- 1Microbiology Department-Research Institute Biomedical A Coruña (INIBIC), Hospital A Coruña (CHUAC), University of A Coruña (UDC), A Coruña, Spain
- 2Study Group on Mechanisms of Action and Resistance to Antimicrobials (GEMARA), Spanish Society of Infectious Diseases and Clinical Microbiology (SEIMC), Madrid, Spain
- 3Microbiology Department-Health Research Institute of the Baleairc Islands (IdISBa), Hospital Son Espases, Palma de Mallorca, Spain
- 4Servicio de Microbiología, Hospital Ramón y Cajal, Instituto Ramón y Cajal de Investigación Sanitaria (IRYCIS), Madrid, Spain
- 5Spanish Network for Research in Infectious Diseases (REIPI), Seville, Spain
- 6Child Health Research Centre, School of Chemistry and Molecular Biosciences, The University of Queensland, Brisbane, QLD, Australia
Bacteriophages are important in bacterial ecology and evolution. Pseudomonas aeruginosa is the most prevalent bacterial pathogen in chronic bronchopulmonary infection in cystic fibrosis (CF). In this study, we used bioinformatics, microbiological and microscopy techniques to analyze the bacteriophages present in 24 P. aeruginosa isolates belonging to the international CF clone (ST274-CC274). Interestingly, we detected the presence of five members of the Inoviridae family of prophages (Pf1, Pf4, Pf5, Pf6, Pf7), which have previously been observed in P. aeruginosa. In addition, we identified a new filamentous prophage, designated Pf8, in the P. aeruginosa AUS411.500 isolate belonging to the international CF clone. We detected only one prophage, never previously described, from the family Siphoviridiae (with 66 proteins and displaying homology with PHAGE_Pseudo_phi297_NC_016762). This prophage was isolated from the P. aeruginosa AUS531 isolate carrying a new gene which is implicated in the phage infection ability, named Bacteriophage Control Infection (bci). We characterized the role of the Bci protein in bacteriophage infection and in regulating the host Quorum Sensing (QS) system, motility and biofilm and pyocyanin production in the P. aeruginosa isogenic mutant AUS531Δbci isolate. The findings may be relevant for the identification of targets in the development of new strategies to control P. aeruginosa infections, particularly in CF patients.
Introduction
Pseudomonas aeruginosa is a ubiquitous Gram-negative microorganism and a multidrug-resistant (MDR) pathogen. It is the main pathogen that causes chronic respiratory infection in cystic fibrosis (CF) and is associated with substantial morbidity and mortality in CF patients.
Bacteriophages are bacterial viruses that infect bacteria. Phages generally undergo a lytic (virulent) or lysogenic (temperate) life cycle. Lytic phages enter host cells and subsequently lyse and kill them, releasing phage progeny into the surrounding medium. Temperate phages possess the ability to go through a lysogenic cycle, entering the host cell and integrating their nucleic acid in the host genome or residing in the host cells as prophages, potentially existing in a stable state for generations until induced to start a lytic cycle (Clokie et al., 2011).
Bacteriophages in the family Inoviridae (inoviruses) have been described in P. aeruginosa biofilms and as promoters of biofilm formation (Whiteley et al., 2001; Webb et al., 2004; Knezevic et al., 2015; Secor et al., 2015). Numerous studies have shown the relationship between CF clinical isolates and Pf filamentous prophages (Finnan et al., 2004; Kirov et al., 2007; Manos et al., 2008; Mathee et al., 2008; Winstanley et al., 2009; Fothergill et al., 2012), which are long, narrow, tubular phages (about 2 μm in length and 6–7 nm in diameter) with positive-sense single-stranded circular DNA (Secor et al., 2015). Pf phages are inoviruses and usually become integrated in the chromosome of P. aeruginosa, although there are some exceptions, such as Pf1, which can replicate without being integrated in the host strain (Secor et al., 2015).
The pathogenic potential of P. aeruginosa is probably due to a combination of many different virulence factors. Several studies suggest that these factors are regulated by Quorum Sensing (QS) systems and/or bacteriophages (Lee and Zhang, 2015). The QS network in this pathogen consists of a series of connected circuits, i.e., LasI/LasR, RhlI/RhlR, QscR and PqsABCDEH/PqsR, which are regulated by molecules known as acyl-homoserine lactones (Wilder et al., 2011; Lee and Zhang, 2015; Papenfort and Bassler, 2016). Detection of these molecules indicates that P. aeruginosa is growing as a biofilm within the lungs of CF patients (Bjarnsholt and Givskov, 2007; Wilder et al., 2009; Winstanley and Fothergill, 2009). This bacterium permanently colonizes the lungs of CF patients, despite antibiotic treatment being administered. Microscope studies of sputum samples from these patients show that P. aeruginosa frequently resides within biofilms (Bjarnsholt and Givskov, 2007). Specific detection of P. aeruginosa via QS signaling may help to identify the agents involved in biofilm formation.
Quorum sensing systems and bacteriophages are associated with virulence and evolution of bacteria during both intermittent and chronic lung infections in CF. Some studies have shown the existence of bacteriophages in the sputum of CF patients (Ojeniyi et al., 1991; Fothergill et al., 2011), supporting the hypothesis that the bacteriophages play a role in respiratory infections in these patients. A strain of P. aeruginosa known as the Liverpool epidemic strain (LES) shows greater resistance to antibiotics than other strains isolated from CF patients. Genomic analysis of isolate LESB58 has demonstrated the presence of several prophages that increase the success of colonization by this P. aeruginosa strain as they form part of the accessory genome, the genes of which contribute to pathogenicity (Winstanley et al., 2009).
Relationships between QS and bacteriophage infection have been analyzed by several authors. Phage φpa3 has been proved to transduce mutations in QS genes in P. aeruginosa PAO1 (Monson et al., 2011). Moreover, it was demonstrated that QS systems may protect bacteria from bacteriophage infection reducing the phage receptor numbers at the stationary phase in Escherichia coli (Tan et al., 2015). In Vibrio cholerae, QS was demonstrated to control the change from a lysogenic cycle to a lytic one in the vibrio phage VP882 by QS-related genes encoded by the bacteriophage itself 30554875 (Silpe and Bassler, 2019).
In the present study, 24 sequences of P. aeruginosa isolates belonging to the international CF clone (ST274-CC274) were analyzed. A new filamentous prophage, designated Pf8, was identified in isolate AUS411, and analysis of its genome revealed a toxin/antitoxin system. Moreover, a new prophage from the Siphoviridae family was identified in isolate AUS531, which harbors a new gene that favors phage infectivity and bacterial QS control, that was named Bacteriophage Control Infection (bci).
Materials and Methods
CF Clinical Isolates
All isolates (9 from CF Australian patients and 15 from Spanish patients from different clinical units), previously classified as belonging to CC274, were respiratory tract isolates from CF patients, except PAMB148, which was a blood sample. Isolates were recovered during an 18-year period (1995–2012) and included sequential isolates from several patients (López-Causapé et al., 2017). The antibiotic susceptibility profile and main antibiotic resistance-related mutations were previously analyzed (López-Causapé et al., 2017).
Genome Sequencing and Analysis of the Isolates Belonging to the ST274 Clonal Complex (CC274)
Next Generation Sequencing (NGS) was performed in a previous study, with the MiSeq sequencing system (Illumina platform) (López-Causapé et al., 2017). The sequences were assembled using the Newbler Roche assembler and Velvet (Velvet v1.2.101). Putative Open Reading Frames (ORFs) were predicted using the GeneMarkS gene prediction program (Lukashin and Borodovsky, 1998). The Blast2Go and RAST servers (Conesa et al., 2005; Aziz et al., 2008) were used for functional annotation of each predicted protein. Reconstructed phage sequences were analyzed using PHAST and PHASTER tools (Zhou et al., 2011; Arndt et al., 2016). All phage proteins detected were manually annotated using the Protein BLAST (Kent, 2002), HHpred tools (Söding et al., 2005), and InterProScan tools (Zdobnov and Apweiler, 2001) and were found to display ≥50% protein homology. Genome sequences of the AUS531phi phage and Pf8_ST274-AUS411 filamentous phage were constructed with the assistance of CSAR-web (Chen and Lu, 2018) and RAST (Aziz et al., 2008).
The presence of the bci gene in prophages throughout the NCBI Nucleotide sequence was checked using BLAST, and its presence in a prophage was confirmed by PHASTER analysis of the bacterial genome. Protein domains of the protein were searched with CD-search in BLAST. Promoter regions were predicted with BPROM tool of SoftBerry2.
Isolation of Clinical Temperate Phages From the ST274 Clonal Complex (CC274)
An overnight culture of the clinical P. aeruginosa isolate AUS531 was diluted in Luria-Bertani (LB) medium and grown for 2.5 h until reaching an OD600 (optical density measured at a wavelength of 600 nm) of 0.6, before being treated with mitomycin C (MMC). MMC was added at a concentration of 10 μg/ml and the culture was incubated at 37°C and shaken at 180 rpm until the cells were lysed. The lysate was incubated in the presence of chloroform for 20 min and centrifuged at 3400 × g for 10 min. Finally, the supernatant was filtered through a 0.45 nm filter (Millipore).
Transmission Electron Microscopy (TEM) Examination of Temperate Phages: Inoviruses and Siphoviruses
Concentrated phage preparations were required for transmission electron microscopy (TEM). Phage particles were precipitated overnight at 4°C with polyethylene glycol (PEG6000) 3∼5% (w/v) and 0.5 M NaCl. The solution was centrifuged at 11000 × g at 4°C for 15 min. The pellet was resuspended with SM buffer (100 mM NaCl; 8 mM MgSO4 7H2O; 50 mM Tris–HCl pH 7.5) and stored at 4°C. Samples were negatively stained with 1% aqueous uranyl acetate before examination by electron microscopy (Hargreaves et al., 2013).
Characterization of Siphovirus Temperate Phage in Relation to Quorum Sensing
bci Deleted Strain and Phage Isolation
To obtain a strain without the bci gene for experiments and to subsequently obtain the AUS531phiΔbci mutant phage, the bci gene was amplified with 1 kb upstream and downstream regions for deletion in the P. aeruginosa AUS531 isolate. The fragment was cloned into the pEX18Gm vector (GenBank: AF047518.1) (Hoang et al., 1998) using the UP_bci(KpnI)/UP_Bci(XhoI) combination of primers for the upstream region and the DOWN_Bci(XhoI)/DOWN_Bci(BamHI) combination for the downstream region (Table 1). Fragments were digested with KpnI and XhoI restriction enzymes (upstream fragment) and XhoI and BamHI (downstream region). Products were ligated into the pEX18Gm plasmid, and the recombinant plasmid was transformed in E. coli TG1 by electroporation.
The resulting plasmid was used to transform the P. aeruginosa AUS531 isolate by electroporation for genomic recombination and resulting gene knockout. Recombinant colonies representing the first crossover event were obtained by gentamicin-mediated selection. Gentamicin-resistant colonies were grown overnight in LB supplemented with 15% sucrose, and they were then plated on the same medium. Secondary crossover events were confirmed by PCR and by sequencing with the primers listed in Table 1. The AUS531phiΔbci phage was obtained from the mutant AUS531Δbci strain by induction with MMC, as previously described.
