; Repizo et al., 2015; Sana et al., 2016; Hsieh et al., 2019; Ungureanu et al., 2019). Here, we demonstrated the importance of both E. cloacae T6SSs in intestinal colonization of BALB/c mice. Both ΔclpV1 and ΔclpV2 mutants showed reduced levels of colonization as demonstrated by a reduced number of colony-forming units recovered in feces as compared with the wild-type strain. However, the absence of a functional T6SS-1 (e.g., in the Δhcp1 or ΔclpV1 mutants) had a higher effect in colonization than the absence of the T6SS-2. Interestingly, both E. cloacae T6SSs showed an additive effect in in vivo intestinal colonization, suggesting that both secretion systems are important in the adherence of E. cloacae to epithelial cells and, most importantly, in the bacterial competition of this microorganism against other bacteria found in the gut microbiota.
Phylogenetically, E. cloacae T6SS-1 is related to the S. Typhi and S. Typhimurium T6SSs located on Salmonella Pathogenicity Island 6, which are required for gut colonization and systemic infection in humanized and non-humanized mice, respectively (Libby et al., 2010; Pezoa et al., 2013; Sana et al., 2016). In contrast, T6SS-2 genes such as clpV, vgrG, PAAR, and tssM, were phylogenetically associated with bacteria found in plants and soil (Liu et al., 2013). The outcome of this study supports a functional role of T6SS-1 and T6SS-2 in intestinal colonization and interactions with abiotic/biotic surfaces, respectively, which is likely to increase the ecological fitness of this microorganism.
In summary, our results demonstrate that the T6SS mutants were hampered in host cell adhesion and biofilm formation, antibacterial activity against multiple pathogens, and importantly, in intestinal colonization in mice. This study uncovers for the first time the presence and function of two T6SSs in E. cloacae, a trait that increases adaptability of this organism to different niches and hosts as part of their pathogenesis scheme.
Data Availability Statement
All datasets presented in this study are included in the article/Supplementary Material.
Ethics Statement
The animal study was reviewed and approved by the Internal Ethics Committee of the Animal Resource Facility of the Universidad Autónoma del Estado de Hidalgo (Approval number: CICUAL/016/2019R to CG-A).
Author Contributions
JS-B and MD conceived and designed the experiments. JS-B and MA performed the experiments. JS-B, MA, CG-A, JG-M, JG, and MD analyzed the data. JS-B, JG, and MD wrote the manuscript. All authors contributed to the article and approved the submitted version.
Conflict of Interest
The authors declare that the research was conducted in the absence of any commercial or financial relationships that could be construed as a potential conflict of interest.
Acknowledgments
JS-B was supported by pre-doctoral fellowships from CONACYT (276773) and IMSS (99097170). We thank Fernando Chimal-Cázares for the help with mice experiments.
Supplementary Material
The Supplementary Material for this article can be found online at: https://www.frontiersin.org/articles/10.3389/fmicb.2020.560488/full#supplementary-material
FIGURE S1 | Expression of reference gene (rrsH) under different bacteriological broths. Quantification of expression is showed as copies of rrsH/μg RNA.
FIGURE S2 | Phagocytosis resistance of wild-type E. cloacae, ΔclpV1, ΔclpV2, and Δhcp1 mutants. Comparison of phagocytosis levels between the different strains using THP-1 human monocyte-derived macrophages. Statistically significant differences between wild-type E. cloacae and their respective T6SS isogenic mutants; ∗∗∗p < 0.001.
FIGURE S3 | Hcp1 does not affect the biofilm formation and cell adherence. Comparison of biofilm formation and cell adherence levels between the Δhcp1 mutant and the wild-type strain.
