- 1Department of Metallurgical Engineering, Faculty of Mining and Petroleum Engineering, Institut Teknologi Bandung, Bandung, Indonesia
- 2Geomicrobiology-Biomining and Biocorrosion Laboratory, Microbial Culture Collection Laboratory, Biosciences and Biotechnology Research Center (BBRC), Institut Teknologi Bandung, Bandung, Indonesia
Biohydrometallurgy is believed to be a promising future study field for the recovery of lead (Pb) from ores/concentrates since the pyrometallurgical/hydrometallurgical processes have been largely applied to recover Pb to date, which operates at high temperature and generates volatile Pb matters that are hazardous and carcinogenic to human health. Hence, the main purpose of this study was to investigate the biohydrometallurgical extraction of Pb from the Indonesian galena concentrate through bioleaching using an iron- and sulfur-oxidizing mixotrophic bacterium (identified as Citrobacter sp.). The bioleaching experiments were conducted in shake flasks containing the modified LB broth medium supplemented with galena concentrate with a particle size of d80 = 75 μm at room temperature. Both semi-direct and direct bioleaching methods were employed in this study. The bacterium was able to extract lead (Pb) from galena concentrate with high selectivity to Cu and Zn (0.99 and 0.86, respectively). The highest extraction level of 90 g lead dissolved/kg galena concentrate was achieved using direct bioleaching method at bioleaching conditions of 2% w/v pulp density, 5 g/L FeCl3, 50 g/L NaCl, 20 g/L molasses and a rotation speed of 180 rpm at room temperature (25°C). The addition of FeCl3, NaCl, and molasses increased the lead leaching efficiencies, which were also evidenced by the FTIR, XRD, and SEM-EDS analyses. From industrial and commercial standpoints, the selective bioleaching represented in this study may be beneficial to the development of lead leaching from sulfide minerals, since insoluble anglesite (PbSO4) precipitates are formed during ferric sulfate oxidation, thus making the recovery of lead through bioleaching unpractical.
Introduction
Recently, the extraction of lead (Pb) from sulfide ores relies on pyrometallurgical smelting processes and combined pyro/hydrometallurgical methods that are the high energy consumption and cause severe environmental pollution problems due to the emission of both SO2 and volatile lead matters. Hydrometallurgical routes of leaching lead concentrates have been developed progressively by testing a number of solvents to overcome such problems (Greet and Smart, 2002; Aydoğan et al., 2007; Long et al., 2009; Wu et al., 2014). However, the chemical solvents used are reported to have a very low solubility of lead, a high corrosiveness, and very high toxicity, and a significantly high temperature (65–85°C) is required to achieve a high lead recovery as listed in Table 1 (Warren et al., 1987; Pashkov et al., 2002; Aydoğan et al., 2007; Qin et al., 2009; Zárate-Gutiérrez et al., 2010, 2015; Baba and Adekola, 2013; Wu et al., 2014; Anugrah et al., 2017; Allen and Igboayaka, 2019).
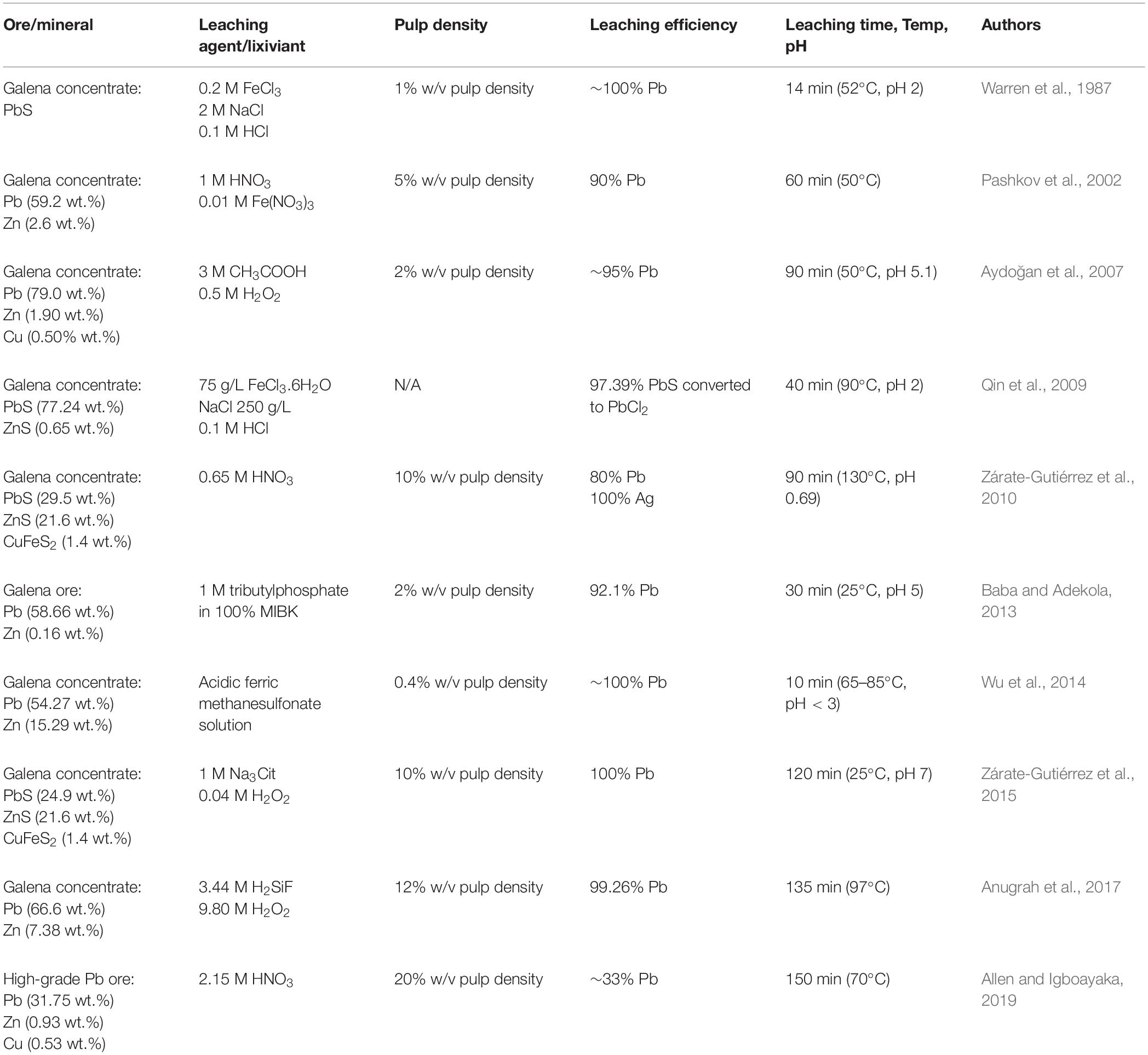
Table 1. Previous studies on leaching lead (Pb) from pure galena, galena concentrates, and high-grade Pb-Zn bearing ores.
Therefore, bioleaching of lead concentrates as a biohydrometallurgical method has emerged as a possible solution for overcoming the aforementioned problems since the bioleaching is a low-cost, environmentally friendly method. Of the bacterial genera and species, Acidithiobacillus ferrooxidans and Acidithiobacillus thiooxidans (chemolithotrophs) have been employed frequently for bioleaching sulfide ores (Garcia Jr. et al., 1995; Da Silva et al., 2003; Da Silva, 2004). However, the bioleaching of galena (PbS) has been poorly studied, since the complete oxidation of galena leads to insoluble anglesite (PbSO4) that thus precludes the recovery of lead from bioleaching and ferric sulfate leaching through conventional solvent extraction/electrowinning routes (Da Silva et al., 2003; Da Silva, 2004). Moreover, several studies demonstrated that the elevated lead bioleaching/leaching efficiencies were achieved in the presence of NaCl (Warren et al., 1987; Liao and Deng, 2004; Ye et al., 2017), FeCl3 (Dutrizac, 1986; Kim et al., 1986; Warren et al., 1987; Dutrizac and Chen, 1990; Long et al., 2009), and ferric (Fe3+) ions (Pashkov et al., 2002). Hence, the present study investigated the use of a local mixotrophic bacterium (identified as Citrobacter sp.) capable of oxidizing iron and sulfur as well as producing biosurfactants, including EPS (extracellular polymeric substances) in extracting lead from galena concentrate to enhance lead recovery. Compared to previous studies on galena (PbS) bioleaching and high-grade Pb ores bioleaching summarized in Table 2 (Bang et al., 1995; Garcia Jr. et al., 1995; Da Silva et al., 2003; Da Silva, 2004; Pacholewska, 2004; Jiang et al., 2008; Baba et al., 2011; Mejía et al., 2012; Ghassa et al., 2014), the present study is different from previous studies and more beneficial from metallurgical standpoints as follows: (1) the bacterium Citrobacter sp. employed in this study belongs to mixotrophic group capable of utilizing both organic and inorganic compounds for energy and carbon sources, thus making much easier for industrial applications since bacterial carbon sources can be derived from any organic wastes abundant in Indonesia and many other tropical countries; (2) the lead bioleaching in this study only employs a pure bacterial culture (herein Citrobacter sp.) that is able to oxidize both iron and sulfur as well as proliferate at high NaCl concentration and highly resistant to toxic metals, while previous studies mostly utilize a mixed culture of chemolithoautotrophs dominated by Acidithiobacillus ferrooxidans, Acidithiobacillus thiooxidans and Leptospirillum ferrooxidans that are very sensitive to organic compounds; (3) The lead bioleaching in this study takes place at room temperature (25°C) and the pH range of 3.5–4.6 that are more advantageous to energy-saving lead leaching process and the reduced usage of expensive anti-corrosive materials; (4) Medium used in this study contains NaCl, which elevates lead bioleaching efficiencies where NaCl can be replaced with seawater that is commonly used in many metallurgy and mining industries, thus providing a cost-effective lead leaching technology; (5) By using this local mixotrophic bacterium Citrobacter sp., the lead bioleaching from the galena concentrate is selective to Cu and Zn, thereby providing a selective lead leaching process that is profitable for subsequent Cu and Zn leaching processes since Pb (PbS) in association with CuFeS2 and ZnS always makes passivation layers that hinder Cu and Zn dissolution; (6) The bacterium Citrobacter sp. also produces biosurfactants (including EPS) that are useful for maintaining Fe3+ solubilization at pH > 4.0.