Expression of the bci Gene in Relation to QS Genes by RT-PCR
To establish the relationship between bci gene and QS, we measured the bci gene expression in the AUS531 strain incubated in the presence of QS signals. One colony of each of P. aeruginosa isolates AUS531 and AUS531Δbci was inoculated in LB broth and incubated overnight at 37°C under stirring at 180 rpm. The overnight culture was diluted (1:100) and allowed to grow until reaching an OD600 of 0.3. Aliquots of 10 μL of QS-system signals 3-Oxo-C12-HSL (Stacy et al., 2012; López et al., 2017) and N-butanoyl-L-HSL (C4-HSL, which regulates through QS) and the same volume of DMSO as used in controls were added. The samples were incubated for 1 h (Karig and Weiss, 2005; Dubeau et al., 2009; Zhang et al., 2013). RNA was extracted using the High Pure RNA Isolation kit (Roche, Germany), and the extract was treated with DNAse (Roche, Germany). The extracted RNA measured was in a NanoDrop ND-100 spectrophotometer (NanoDrop Technologies). The concentration of the samples was adjusted to 50 ng/μL to yield efficiencies of 90–110% (Rumbo et al., 2013). The expression studies were carried out with Lightcycler 480 RNA Master Hydrolysis Probe (Roche, Germany), under the following conditions: reverse transcription at 63°C for 3 min, denaturation at 95°C for 30 s, followed by 45 cycles of 15 s at 95°C and 45 s at 60°C and, finally, cooling at 40°C for 30 s. In all of the experiments, the final volume was 20 μL per well (18 μL of master mix and 2 μL of RNA at 50 ng/μL). Primers and the respective Universal Probe Library (UPL) probes are listed in Table 1. For each isolate, expression of all genes, primers and probes was normalized relative to the reference or housekeeping gene, proC (Savli et al., 2003). All of the samples were analyzed in triplicate. Statistically significant differences were determined by Student’s t-test (GraphPad Prism v.6).
In order to analyze the effect of the phage with and without bci gene on QS, we analyzed the expression of QS genes (lasR, rhlR, qscR, and pqsR) in AUS531Δbci incubated for 30 min with AUS531phi and AUS531phiΔbci phages in an early step of bacterial growth. An overnight culture was diluted (1:100) in LB broth with 10 mM MgSO4 and 10 mM CaCl2 and then grown until reaching an OD600 of 0.2–0.4. Both wild type AUS531phi and AUS531phiΔbci phages were added at a multiplicity of infection (MOI) of 10. All controls were prepared by adding the same volume of phage buffer. RNA extraction and expression studies were carried out in the same way as in the previous step. All of the samples were analyzed in triplicate. Statistically significant differences were determined by Student’s t-test.
Effect of the bci Gene Interaction Carried by Bacteriophage on the QS: Infective Capacity, Biofilm Production, Bacterial Motility and Pyocyanin Secretion
To characterize the infection curve for the bacteriophages, an overnight culture of P. aeruginosa AUS531Δbci was diluted 1:100 in LB broth supplemented with MgSO4 and CaCl2 (both at a concentration of 10 mM). The mixture was incubated at 37°C at 180 rpm until reaching an OD600 nm of 0.1, before being infected with the phage AUS531phi and with the phage AUS531phiΔbci at a MOI of 1 and 10. Measurements were made during 6 h at 1-h intervals. Statistically significant differences were determined by Student’s t-test (GraphPad Prism v.6) by comparing the data obtained every hour.
To study the effect on bacterial motility, an overnight culture of P. aeruginosa AUS531Δbci was diluted 1:100 in LB broth with 10 mM MgSO4 and 10 mM CaCl2 until reaching an OD600 of 0.5∼0.6. A spot of 1 μL of a mixture of AUS531Δbci culture and each phage (wild type AUS531phi and mutant AUS531phiΔbci at a MOI of 1) was placed in plates containing LB medium and 0.3% agar supplemented with 10 mM MgSO4 and 10 mM CaCl2 (Clemmer et al., 2011).
To study the effect on biofilm production, we used the modified version of the biofilm formation assay (O’Toole, 2011). Briefly, an overnight culture of P. aeruginosa AUS531Δbci was adjusted to 107 CFU/mL in LB broth supplemented with 10 mM MgSO4, 10 mM CaCl2 and 2% glucose, and 100 μL was finally added to each well of a “U”-bottom 96-well microtiter plate and incubated at 37°C for 24 h. Thirty wells were infected at MOI 10 with AUS531phi wild temperate phage and the other 30 with AUS531Δbci mutant strain. Planktonic cell growth was measured at OD600 before being removed. The cells were rinsed three times with distilled water and then fixed at 60° for 1 h. Biofilms were stained with 125 μL of 0.4% crystal violet (CV) for 15 min, washed four times with distilled water, and the CV retained was solubilized with 125 μL of 30% acetic acid and measured at OD595. Biofilm production was calculated by dividing the OD595 of the CV-stained culture by the OD600 of the growth for each well. Statistical differences were determined with a Student’s t-test. In order to confirm integration of the temperate phages, the presence of the bci gene was checked by PCR in 10 isolated colonies in each biofilm assay.
To analyze pyocyanin secretion, an overnight culture of P. aeruginosa AUS531Δbci was diluted 1:100 in 10 mL of LB broth enriched with 10 mM MgSO4 and 10 mM CaCl2 and then grown until an OD600 of 0.2 was reached. The culture was then infected with phages AUS531phi and AUS531phiΔbci at 10 MOI and incubated for 6 h. The pyocyanin was extracted by adding 6 mL of chloroform to the culture and incubating the solution for 2 h at 37°C under continuous stirring at 180 rpm. Two mL of 0.2 N HCl was then added to yield a pink to deep red solution. The absorbance of this solution was measured at an OD of 520 nm. The concentrations, expressed as micrograms of pyocyanin produced per milliliter of culture supernatant (μg/mL), were determined by multiplying the optical density at 520 nm by 17.072 (Essar et al., 1990; Clemmer et al., 2011). Statistically significant differences were determined by Student’s t-test (GraphPad Prism v.6).
Results
Analysis of QS Network and Temperate Phages in the Genome of P. aeruginosa CF Clone (ST274-CC274) Isolates
We performed a genomic analysis of the 24 P. aeruginosa isolates belonging to the ST274 clonal complex (CC274) obtained from CF patients and of the reference P. aeruginosa PAO1 strain genome (GenBank: AE004091.2) (Table 2). Complete prophage sequences were present in three strains in the P. aeruginosa sequences: AUS411, AUS531, and FQSE15-1110 (Table 2). Three of these showed high similarity to the Pseudomonas Pf inovirus, constituted by 9-15 proteins in isolates AUS411, AUS531, and FQSE15-1110. The inoviruses present in isolates AUS531 and FQSE15 were similar to the Pseudomonas Pf4 and Pf5 inoviruses, but the prophage detected in AUS411 was a new phage, designated Pf8_ST274-AUS411 (hereinafter referred to as Pf8) (Hay and Lithgow, 2019; Li et al., 2019). The genome of the Pf8 filamentous phage is of size 10 Kb and has a total of 16 proteins and one tRNA coding region (Genbank:MN710383). It has a GC content of 58.1%. Interestingly, Pf8 showed high protein identity with the filamentous bacteriophages Pf4 (P. aeruginosa PAO1) and Pf5 (P. aeruginosa PA14) (Mooij et al., 2007) (Figure 1A). However, new proteins involved in viral defense were identified in the Pf8 bacteriophage, including a putative toxin-antitoxin module (Genbank: QGZ15329.1 and QGZ15330.1) and methyltransferase (Genbank: QGZ15339.1). The prophage designated AUS531phi (accession number MN585195), detected in isolate AUS531 was found to be homologous with the Pseudomonas Phi297 bacteriophage.
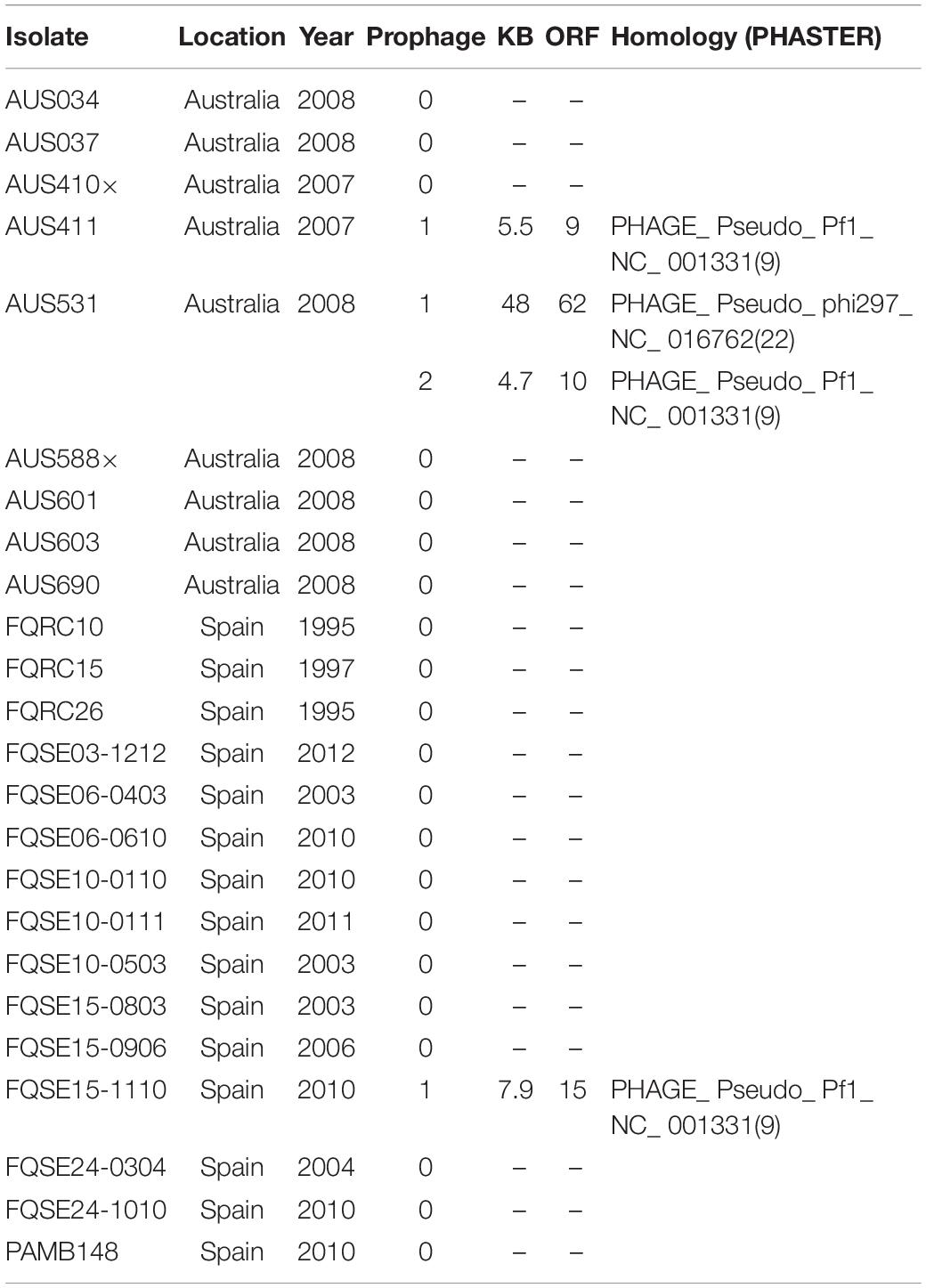
Table 2. Cystic Fibrosis clone isolates in the study (ST274-CC274) and their complete prophage presence.