Footnotes
- ^ http://www.bioinformatics.nl/cgi-bin/primer3plus/primer3plus.cgi/
- ^ https://www.ncbi.nlm.nih.gov/refseq/about/non-redundantproteins/
- ^ http://prospector.ucsf.edu/prospector
References
Ares, M. A., Abundes-Gallegos, J., Rodriguez-Valverde, D., Panunzi, L. G., Jimenez-Galicia, C., Jarillo-Quijada, M. D., et al. (2019). The Coli Surface Antigen CS3 of Enterotoxigenic Escherichia coli is differentially regulated by H-NS, CRP, and CpxRA Global Regulators. Front. Microbiol. 10:1685. doi: 10.3389/fmicb.2019.01685
Ares, M. A., Fernandez-Vazquez, J. L., Rosales-Reyes, R., Jarillo-Quijada, M. D., Von Bargen, K., Torres, J., et al. (2016). H-NS Nucleoid Protein controls virulence features of Klebsiella pneumoniae by regulating the expression of type 3 Pili and the capsule polysaccharide. Front. Cell. Infect. Microbiol. 6:13. doi: 10.3389/fcimb.2016.00013
Aschtgen, M. S., Bernard, C. S., De Bentzmann, S., Lloubes, R., and Cascales, E. (2008). SciN is an outer membrane lipoprotein required for type VI secretion in enteroaggregative Escherichia coli. J. Bacteriol. 190, 7523–7531. doi: 10.1128/jb.00945-08
Barnes, A. I., Ortiz, C., Paraje, M. G., Balanzino, L. E., and Albesa, I. (1997). Purification and characterization of a cytotoxin from Enterobacter cloacae. Can. J. Microbiol. 43, 729–733. doi: 10.1139/m97-105
Basler, M., Pilhofer, M., Henderson, G. P., Jensen, G. J., and Mekalanos, J. J. (2012). Type VI secretion requires a dynamic contractile phage tail-like structure. Nature 483, 182–186. doi: 10.1038/nature10846
Bingle, L. E., Bailey, C. M., and Pallen, M. J. (2008). Type VI secretion: a beginner’s guide. Curr. Opin. Microbiol. 11, 3–8.
Blair, J. M., Richmond, G. E., Bailey, A. M., Ivens, A., and Piddock, L. J. (2013). Choice of bacterial growth medium alters the transcriptome and phenotype of Salmonella enterica Serovar Typhimurium. PLoS One 8:e63912. doi: 10.1371/journal.pone.0063912
Bleumink-Pluym, N. M., Van Alphen, L. B., Bouwman, L. I., Wosten, M. M., and Van Putten, J. P. (2013). Identification of a functional type VI secretion system in Campylobacter jejuni conferring capsule polysaccharide sensitive cytotoxicity. PLoS Pathog. 9:e1003393. doi: 10.1371/journal.pone.0063912
Boyer, F., Fichant, G., Berthod, J., Vandenbrouck, Y., and Attree, I. (2009). Dissecting the bacterial type VI secretion system by a genome wide in silico analysis: What can be learned from available microbial genomic resources? BMC Genomics 10:104. doi: 10.1186/1471-2164-10-104
Brunet, Y. R., Espinosa, L., Harchouni, S., Mignot, T., and Cascales, E. (2013). Imaging type VI secretion-mediated bacterial killing. Cell Rep. 3, 36–41. doi: 10.1016/j.celrep.2012.11.027
Brunet, Y. R., Henin, J., Celia, H., and Cascales, E. (2014). Type VI secretion and bacteriophage tail tubes share a common assembly pathway. EMBO Rep. 15, 315–321. doi: 10.1002/embr.201337936
Chen, C., Yang, X., and Shen, X. (2019). Confirmed and potential roles of bacterial T6SSs in the intestinal ecosystem. Front. Microbiol. 10:1484. doi: 10.3389/fmicb.2019.01484
Cherrak, Y., Flaugnatti, N., Durand, E., Journet, L., and Cascales, E. (2019). Structure and Activity of the Type VI Secretion System. Microbiol. Spectr. 7:PSIB-0031-2019.
Datsenko, K. A., and Wanner, B. L. (2000). One-step inactivation of chromosomal genes in Escherichia coli K-12 using PCR products. Proc. Natl. Acad. Sci. U.S.A. 97, 6640–6645. doi: 10.1073/pnas.120163297
De La Cruz, M. A., Ruiz-Tagle, A., Ares, M. A., Pacheco, S., Yanez, J. A., Cedillo, L., et al. (2017). The expression of Longus type 4 pilus of enterotoxigenic Escherichia coli is regulated by LngR and LngS and by H-NS, CpxR and CRP global regulators. Environ. Microbiol. 19, 1761–1775. doi: 10.1111/1462-2920.13644
de Pace, F., Boldrin De Paiva, J., Nakazato, G., Lancellotti, M., Sircili, M. P., Guedes Stehling, E., et al. (2011). Characterization of IcmF of the type VI secretion system in an avian pathogenic Escherichia coli (APEC) strain. Microbiology 157, 2954–2962. doi: 10.1099/mic.0.050005-0
Dho, M., and Lafont, J. P. (1982). Escherichia coli colonization of the trachea in poultry: comparison of virulent and avirulent strains in gnotoxenic chickens. Avian Dis. 26, 787–797. doi: 10.2307/1589865
Dong, T. G., and Mekalanos, J. J. (2012). Characterization of the RpoN regulon reveals differential regulation of T6SS and new flagellar operons in Vibrio cholerae O37 strain V52. Nucleic Acids Res. 40, 7766–7775. doi: 10.1093/nar/gks567
Douzi, B., Brunet, Y. R., Spinelli, S., Lensi, V., Legrand, P., Blangy, S., et al. (2016). Structure and specificity of the Type VI secretion system ClpV-TssC interaction in enteroaggregative Escherichia coli. Sci. Rep. 6:34405.