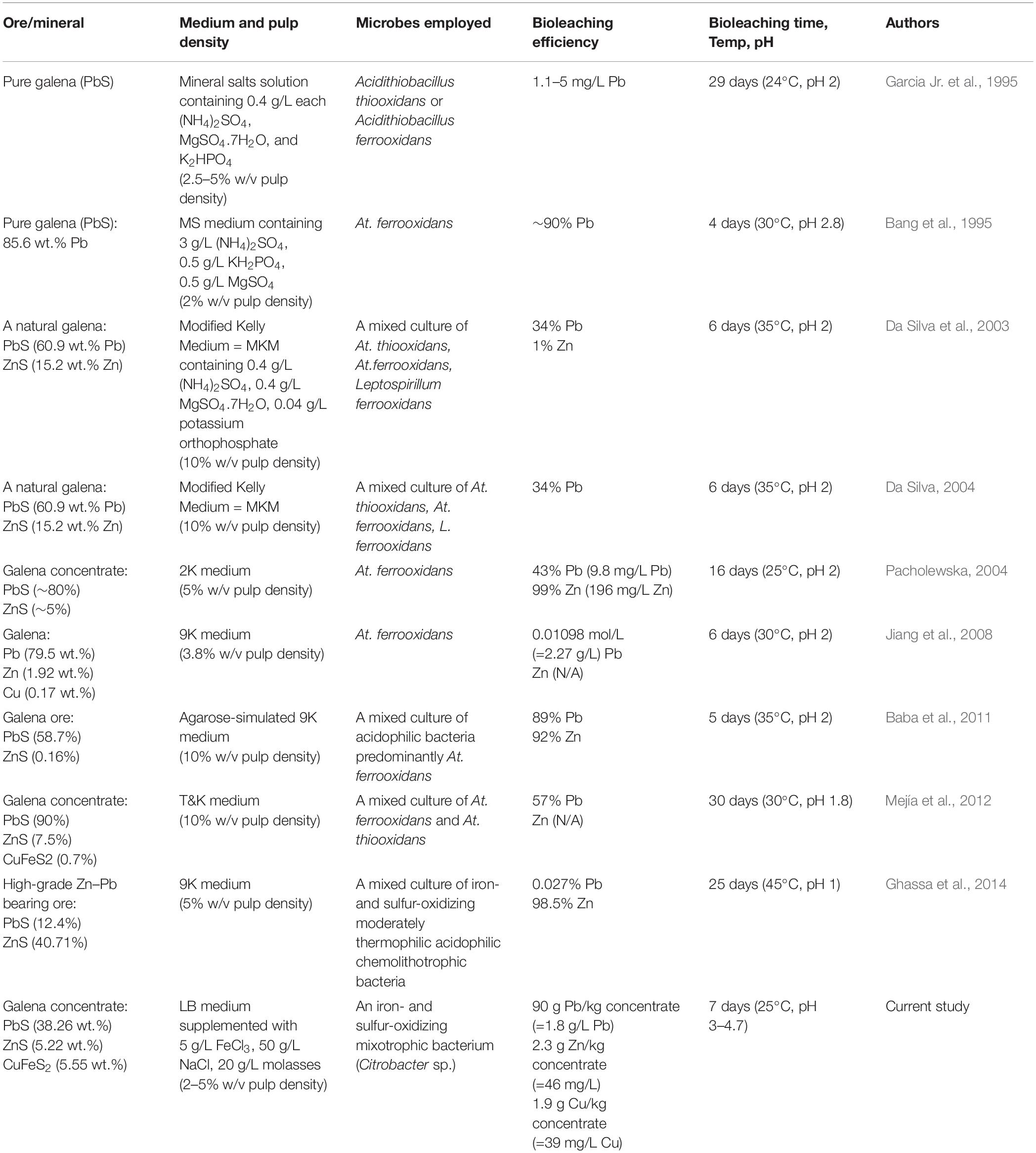
Table 2. Previous studies and the current study on bioleaching lead (Pb) from pure galena, galena concentrates, and high-grade Pb-Zn bearing ores.
Therefore, the specific aims of the current study were: (1) to investigate biohydrometallurgical leaching process of Indonesian galena concentrates (the main composition of PbS, CuFeS2, and ZnS) by the local mixotrophic bacterium Citrobacter sp. at room temperature (25°C) by employing two different bioleaching methods (i.e., semi-direct and direct bioleaching) and utilizing the LB medium supplemented with FeCl3, NaCl and molasses to minimize the formation of PbSO4 precipitates, which had the low solubility of lead within a sulfate system, (2) to evaluate the effect of different molasses concentrations on lead bioleaching efficiency since the bacterium Citrobacter sp. produced high amounts of biosurfactants by consuming organic carbon (herein molasses), and (3) to assess the effect of different NaCl and FeCl3 concentrations on lead bioleaching efficiency since the presence of NaCl and FeCl3 enhanced lead recovery. The findings of this study may provide further insights into the bioleaching of galena that is rarely studied due to insoluble anglesite formation and the toxicity of high lead content in galena to microbes. For our knowledge, this is the first report on the selective bioleaching of lead sulfide ores (herein galena concentrate) from Indonesia that is always associated with sphalerite and chalcopyrite using the local mixotrophic bacterium Citrobacter sp. in molasses-supplemented medium containing high NaCl concentration, which thus has potential industrial application since most of the metallurgy and mining industries use seawater in their mineral processing operations.
Materials and Methods
Bacterium and Growth Medium
A local mixotrophic bacterium used in this study was isolated from an Indonesian mine site (designated SKC2), which has the ability to oxidize iron and sulfur and produce extracellular polymeric substances (EPS) (Mubarok et al., 2017). Based on the 16S rRNA sequencing analysis, this bacterium was identified as Citrobacter sp. (98.45% similarity). The LB broth medium (10 g/L tryptone, 5 g/L yeast extract, 10 g/L NaCl) was used for both semi-direct and direct bioleaching experiments since the preliminary experiments in screening the appropriate medium for lead bioleaching with this bacterium showed the higher lead extraction than the modified Fe-broth medium [0.5 g/L MgSO4.7H2O, 3 g/L (NH4)2SO4, 0.5 g/L K2HPO4, 0.1 g/L KCl, 0.5 g/L tryptone, 5 g/L Na2S2O3.5H2O, and 1 g/L FeSO4.7H2O]. In addition, molasses was obtained from a sugar company, Padalarang, West Java, Indonesia.
Galena Concentrate
The galena concentrate employed in this study was kindly provided by an Indonesian mining company in Bogor, West Java, Indonesia (06°29′ S and 106°33′ E), with a particle size of d80 = 75 μm. ED-XRF analysis of the concentrate determined its chemical composition, as summarized in Table 3. X-ray powder diffractometry (XRD) analysis showed its mineralogical composition in which galena was predominant in the concentrate sample with a low amount of other minerals such as chalcopyrite and sphalerite (data not shown).
Experimental Procedure
Two leaching experiments were performed in this study: (1) semi-direct bioleaching experiments, and (2) direct bioleaching experiments. Bioleaching experiments were conducted in duplicate in sterile 300 ml Erlenmeyer flasks containing 150 ml of bacterial growth medium (LB medium) under aerobic conditions. The growth medium was then supplemented with various concentrations of FeCl3 (5, 15, and 25 g/L FeCl3), NaCl (30 and 50 g/L NaCl) and molasses (10, 20, and 30 g/L molasses) at various pulp densities (2 and 5% w/v) of the galena concentrate, and the pH was adjusted to 4.0 with HCl. Molasses was supplemented to enable the bacterium Citrobacter sp. to generate large amounts of biosurfactants (including EPS), thus being capable of preventing ferric (Fe3+) ions and PbSO4 precipitation. Bacterial inoculum (15% v/v) was subsequently introduced into bioleaching suspension, and the cultures were then incubated for 7 days at room temperature (25°C) with shaking at 180 rpm. Periodically, the pH of the suspension was measured using a pH meter (Lutron PE-03), while the redox potential (Eh) of the suspension was measured using an ORP electrode with Ag/AgCl reference (Lutron ORP-14). The solution (5 ml) was removed daily for measuring dissolved metal concentration by using atomic absorption spectrophotometer (AAS; Shimadzu AA-6300, Japan). The percentage of metal extraction (Pb, Cu, and Zn) and selectivity for lead leaching by iron- and sulfur-oxidizing mixotrophic bacterium to Cu (SCu) and to Zn (SZn) were calculated using the equations as described in our earlier work (Chaerun et al., 2017). After 7 days of bioleaching, separate sets of samples (i.e., the galena concentrate residues resulting from the experiments which led to the highest lead extraction for both semi-direct and direct bioleaching experiments) were made up and prepared for analysis by X-ray powder diffraction analysis (XRD; Rigaku Smartlab), Fourier transform infrared (FTIR Prestige 21, Shimadzu, Japan) and scanning electron microscopy equipped with energy dispersive spectroscopy (SEM-EDS; JEOL JSM-J6510 A). Samples were washed three times with deionized water before being observed by FTIR and XRD, while XRD measurement was carried out using Cu-Kα radiation, generated at 40 kV and 30 mA, using the 2θ/θ method at a scan speed of 2o/min. For SEM-EDS observation, samples were fixed with 2.5% v/v glutaraldehyde in 5 mM phosphate buffer at pH 7.0 for 24 h at 4°C, washed twice with 5 mM phosphate buffer, dehydrated in a graded series of acetone (25, 50, 75, and 100%) for 15 min, 15 min, 15 min, and 24 h, respectively.
For semi-direct bioleaching experiments, the galena concentrate was introduced into the culture medium after 3 days of incubation. For direct bioleaching experiments, the concentrate was introduced into the medium at the onset of the experiments. In comparison, the abiotic control leaching experiments were also conducted to ensure the bacterial participation in lead bioleaching processes, which were identical to those for semi-direct and direct bioleaching experiments, except that the bacterium was not added. The data are presented as the averages obtained from the duplicate experiments, and error bars represent standard deviation. Also, biosurfactant production by the mixotrophic bacterium during its bacterial growth for 48 h was assayed by measuring the emulsifying activity index (EI, %) following the work of Berg et al. (1990) with modification (Chaerun et al., 2018). This assay was conducted to confirm the role of the generated biosurfactants (including EPS as high-molecular-weight biosurfactants) in promoting lead bioleaching efficiencies. Briefly, the sample (2 mL) of the bacterial culture broth was mixed with 5 mL of TM buffer, which contained 20 mmol Tris-HCl buffer (pH 7.0) and 10 mmol MgSO4.7H2O per liter of deionized water, followed by addition of 1 mL coconut oil. After the mixture was vortexed for 3 min and incubated at room temperature for 1 min, the first turbidity (A1) of the aqueous phase was measured at 600 nm. Subsequently, after the mixture was incubated at room temperature for 60–90 min, the second turbidity (A2) of the aqueous phase was measured. Emulsifying activity index (EI, %) was then expressed in Eq. (1).
