- 1Key Laboratory of Resource Biology and Biotechnology in Western China (Ministry of Education), Northwest University, Xi’an, China
- 2College of Agronomy, Northwest A&F University, Yangling, China
- 3Department of Microbiology and Plant Biology, Center for Spatial Analysis, University of Oklahoma, Norman, OK, United States
- 4College of Urban and Environmental Sciences, Northwest University, Xi’an, China
Elevational gradients strongly affect microbial biodiversity in bulk soil through altering plant and soil properties, but the effects on rhizosphere microbial patterns remain unclear, especially at large spatial scales. We therefore designed an elevational gradient experiment to examine rhizosphere microbial (bacteria, fungi and arbuscular mycorrhizal fungi) diversity and composition using Illumina sequencing of the 16S rRNA and ITS genes for comparison to plant and soil properties. Our results showed that bacterial and fungal alpha diversity was significantly higher at mid-elevation, while AMF alpha diversity decreased monotonically. The beta diversities of the three groups were significantly affected by elevational gradients, but the effect on bacterial beta diversity was larger than on fungal and AMF beta diversity. Proteobacteria, the dominant phyla of bacteria, was significantly higher at the mid-elevation, while Acidobacteria and Actinobacteria significantly decreased as elevation increased. The main fungal taxa, Basidiomycota, significantly decreased with elevation, and Ascomycota significantly increased with elevation. Glomeromycota, the dominant AMF phyla, responded insignificantly to the elevational gradients. The responses of bacterial and fungal alpha diversity were mostly associated with tree diversity and organic carbon, whereas AMF alpha diversity mainly depended on litter N and P. Changes in bacterial community composition along the elevational gradient were explained primarily by litter N and P, and litter P was the main driver of fungal and AMF community composition. Overall, our results suggest that plant litter, particularly litter N and P, were the main source of external carbon input and drove the observed differences in rhizosphere microbial diversity and community composition. Our results highlight the importance of litter nutrition in structuring rhizosphere microbial communities in mountain ecosystems.
Introduction
In mountain ecosystems, many climate factors can vary drastically over a short spatial distance (see review by Körner, 2007), which not only influence aboveground macroorganisms but also microorganisms belowground (Fierer et al., 2011; Sundqvist et al., 2013). Therefore, elevational gradients can be used in experimental design to investigate the ecological responses of the microbial community to changing environments (Bragazza et al., 2015; Siles and Margesin, 2017). To date, a variety of studies have investigated the response of microbial community in bulk soil to elevational gradients, suggesting that elevational gradients strongly affect the microbial diversity and community composition of bulk soils by altering plant and soil characteristics (Meng et al., 2013; Li et al., 2018; Saitta et al., 2018). In such dynamic environments, the shift of the microbial community in bulk soil and its interaction with plants, particularly plant roots, can potentially alter rhizosphere microorganisms. However, few studies have examined the elevational patterns of rhizosphere microorganisms and its drivers, especially in large elevational scales. The lack of research in this area greatly hinders our predictions of nutrient cycling of terrestrial ecosystem under climate warming, despite the importance of rhizosphere microorganisms in mediating biogeochemical cycles (Van der Heijden et al., 2008; Delgado-Baquerizo et al., 2016).
Compared with microbes in bulk soil, rhizosphere microbes are strongly influenced by aboveground plants (Berg and Smalla, 2009; Pii et al., 2016). Plants are broadly perceived to influence rhizosphere microbes through the provision of carbon compounds in root exudates, plant litter, or plant secondary metabolites (Bardgett and Wardle, 2010; Schlatter et al., 2015). Plants can also significantly impact rhizosphere microbes through plant-induced changes to soil properties in the rhizosphere (e.g., N, P) (Herold et al., 2014). In montane forests, the changes in plant and soil properties induced by elevational gradients would inevitably lead to dramatic changes in the rhizosphere microbial community. In particular, shifts in plant communities could result in different microbial assemblages in the rhizosphere due to changes in aboveground (litter) and belowground (root exudation) resource inputs (Kaiser et al., 2015; Zhalnina et al., 2018). For example, arbuscular mycorrhizal fungi (AMF), which play key roles in multitrophic interactions between plants and soils, is strongly influenced by root exudates (Lugo et al., 2008). The dramatic changes in climate and vegetation that occur along the elevational gradient also influence the return rate and quality of litter nutrients (Aponte et al., 2012; Saura-Mas et al., 2012) and indirectly affect the assembly of rhizosphere microbes (Aponte et al., 2011). For example, Lang et al. (2016) found that soil phosphorus in the forest mainly comes from litter decomposition, which shape both plant and microbial communities. However, how elevational gradients affect rhizosphere microbial communities by altering above and below-ground properties is still unclear.