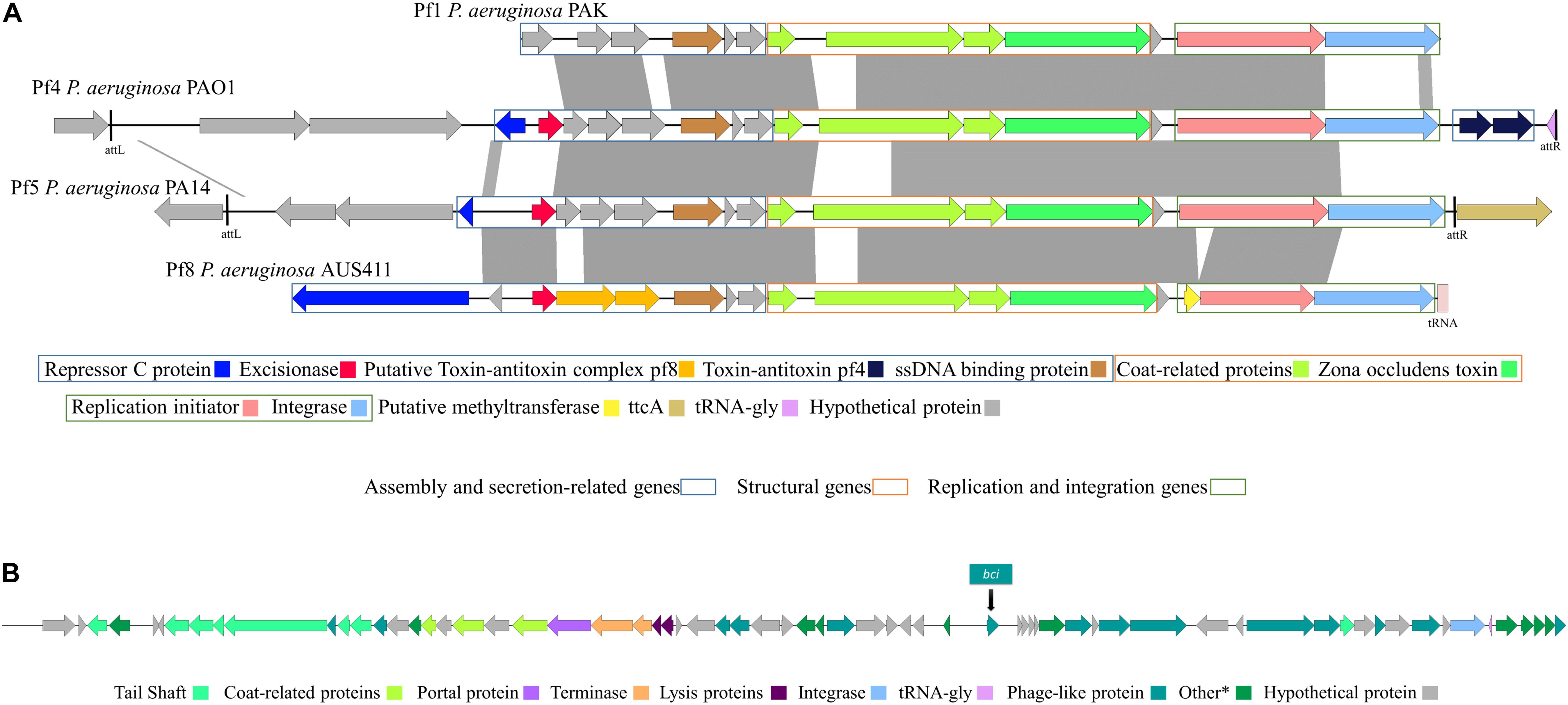
Figure 1. (A) Schematic representation and comparison of filamentous phages Pf1, Pf4, Pf5, and Pf8. Genes are classified by function into assembly and secretion, structural, and replication/integration genes. Dark gray regions represent >90% of nucleotide sequence identity between Pf genome regions. (B) Schematic representation of the genome of siphovirus phage AUS531phi and position of the Bci protein (GenBank: MN585195.1).
The genome of the AUS531phi prophage is almost 50 Kb in size and contains a total of 66 proteins, one tRNA coding region and 63% GC content. The genome of the AUS531phi (Figure 1B) carries prophage assembly proteins, such as tail shaft proteins (GenBank: QGF21321.1, QGF21325.1, QGF21326.1, QGF21327.1, QGF21328.1, QGF21330.1, QGF21331.1, and QGF21373.1), coat-related proteins (GenBank: QGF21339.1, QGF21337.1, and QGF21335.1), a portal protein (GenBank: QGF21340.1), terminase proteins (GenBank: QGF21341.1 and QGF21342.1), lysis proteins (GenBank:QGF21343.1 and QGF21344.1), an integrase (GenBank: QGF21379.1) and other phage-related proteins. The genome harbors a carbon storage regulator (Genbank: QGF21359.1) (QS regulator associated with biofilm inhibition), called Bci protein (Figure 1B). The bci gene has 372 bp and the Bci protein has 123 amino acids (Supplementary Figures S1A,B, respectively) with a promoter region in the upstream sequence between the nucleotides 30327 and 30372 (Figure S1C) (GenBank: MN585195). There is a putative rhl-las box with a motif CT-(N13)-AG between the nucleotides 30342 and 30358 (Figure S1C and Supplementary Material). Following a CD-search in BLAST, CsrA superfamily domain is present between amino acids 1 and 51 with an e-value of 2.77e-27. We analyzed the distribution of the bci gene among the P. aeruginosa genomes deposited in the NCBI database (Table 3). We found that this gene was present in 33 different P. aeruginosa strains, with high homology (>95% of protein homology in most of these sequences). Furthermore, we found (using the PHASTER search tool) that the bci gene was only present in prophage sequences in these strains (Table 3). Moreover, in 6 of the isolates, the DNA region in this gene showed high homology (>95%) with repeat sequences of previously characterized systems (Table 3), showing that the bci gene present in phages as CRISPR sequences are constructed with previously infected DNA bacteriophage fragments (Cady et al., 2011).
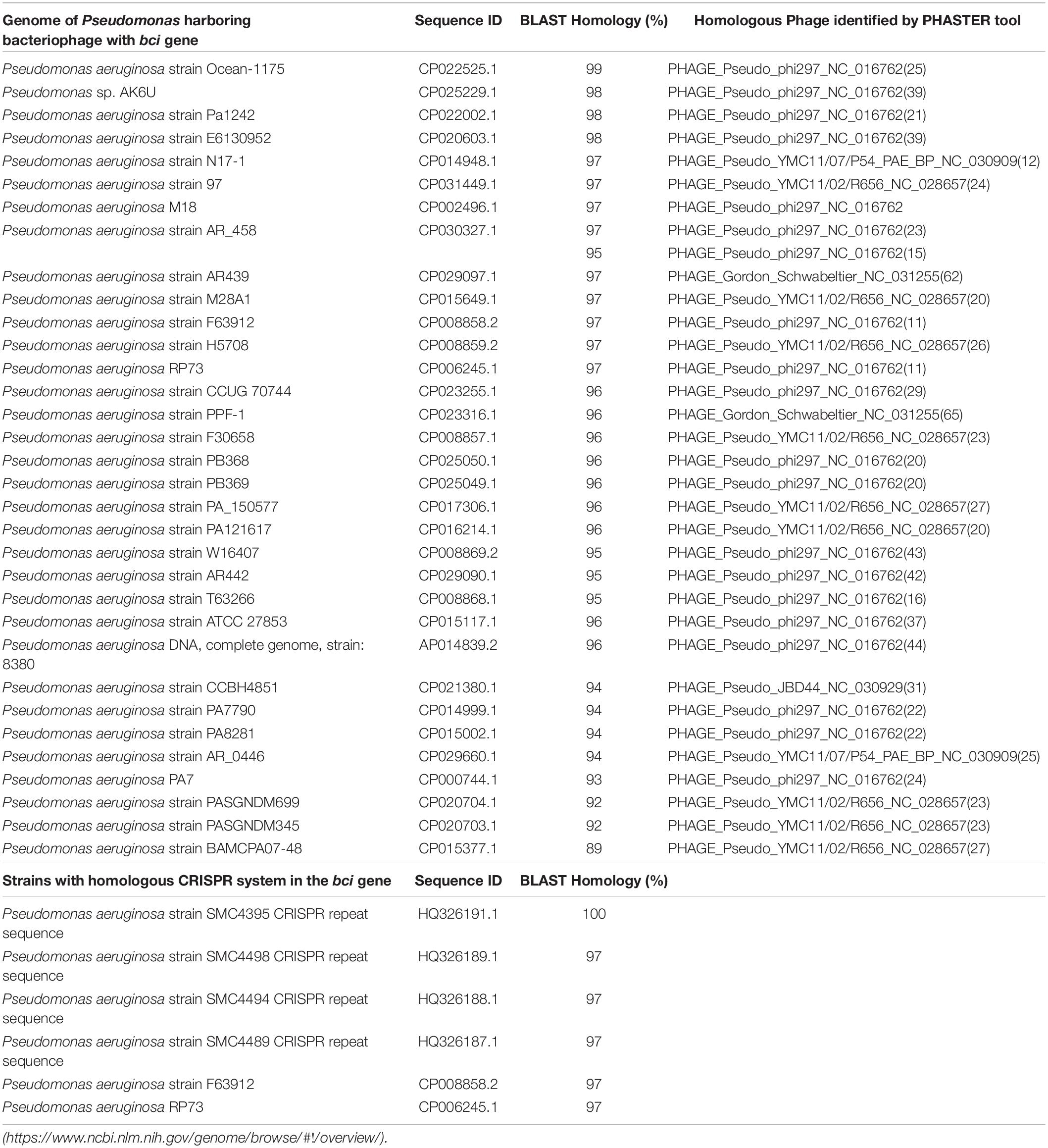
Table 3. Genomes of the P. aeruginosa isolates carrying bacteriophages with the bci gene and homologous CRISPR system in the bci gene.