Flaugnatti, N., Le, T. T., Canaan, S., Aschtgen, M. S., Nguyen, V. S., Blangy, S., et al. (2016). A phospholipase A1 antibacterial Type VI secretion effector interacts directly with the C-terminal domain of the VgrG spike protein for delivery. Mol. Microbiol. 99, 1099–1118. doi: 10.1111/mmi.13292
Gallique, M., Decoin, V., Barbey, C., Rosay, T., Feuilloley, M. G., Orange, N., et al. (2017). Contribution of the Pseudomonas fluorescens MFE01 Type VI Secretion System to Biofilm Formation. PLoS One 12:e0170770. doi: 10.1371/journal.pone.0170770
Garcia-Morales, L., Leon-Solis, L., Monroy-Munoz, I. E., Talavera-Paulin, M., Serafin-Lopez, J., Estrada-Garcia, I., et al. (2017). Comparative proteomic profiles reveal characteristic Mycobacterium tuberculosis proteins induced by cholesterol during dormancy conditions. Microbiology 163, 1237–1247. doi: 10.1099/mic.0.000512
Ho, B. T., Dong, T. G., and Mekalanos, J. J. (2014). A view to a kill: the bacterial type VI secretion system. Cell Host Microbe 15, 9–21. doi: 10.1016/j.chom.2013.11.008
Hood, R. D., Singh, P., Hsu, F., Guvener, T., Carl, M. A., Trinidad, R. R., et al. (2010). A type VI secretion system of Pseudomonas aeruginosa targets a toxin to bacteria. Cell Host Microbe 7, 25–37. doi: 10.1016/j.chom.2009.12.007
Hsieh, P. F., Lu, Y. R., Lin, T. L., Lai, L. Y., and Wang, J. T. (2019). Klebsiella pneumoniae type VI secretion system contributes to bacterial competition, cell invasion, type-1 fimbriae expression, and in vivo colonization. J. Infect. Dis. 219, 637–647. doi: 10.1093/infdis/jiy534
Jahn, C. E., Charkowski, A. O., and Willis, D. K. (2008). Evaluation of isolation methods and RNA integrity for bacterial RNA quantitation. J. Microbiol. Methods 75, 318–324. doi: 10.1016/j.mimet.2008.07.004
Journet, L., and Cascales, E. (2016). The Type VI Secretion System in Escherichia coli and Related Species. EcoSal Plus 7, 1–20.
Koskiniemi, S., Lamoureux, J. G., Nikolakakis, K. C., T’kint De Roodenbeke, C., Kaplan, M. D., Low, D. A., et al. (2013). Rhs proteins from diverse bacteria mediate intercellular competition. Proc. Natl. Acad. Sci. U.S.A. 110, 7032–7037. doi: 10.1073/pnas.1300627110
Krzyminska, S., Koczura, R., Mokracka, J., Puton, T., and Kaznowski, A. (2010). Isolates of the Enterobacter cloacae complex induce apoptosis of human intestinal epithelial cells. Microb. Pathog. 49, 83–89. doi: 10.1016/j.micpath.2010.04.003
Krzyminska, S., Mokracka, J., Koczura, R., and Kaznowski, A. (2009). Cytotoxic activity of Enterobacter cloacae human isolates. FEMS Immunol. Med. Microbiol. 56, 248–252.