To comprehensively understand the changes in rhizosphere microbial communities along elevational gradient and reveal their biotic and abiotic determinants, we investigated the diversity and composition of different microbial communities (bacteria, fungi, and AMF) in rhizosphere soil along elevational gradients of approximate1300 mon Taibai Mountain. Several studies performed in this elevation range have found patterns in above-plant diversity and microbes in bulk soil (Tang et al., 2012; Ren et al., 2018a). However, the responses of rhizosphere microbial communities to the elevational gradient were not the focus of these studies. Here, we hypothesized that the elevational gradient effects on rhizosphere microbial communities depend primarily on plant and soil properties, and that different microbial communities (bacteria, fungi, and AMF) would respond differently to plant and soil properties. Specifically, we aimed to: (1) reveal how rhizosphere microbial communities respond to elevational gradient; (2) compare how rhizosphere microbial community (bacterial, fungal, and AMF) diversity and composition respond to elevational gradient; and (3) evaluate the effect of plant and soil properties on the rhizosphere microbial community.
Materials and Methods
Site Description
Our study was conducted on Taibai Mountain, Shaanxi Province, China (33°49–34°10′N; 107°19′–107°58′E, Figure 1A), which spans from 530 to 3767 m in elevation and is the highest mountain in the Qinling Mountain range. The Qinling Mountains run east–west in central China and form a transitional zone between northern subtropical and warm-temperate zones, which make it a global biodiversity hotspot (Dang et al., 2007; Guo et al., 2019). Taibai Mountain is in the northern slope of range and is in a warm temperate region. Mean annual temperature and precipitation are 11.4°C and 910.6 mm, respectively. The large span in elevation means that Taibai Mountain hosts the most complete spectrum of vegetation types in the Qingling Mountains. The natural vegetation types along the elevational gradient are oak forests (Quercus sp.) (<2200 m), birchforests (Betula sp.) (2300–2800 m), fir forests (Abies sp.) (2800–3200 m), larchforests (Larix sp.) (3000–3400 m), and alpine shrubs (>3400 m) (Ren et al., 2006).
Our experiment was conducted between elevations of 1200–2700 m where the topography was relatively consistent but the vegetation varied with elevation and included Quercus acutidentata forest (1200–1800 m), Quercus liaotungensi (1900–2300 m), Betula albosinensis (2300–2600 m), and Betula utilis (2600–2700 m). Within the elevational range, we selected six elevations (1308, 1603, 1915, 2292, 2405, and 2600 m) as our experimental sites (Figure 1B). For each elevation, samples were collected from the three independent replicate plots (50 × 50 m each), the plots were separated by ≥ 13.5 m to obtain independent samples for statistical analysis (Marriott et al., 1997). For each variable, we averaged measurements from the three replicates at the same elevation to represent the observations of the study site. In total, 18 observations were obtained (six elevations × three replicates) for each variable. The experiments were carried out in July 2018.
Plant Survey and Soil Sampling
For plant investigation, three 10 × 10 m quadrants, five 5 × 5 m quadrants, and ten 1 × 1 m quadrants were randomly selected in each 50 × 50 m plot to determine the composition and richness for tree, shrub, and herb, respectively. For woody plants, the percentage of total base diameter for each family was calculated to reflect the relative importance. For herbaceous plants, the relative cover of each family was calculated to represent the relative importance. The leaves, litter, and fine roots (0–10 cm depth) were collected from major tree species at each elevation to determine their carbon, nitrogen, and phosphorus content. The fine roots were separated from the soil via water bath extraction and with the help of a fine meshed sieve.
Soil was sampled only for the rhizosphere soil, which we defined as soil tightly adhering to the root surface of the dominant tree species. Rhizosphere soil was also collected from the three independent replicate plots for each elevation. For each 50 × 50 m plot, the rhizosphere soil of ten individuals of the dominant tree species were show collected with a sterile soft brush and then were homogenized to represent the rhizosphere soil sample of the plot. After sampling, each soil sample was divided into two subsamples. One subsample was immediately place in an insulated container with ice, transported to the laboratory, and then stored at −80°C for DNA extraction. The other subsample was air-dried after passing through a 2 mm sieve to analyze soil properties.