Results and Discussion
Semi-Direct Bioleaching
Semi-direct bioleaching experiments were conducted by adding various concentrations of FeCl3 (5 and 25 g/L), NaCl (30 and 50 g/L) and molasses (10, 20, and 30 g/L) at 5% w/v pulp density to evaluate their effects on lead recovery. Figures 1A–C demonstrate the effect of various concentrations of FeCl3 (5 and 25 g/L) and NaCl (30 and 50 g/L) on lead, copper and zinc extraction (mg metals of Pb, Cu, Zn dissolved per kg concentrate; mg/kg) by iron-and sulfur-oxidizing mixotrophic bacterium (Citrobacter sp.) in the semi-direct bioleaching of the galena concentrate over a 7-day period of the experiment at bioleaching parameters of 15% v/v bacterial inoculum, 5% w/v pulp density, and 10 g/L molasses. Lead bioleaching efficiencies from the concentrate increased rapidly during the first 1 day and subsequently increased slightly for the remaining bioleaching time, which achieved the extraction levels of 37–39 g/kg (for the addition of 5 g/L FeCl3 with 30 g/L or 50 g/L NaCl) and 22 g/kg (for the addition of 25 g/L FeCl3 with 30 g/L or 50 g/L NaCl) (Figure 1A). These increments were concomitant with an increase in copper and zinc bioleaching efficiencies (Figures 1B,C), except for copper dissolution with the addition of 5 g/L FeCl3 with 30 g/L or 50 g/L NaCl which was negligible (Figure 1B). Zinc dissolution was quite low compared to lead and copper extraction, thus being considered to be selective bioleaching to zinc. No difference was observed for lead extraction from the galena concentrate at any NaCl concentration (30 g/L or 50 g/L NaCl), and its extraction was apparently governed by the presence of FeCl3, of which 5 g/L was the best concentration (Figure 1A). The presence of FeCl3 also affected the initial pH of the suspension, where the higher FeCl3 concentration introduced into the solution yielded the more acidic suspension (Figure 1D). This lower pH was a result of the hydrolysis of FeCl3, generating HCl, which therefore lowered the suspension pH. Moreover, the pH of the suspensions tended to increase over time as a result of sulfide mineral oxidation that consumed proton (H+), whereas the suspension Eh values containing the higher FeCl3 concentration (25 g/L) were observed to be higher than those containing the lower FeCl3 concentration (5 g/L) due to the oxidizing capacity of FeCl3, which had a more oxidizing agent of Fe3+ (Figure 1E). Since the semi-direct bioleaching at 5 g/L FeCl3 and 50 g/L NaCl was highly selective to Cu (SCu = 0.99) and was relatively selective to Zn (SZn = 0.83) (Figures 1B,C and Table 4), hereafter the concentration was used for further bioleaching experiments to enhance the extraction of Pb as well as to prevent the formation of PbSO4 precipitates as well as ferric (Fe3+) ion precipitation by adding organic compounds (herein molasses).
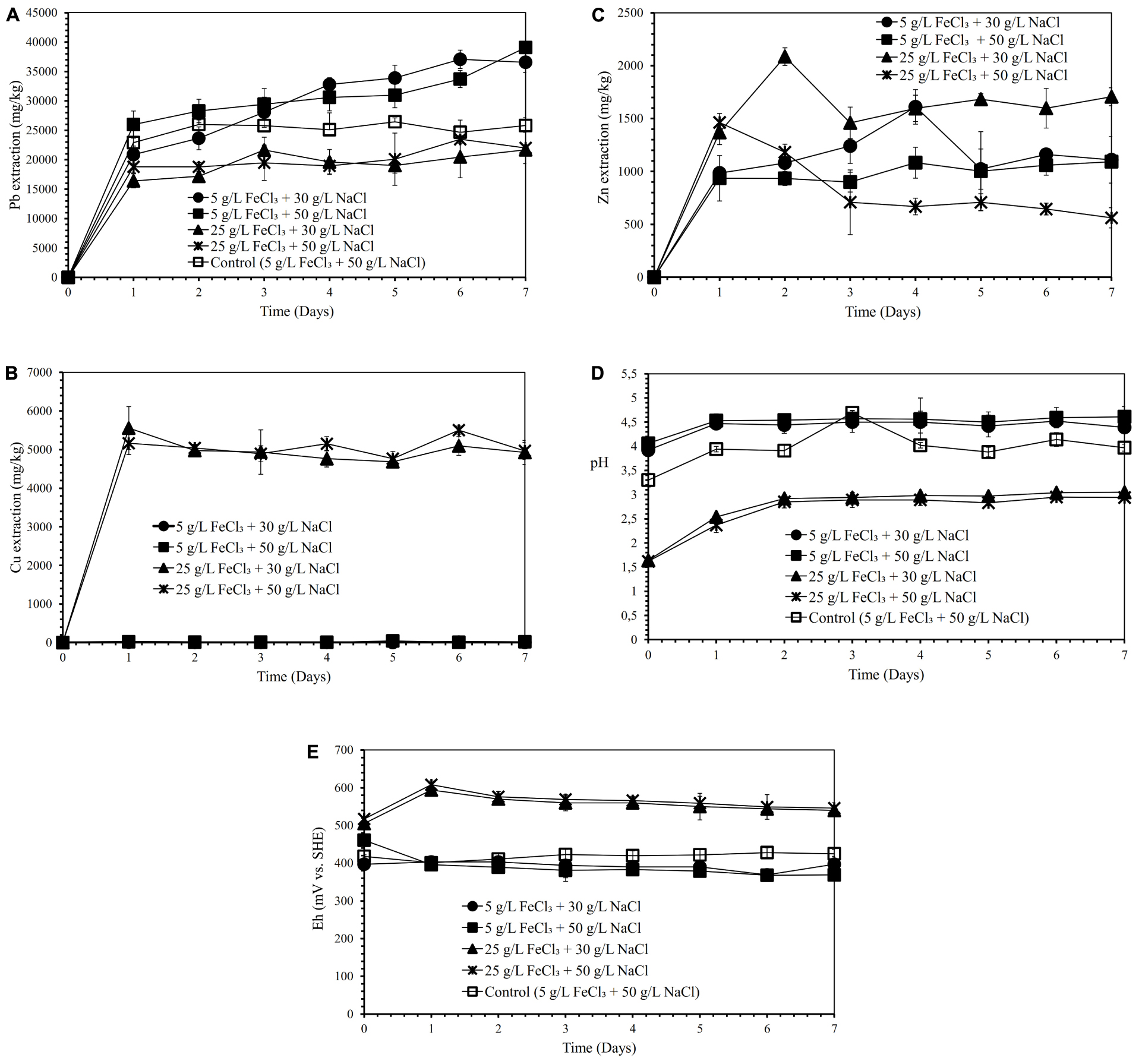
Figure 1. Lead (Pb) extraction (mg Pb dissolved/kg galena concentrate) (A), Copper (Cu) extraction (mg Cu dissolved/kg galena concentrate) (B), Zinc (Zn) extraction (C) (mg Zn dissolved/kg galena concentrate), pH (D) and Eh (mV vs. SHE) (E) of the galena concentrate semi-direct bioleaching suspension by an iron- and sulfur-oxidizing mixotrophic bacterium (Citrobacter sp.) at various concentrations of FeCl3 (5 and 25 g/L) and NaCl (30 and 50 g/L) at bioleaching parameters of 15% v/v bacterial inoculum, 5% w/v pulp density, 10 g/L molasses, a rotation speed of 180 rpm over a 7-day period of the experiment in comparison with those of the abiotic control semi-direct leaching (without bacteria) at 5 g/L FeCl3 and 50 g/L NaCl.
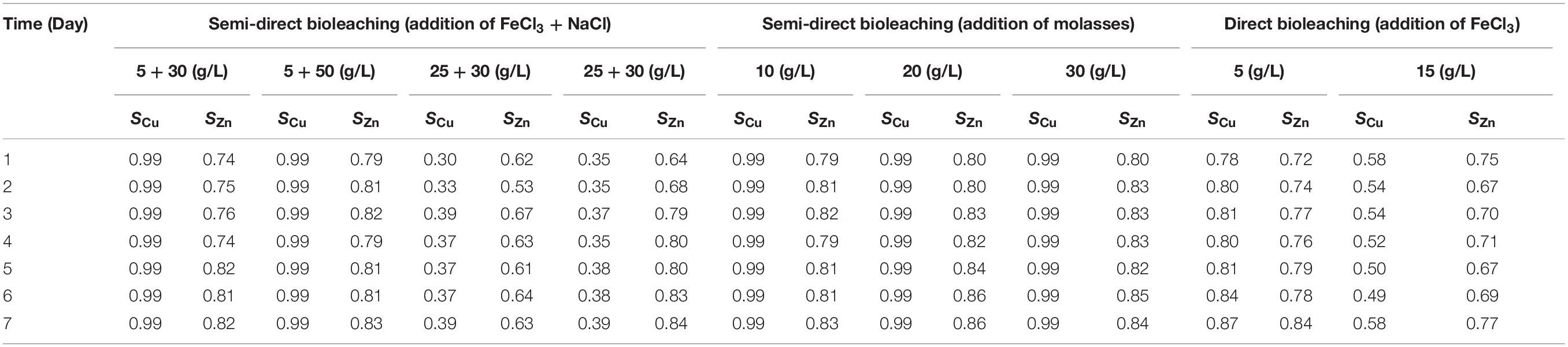
Table 4. The selectivity of lead (Pb) bioleaching by an iron- and sulfur-oxidizing mixotrophic bacterium (Citrobacter sp.) to Cu (SCu) and Zn (SZn) based on the effect of varying concentrations of FeCl3, NaCl and molasses as well as different bioleaching methods (Figures 1–3) over a 7-day period of bioleaching experiment.
Figures 2A–C show the effects of various molasses concentrations (10, 20, and 30 g/L) on lead, copper, and zinc extraction (mg metals of Pb, Cu, Zn dissolved per kg concentrate; mg/kg) by iron- and sulfur-oxidizing mixotrophic bacterium (Citrobacter sp.) in semi-direct bioleaching of the galena concentrate over 7 days of the experiment at bioleaching parameters of 15% v/v bacterial inoculum, 5% w/v pulp density, 5 g/L FeCl3 and 50 g/L NaCl. Lead extraction (39∼50 g/kg) from the concentrate was observed for three different molasses concentrations tested, and molasses concentration of 20 and 30 g/L appeared to bring about higher lead extraction than that of 10 g/L. The lead bioleaching efficiencies from the concentrate increased rapidly during the first 1 day and subsequently increased slightly for another 6 days (Figure 2A). It appeared that the addition of molasses created selective bioleaching, the high selectivity for copper, and the relatively high selectivity for zinc (Figures 2B,C and Table 4). During the bioleaching experiments, the suspension pH tended to increase, while the Eh otherwise decreased (Figures 2D,E). Since 20 g/L molasses was the best concentration for lead extraction with the high selectivity of lead bioleaching to Cu (SCu = 0.99) and Zn (SZn = 0.86), hereafter, the concentration was used in the subsequent experiments (direct bioleaching experiments).