TEM Micrographs of Bacteriophages
We confirmed the presence of Inoviridae type phages by TEM examination of extracts of overnight supernatant cultures of P. aeruginosa isolate AUS411 (Figure 2A). Moreover, we confirmed the presence of the Siphoviridae type phages AUS531phi and AUS531phiΔbci by TEM examination of the preparations (Figure 2B). The morphology of the structures seen in the pictures is clearly that of Siphoviridae type phages (Alič et al., 2017).
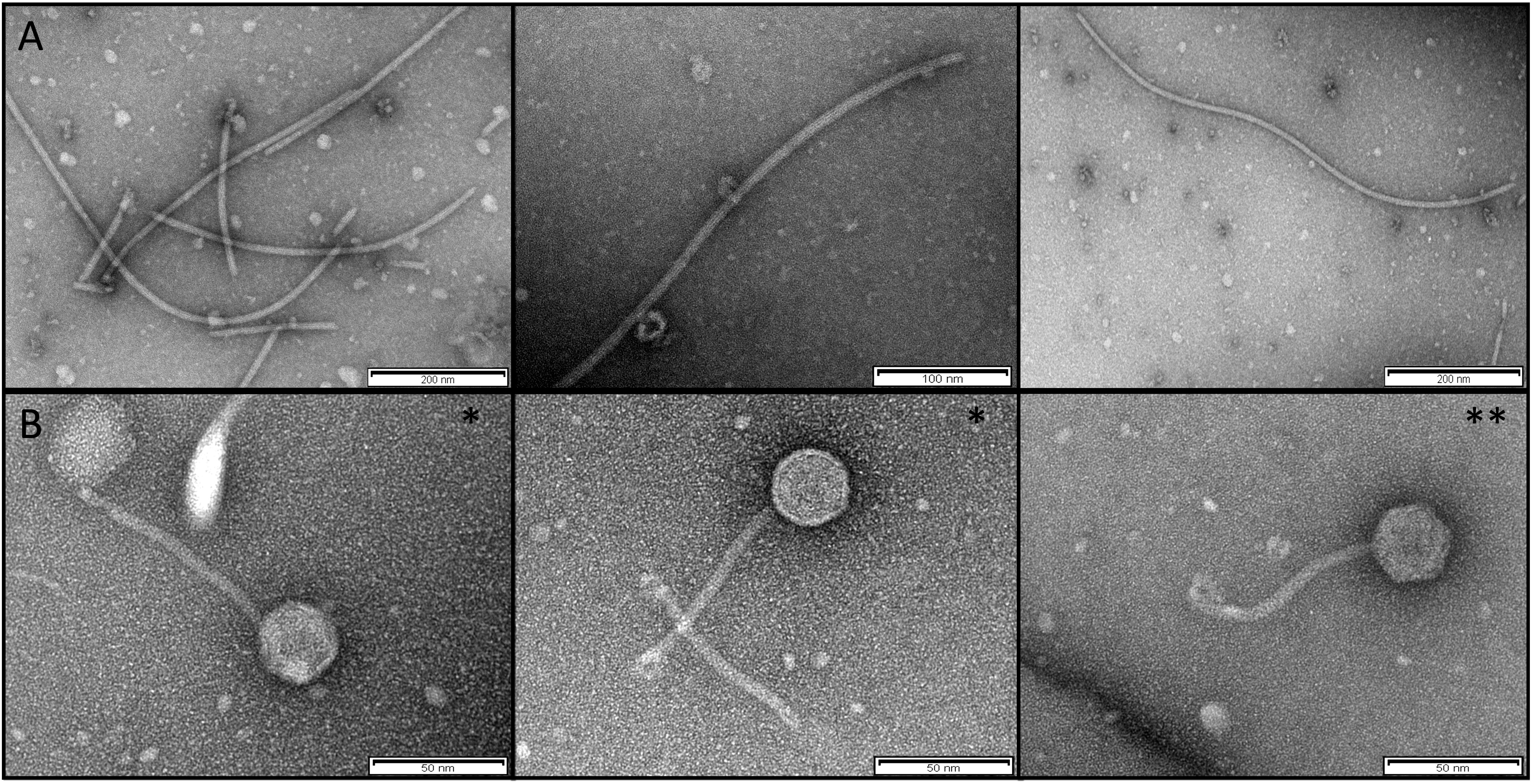
Figure 2. (A) TEM of the Inoviridae Pf8 bacteriophage of P. aeruginosa clinical isolate AUS411 (Pf8_ST274-AUS411). (B) TEM of Siphoviridae bacteriophage of P. aeruginosa clinical isolates AUS531 (AUS531phi*) and AUS531Δbci (AUS531Δbciphi**).
Relationship Between the Bacteriophages and the QS System
Gene Expression
We observed an increase in the expression of the bci gene in the prophage region in the presence of two acyl-homoserine lactone QS inducers: 3-oxo-C12-HSL and C4-HSL (Figure 3), demonstrating that the bci gene is associated with the QS system.
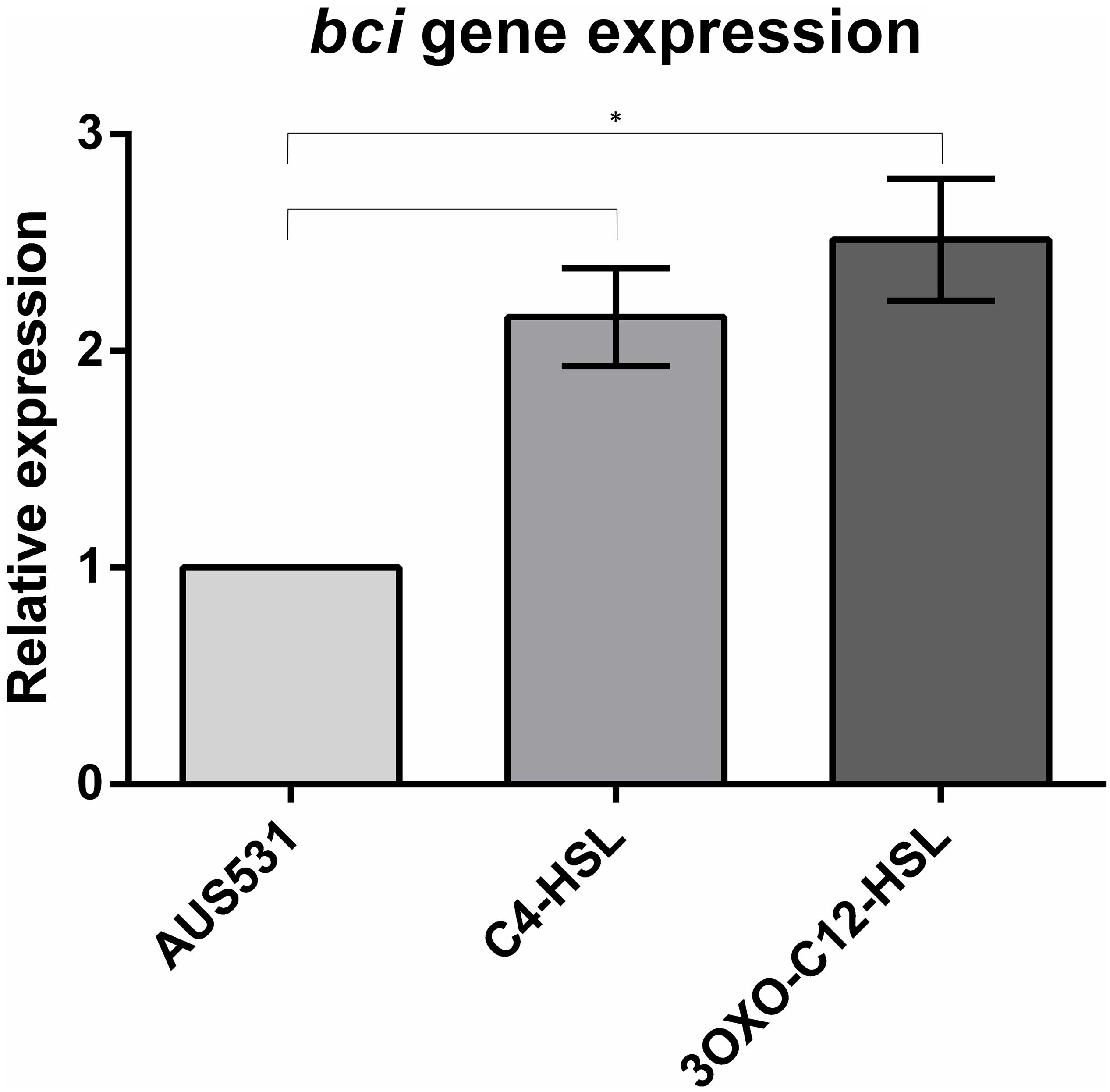
Figure 3. Relative expression of the bci gene in AUS531 isolate under the quorum sensing inducers C4-HSL and 3OXO-C12-HSL. Standard deviations are indicated. (∗) Statistically significant differences (p < 0.05) were determined by Student’s t-test (GraphPad Prism v.6).
In addition, we studied the effect of infection by bacteriophages AUS531phi and AUS531phiΔbci in the expression of the genes lasR, rhlR, qscR, and pqsR of the QS in AUS531Δbci bacterial strain to check the effect of the gene in the first step of bacteriophage infection (Figure 4). The graphic representation shows a fold change of around 5.0 for lasR, lhlR, and qscR when isolate AUS531Δbci was infected with the mutant phage AUS531phiΔbci, while infection with the wild phage AUS531phi yielded fold changes of around 1.0 in these genes. The differences in the expression for infection with a phage containing a bci gene and in the absence of this gene suggest that these genes are involved in regulating the QS system in order to overcome it and infect the bacteria. Interestingly, there was a fold change of around 13.0 in the pqsR gene when isolate AUS531Δbci was infected with phage AUS531phiΔbci, in contrast to a fold change of around 1.5 when the isolate was infected with the wild phage AUS531phi.