Plant and Soil Properties Analysis
We first dried plant samples (leaves, roots, and litter)to a constant weight in an oven at 60°C and then determined their C, N, and P contents after grinding finely to 0.15 mm with a ball mill (Wang et al., 2015). The C content was determined using the K2Cr2O7 oxidation method, and the N and P contents of the digested solution were determined using the Kjeldahl and colorimetric (UV spectrophotometer) methods, respectively (Lin et al., 2011). For rhizosphere soil samples, the Walkley-Black method (Nelson et al., 1982), the Kjeldahl method (Bremner and Mulvaney, 1982), and the colorimetric method after wet digestion with H2SO4 + HClO4 were used to determine soil organic carbon (SOC), total nitrogen (TN), and total phosphorus (TP), respectively.
DNA Extraction and Sequencing
Following the manufacturer’s instructions, soil (0.5 g fresh weight) was extracted using the FastDNA Spin Kit (MP Biomedicals, Cleveland, United States). The concentration and quality of the DNA were evaluated by a NanoDrop 2000 spectrophotometer (Thermo Fisher Scientific, Wilmington, DE, United States). PCR of the bacterial 16S rRNA gene targeting the V4 region was amplified using primers 515F (5′-GTGCCAGCMGCCGCGG-3′) and 907R (5′-CCGTC AATT CMTTTRAGTTT-3′) (Biddle et al., 2008). The amplification of the fungal ITS-1 region was achieved using primers ITS1F (50-ACTTGGTCATTTAGAG-GAAGTAA-30) and ITS2 (50-BGCTGCGTTCTTCATCGATGC-30) (Mukherjee et al., 2014). Partial small subunit (SSU) ribosomal RNA gene fragments of arbuscular mycorrhizal fungi were amplified using nested PCR (Borriello et al., 2012), with the universal eukaryotic primers NS1 and NS4(White et al., 1990), and a subsequent amplification round with the Glomeromycota-specific primers AML1 and AML2 (Jaikoo et al., 2010). The PCR products were extracted from 2% agarose gels and purified using the AxyPrep DNA Gel Extraction Kit (Axygen Bio-sciences, Union City, CA, United States) according to the manufacturer’s instructions and quantified using QuantiFluorTM −ST (Promega, United States.). Finally, an equal amount of PCR product from each sample was sent for pyrosequencing using the Illumina’s MiSeq platform at Personal Biotechnology Co., Ltd. Shanghai, China.
For all sequencing reads, raw fastq files were demultiplexed, quality-filtered using following criteria: (i) the reads were truncated with an average quality score < 20 in a 50 bp sliding window; (ii) primers were exactly matched allowing two nucleotide mismatches, and reads containing ambiguous bases were removed; (iii) sequences with > 10 bp overlap were merged according to their overlap sequence. Quantitative Insights Into Microbial Ecology pipeline software (QIIME) software (v1.8.0)1 was used to obtain 16S rRNA operational taxonomic units (OTUs) (Caporaso et al., 2012). Sequence analysis was performed using the USEARCH v5.2.32 to filter and eliminate noise from the data by clustering similar sequences with more than 97% similarity. Finally, the complete dataset was sent to the Sequence Read Archive (SRA) database of the National Center for Biotechnology Information (NCBI) under Accession NO. SRP223550 for bacteria, SRP223554 for fungi and SRP223556 for AMF.
Statistical Analyses
We used species richness, Shannon index, and Simpson’s index (1/D) to estimate the alpha diversity of the plant community and the Shannon index to calculate the alpha diversity of rhizosphere microbial communities (bacterial, fungal and AMF). In order to investigate the relationships between biological communities and elevational gradient, a non-metric multidimensional scaling (NMDS) analysis was conducted for the plant and rhizosphere microbial communities based on Bray-Curtis distances. In addition, we used the analysis of similarities (ANOSIM) to determine the significance of separation along the climate gradients (Clarke et al., 2014). The alpha diversity index was calculated using Mothur software (Schloss et al., 2009). NMDS and ANOSIM were performed using the“vegan” package of R program (R Core Team, 2016).