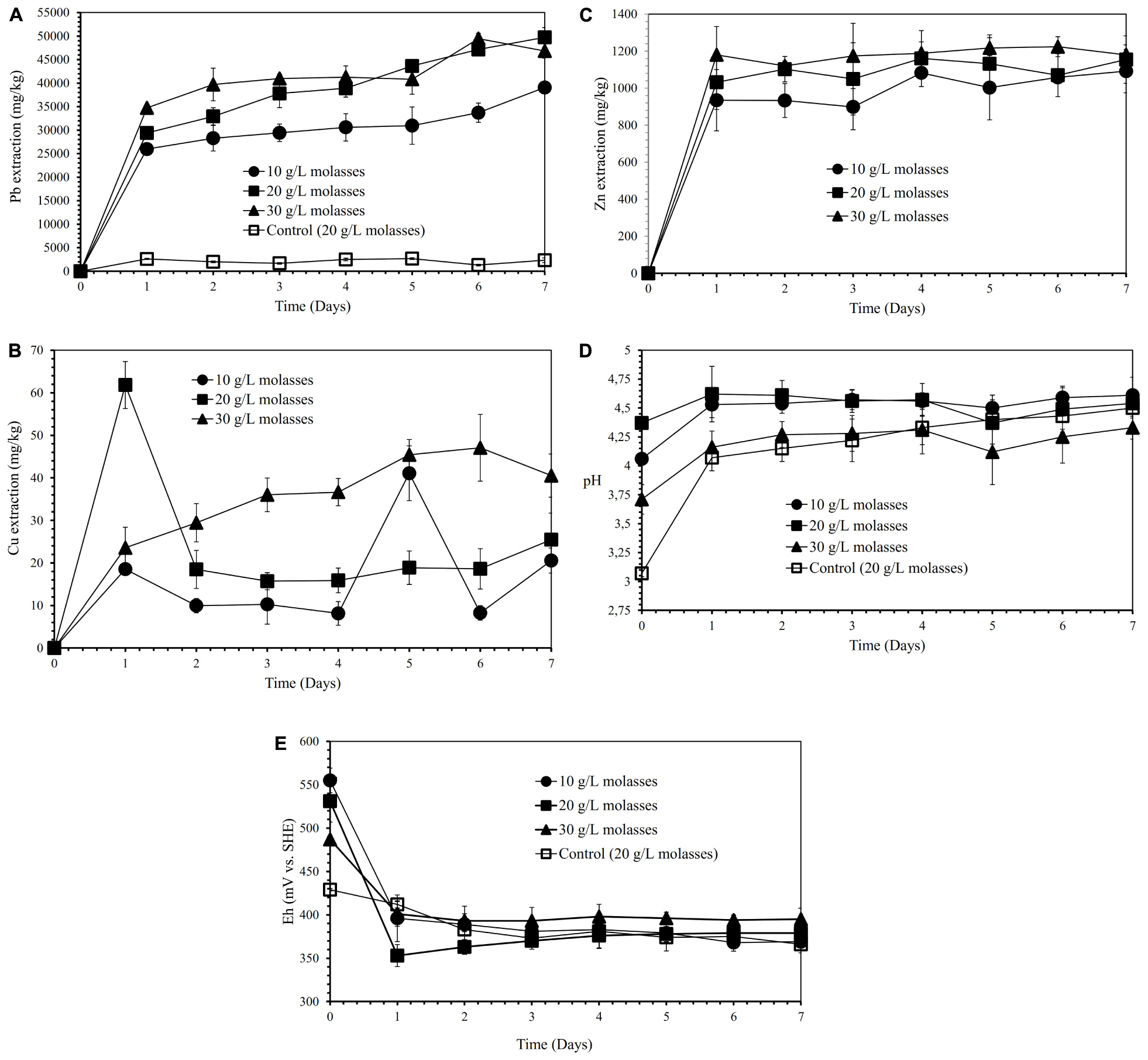
Figure 2. Lead (Pb) extraction (mg Pb dissolved/kg galena concentrate) (A), Copper (Cu) extraction (mg Cu dissolved/kg galena concentrate) (B), Zinc (Zn) extraction (C) (mg Zn dissolved/kg galena concentrate), pH (D) and Eh (mV vs. SHE) (E) of the galena concentrate semi-direct bioleaching suspension by an iron- and sulfur-oxidizing mixotrophic bacterium (Citrobacter sp.) at various molasses concentrations (10, 20, and 30 g/L) at bioleaching parameters of 15% v/v bacterial inoculum, 5% w/v pulp density, 5 g/L FeCl3, 50 g/L NaCl, a rotation speed of 180 rpm over a 7-day period of the experiment in comparison with those of the abiotic control semi-direct leaching (without bacteria) at 20 g/L molasses.
Direct Bioleaching
Figures 3A–C show the effects of various FeCl3 concentrations (5 and 15 g/L) on lead, copper, and zinc extraction (mg metals of Pb, Cu, Zn dissolved/kg galena concentrate) by iron- and sulfur-oxidizing mixotrophic bacterium (Citrobacter sp.) in the direct bioleaching of the galena concentrate over a 7-day period of the experiment at bioleaching parameters of 15% v/v bacterial inoculum, 2% w/v pulp density, 20 g/L molasses, and 50 g/L NaCl. Through the direct bioleaching method, a higher level of Pb extraction (84∼90 g/kg) than semi-direct bioleaching was observed for two FeCl3 concentrations (5 and 15 g/L) examined (Figure 3A). However, no significant difference was observed for lead extraction in both FeCl3 concentrations. Again, the lead bioleaching efficiencies from galena concentrate increased rapidly during the first 1 day, subsequently remained relatively constant for another 5 days, and slightly increased up to 7 d of bioleaching period. These increases occurred simultaneously with an increase in copper and zinc extraction (Figures 3B,C) with the exception of copper dissolution at 5 g/L FeCl3, which was quite low (Figure 3B). It was suggested from the results of this study that the direct bioleaching of galena concentrate at 5 g/L FeCl3 led to the high selectivity of lead bioleaching to copper (SCu = ∼0.9) and was relatively selective to zinc (SZn = 0.84) (Figures 3B,C and Table 4). Again, the initial pH of the suspensions containing the higher FeCl3 concentration resulted in more acidic suspension, and the pH values of the bacterial suspensions tended to elevate due to sulfide oxidation (Figure 3D). In contrast, the suspension Eh values tended to decline over time (Figure 3E). From the results of the lead extraction (Figures 1A, 2A, 3A), the lead extraction levels in control leaching were lower than those achieved in the bioleaching, indicating that the bacteria greatly participated in the lead bioleaching.
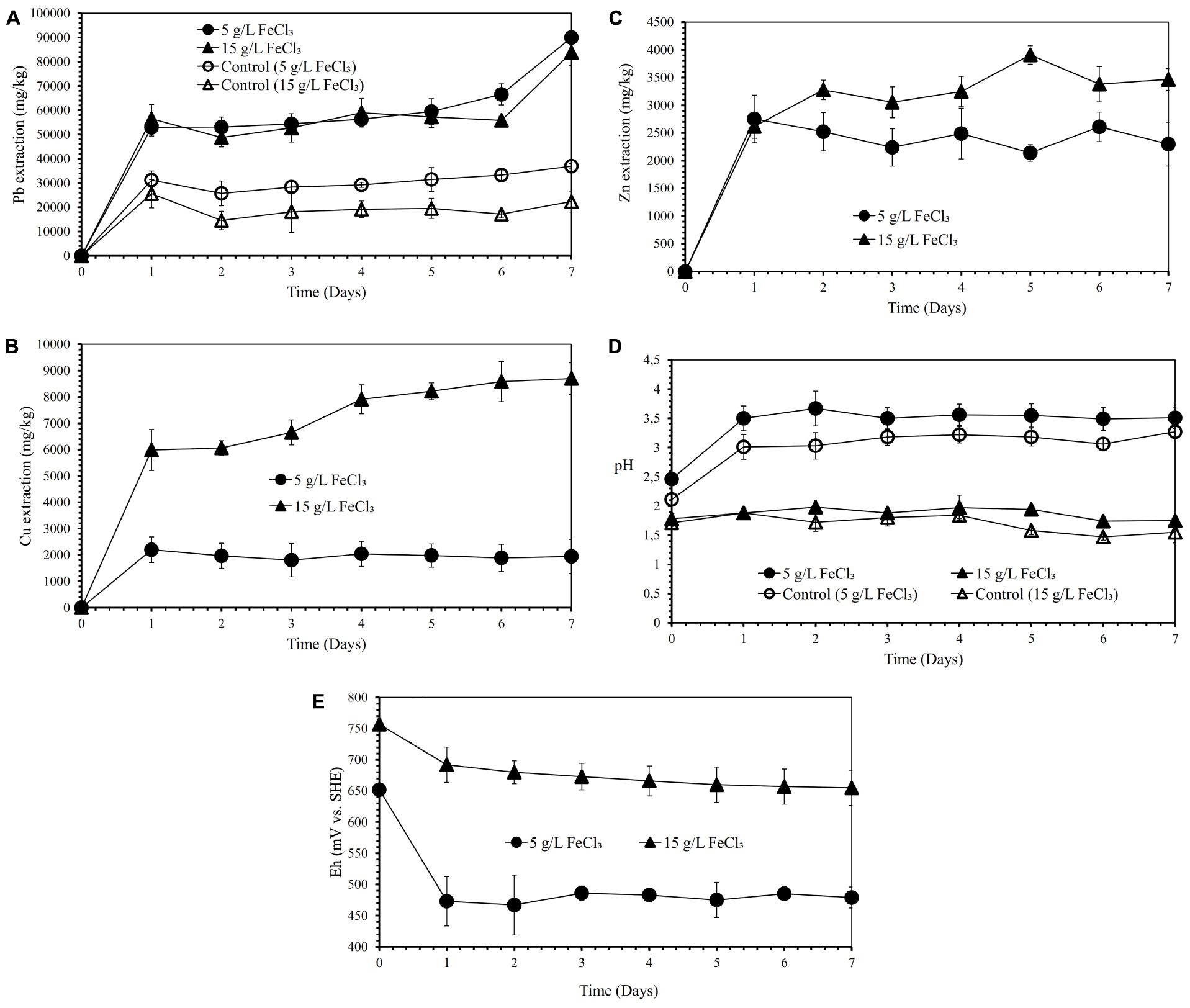
Figure 3. Lead (Pb) extraction (mg Pb dissolved/kg galena concentrate) (A), Copper (Cu) extraction (mg Cu dissolved/kg galena concentrate) (B), Zinc (Zn) extraction (C) (mg Zn dissolved/kg galena concentrate), pH (D) and Eh (mV vs. SHE) (E) of the galena concentrate direct bioleaching suspension by an iron- and sulfur-oxidizing mixotrophic bacterium (Citrobacter sp.) and the abiotic control direct leaching (without bacteria) at various FeCl3 concentrations (5 and 15 g/L) at bioleaching parameters of 15% v/v bacterial inoculum, 2% w/v pulp density, 20 g/L molasses, 50 g/L NaCl, a rotation speed of 180 rpm over a 7-day period of the experiment.