Kube, S., Kapitein, N., Zimniak, T., Herzog, F., Mogk, A., and Wendler, P. (2014). Structure of the VipA/B type VI secretion complex suggests a contraction-state-specific recycling mechanism. Cell Rep. 8, 20–30. doi: 10.1016/j.celrep.2014.05.034
Lertpiriyapong, K., Gamazon, E. R., Feng, Y., Park, D. S., Pang, J., Botka, G., et al. (2012). Campylobacter jejuni type VI secretion system: roles in adaptation to deoxycholic acid, host cell adherence, invasion, and in vivo colonization. PLoS One 7:e42842. doi: 10.1371/journal.pone.0042842
Levine, M. M., Bergquist, E. J., Nalin, D. R., Waterman, D. H., Hornick, R. B., Young, C. R., et al. (1978). Escherichia coli strains that cause diarrhoea but do not produce heat-labile or heat-stable enterotoxins and are non-invasive. Lancet 1, 1119–1122. doi: 10.1016/s0140-6736(78)90299-4
Levine, M. M., Ristaino, P., Marley, G., Smyth, C., Knutton, S., Boedeker, E., et al. (1984). Coli surface antigens 1 and 3 of colonization factor antigen II-positive enterotoxigenic Escherichia coli: morphology, purification, and immune responses in humans. Infect. Immun. 44, 409–420. doi: 10.1128/iai.44.2.409-420.1984
Libby, S. J., Brehm, M. A., Greiner, D. L., Shultz, L. D., Mcclelland, M., Smith, K. D., et al. (2010). Humanized nonobese diabetic-scid IL2rgammanull mice are susceptible to lethal Salmonella Typhi infection. Proc. Natl. Acad. Sci. U.S.A. 107, 15589–15594. doi: 10.1073/pnas.1005566107
Liu, W. Y., Wong, C. F., Chung, K. M., Jiang, J. W., and Leung, F. C. (2013). Comparative genome analysis of Enterobacter cloacae. PLoS One 8:e74487. doi: 10.1371/journal.pone.0074487
Livak, K. J., and Schmittgen, T. D. (2001). Analysis of relative gene expression data using real-time quantitative PCR and the 2(-Delta Delta C(T)) Method. Methods 25, 402–408. doi: 10.1006/meth.2001.1262
Ma, A. T., Mcauley, S., Pukatzki, S., and Mekalanos, J. J. (2009). Translocation of a Vibrio cholerae type VI secretion effector requires bacterial endocytosis by host cells. Cell Host Microbe 5, 234–243. doi: 10.1016/j.chom.2009.02.005
MacIntyre, D. L., Miyata, S. T., Kitaoka, M., and Pukatzki, S. (2010). The Vibrio cholerae type VI secretion system displays antimicrobial properties. Proc. Natl. Acad. Sci. U.S.A. 107, 19520–19524. doi: 10.1073/pnas.1012931107
Mayer, M. P. (1995). A new set of useful cloning and expression vectors derived from pBlueScript. Gene 163, 41–46. doi: 10.1016/0378-1119(95)00389-n
McAdam, A. J. (2020). Enterobacteriaceae? Enterobacterales? What Should We Call Enteric Gram-Negative Bacilli? A Micro-Comic Strip. J. Clin. Microbiol. 58:e01888-19.