To further investigate the possible pathways through which plant communities influence the composition of the rhizosphere microbial community directly and indirectly along the spatial gradients, we preformed partial least squares path modeling (PLS-PM). Through PLS-PM analysis, the observed variables can be explained by the latent variables (Sanchez, 2013). In our study, the latent variables included elevation, plant properties, soil properties, and rhizosphere microbial communities (bacterial, fungal and AMF). Each latent variable could include at least one observed variable. In the PLS-PM, the direction and strength of linear relationships between latent variables was represented by the path coefficients and the explained variability (R2) was also estimated. The effective model was evaluated by the average variance extracted (AVE) and the composite reliability (CR). When the AVE and CR are higher than 0.5 and 0.7, respectively, this model is acceptable. This analysis was conducted using PLS, provided by the Smart PLS 2.0 M3 software. In our study, both the AVE and CR fit these standards (AVE > 0.5; CR > 0.7) (Vanalle et al., 2017).
Furthermore, the relationships between the rhizosphere microbial characteristics and the plant and soil properties were determined using spearman correlation analysis. Correlations between the rhizosphere microbial compositions and the plant and soil properties were determined using redundancy analysis (RDA) (Clarke et al., 2014). The one-way analysis of variation (ANOVA) was used to test the effect of elevation gradients on plant and soil characteristics, rhizosphere microbial diversity, and dominant phyla. The analysis were conducted using the “vegan” package of R program. P < 0.05 was considered statistically significant.
Results
Plant and Soil Properties Along the Elevational Gradient
Plant and soil characteristics were affected greatly by elevational gradient but showed different trends (Table 1). For plant communities, the alpha diversity (richness, Simpson, and Shannon index) of trees and shrubs were significantly higher at mid-elevation, while the alpha diversity of herb community had no significant trend with elevation. The species composition of the plant communities also changed along the elevational gradient (Supplementary Table S1). Particularly for woody plant community, the NMDS ordination showed a clear separation of sites based on the species composition along the elevation (Figure 2). In addition, leaf C, leaf N, leaf P, litter C, root C, and root N were significantly higher at mid-elevation. Litter N increased with elevation, and litter P was higher at low elevation. For rhizosphere soil characteristics, SOC and TN were also higher at mid-elevation. However, root P and soil TP did not significantly change with the elevation.
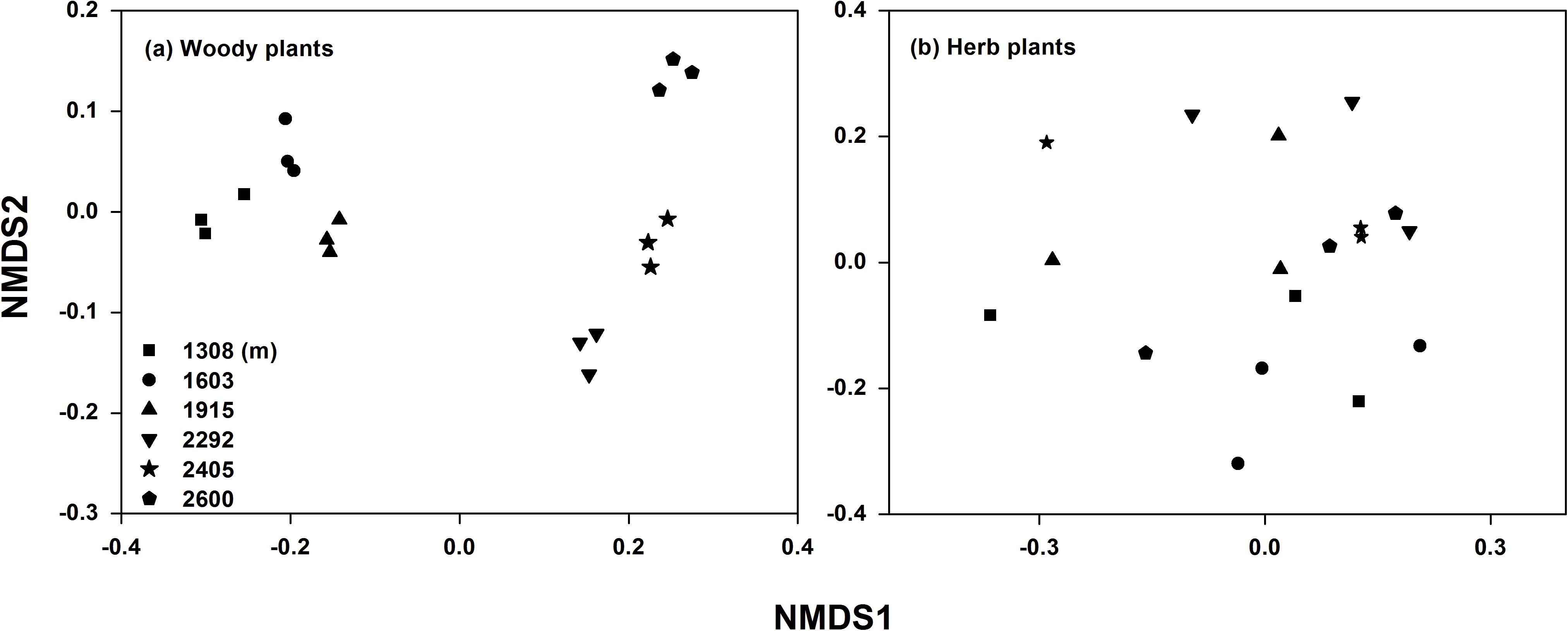
Figure 2. Non-metric multidimensional scaling (NMDS) analysis of woody (a) and herb (b) plant community compositions along the elevational gradient.