Characterization of Galena Concentrate Residues After Bioleaching
Figure 4 shows the XRD patterns of the galena concentrate and its residues in semi-direct bioleaching and direct bioleaching after 7 days of the bioleaching experiments. Both bioleaching techniques led to the formation of anglesite, thus hindering the enhancement of Pb extraction from the concentrate (Figures 2A, 3A). In addition, galena remained as a predominant mineral in leach residues in association with a small amount of chalcopyrite and sphalerite. From the XRD spectra, changes in mineralogical structure due to bioleaching were not discernible; hence FTIR analysis was performed to investigate chemical bondings in galena concentrate before and after bioleaching (Figure 5). The FTIR spectrum of starting galena concentrate contains bands at 400–800 cm–1 (inorganic components such as minerals and clays), 621 cm–1 (C-S stretching), 1,000–1,230 cm–1 (Si-O stretching), ∼1,081 cm–1 (C-O stretching vibrations of C-O-C groups, e.g., cellulose), ∼1,400 cm–1 (carboxylic and carbonylic groups), 1,620–1,640 cm–1 (hydrophilic C=O groups), and 3,000–3,700 cm–1 (O-H stretching, H-bonds, and OH-groups). It exhibited that the bands at 400–800 cm–1 (inorganic components such as minerals including galena) were reduced after bioleaching (both semi-direct and direct bioleaching processes) but not before the bioleaching process (as galena concentrate) (Figure 5). This reduction could be as a result of the bacterial role in bioleaching lead from galena concentrate, which was also supported by the sharp peaks of the leach residues at 1,385 and 1,535–1,660 cm–1 as an obvious indicator of the presence of bacterial cells (Chaerun et al., 2013). Moreover, the increased intensity of a broad band at 3,000–3,600 cm–1 (H-bonds and OH-groups) and two peaks at 2,860 and 2,920 cm–1 (asymmetric and symmetric C-H stretching vibrations of CH3 and CH2 groups) were observed for the leach residues. These results indicated that the residues retained more water and hydrophobic organic matters than the concentrates, which corresponded to the presence of extracellular polymeric substances (EPS) generated by the bacteria in this study. This observation is in agreement with the result of Chaerun et al. (2013), who reported that EPS, as a hygroscopic, highly hydrated biopolymer has the ability to retain water entropically. Other works also provide additional support for our FTIR results on EPS generation, demonstrating that the EPS is cell-bound and causes the bacterial cell surface to become more hydrophobic due to the hydrophobic properties of the EPS (Govender and Gericke, 2011) that are attributed to compounds, such as polysaccharide-linked methyl and acetyl groups (Flemming and Wingender, 2010).
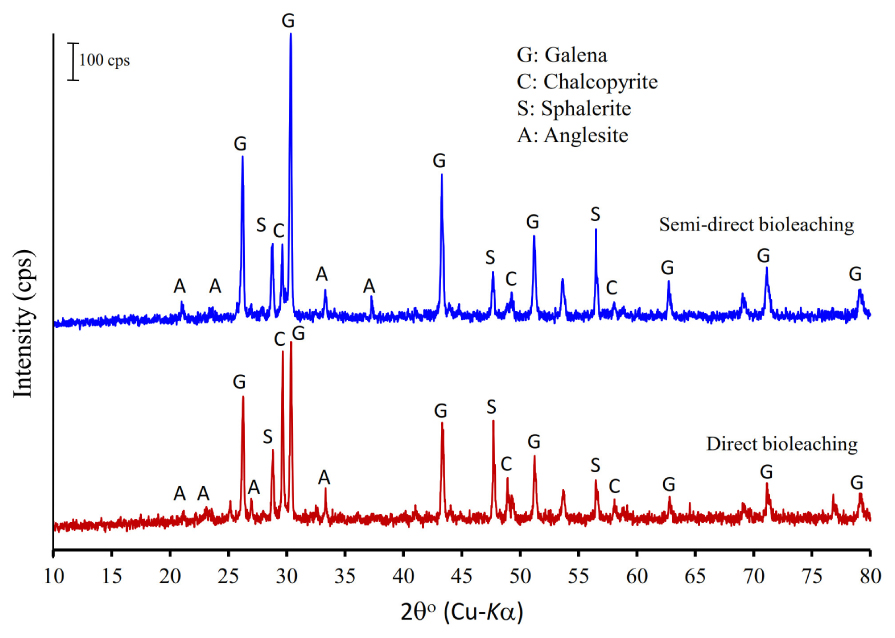
Figure 4. X-ray powder diffraction patterns of the galena concentrate residues in semi-direct bioleaching at bioleaching parameters of 5% w/v pulp density, 5 g/L FeCl3, 50 g/L NaCl, 20 g/L molasses and in the direct bioleaching at bioleaching parameters of 2% w/v pulp density, 5 g/L FeCl3, 50 g/L NaCl, 20 g/L molasses by iron- and sulfur-oxidizing mixotrophic bacterium (Citrobacter sp.) after 7 days of the bioleaching experiment.
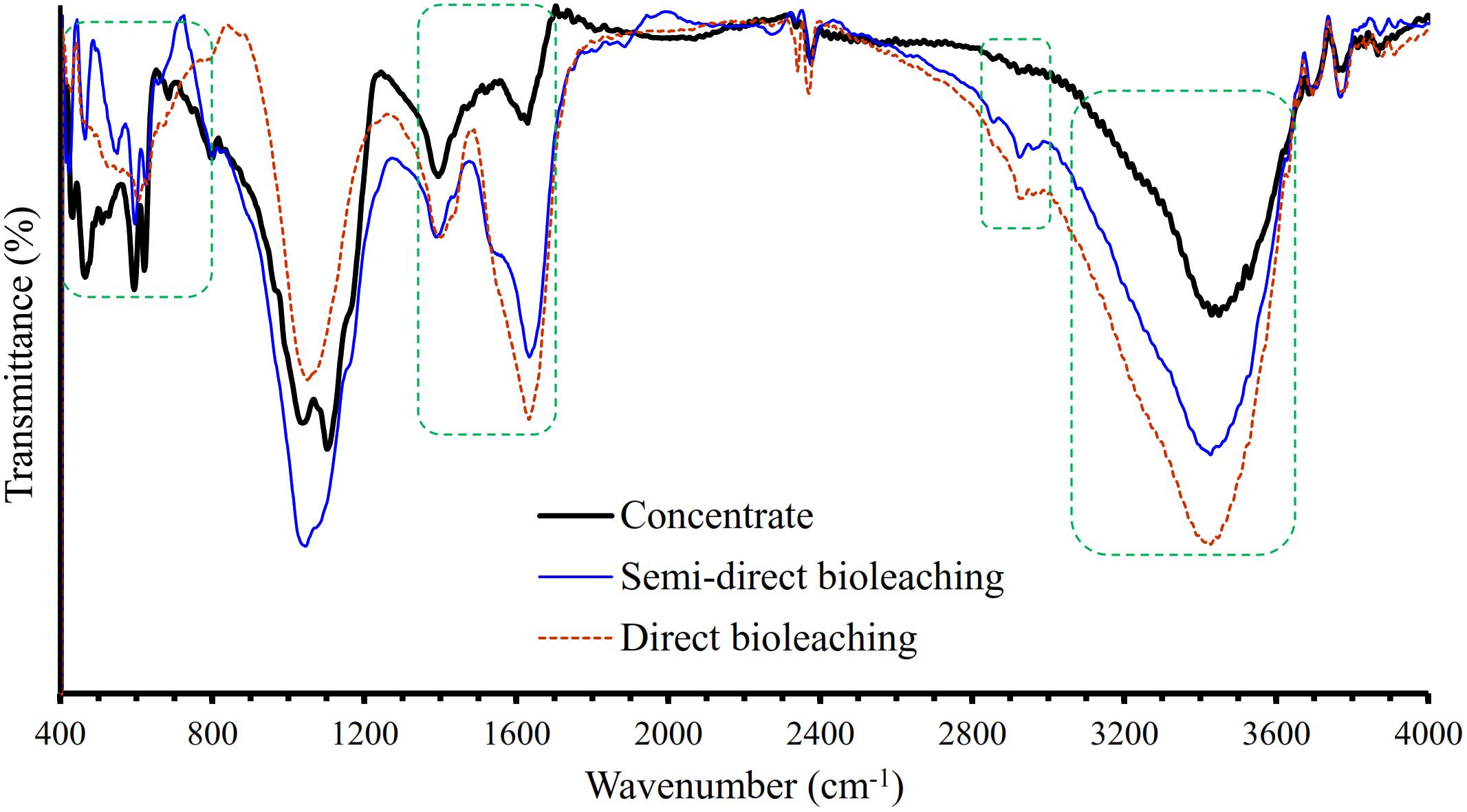
Figure 5. Fourier transform infrared (FTIR) spectra of the galena concentrate residues in semi-direct bioleaching at bioleaching parameters of 5% w/v pulp density, 5 g/L FeCl3, 50 g/L NaCl, 20 g/L molasses and in the direct bioleaching at bioleaching parameters of 2% w/v pulp density, 5 g/L FeCl3, 50 g/L NaCl, 20 g/L molasses by iron- and sulfur-oxidizing mixotrophic bacterium (Citrobacter sp.) after 7 days of the bioleaching experiment.
Furthermore, our SEM-EDS mapping observation of the galena concentrate residues (Figures 6, 7) also supports this hypothesis in that an iron- and sulfur-oxidizing mixotrophic bacterium (Citrobacter sp.) used in this study generates EPS, which thus forms the EPS-concentrate complexes and is consequently able to promote the interfacial degradation of the galena concentrate as well as the bioleaching of lead from the concentrate. This was represented by the formation of aggregates as a result of bacterial attachment to galena surfaces (due to EPS), which was also evidenced by the presence of C and N in the residues as the main component of bacterial cells and EPS content (Figures 6, 7). Small amounts of Cu and Zn revealed by SEM-EDS mapping observation in this study also confirmed the bioleaching of lead, copper, and zinc, as shown in Figures 2, 3. This result was also supported by the elemental contents of the residues (Table 5), demonstrating larger amounts of C, N, P, and smaller amounts of Pb, Cu, Zn in the residues than in the concentrate. In addition to FTIR and SEM-EDS observations, the role of EPS generated by the bacterium in elevating the lead bioleaching efficiencies rapidly during the first 1 day (Figures 1A, 2A, 3A) was also confirmed by the biosurfactant production (as represented by emulsifying activity index = EI) of the bacterium over 48 h of bacterial growth in the modified LB medium, demonstrating that the highest biosurfactant production was attained at 4 h of bacterial growth (Figure 8).