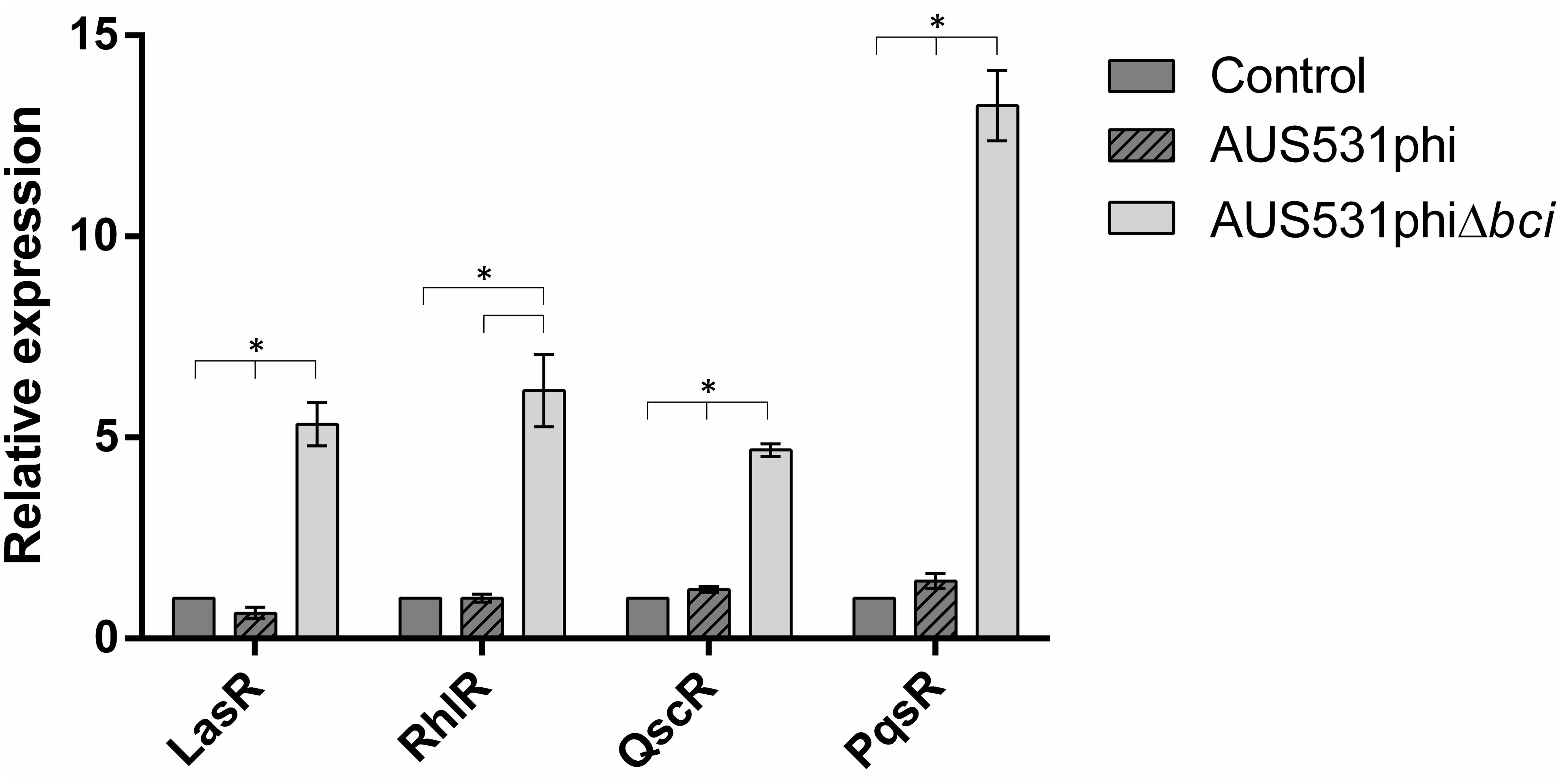
Figure 4. Relative expression of quorum sensing-related genes of the AUS531Δbci isolate infected with AUS531phi and AUS531phiΔbci bacteriophages. Standard deviations are indicated. (∗) Statistically significant differences (p < 0.05) were determined by Student’s t-test (GraphPad Prism v.6).
Infection Curve
The relationship between the bci gene and the ability of the phage to infect the host was demonstrated in the infection curves. The host isolate AUS531Δbci grew less when infected with the wild type phage AUS531phi than when it was infected with the mutated phage, AUS531phiΔbci at all the MOI assayed (Figure 5). The infection curves for phage AUS531phi were significantly different (p < 0.05) at MOI 0.1, 1 and also at MOI 10 (p < 0.0001). In addition, the infection curves for phage AUS531phi were significantly different from the corresponding control curves at MOI 1 and 10 (p < 0.05), but not at MOI 0.1. Comparison of the growth of the culture infected with the wild type phage AUS531phi and the mutant phage AUS531phiΔbci revealed significant differences at MOI 0.1 and 1 (p < 0.05) (Figures 5A,B) at all time points measured, and at MOI10 the differences were significant (p < 0.0001) (Figure 5C) at 2, 3, and 4 h. These results indicate that capacity of infection of AUS531phi is higher than that of AUS531Δbci, thus confirming that the bci gene is related to the infection capacity of this phage.
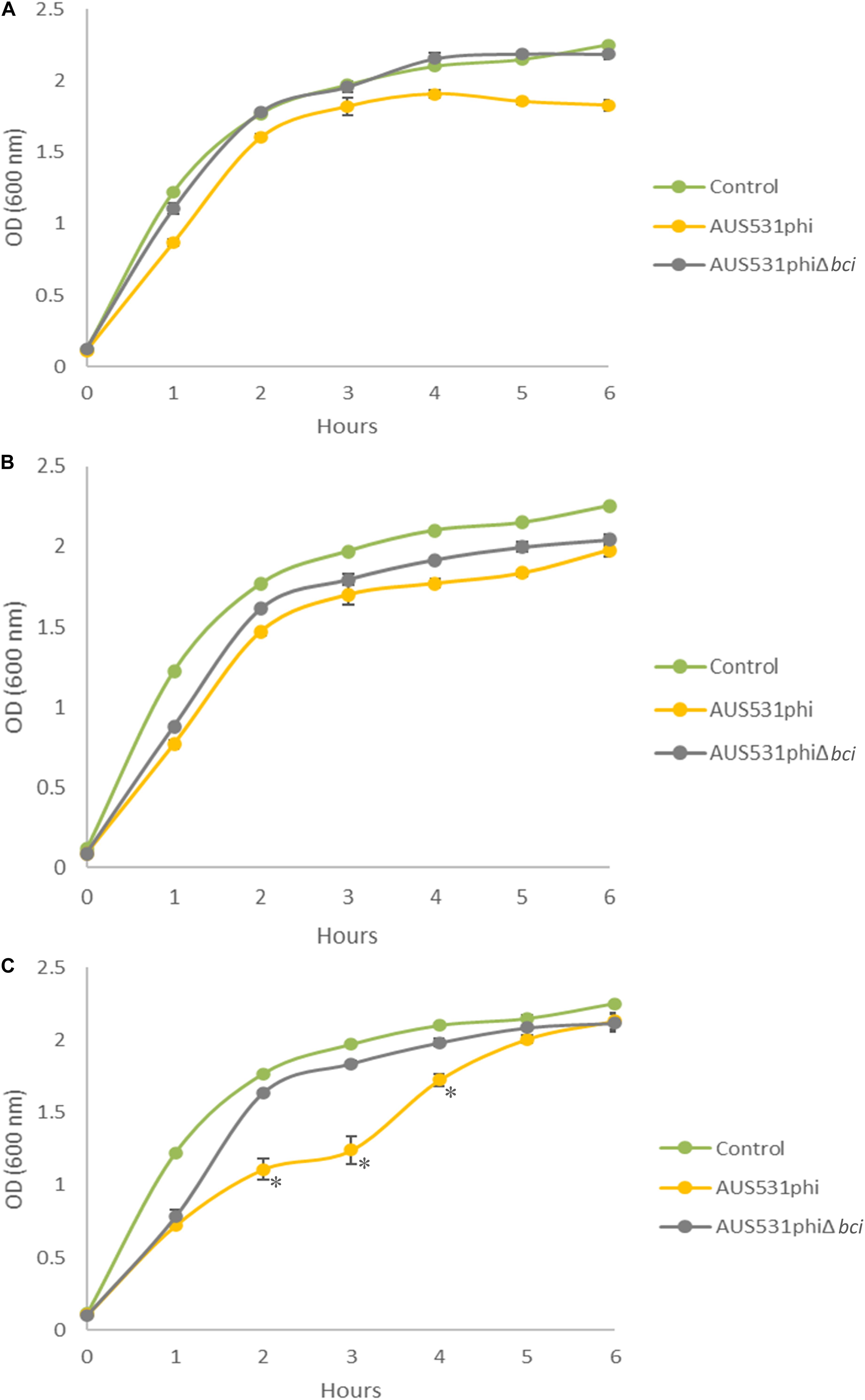
Figure 5. Infection curves for the lysogenic phages AUS531phi (A) and AUS531phiΔbci at MOIs 0.1 (A), 1 (B), and 10 (C) during 6 h. Standard deviations are indicated. Statistically significant differences were determined by Student’s t-test for each point on the curve (GraphPad Prism v.6). (*) indicates a strongly significant difference (p < 0.0001).
Relationship Between the Phage Infection and Virulence Factors: Motility, Biofilm and Pyocyanin Production
In order to verify the relationship between the bci gene from the bacteriophage and bacterial virulence, we performed motility, biofilm and pyocyanin assays. When isolate AUS531Δbci was infected with the wild type phage AUS531phi, a reduction in motility was observed. By contrast, when the same isolate was infected with the bci gene deleted from prophage AUS531phiΔbci, there was no difference in motility relative to the control (Figure 6A). When isolate AUS531Δbci was infected with the wild type phage AUS531phi, enhanced biofilm production was observed relative to the infection of mutant phage AUS531Δbci (Figure 6B). The PCR of the biofilm isolated colonies showed the presence of the bci gene in strain AUS531Δbci infected with the wild-type phage, thus confirming integration of this phage in the genome. Similarly, pyocyanin secretion was higher with the AUS531phi bacteriophage than with the AUS531phiΔbci bacteriophage (Figure 6C) confirming that the bci gene influences bacterial virulence.