Microbial Diversity and Composition Along the Elevational Gradient
After quality sequencing, a total of 1,154,395 bacterial sequences, 1,321,332 fungal sequences and 1,614,413 AMF sequences were identified from the 18 soil samples. For bacteria, there were 48,477–76,746 sequences per sample, with a mean of 64,133 sequences. For fungi, the number of sequences ranged from 49,297 to 129,882 per sample, with a mean of 73,407 sequences. For AMF, the sequences varied from 80,476 to 105,892 per sample, with a mean of 89,689 sequences. To minimize any bias in the distribution of taxa, bacterial, fungal and AMF diversity of each treatment were calculated based on randomly selected sequence until the count reached saturation in the rarefaction curves. For the downstream analysis of bacteria, datasets were rarefied to 48,000 sequences. For downstream analysis of fungi, datasets were rarefied to 49,000 sequences. For downstream analysis of AMF, datasets were rarefied to 70,000 sequences.
Based on the Shannon index, rhizosphere microbial alpha diversity significantly changed with elevational gradient, and elevational gradient had more of an impact on bacterial alpha diversity than fungal and AMF alpha diversity (Figure 3A). More specifically, bacterial and fungal alpha diversity were significantly higher at mid-elevation, while AMF alpha diversity declined as elevation increased. Furthermore, the NMDS ordination reflected a clear separation of sites along the elevation gradient for the rhizosphere bacterial and AMF communities (Figures 3B,D). For fungi, the degree of aggregation of the sample sites at the lower elevation was distant but not for sites at higher elevation (Figure 3C). Analysis