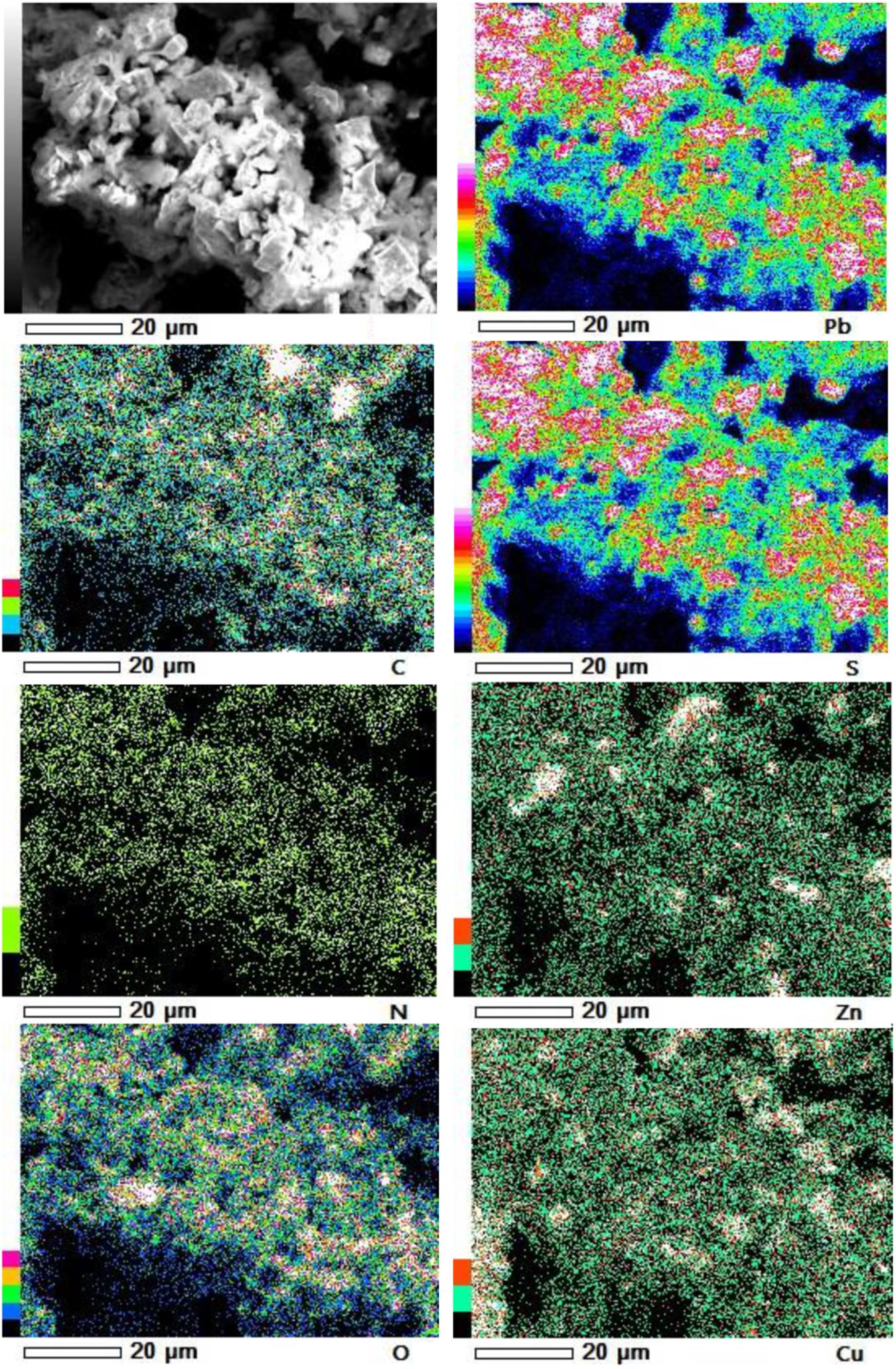
Figure 6. SEM-EDS maps of elemental compositions of the galena concentrate residues in semi-direct bioleaching at bioleaching parameters of 5% w/v pulp density, 5 g/L FeCl3, 50 g/L NaCl, 20 g/L molasses by iron- and sulfur-oxidizing mixotrophic bacterium (Citrobacter sp.) after 7 days of the bioleaching experiment.
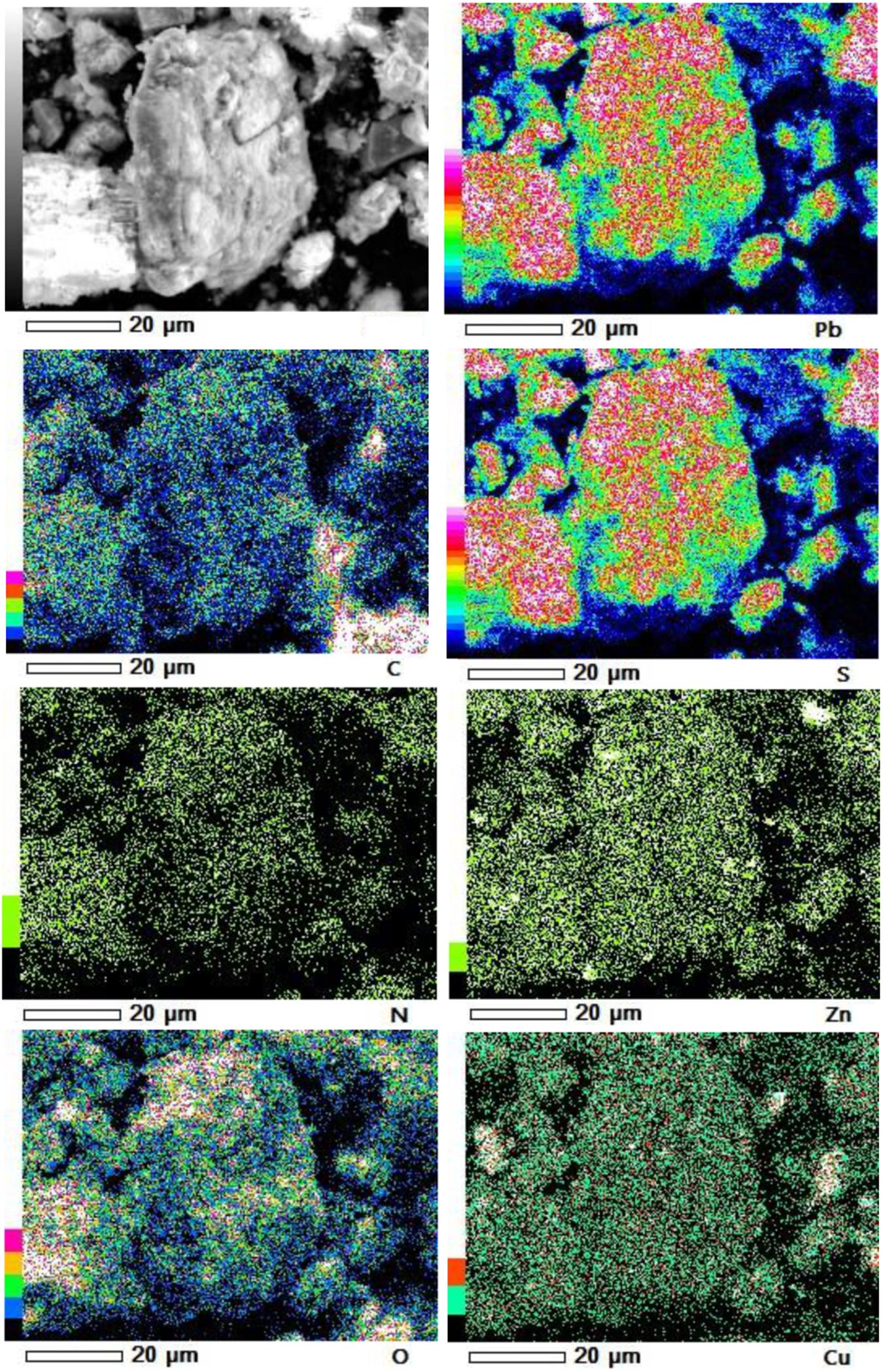
Figure 7. SEM-EDS maps of elemental compositions of the galena concentrate residues in the direct bioleaching at bioleaching parameters of 2% w/v pulp density, 5 g/L FeCl3, 50 g/L NaCl, 20 g/L molasses by iron- and sulfur-oxidizing mixotrophic bacterium (Citrobacter sp.) after 7 days of the bioleaching experiment.
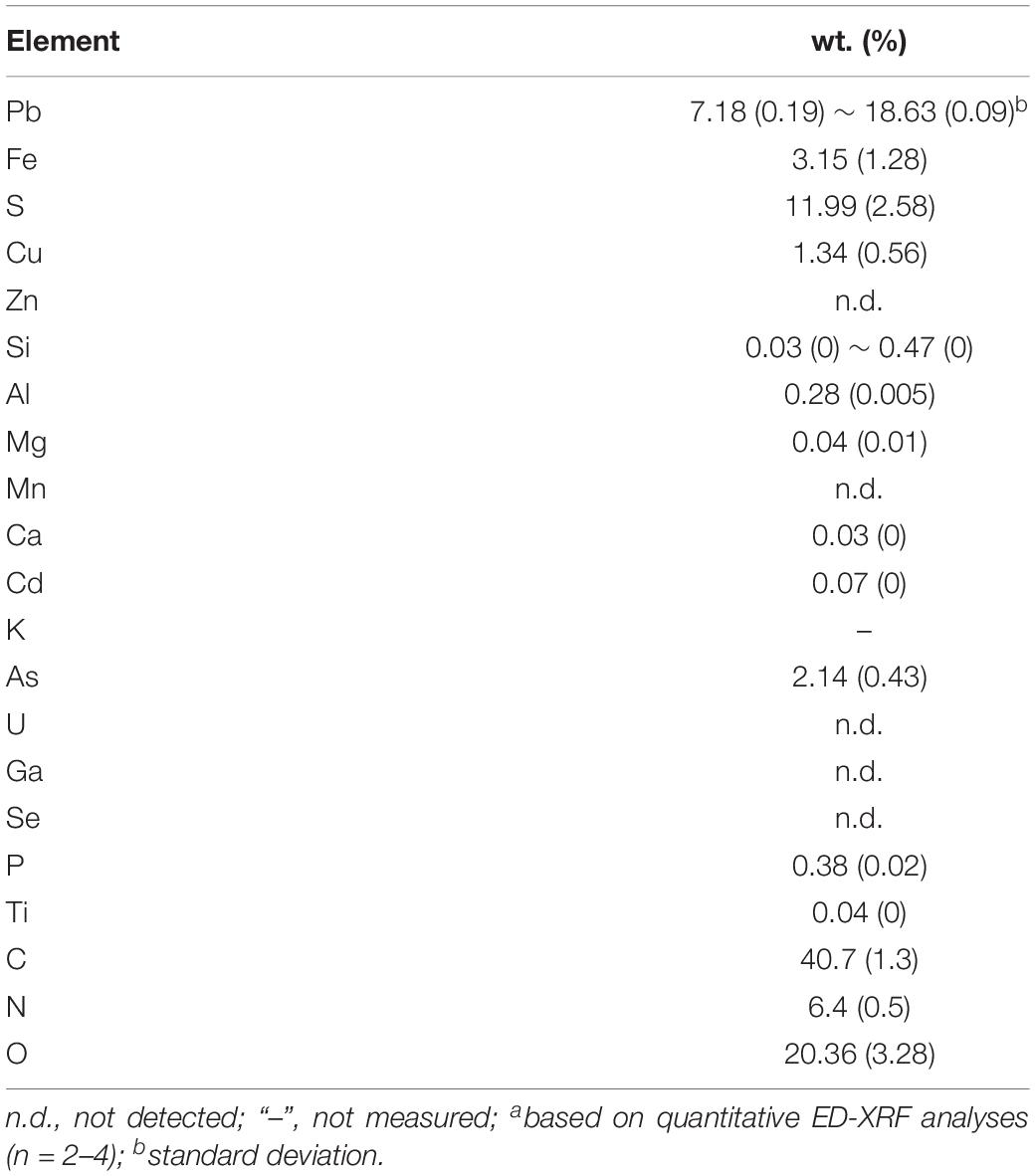
Table 5. Elemental concentration (a) of Indonesian galena concentrate after 7 days of bioleaching by an iron- and sulfur-oxidizing mixotrophic bacterium (Citrobacter sp.).