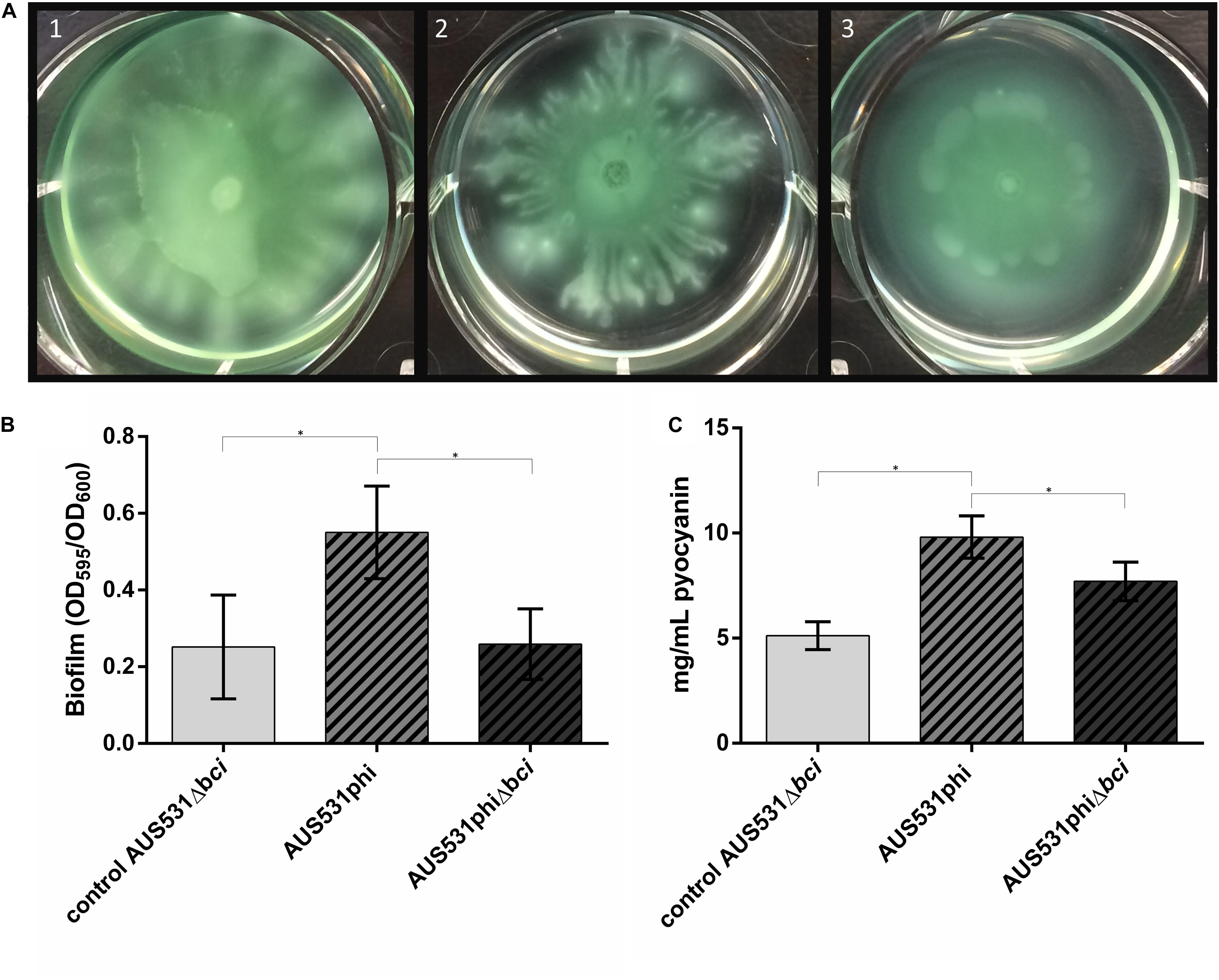
Figure 6. (A) Motility assay in AUS531Δbci under normal conditions (1), adding AUS531phi phage (2), and adding AUS531phiΔbci phage (3). (B) Biofilm production after 24 h of AUS531 wild type isolate, AUS531Δbci under normal conditions, AUS531Δbci in response to addition of the wild type AUS531phi phage and the mutant AUS531Δbci phage. (∗) Statistically significant differences were determined by Student’s t-test (GraphPad Prism v.6). (C) Pyocyanin production of controls AUS531 and AUS531Δbci and AUS531Δbci in response to infection with the wild-type strain.
Discussion
Cystic fibrosis is the main life-limiting recessive genetic disorder in the Caucasian population. It affects multiple organs, but is particularly damaging to the lungs. Colonization of the respiratory tract by some pathogens such as P. aeruginosa exacerbates the severity of the disease in CF patients (Rey et al., 2018).
Temperate bacteriophages of P. aeruginosa are involved in the horizontal transfer of DNA and show selective preference for developing and accumulating in the specific conditions of the lower lung (Tariq et al., 2015). Although most phages are pathogens that kill their bacterial hosts, filamentous phages live together with their host (Mai-Prochnow et al., 2015). Filamentous phages are widely distributed in Gram-negative bacteria and they have a strong impact on the physiology, adaptation and virulence of their host bacteria, with a high presence in P. aeruginosa biofilms (Rice et al., 2009; Secor et al., 2015).
The high-risk clone P. aeruginosa ST274 is one of the most prevalent clones in CF patients (Kidd et al., 2012). Genomic analysis shows the presence of complete prophage regions in 3 of the 24 isolates of the P. aeruginosa CC274 clone. Inovirus-type phages are present in three different isolates of this clone, two corresponding to previously described phages (Knezevic et al., 2015), and one, the pf8 phage present in the AUS411 isolate, which is a new type of Pf inovirus characterized by the presence of a putative toxin/antitoxin system and a methyltransferase. However, only the AUS531 isolate contains a complete siphovirus type phage, never previously described, the AUS531phi phage.
The Pf8 phage carries a putative novel type of toxin/antitoxin system (Mai-Prochnow et al., 2015), located between an excisionase (acc. no. QGZ15328.1). The genes that encode toxin-antitoxin systems are common in bacteria and are usually located adjacent to genes related to plasmids and other mobile genetic elements (DeShazer, 2004; Dziewit et al., 2007). In prophages preserve their genomes in bacterial hosts via the toxin/antitoxin system, giving them a selective advantage under different stress conditions (Wen et al., 2017).
Temperate bacteriophages can also drive host genome evolution through gene disruption, duplication, transduction or by acting as anchor points for major chromosomal rearrangements (Davies et al., 2016). Previous studies have demonstrated a possible relationship between QS signaling and regulation. The QS system is able to control anti-phage defense mechanisms, leading to lower susceptibility to phage infection in QS-proficient cells. In Vibrio anguillarum, QS downregulates expression of the ompK gene, thus increasing the resistance to phage KVP40 (Tan et al., 2015; Hoque et al., 2016). In E. coli, LamB phage receptors can shield isolates from attack by lytic bacteriophage λ (Høyland-Kroghsbo et al., 2013). However, bacteriophages infect bacteria with a functional QS, as in P. aeruginosa, because once the barrier to infection has been overcome it is advantageous for the phage to remain in the genome as a temperate phage improving cooperative behavior by eliminating QS-deficient social cheaters, which not have the phages, despite the fact that phage adsorption is higher in those with QS-deficient strain (Saucedo-Mora et al., 2017). In addition, molecular evolution of clinical strains of Acinetobacter baumannii has been demonstrated to have occurred between 2000 and 2010, leading to possession of a functional quorum network and the acquisition of bacteriophages (López et al., 2018).
The QS regulatory role of the bci in the prophage was demonstrated by the increase in the expression of this gene in presence of two acyl-homoserine lactone QS inducers, C4-HSL and 3oxo-C12-HSL, which activate the receptors RhlR and LasR, respectively and may induce the bci expression (Medina et al., 2003). In the promoter region of the gene, there is a putative rhl-las box (Subramoni et al., 2015), that have been predicted to be upstream QS-controlled genes (Whiteley et al., 1999). Also, when an infection with the wild prophage AUS531phi and with the mutant phage AUS531phiΔbci were done, the bacterial QS expression was regulated by the wild type phage, which suggest that the bci gene has a role in the control of the bacterial QS, favoring the infection by the temperate phages as was also observed in the infection curves.
Virulence factors as pyocyanin production, biofilm and motility are regulated by QS and also influenced by the phage infections (Morkunas et al., 2012; Hosseinidoust et al., 2013; Latino et al., 2014; Castañeda-Tamez et al., 2018; Tariq et al., 2019). The infection with the wild type phage, AUS531phi, carrying the bci gene, increased the production of virulence factors, pyocyanin and biofilm, whose presence is characteristic in the lung of CF patients (Castañeda-Tamez et al., 2018). The increase in both biofilm and pyocyanin and a reduction in the swarming motility, are a response to the phage infection which is higher when the bci gene is present, but also due to the integration of the temperate phage and the bci gene in the bacterial genome, as was described previously. Pyocyanin production has proven to be protective against oxidative stress environments for P. aeruginosa (Vinckx et al., 2010). The higher pyocyanin production may be due to a protective response to a higher infectivity capacity of the phage AUS531phi. Temperate phages could help P. aeruginosa select for bacterial characteristics that favor persistence of bacteria in the lung (Latino et al., 2014; Tariq et al., 2019). Thus, the bci gene may help clinical isolates of P. aeruginosa to survive in lung infections, increasing their chance of being infected by temperate phages.
In this research we identified two new prophages, Pf8 and AUS531phi, present in clinical P. aeruginosa strains of the CC274 clone, which cause infections in CF patients. Further research is required to determine the role of Pf8 inovirus bacteriophages (filamentous prophages) and their putative toxin/antitoxin system in chronic lung infections by P. aeruginosa. Also, we describe a new gene, bci (present in prophage AUS531phi), which is involved in regulating the bacterial QS system and favoring the infective capacity of the strain and therefore favoring the presence of this phage in the CF CC274 clone characterized by a low presence of prophages.
Data Availability Statement
The datasets presented in this study can be found in online repositories. The names of the repository/repositories and accession number(s) can be found below: https://www.ncbi.nlm.nih.gov/genbank/, (https://www.ncbi.nlm.nih.gov/nuccore/MN710383). https://www.ncbi.nlm.nih.gov/genbank/, (https://www.ncbi.nlm.nih.gov/nuccore/MN585195).
Ethics Statement
This study uses strains obtained from the work titled Clonal dissemination, emergence of mutator lineages and antibiotic resistance evolution in Pseudomonas aeruginosa cystic fibrosis chronic lung infection from PlosOne 2013 (PMID: 23951065) and Evolution of the Pseudomonas aeruginosa mutational resistome in an international Cystic Fibrosis clone published in Scientific Report 2017 (PMID: 28717172). Research Committee from A Coruña Hospital (Spain) which president is Maria Tomas, confirm it did not require the study to be reviewed or approved by an Ethics Committee because this collection of strains was previously published and in this work is not included clinical data from patients.
Author Contributions
AA, LB, CL-C, RT, LF-G, IB, MP-A, OP, and ML conducted the experiments, analyzed the results, and wrote the manuscript. RC and TK revised the results. GB and AO revised the manuscript. MT obtained the research funding, directed the experiments, and supervised the writing of the manuscript. All authors contributed to the article and approved the submitted version.
Conflict of Interest
The authors declare that the research was conducted in the absence of any commercial or financial relationships that could be construed as a potential conflict of interest.
Funding
This study was funded by grants PI16/01163 and PI19/00878 awarded to MT within the State Plan for R + D + I 2013-2016 (National Plan for Scientific Research, Technological Development and Innovation 2008-2011) and co-financed by the ISCIII-Deputy General Directorate for Evaluation and Promotion of Research – European Regional Development Fund “A way of Making Europe” and Instituto de Salud Carlos III FEDER, Spanish Network for the Research in Infectious Diseases (REIPI, RD16/0016/0004, RD16/0016/0006, and RD16/0016/0011) and by the Study Group on Mechanisms of Action and Resistance to Antimicrobials, GEMARA (SEIMC, http://www.seimc.org/). MT was financially supported by the Miguel Servet Research Programme (SERGAS and ISCIII). RT and LF-G were financially supported by respectively a SEIMC grant and predoctoral fellowship from the Xunta de Galicia (GAIN, Axencia de Innovación). TK was supported by a National Health and Medical Research Council Early Career Fellowship (GNT1088448).