Mezzatesta, M. L., Gona, F., and Stefani, S. (2012). Enterobacter cloacae complex: clinical impact and emerging antibiotic resistance. Future Microbiol. 7, 887–902. doi: 10.2217/fmb.12.61
Mobley, H. L., Green, D. M., Trifillis, A. L., Johnson, D. E., Chippendale, G. R., Lockatell, C. V., et al. (1990). Pyelonephritogenic Escherichia coli and killing of cultured human renal proximal tubular epithelial cells: role of hemolysin in some strains. Infect. Immun. 58, 1281–1289. doi: 10.1128/iai.58.5.1281-1289.1990
Mougous, J. D., Cuff, M. E., Raunser, S., Shen, A., Zhou, M., Gifford, C. A., et al. (2006). A virulence locus of Pseudomonas aeruginosa encodes a protein secretion apparatus. Science 312, 1526–1530. doi: 10.1126/science.1128393
Murdoch, S. L., Trunk, K., English, G., Fritsch, M. J., Pourkarimi, E., and Coulthurst, S. J. (2011). The opportunistic pathogen Serratia marcescens utilizes type VI secretion to target bacterial competitors. J. Bacteriol. 193, 6057–6069. doi: 10.1128/jb.05671-11
Nataro, J. P., Baldini, M. M., Kaper, J. B., Black, R. E., Bravo, N., and Levine, M. M. (1985). Detection of an adherence factor of enteropathogenic Escherichia coli with a DNA probe. J. Infect. Dis. 152, 560–565. doi: 10.1093/infdis/152.3.560
Navarro-Garcia, F., Ruiz-Perez, F., Cataldi, A., and Larzabal, M. (2019). Type VI secretion system in pathogenic Escherichia coli: structure, role in virulence, and acquisition. Front. Microbiol. 10:1965. doi: 10.3389/fmicb.2019.01965
Paauw, A., Caspers, M. P., Schuren, F. H., Leverstein-Van Hall, M. A., Deletoile, A., Montijn, R. C., et al. (2008). Genomic diversity within the Enterobacter cloacae complex. PLoS One 3:e3018. doi: 10.1371/journal.pone.0003018
Pezoa, D., Yang, H. J., Blondel, C. J., Santiviago, C. A., Andrews-Polymenis, H. L., and Contreras, I. (2013). The type VI secretion system encoded in SPI-6 plays a role in gastrointestinal colonization and systemic spread of Salmonella enterica serovar Typhimurium in the chicken. PLoS One 8:e63917. doi: 10.1371/journal.pone.0063917
Pukatzki, S., Ma, A. T., Sturtevant, D., Krastins, B., Sarracino, D., Nelson, W. C., et al. (2006). Identification of a conserved bacterial protein secretion system in Vibrio cholerae using the Dictyostelium host model system. Proc. Natl. Acad. Sci. U.S.A. 103, 1528–1533. doi: 10.1073/pnas.0510322103
Repizo, G. D., Gagne, S., Foucault-Grunenwald, M. L., Borges, V., Charpentier, X., Limansky, A. S., et al. (2015). Differential role of the T6SS in Acinetobacter baumannii virulence. PLoS One 10:e0138265. doi: 10.1371/journal.pone.0138265
Riley, L. W., Remis, R. S., Helgerson, S. D., Mcgee, H. B., Wells, J. G., Davis, B. R., et al. (1983). Hemorrhagic colitis associated with a rare Escherichia coli serotype. N. Engl. J. Med. 308, 681–685.
Russell, A. B., Peterson, S. B., and Mougous, J. D. (2014). Type VI secretion system effectors: poisons with a purpose. Nat. Rev. Microbiol. 12, 137–148. doi: 10.1038/nrmicro3185
Sana, T. G., Flaugnatti, N., Lugo, K. A., Lam, L. H., Jacobson, A., Baylot, V., et al. (2016). Salmonella Typhimurium utilizes a T6SS-mediated antibacterial weapon to establish in the host gut. Proc. Natl. Acad. Sci. U.S.A. 113, E5044–E5051.
Sheng, L., Lv, Y., Liu, Q., Wang, Q., and Zhang, Y. (2013). Connecting type VI secretion, quorum sensing, and c-di-GMP production in fish pathogen Vibrio alginolyticus through phosphatase PppA. Vet. Microbiol. 162, 652–662. doi: 10.1016/j.vetmic.2012.09.009
Shneider, M. M., Buth, S. A., Ho, B. T., Basler, M., Mekalanos, J. J., and Leiman, P. G. (2013). PAAR-repeat proteins sharpen and diversify the type VI secretion system spike. Nature 500, 350–353. doi: 10.1038/nature12453
Soto, E., Espinosa, N., Diaz-Guerrero, M., Gaytan, M. O., Puente, J. L., and Gonzalez-Pedrajo, B. (2017). Functional Characterization of EscK (Orf4), a Sorting Platform Component of the Enteropathogenic Escherichia coli Injectisome. J. Bacteriol. 199:e00538-16.