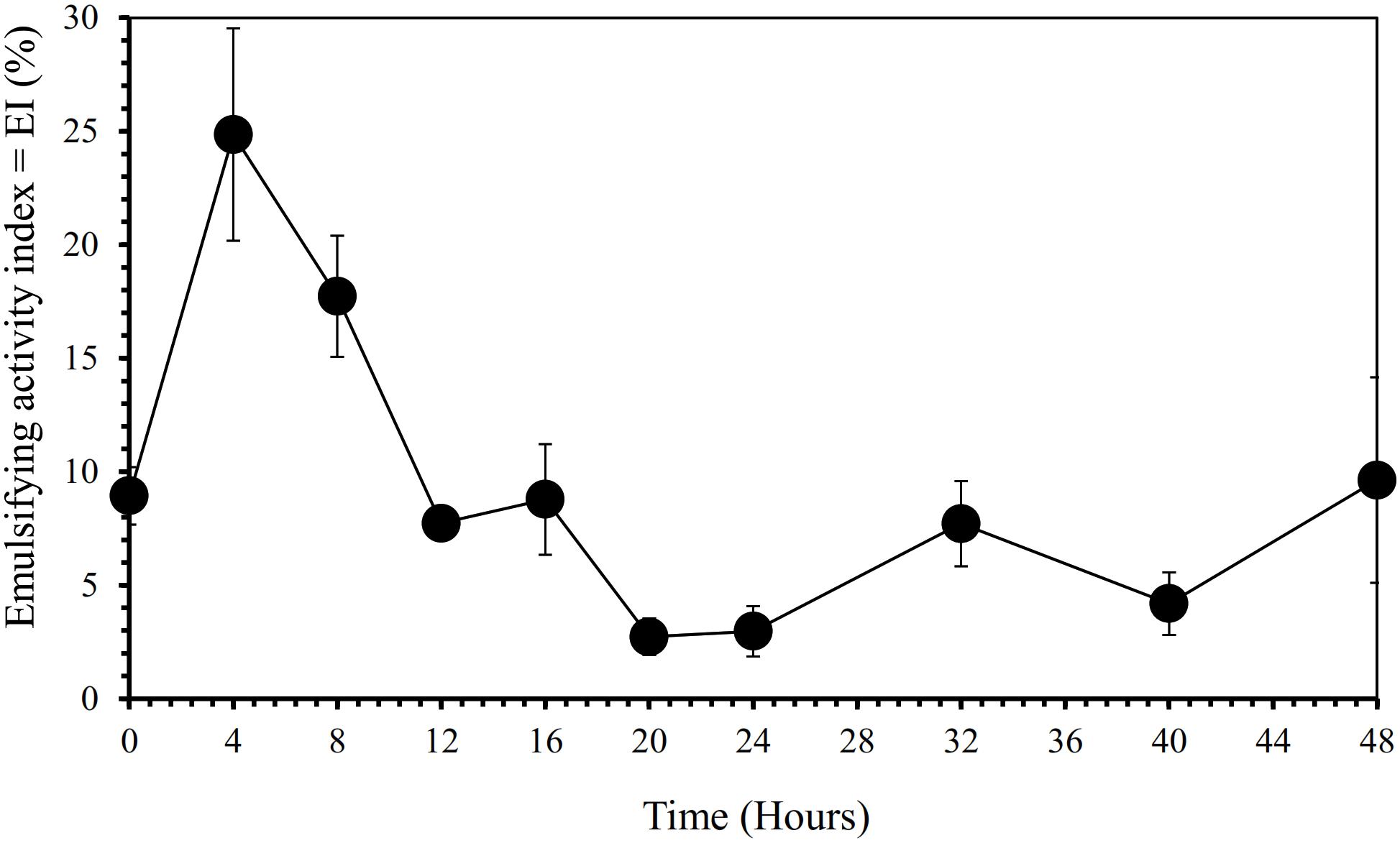
Figure 8. Biosurfactant production by iron- and sulfur-oxidizing mixotrophic bacterium (Citrobacter sp.) as represented by emulsifying activity index (EI in %) over 48 h of the bacterial growth in the modified LB medium.
From the results of this study, it could be seen that both semi-direct and direct bioleaching took place at the pH range of 1.6∼4.6 at which the bacterium Citrobacter sp. used in this study was able to proliferate, which had pH optima near neutrality and minimum and maximum pH values for growth 1.5 and 9.5, respectively. Meanwhile, both semi-direct and direct bioleaching exhibited the elevated lead extraction levels (∼50 and ∼90 g/kg, respectively) compared with copper (∼0.03 and ∼2 g/kg, respectively) and zinc (∼1 and ∼2.3 g/kg, respectively) extraction levels (Figures 2A–C, 3A–C), which were attained at the addition of 5 g/L FeCl3, 50 g/L NaCl, 20 g/L molasses at pulp density of 5% w/v (for semi-direct bioleaching) and 2% w/v (for direct bioleaching). The mixotrophic bacterium Citrobacter sp. (as a single culture) which exhibited the excellent selectivity of lead bioleaching to copper and zinc might be more beneficial for subsequent copper and zinc leaching processes than chemolithoautotrophic bacteria used in previous studies (listed in Table 2), which were not selective to zinc (Pacholewska, 2004; Baba et al., 2011; Ghassa et al., 2014), while selectivity for copper was not determined (Jiang et al., 2008; Mejía et al., 2012). It is reported that galena (PbS) that is often associated with zinc sulfides such as sphalerite (ZnS) and copper sulfides such as chalcopyrite (CuFeS2) frequently makes passivation layers that hinder Cu and Zn dissolution (Dutrizac, 1986; Dutrizac and Chen, 1990; Da Silva et al., 2003).
The overall reaction of PbS dissolution in the presence of FeCl3 and NaCl and the Gibbs free energy for PbS oxidation are presented in Eqs. (2)–(8) (Shock et al., 1997; Chase, 1998). According to the Gibbs free energy values, the reactions had negative values, indicating the spontaneous reaction. However, the negative values obtained said nothing about the kinetics because the kinetics was affected by the slowest reaction step in the leaching process. For example, Gibbs free energy for the dissolution of chalcopyrite (CuFeS2) in acidified ferric sulfate solution was negative (Hiroyoshi et al., 2000), but in fact, the copper extraction level in this system was very low, and long periods of time and high temperature were needed for the complete dissolution (Dutrizac, 1978). Moreover, if the dissolution process brings about non-porous solid products on the mineral surfaces, then the products can preclude the leaching agents such as ferric iron from reacting with the minerals and thus retard the kinetics, while the reactions might proceed faster or slower. The presence of bacteria acts as the catalyst, thus accelerating the reactions. In this study, the bacterium Citrobacter sp. was shown to increase Pb dissolution from the galena concentrate compared to abiotic control leaching without bacteria.
The oxidation reaction of PbS by ferric ions:
Oxidation reaction of elemental sulfur and ferrous ions by bacteria:
Reaction of Pb ions with chloride or sulfate ions:
Correspondingly, the molasses introduced into the bioleaching systems enabled the mixotrophic bacterium Citrobacter sp. to gain the energy needed to carry out its metabolic processes from organic compounds (as organic carbon source) in the presence of oxygen in which the bacterium was essential participants in the complete oxidation of organic compounds (CH2O) to CO2 + H2O along with a much greater yield of energy [Eq. (9)]. By utilizing the organic carbon, the bacterium was capable of producing large amounts of biosurfactants (including EPS), which mediated tight bacterial adherence to galena concentrate as well as kept solubilizing ferric iron even at pH > 4.0 as a result of complexation of the ferric ion with EPS (Vardanyan et al., 2020).
In summary, the current study presents a biohydrometallurgical leaching process of the Indonesian galena concentrate containing chalcopyrite and sphalerite at room temperature and atmospheric pressure, which exhibits selective leaching of lead to copper and zinc where this leaching technique prevents the release of SO2 and lead (Pb) dust emission. It is necessary to conduct further research to obtain the optimum lead bioleaching conditions by adapting the bacterium to galena concentrate first before employing it in the bioleaching systems since the current leaching study uses non-adapted bacteria with a low pulp density (2% w/v). By having the adapted bacteria, the higher pulp density in the leaching process can be increased, and Pb toxicity of higher pulp density can be reduced. Compared to the smelting technology currently used in the Pb extraction from galena (PbS), the biohydrometallurgical Pb leaching can potentially compete because of its advantage in the following aspects: more eco-friendly method, energy-saving leaching process, selective leaching of Pb to Cu and Zn. The selective Pb leaching to Cu and Zn demonstrated in this study thus makes a subsequent Cu and Zn extraction much easier in the downstream process (through biohydrometallurgical or hydrometallurgical route) since Pb has been removed. Moreover, the bacterium Citrobacter sp. in this study has shown to have the capacity to extract Pb from galena concentrate in the LB medium containing high NaCl concentration, therefore in the further research, the use of NaCl can be replaced with seawater, which is commonly used in mining and metallurgy industries, whereas tryptone can be substituted by molasses.
Conclusion
The present study has shown that an iron- and sulfur-oxidizing mixotrophic bacterium (Citrobacter sp.) used in this study is capable of extracting lead (Pb) from the Indonesian galena concentrate which achieves the highest extraction level of 90 g lead dissolved/kg galena concentrate using direct bioleaching method at bioleaching parameters of 2% w/v pulp density, 5 g/L FeCl3, 50 g/L NaCl, 20 g/L molasses and a rotation speed of 180 rpm at room temperature (25°C). The results of this study may, therefore, be advantageous to the improvement of Pb leaching from sulfide ores through a more environmentally friendly biohydrometallurgical route.
Data Availability Statement
The raw data supporting the conclusions of this article will be made available by the authors, without undue reservation, to any qualified researcher.
Author Contributions
EAP performed the experiments under the supervision of SKC. SKC wrote the manuscript, while MZM made figures and tables. All authors revised the manuscript.
Funding
This work was funded by a grant from the Research Program (P3MI), Institute for Research and Community Services, Institut Teknologi Bandung, Indonesia, to SKC, and was partially funded by the Indonesian Ministry of Research and Technology/National Agency for Research and Innovation, and Indonesian Ministry of Education and Culture, under World Class University Program managed by Institut Teknologi Bandung.
Conflict of Interest
The authors declare that the research was conducted in the absence of any commercial or financial relationships that could be construed as a potential conflict of interest.