Supplementary Material
The Supplementary Material for this article can be found online at: https://www.frontiersin.org/articles/10.3389/fmicb.2020.556706/full#supplementary-material
Footnotes
References
Alič, Š, Naglič, T., Tušek-Žnidariè, M., Ravnikar, M., Raèki, N., Peterka, M., et al. (2017). Newly Isolated Bacteriophages from the Podoviridae, Siphoviridae, and Myoviridae Families Have Variable Effects on Putative Novel Dickeya spp. Front Microbiol 8:1870. doi: 10.3389/fmicb.2017.01870
Arndt, D., Grant, J. R., Marcu, A., Sajed, T., Pon, A., Liang, Y., et al. (2016). PHASTER: a better, faster version of the PHAST phage search tool. Nucleic Acids Res. 07, W16–W21. doi: 10.1093/nar/gkw387
Aziz, R. K., Bartels, D., Best, A. A., DeJongh, M., Disz, T., Edwards, R. A., et al. (2008). The RAST Server: rapid annotations using subsystems technology. BMC Genomics. Feb 9:75. doi: 10.1186/1471-2164-9-75
Bjarnsholt, T., and Givskov, M. (2007). Quorum-sensing blockade as a strategy for enhancing host defences against bacterial pathogens. Philos Trans R Soc Lond B Biol Sci. Jul 362, 1213–1222. doi: 10.1098/rstb.2007.2046
Cady, K. C., White, A. S., Hammond, J. H., Abendroth, M. D., Karthikeyan, R. S., Lalitha, P., et al. (2011). Prevalence, conservation and functional analysis of Yersinia and Escherichia CRISPR regions in clinical Pseudomonas aeruginosa isolates. Microbiology. Feb 157, 430–437. doi: 10.1099/mic.0.045732-0
Castañeda-Tamez, P., Ramírez-Peris, J., Pérez-Velázquez, J., Kuttler, C., Jalalimanesh, A., Saucedo-Mora, M. Á., et al. (2018). Pyocyanin restricts social cheating in Pseudomonas aeruginosa. Front. Microbiol. 9:1348. doi: 10.3389/fmicb.2018.01348
Chen, K. T., and Lu, C. L. (2018). CSAR-web: a web server of contig scaffolding using algebraic rearrangements. Nucleic Acids Res. 07, W55–W59. doi: 10.1093/nar/gky337
Clemmer, K. M., Bonomo, R. A., and Rather, P. N. (2011). Genetic analysis of surface motility in Acinetobacter baumannii. Microbiology. Sep 157, 2534–2544. doi: 10.1099/mic.0.049791-0
Clokie, M. R., Millard, A. D., Letarov, A. V., and Heaphy, S. (2011). Phages in nature. Bacteriophage. Jan 1, 31–45. doi: 10.4161/bact.1.1.14942
Conesa, A., Götz, S., García-Gómez, J. M., Terol, J., Talón, M., and Robles, M. (2005). Blast2GO: a universal tool for annotation, visualization and analysis in functional genomics research. Bioinformatics. Sep 21, 3674–3676. doi: 10.1093/bioinformatics/bti610
Davies, E. V., Winstanley, C., Fothergill, J. L., and James, C. E. (2016). The role of temperate bacteriophages in bacterial infection. FEMS Microbiol Lett. Mar 363, fnw015. doi: 10.1093/femsle/fnw015
DeShazer, D. (2004). Genomic diversity of Burkholderia pseudomallei clinical isolates: subtractive hybridization reveals a Burkholderia mallei-specific prophage in B. pseudomallei 1026b. J Bacteriol. Jun 186, 3938–3950. doi: 10.1128/JB.186.12.3938-
Dubeau, D., Déziel, E., Woods, D. E., and Lépine, F. (2009). Burkholderia thailandensis harbors two identical rhl gene clusters responsible for the biosynthesis of rhamnolipids. BMC Microbiol. Dec 9:263. doi: 10.1186/1471-2180-9-263
Dziewit, L., Jazurek, M., Drewniak, L., Baj, J., and Bartosik, D. (2007). The SXT conjugative element and linear prophage N15 encode toxin-antitoxin-stabilizing systems homologous to the tad-ata module of the Paracoccus aminophilus plasmid pAMI2. J Bacteriol. Mar 189, 1983–1997. doi: 10.1128/JB.01610-06
Essar, D. W., Eberly, L., Hadero, A., and Crawford, I. P. (1990). Identification and characterization of genes for a second anthranilate synthase in Pseudomonas aeruginosa: interchangeability of the two anthranilate synthases and evolutionary implications. J Bacteriol. Feb 172, 884–900. doi: 10.1128/jb.172.2.884-900.1990
Finnan, S., Morrissey, J. P., O’Gara, F., and Boyd, E. F. (2004). Genome diversity of Pseudomonas aeruginosa isolates from cystic fibrosis patients and the hospital environment. J Clin Microbiol. Dec 42, 5783–5792. doi: 10.1128/JCM.42.12.5783-5792.2004
Fothergill, J. L., Mowat, E., Walshaw, M. J., Ledson, M. J., James, C. E., and Winstanley, C. (2011). Effect of antibiotic treatment on bacteriophage production by a cystic fibrosis epidemic strain of Pseudomonas aeruginosa. Antimicrob Agents Chemother. Jan 55, 426–428. doi: 10.1128/AAC.01257-10
Fothergill, J. L., Walshaw, M. J., and Winstanley, C. (2012). Transmissible strains of Pseudomonas aeruginosa in cystic fibrosis lung infections. Eur Respir J. Jul 40, 227–238. doi: 10.1183/09031936.00204411
Hargreaves, K. R., Colvin, H. V., Patel, K. V., Clokie, J. J., and Clokie, M. R. (2013). Genetically diverse Clostridium difficile strains harboring abundant prophages in an estuarine environment. Appl Environ Microbiol. Oct 79, 6236–6243. doi: 10.1128/AEM.01849-13
Hay, I. D., and Lithgow, T. (2019). Filamentous phages: masters of a microbial sharing economy. EMBO Rep. 06, 20. doi: 10.15252/embr.201847427
Hoang, T. T., Karkhoff-Schweizer, R. R., Kutchma, A. J., and Schweizer, H. P. (1998). A broad-host-range Flp-FRT recombination system for site-specific excision of chromosomally-located DNA sequences: application for isolation of unmarked Pseudomonas aeruginosa mutants. Gene. May 212, 77–86. doi: 10.1016/s0378-1119(98)00130-9
Hoque, M. M., Naser, I. B., Bari, S. M., Zhu, J., Mekalanos, J. J., and Faruque, S. M. (2016). Quorum Regulated Resistance of Vibrio cholerae against Environmental Bacteriophages. Sci Rep. 11, 37956. doi: 10.1038/srep37956
Hosseinidoust, Z., van de Ven, T. G. M., and Tufenkji, N. (2013). Evolution of Pseudomonas aeruginosa virulence as a result of phage predation. Appl. Environ. Microbiol. 79, 6110–6116. doi: 10.1128/AEM.01421-13
Høyland-Kroghsbo, N. M., Maerkedahl, R. B., and Svenningsen, S. L. (2013). A quorum-sensing-induced bacteriophage defense mechanism. MBio. Feb 4, e362–e312. doi: 10.1128/mBio.00362-12
Karig, D., and Weiss, R. (2005). Signal-amplifying genetic circuit enables in vivo observation of weak promoter activation in the Rhl quorum sensing system. Biotechnol Bioeng. Mar 89, 709–718. doi: 10.1002/bit.20371
Kent, W. J. (2002). BLAT–the BLAST-like alignment tool. Genome Res. Apr 12, 656–664. doi: 10.1101/gr.229202
Kidd, T. J., Ritchie, S. R., Ramsay, K. A., Grimwood, K., Bell, S. C., and Rainey, P. B. (2012). Pseudomonas aeruginosa exhibits frequent recombination, but only a limited association between genotype and ecological setting. PLoS One. 7:e44199. doi: 10.1371/journal.pone.0044199
Kirov, S. M., Webb, J. S., O’May, C. Y., Reid, D. W., Woo, J. K. K., Rice, S. A., et al. (2007). Biofilm differentiation and dispersal in mucoid Pseudomonas aeruginosa isolates from patients with cystic fibrosis. Microbiology. Oct 153, 3264–3274. doi: 10.1099/mic.0.2007/009092-0
Knezevic, P., Voet, M., and Lavigne, R. (2015). Prevalence of Pf1-like (pro)phage genetic elements among Pseudomonas aeruginosa isolates. Virology. Sep 483, 64–71. doi: 10.1016/j.virol.2015.04.008
Latino, L., Essoh, C., Blouin, Y., Vu Thien, H., and Pourcel, C. (2014). A novel Pseudomonas aeruginosa bacteriophage, Ab31, a chimera formed from temperate phage PAJU2 and P. putida lytic phage AF: characteristics and mechanism of bacterial resistance. PLoS One 9:e93777. doi: 10.1371/journal.pone.0093777
Lee, J., and Zhang, L. (2015). The hierarchy quorum sensing network in Pseudomonas aeruginosa. Protein Cell. Jan 6, 26–41. doi: 10.1007/s13238-014-0100-x
Li, Y., Liu, X., Tang, K., Wang, P., Zeng, Z., Guo, Y., et al. (2019). Excisionase in Pf filamentous prophage controls lysis-lysogeny decision-making in Pseudomonas aeruginosa. Mol Microbiol 111, 495–513. doi: 10.1111/mmi.14170
López, M., Mayer, C., Fernández-García, L., Blasco, L., Muras, A., Ruiz, F. M., et al. (2017). Quorum sensing network in clinical strains of A. baumannii: AidA is a new quorum quenching enzyme. PLoS One 12:e0174454. doi: 10.1371/journal.pone.0174454
López, M., Rueda, A., Florido, J. P., Blasco, L., Fernández-García, L., Trastoy, R., et al. (2018). Evolution of the Quorum network and the mobilome (plasmids and bacteriophages) in clinical strains of Acinetobacter baumannii during a decade. Sci Rep 8, 2523. doi: 10.1038/s41598-018-20847-7
López-Causapé, C., Sommer, L. M., Cabot, G., Rubio, R., Ocampo-Sosa, A. A., Johansen, H. K., et al. (2017). Evolution of the Pseudomonas aeruginosa mutational resistome in an international Cystic Fibrosis clone. Sci Rep. Jul 7, 5555. doi: 10.1038/s41598-017-05621-5
Lukashin, A. V., and Borodovsky, M. (1998). GeneMark.hmm: new solutions for gene finding. Nucleic Acids Res. Feb 26, 1107–1115. doi: 10.1093/nar/26.4.1107
Mai-Prochnow, A., Hui, J. G., Kjelleberg, S., Rakonjac, J., McDougald, D., and Rice, S. A. (2015). Big things in small packages: the genetics of filamentous phage and effects on fitness of their host. FEMS Microbiol Rev. Jul 39, 465–487. doi: 10.1093/femsre/fuu007
Manos, J., Arthur, J., Rose, B., Tingpej, P., Fung, C., Curtis, M., et al. (2008). Transcriptome analyses and biofilm-forming characteristics of a clonal Pseudomonas aeruginosa from the cystic fibrosis lung. J Med Microbiol. Dec 57, 1454–1465. doi: 10.1099/jmm.0.2008/005009-0