Starsta, M., Hammarlof, D. L., Waneskog, M., Schlegel, S., Xu, F., Heden Gynna, A., et al. (2020). RHS-elements function as type II toxin-antitoxin modules that regulate intra-macrophage replication of Salmonella Typhimurium. PLoS Genet. 16:e1008607. doi: 10.1371/journal.pgen.1008607
Suarez, G., Sierra, J. C., Sha, J., Wang, S., Erova, T. E., Fadl, A. A., et al. (2008). Molecular characterization of a functional type VI secretion system from a clinical isolate of Aeromonas hydrophila. Microb. Pathog. 44, 344–361. doi: 10.1016/j.micpath.2007.10.005
Tang, L., Liang, X., Moore, R., and Dong, T. G. (2015). Commentary: the icmF3 Locus is Involved in Multiple Adaptation- and Virulence-related Characteristics in Pseudomonas aeruginosa PAO1. Front. Cell. Infect. Microbiol. 5:83. doi: 10.3389/fcimb.2015.00083
Toska, J., Ho, B. T., and Mekalanos, J. J. (2018). Exopolysaccharide protects Vibrio cholerae from exogenous attacks by the type 6 secretion system. Proc. Natl. Acad. Sci. U.S.A. 115, 7997–8002.
Ungureanu, V. A., Stratakos, A. C., Gundogdu, O., Stef, L., Pet, I., Pet, E., et al. (2019). Virulence of a T6SS Campylobacter jejuni chicken isolate from North Romania. BMC Res. Notes 12:180. doi: 10.1186/s13104-019-4201-8
Wan, B., Zhang, Q., Ni, J., Li, S., Wen, D., Li, J., et al. (2017). Type VI secretion system contributes to Enterohemorrhagic Escherichia coli virulence by secreting catalase against host reactive oxygen species (ROS). PLoS Pathog. 13:e1006246. doi: 10.1371/journal.ppat.1006246
Wexler, A. G., Bao, Y., Whitney, J. C., Bobay, L. M., Xavier, J. B., Schofield, W. B., et al. (2016). Human symbionts inject and neutralize antibacterial toxins to persist in the gut. Proc. Natl. Acad. Sci. U.S.A. 113, 3639–3644. doi: 10.1073/pnas.1525637113
Weyrich, L. S., Rolin, O. Y., Muse, S. J., Park, J., Spidale, N., Kennett, M. J., et al. (2012). A Type VI secretion system encoding locus is required for Bordetella bronchiseptica immunomodulation and persistence in vivo. PLoS One 7:e45892. doi: 10.1371/journal.pone.0045892
Zhou, G., Li, L. J., Shi, Q. S., Ouyang, Y. S., Chen, Y. B., and Hu, W. F. (2014). Efficacy of metal ions and isothiazolones in inhibiting Enterobacter cloacae BF-17 biofilm formation. Can. J. Microbiol. 60, 5–14. doi: 10.1139/cjm-2013-0492
Zong, B., Zhang, Y., Wang, X., Liu, M., Zhang, T., Zhu, Y., et al. (2019). Characterization of multiple type-VI secretion system (T6SS) VgrG proteins in the pathogenicity and antibacterial activity of porcine extra-intestinal pathogenic Escherichia coli. Virulence 10, 118–132. doi: 10.1080/21505594.2019.1573491
Zoued, A., Brunet, Y. R., Durand, E., Aschtgen, M. S., Logger, L., Douzi, B., et al. (2014). Architecture and assembly of the Type VI secretion system. Biochim. Biophys. Acta 1843, 1664–1673.
Keywords: E. cloacae, T6SS, ClpV, Hcp, virulence
Citation: Soria-Bustos J, Ares MA, Gómez-Aldapa CA, González-y-Merchand JA, Girón JA and De la Cruz MA (2020) Two Type VI Secretion Systems of Enterobacter cloacae Are Required for Bacterial Competition, Cell Adherence, and Intestinal Colonization. Front. Microbiol. 11:560488. doi: 10.3389/fmicb.2020.560488
Received: 09 May 2020; Accepted: 12 August 2020;
Published: 24 September 2020.
Edited by:
Axel Cloeckaert, Institut National de la Recherche Agronomique (INRA), FranceReviewed by:
Brian Hammer, Georgia Institute of Technology, United StatesAnnabelle Merieau, Université de Rouen, France
Copyright © 2020 Soria-Bustos, Ares, Gómez-Aldapa, González-y-Merchand, Girón and De la Cruz. This is an open-access article distributed under the terms of the Creative Commons Attribution License (CC BY). The use, distribution or reproduction in other forums is permitted, provided the original author(s) and the copyright owner(s) are credited and that the original publication in this journal is cited, in accordance with accepted academic practice. No use, distribution or reproduction is permitted which does not comply with these terms.
*Correspondence: Miguel A. De la Cruz, miguel_angel_81@live.com