of similarities (ANOSIM) also showed that the influence of the elevational gradient on bacterial beta diversity (R = 0.90, p < 0.01) was higher than its influence on fungal beta diversity (R = 0.69, p < 0.01) and AMF beta diversity (R = 0.61, p < 0.01).
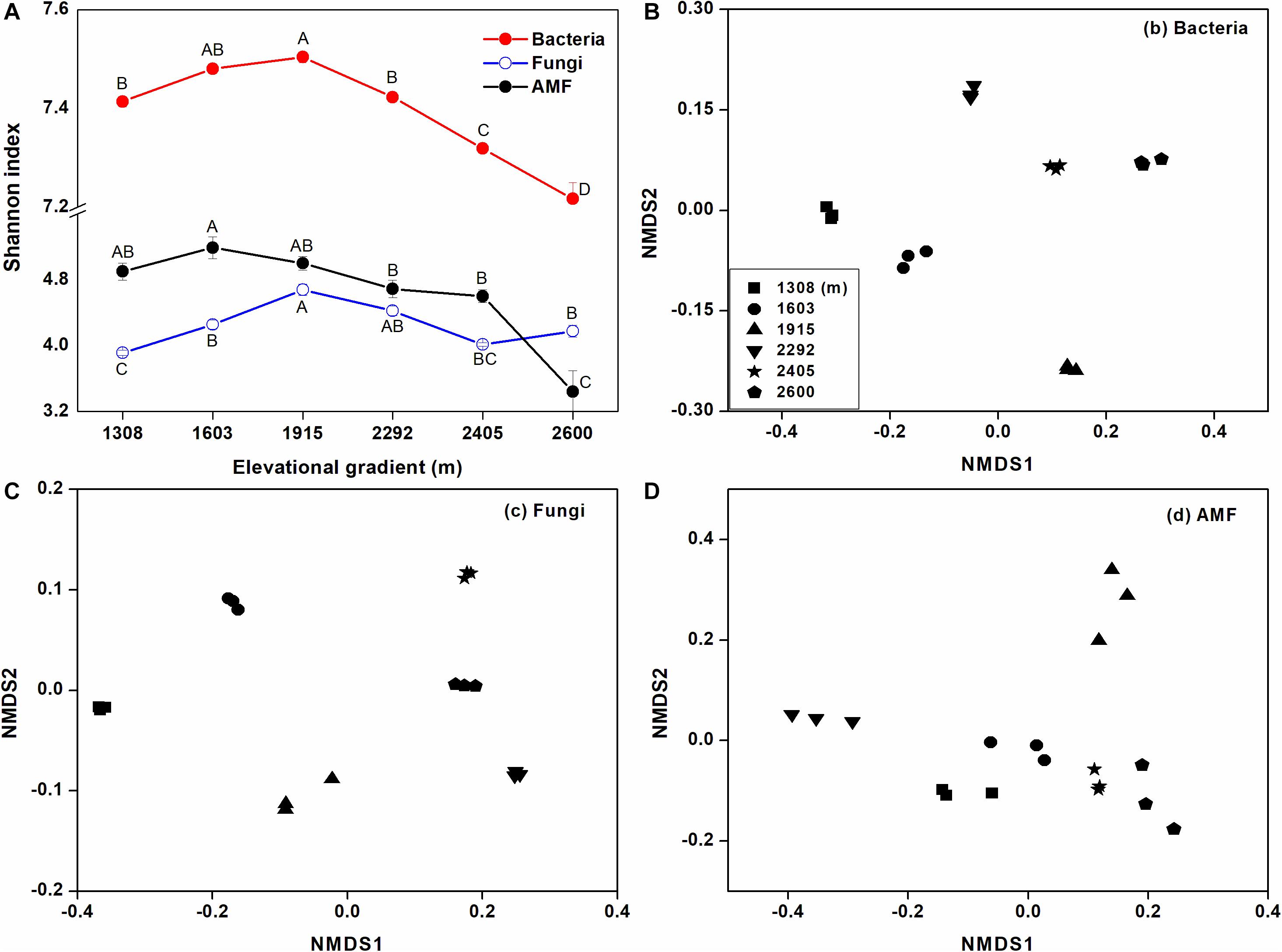
Figure 3. The effects of elevational gradient on microbial (bacterial, fungal, and AMF) alpha (A) and beta diversity (B–D) in rhizosphere soil. The bar represents standard deviation (SD). The beta diversity is represented by unweighted UniFrac distances based on the relative abundances of OTUs.
As for rhizosphere microbial composition (Figure 4), most of the bacterial community was Proteobacteria (44.58–52.25%), Acidobacteria (21.89–29.61%), and Actinobacteria (5.8–10.68%), with Chloroflexi, Gemmatimonadetes, Planctomycetes, and Bacteroidetes each accounting for ≤ 5.0% (Supplementary Table S2). Of those bacteria, Proteobacteria was significantly more abundant at the mid-elevation. Acidobacteria and Actinobacteria both significantly decreased with elevation, whereas Nitrospirae and Chloroflexi increased with elevation. For the fungal community, the dominant phyla were Basidiomycota (64.08–82.05%), Ascomycota (12.36–28.53%), Zygomycota (1.14–5.81%), and a small proportion of unidentified fungi (0.33–1.58%) (Supplementary Table S3). Basidiomycota significantly decreased with elevation and Ascomycota significantly increased with elevation. The AMF community was dominated by Glomeromycota (96.29–99.25%) and a small proportion of the community was unidentified (≤ 5.0%) (Supplementary Table S4). However, the composition of the AMF community did not significantly change with the elevation.