Mathee, K., Narasimhan, G., Valdes, C., Qiu, X., Matewish, J. M., Koehrsen, M., et al. (2008). Dynamics of Pseudomonas aeruginosa genome evolution. Proc Natl Acad Sci U S A. Feb 105, 3100–3105. doi: 10.1073/pnas.0711982105
Medina, G., Juárez, K., Valderrama, B., and Soberón-Chávez, G. (2003). Mechanism of Pseudomonas aeruginosa RhlR transcriptional regulation of the rhlAB promoter. J Bacteriol. Oct 185, 5976–5983. doi: 10.1128/JB.185.20.5976-5983.2003
Monson, R., Foulds, I., Foweraker, J., Welch, M., and Salmond, G. P. C. (2011). The Pseudomonas aeruginosa generalized transducing phage phiPA3 is a new member of the phiKZ-like group of ‘jumbo’ phages, and infects model laboratory strains and clinical isolates from cystic fibrosis patients. Microbiology. Mar 157, 859–867. doi: 10.1099/mic.0.044701-0
Mooij, M. J., Drenkard, E., Llamas, M. A., Vandenbroucke-Grauls, C. M. J. E., Savelkoul, P. H. M., Ausubel, F. M., et al. (2007). Characterization of the integrated filamentous phage Pf5 and its involvement in small-colony formation. Microbiology. Jun 153, 1790–1798. doi: 10.1099/mic.0.2006/003533-0
Morkunas, B., Galloway, W. R. J. D., Wright, M., Ibbeson, B. M., Hodgkinson, J. T., O’Connell, K. M. G., et al. (2012). Inhibition of the production of the Pseudomonas aeruginosa virulence factor pyocyanin in wild-type cells by quorum sensing autoinducer-mimics. Org. Biomol. Chem. 10, 8452–8464. doi: 10.1039/c2ob26501j
Ojeniyi, B., Birch-Andersen, A., Mansa, B., Rosdahl, V. T., and Høiby, N. (1991). Morphology of Pseudomonas aeruginosa phages from the sputum of cystic fibrosis patients and from the phage typing set. An electron microscopy study. APMIS. Oct 99, 925–930. doi: 10.1111/j.1699-0463.1991.tb01280.x
O’Toole, G. A. (2011). Microtiter dish biofilm formation assay. J Vis Exp 30, 2437. doi: 10.3791/2437
Papenfort, K., and Bassler, B. L. (2016). Quorum sensing signal-response systems in Gram-negative bacteria. Nat Rev Microbiol. 08, 576–588. doi: 10.1038/nrmicro.2016.89
Rey, M. M., Bonk, M. P., and Hadjiliadis, D. (2018). Cystic Fibrosis: Emerging Understanding and Therapies. Annu Rev Med doi: 10.1146/annurev-med-112717-094536
Rice, S. A., Tan, C. H., Mikkelsen, P. J., Kung, V., Woo, J., Tay, M., et al. (2009). The biofilm life cycle and virulence of Pseudomonas aeruginosa are dependent on a filamentous prophage. ISME J. 3, 271–282. doi: 10.1038/ismej.2008.109
Rumbo, C., Gato, E., Lopez, M., Ruiz de Alegria, C., Fernandez-Cuenca, F., Martinez-Martinez, L., et al. (2013). Contribution of efflux pumps, porins, and beta-lactamases to multidrug resistance in clinical isolates of Acinetobacter baumannii. Antimicrob Agents Chemother 57, 5247–5257. doi: 10.1128/AAC.00730-13
Saucedo-Mora, M. A., Castañeda-Tamez, P., Cazares, A., Pérez-Velázquez, J., Hense, B. A., Cazares, D., et al. (2017). Selection of Functional Quorum Sensing Systems by Lysogenic Bacteriophages in Pseudomonas aeruginosa. Front Microbiol 8:1669. doi: 10.3389/fmicb.2017.01669
Savli, H., Karadenizli, A., Kolayli, F., Gundes, S., Ozbek, U., and Vahaboglu, H. (2003). Expression stability of six housekeeping genes: A proposal for resistance gene quantification studies of Pseudomonas aeruginosa by real-time quantitative RT-PCR. J Med Microbiol. May 52, 403–408. doi: 10.1099/jmm.0.05132-0
Secor, P. R., Sweere, J. M., Michaels, L. A., Malkovskiy, A. V., Lazzareschi, D., Katznelson, E., et al. (2015). Filamentous Bacteriophage Promote Biofilm Assembly and Function. Cell Host Microbe. Nov 18, 549–559. doi: 10.1016/j.chom.2015.10.013
Silpe, J. E., and Bassler, B. L. (2019). A Host-Produced Quorum-Sensing Autoinducer Controls a Phage Lysis-Lysogeny Decision. Cell 176, 268.e–280.e. doi: 10.1016/j.cell.2018.10.059
Söding, J., Biegert, A., and Lupas, A. N. (2005). The HHpred interactive server for protein homology detection and structure prediction. Nucleic Acids Res 33, W244–W248.
Stacy, D. M., Welsh, M. A., Rather, P. N., and Blackwell, H. E. (2012). Attenuation of quorum sensing in the pathogen Acinetobacter baumannii using non-native N-Acyl homoserine lactones. ACS Chem Biol. Oct 7, 1719–1728. doi: 10.1021/cb300351x
Subramoni, S., Florez Salcedo, D. V., and Suarez-Moreno, Z. R. (2015). A bioinformatic survey of distribution, conservation, and probable functions of LuxR solo regulators in bacteria. Front Cell Infect Microbiol. 5:16. doi: 10.3389/fcimb.2015.00016
Tan, D., Svenningsen, S. L., and Middelboe, M. (2015). Quorum Sensing Determines the Choice of Antiphage Defense Strategy in Vibrio anguillarum. mBio. Jun 6, e00627. doi: 10.1093/nar/gki408
Tariq, M. A., Everest, F. L., Cowley, L. A., De Soyza, A., Holt, G. S., Bridge, S. H., et al. (2015). A metagenomic approach to characterize temperate bacteriophage populations from Cystic Fibrosis and non-Cystic Fibrosis bronchiectasis patients. Front Microbiol. 6:97. doi: 10.3389/fmicb.2015.00097
Tariq, M. A., Everest, F. L. C., Cowley, L. A., Wright, R., Holt, G. S., Ingram, H., et al. (2019). Temperate Bacteriophages from Chronic Pseudomonas aeruginosa Lung Infections Show Disease-Specific Changes in Host Range and Modulate Antimicrobial Susceptibility. mSystems 4, e191–e118. doi: 10.1128/mSystems.00191-18
Vinckx, T., Wei, Q., Matthijs, S., and Cornelis, P. (2010). The Pseudomonas aeruginosa oxidative stress regulator OxyR influences production of pyocyanin and rhamnolipids: protective role of pyocyanin. Microbiology 156, 678–686. doi: 10.1099/mic.0.031971-0
Webb, J. S., Lau, M., and Kjelleberg, S. (2004). Bacteriophage and phenotypic variation in Pseudomonas aeruginosa biofilm development. J Bacteriol 186, 8066–8073. doi: 10.1128/JB.186.23.8066-8073.2004
Wen, Z., Wang, P., Sun, C., Guo, Y., and Wang, X. (2017). Interaction of Type IV Toxin/Antitoxin Systems in Cryptic Prophages of Escherichia coli K-12. Toxins (Basel) 9, 77. doi: 10.3390/toxins9030077
Whiteley, M., Bangera, M. G., Bumgarner, R. E., Parsek, M. R., Teitzel, G. M., Lory, S., et al. (2001). Gene expression in Pseudomonas aeruginosa biofilms. Nature 413, 860–864. doi: 10.1038/35101627
Whiteley, M., Lee, K. M., and Greenberg, E. P. (1999). Identification of genes controlled by quorum sensing in Pseudomonas aeruginosa. Proc Natl Acad Sci U S A 96, 13904–13909. doi: 10.1073/pnas.96.24.13904
Wilder, C. N., Allada, G., and Schuster, M. (2009). Instantaneous within-patient diversity of Pseudomonas aeruginosa quorum-sensing populations from cystic fibrosis lung infections. Infect Immun 77, 5631–5639. doi: 10.1128/IAI.00755-09
Wilder, C. N., Diggle, S. P., and Schuster, M. (2011). Cooperation and cheating in Pseudomonas aeruginosa: the roles of the las, rhl and pqs quorum-sensing systems. ISME J 5, 1332–1343. doi: 10.1038/ismej.2011.13
Winstanley, C., and Fothergill, J. L. (2009). The role of quorum sensing in chronic cystic fibrosis Pseudomonas aeruginosa infections. FEMS Microbiol Lett 290, 1–9. doi: 10.1111/j.1574-6968.2008.01394.x
Winstanley, C., Langille, M. G., Fothergill, J. L., Kukavica-Ibrulj, I., Paradis-Bleau, C., Sanschagrin, F., et al. (2009). Newly introduced genomic prophage islands are critical determinants of in vivo competitiveness in the Liverpool Epidemic Strain of Pseudomonas aeruginosa. Genome Res 19, 12–23. doi: 10.1101/gr.086082.108
Zdobnov, E. M., and Apweiler, R. (2001). InterProScan–an integration platform for the signature-recognition methods in InterPro. Bioinformatics 17, 847–848. doi: 10.1093/bioinformatics/17.9.847
Zhang, Y., Yang, Y., Wang, L., and Weng, L. (2013). Identification of a Pseudomonas sp. that Inhibits RHL System of Quorum Sensing. Indian J Microbiol 53, 28–35. doi: 10.1007/s12088-012-0340-5
Keywords: prophages, inovirus, siphovirus, Pseudomonas, CC274 clone, cystic fibrosis
Citation: Ambroa A, Blasco L, López-Causapé C, Trastoy R, Fernandez-García L, Bleriot I, Ponce-Alonso M, Pacios O, López M, Cantón R, Kidd TJ, Bou G, Oliver A and Tomás M (2020) Temperate Bacteriophages (Prophages) in Pseudomonas aeruginosa Isolates Belonging to the International Cystic Fibrosis Clone (CC274). Front. Microbiol. 11:556706. doi: 10.3389/fmicb.2020.556706
Received: 28 April 2020; Accepted: 26 August 2020;
Published: 25 September 2020.
Edited by:
Cesar de la Fuente-Nunez, University of Pennsylvania, United StatesReviewed by:
Dinesh Sriramulu, Independent Researcher, IndiaGloria Soberón-Chávez, National Autonomous University of Mexico, Mexico
Paul Laszlo Bollyky, Stanford University, United States
Copyright © 2020 Ambroa, Blasco, López-Causapé, Trastoy, Fernandez-García, Bleriot, Ponce-Alonso, Pacios, López, Cantón, Kidd, Bou, Oliver and Tomás. This is an open-access article distributed under the terms of the Creative Commons Attribution License (CC BY). The use, distribution or reproduction in other forums is permitted, provided the original author(s) and the copyright owner(s) are credited and that the original publication in this journal is cited, in accordance with accepted academic practice. No use, distribution or reproduction is permitted which does not comply with these terms.
*Correspondence: Maria Tomás, TUEuZGVsLk1hci5Ub21hcy5DYXJtb25hQHNlcmdhcy5lcw==
†These authors have contributed equally to this work