Acknowledgments
We thank all the students of the Geomicrobiology-Biomining & Biocorrosion Laboratory and Microbial Culture Collection Laboratory, Biosciences and Biotechnology Research Center (BBRC), Institut Teknologi Bandung for their cooperation and assistance. We gratefully acknowledge Ronny Winarko (The University of British Columbia, Canada) for helpful discussion. We also thank Dr. Zhen Yu and Dr. Eldon R. Rene for their constructive comments.
References
Allen, M. A., and Igboayaka, E. C. (2019). The leaching behaviour of HNO3 solution in ishiagu ore, Ebonyi State Nigeria. Umudike J. Eng. Technol. 5, 130–138. doi: 10.33922/j.ujet_v5i1_15
Anugrah, R. I., Mubarok, M. Z., and Amalia, D. (2017). “Study on the leaching behavior of galena concentrate in fluosilicic acid solution using hydrogen peroxide as oxidant,” in Proceedings of the 1st International Process Metallurgy Conference (IPMC 2016). (Bandung: IPMC), 030006. doi: 10.1063/1.4974417
Aydoğan, S., Aras, A., Uçar, G., and Erdemoğlu, M. (2007). Dissolution kinetics of galena in acetic acid solutions with hydrogen peroxide. Hydrometallurgy 89, 189–195. doi: 10.1016/j.hydromet.2007.07.004
Baba, A. A., and Adekola, F. A. (2013). Solvent extraction of Pb(II) and Zn(II) from a Nigerian galena ore leach liquor by tributylphosphate and bis(2,4,4-trimethylpentyl)phosphinic acid. J. King Saud Univ. Sci. 25, 297–305. doi: 10.1016/j.jksus.2013.07.003
Baba, A. A., Adekola, F. A., Atata, R. F., Ahmed, R. N., and Panda, S. (2011). Bioleaching of Zn(II) and Pb(II) from Nigerian sphalerite and galena ores by mixed culture of acidophilic bacteria. Trans. Nonferrous Metals Soc. China 21, 2535–2541. doi: 10.1016/S1003-6326(11)61047-9
Bang, S. S., Deshpande, S. S., and Han, K. N. (1995). The oxidation of galena using Thiobacillus ferrooxidans. Hydrometallurgy 37, 181–192. doi: 10.1016/0304-386X(94)00059-C
Berg, G., Seech, A. G., Lee, H., and Trevors, J. T. (1990). Identification and characterization of a soil bacterium with extracellular emulsifying activity. J. Environ. Sci. Health A Environ. Sci. Eng. Toxicol. 25, 753–764. doi: 10.1080/10934529009375595
Chaerun, S. K., Frideni, Y. P., Minwal, W. P., Ichlas, Z. T., and Mubarok, M. Z. (2018). Bacterial leaching of an Indonesian complex copper sulfide ore using an iron-oxidizing indigenous bacterium. Microbiol. Indones 12, 1–6. doi: 10.5454/mi.12.1.1
Chaerun, S. K., Sulistyo, R. S., Minwal, W. P., and Mubarok, M. Z. (2017). Indirect bioleaching of low-grade nickel limonite and saprolite ores using fungal metabolic organic acids generated by Aspergillus niger. Hydrometallurgy 174, 29–37. doi: 10.1016/j.hydromet.2017.08.006
Chaerun, S. K., Tazaki, K., and Okuno, M. (2013). Montmorillonite mitigates the toxic effect of heavy oil on hydrocarbon-degrading bacterial growth: implications for marine oil spill bioremediation. Clay Miner. 48, 639–654. doi: 10.1180/claymin.2013.048.4.17
Da Silva, G. (2004). Kinetics and mechanism of the bacterial and ferric sulphate oxidation of galena. Hydrometallurgy 75, 99–110.
Da Silva, G., Lastra, M. R., and Budden, J. R. (2003). Electrochemical passivation of sphalerite during bacterial oxidation in the presence of galena. Miner. Eng. 16, 199–203. doi: 10.1016/S0892-6875(03)00010-14
Dutrizac, J. E. (1978). The kinetics of dissolution of chalcopyrite in ferric ion media. MTB 9, 431–439. doi: 10.1007/BF02654418
Dutrizac, J. E. (1986). The dissolution of galena in ferric chloride media. MTB 17, 5–17. doi: 10.1007/BF02670814
Dutrizac, J. E., and Chen, T. T. (1990). The effect of the elemental sulfur reaction product on the leaching of galena in ferric chloride media. MTB 21, 935–943. doi: 10.1007/BF02670264
Flemming, H.-C., and Wingender, J. (2010). The biofilm matrix. Nat. Rev. Microbiol. 8, 623–633. doi: 10.1038/nrmicro2415
Garcia, O. Jr., Tuovinen, O. H., and Bigham, J. M. (1995). Oxidation of galena by Thiobacillus ferrooxidans and Thiobacillus thiooxidans. Can. J. Microbiol. 41, 508–514. doi: 10.1139/m95-067
Ghassa, S., Boruomand, Z., Abdollahi, H., Moradian, M., and Akcil, A. (2014). Bioleaching of high grade Zn–Pb bearing ore by mixed moderate thermophilic microorganisms. Sep. Purif. Technol. 136, 241–249. doi: 10.1016/j.seppur.2014.08.029
Govender, Y., and Gericke, M. (2011). Extracellular polymeric substances (EPS) from bioleaching systems and its application in bioflotation. Miner. Eng. 24, 1122–1127. doi: 10.1016/j.mineng.2011.02.016
Greet, C., and Smart, R. S. C (2002). Diagnostic leaching of galena and its oxidation products with EDTA. Miner. Eng. 15, 515–522. doi: 10.1016/S0892-6875(02)00075-4
Hiroyoshi, N., Miki, H., Hirajima, T., and Tsunekawa, M. (2000). A model for ferrous-promoted chalcopyrite leaching. Hydrometallurgy 57, 31–38. doi: 10.1016/S0304-386X(00)00089-X
Jiang, L., Zhou, H., Peng, X., and Ding, Z. (2008). Bio-oxidation of galena particles by Acidithiobacillus ferrooxidans. Particuology 6, 99–105.
Kim, S.-H., Henein, H., and Warren, G. W. (1986). An investigation of the thermodynamics and kinetics of the ferric chloride brine leaching of galena concentrate. MTB 17, 29–39. doi: 10.1007/BF02670816
Liao, M. X., and Deng, T. L. (2004). Zinc and lead extraction from complex raw sulfides by sequential bioleaching and acidic brine leach. Miner. Eng. 17, 17–22. doi: 10.1016/j.mineng.2003.09.007
Long, H., Chai, L., Liu, H., and Qin, W. (2009). Hydro-chemical conversion of galena in FeCl3-KCl solution. Trans. Nonferrous Metals Soc. China 19, 1331–1335. doi: 10.1016/S1003-6326(08)60445-8
Mejía, E. R., Ospina, J. D., Márquez, M. A., and Morales, A. L. (2012). “Bioleaching of Galena (PbS),” in Fourier Transform-Materials Analysis, ed. S. M. Salih (Croatia: InTech), 191–206.
Mubarok, M. Z., Winarko, R., Chaerun, S. K., Rizki, I. N., and Ichlas, Z. T. (2017). Improving gold recovery from refractory gold ores through biooxidation using iron-sulfur-oxidizing/sulfur-oxidizing mixotrophic bacteria. Hydrometallurgy 168, 69–75. doi: 10.1016/j.hydromet.2016.10.018
Pacholewska, M. (2004). Bioleaching of galena flotation concentrate. Physicochem. Probl. Miner. Process. 38, 281–290.
Pashkov, G. L., Mikhlina, E. V., Kholmogorov, A. G., and Mikhlin, Y. L. (2002). Effect of potential and ferric ions on lead sulfide dissolution in nitric acid. Hydrometallurgy 63, 171–179. doi: 10.1016/S0304-386X(01)00221-3
Qin, W., Liu, H., Tang, S., and Sun, W. (2009). Preparation of lead sulfate powder directly from galena concentrates. Trans. Nonferrous Metals Soc. China 19, 479–483. doi: 10.1016/S1003-6326(08)60299-X
Shock, E. L., Sassani, D. C., Willis, M., and Sverjensky, D. A. (1997). Inorganic species in geologic fluids: correlations among standard molal thermodynamic properties of aqueous ions and hydroxide complexes. Geochimica Cosmochimica Acta 61, 907–950. doi: 10.1016/S0016-7037(96)00339-0
Vardanyan, N., Badalyan, H., Markosyan, L., Vardanyan, A., Zhang, R., and Sand, W. (2020). Newly isolated Acidithiobacillus sp. Ksh from Kashen copper ore: peculiarities of EPS and colloidal exopolysaccharide. Front. Microbiol. 11:1802. doi: 10.3389/fmicb.2020.01802
Warren, G. W., Kim, S., and Henein, H. (1987). The effect of chloride ion on the ferric chloride leaching of galena concentrate. MTB 18, 59–69. doi: 10.1007/BF02658432
Wu, Z., Dreisinger, D. B., Urch, H., and Fassbender, S. (2014). The kinetics of leaching galena concentrates with ferric methanesulfonate solution. Hydrometallurgy 142, 121–130. doi: 10.1016/j.hydromet.2013.10.017
Ye, M., Yan, P., Sun, S., Han, D., Xiao, X., Zheng, L., et al. (2017). Bioleaching combined brine leaching of heavy metals from lead-zinc mine tailings: transformations during the leaching process. Chemosphere 168, 1115–1125. doi: 10.1016/j.chemosphere.2016.10.095
Zárate-Gutiérrez, R., Gregorio-Vázquez, L., and Lapidus, G. T. (2015). Selective leaching of lead from a lead–silver–zinc concentrate with hydrogen peroxide in citrate solutions. Can. Metall. Q. 54, 305–309. doi: 10.1179/1879139515Y.0000000020
Keywords: semi-direct bioleaching, direct bioleaching, galena, lead (Pb), an iron- and sulfur-oxidizing mixotrophic bacterium
Citation: Chaerun SK, Putri EA and Mubarok MZ (2020) Bioleaching of Indonesian Galena Concentrate With an Iron- and Sulfur-Oxidizing Mixotrophic Bacterium at Room Temperature. Front. Microbiol. 11:557548. doi: 10.3389/fmicb.2020.557548
Received: 30 April 2020; Accepted: 10 September 2020;
Published: 08 October 2020.
Edited by:
Jinlan Xia, Central South University, ChinaReviewed by:
Zhen Yu, Guangdong Institute of Eco-Environmental Science and Technology, ChinaEldon R. Rene, IHE Delft Institute for Water Education, Netherlands
Copyright © 2020 Chaerun, Putri and Mubarok. This is an open-access article distributed under the terms of the Creative Commons Attribution License (CC BY). The use, distribution or reproduction in other forums is permitted, provided the original author(s) and the copyright owner(s) are credited and that the original publication in this journal is cited, in accordance with accepted academic practice. No use, distribution or reproduction is permitted which does not comply with these terms.
*Correspondence: Siti Khodijah Chaerun, c2tjaGFlcnVuQGdtYWlsLmNvbQ==; c2tjaGFlcnVuQG1ldGFsbHVyZ3kuaXRiLmFjLmlk