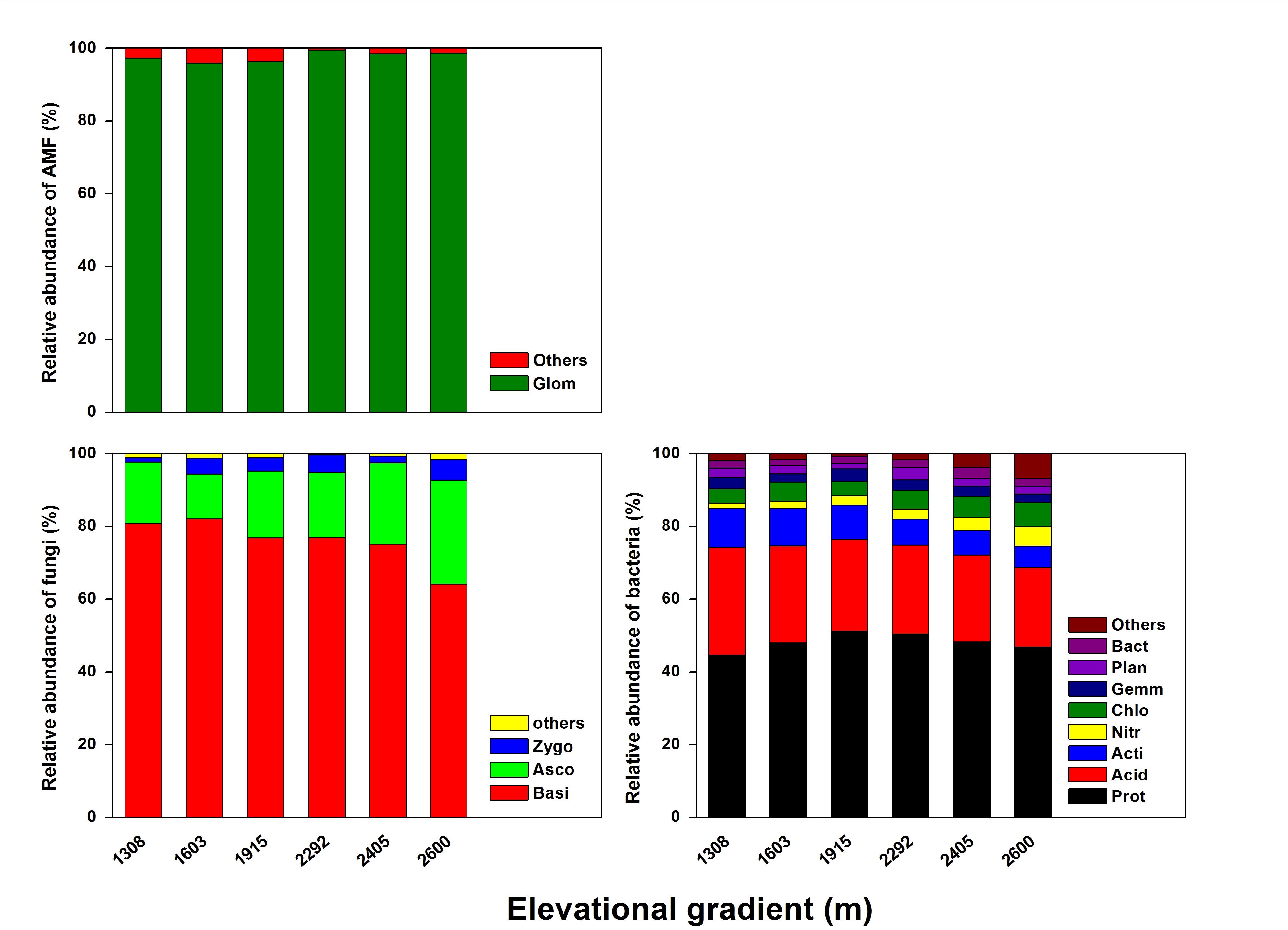
Figure 4. The effects of elevation gradient on the bacterial, fungal, and AMF taxonomic compositions in rhizosphere soil. These are the abbreviations of microbial taxa: Proteobacteria (Prot), Acidobacteria (Acid), Actinobacteria (Acti), Nitrospirae (Nitr), Chloroflexi (Chlo), Gemmatimonadetes (Gemm), Planctomycetes (Plan), Bacteroidetes (Bact), Basidiomycota (Basi), Ascomycota (Asco), Zygomycota (Zygo), Glomeromycota (Glom).
Effect of Plant and Soil Properties on Microbial Diversity and Composition
Plant and soil properties had significant relationships with rhizosphere microbial diversity (Table 2). Among the plant properties, tree alpha diversity was the most significant attribute affecting bacterial and fungal alpha diversity, while AMF alpha diversity was mostly affected by litter N and litter P. Litter N and litter P were the most significant attributes affecting bacterial and fungal beta diversity, while AMF beta diversity was mostly affected by leaf N and leaf P. Among the soil properties, SOC was the most significant attribute affecting bacterial, fungal, and AMF alpha diversity. Soil TN and TP significantly affected bacterial and fungal beta diversity, and AMF beta diversity was not affected by soil properties. Our PLS-PM analysis showed that rhizosphere bacteria were affected by plant and soil properties together, while fungi and AMF was affected mainly by plant and litter properties and weakly influenced by soil properties (Figure 5).
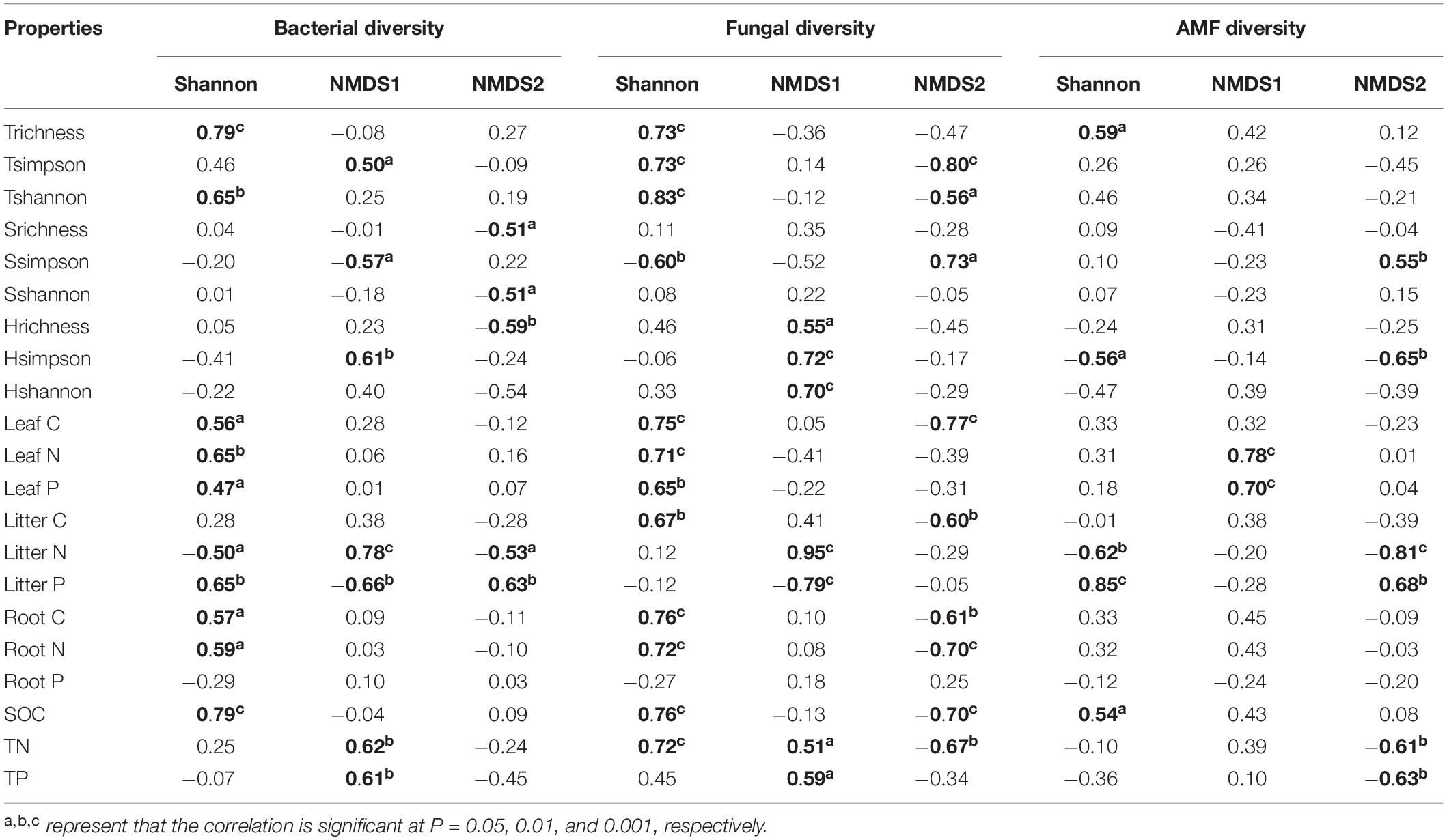
Table 2. The relationships of microbial diversity with plant and soil properties based on spearman rank correlation analysis.
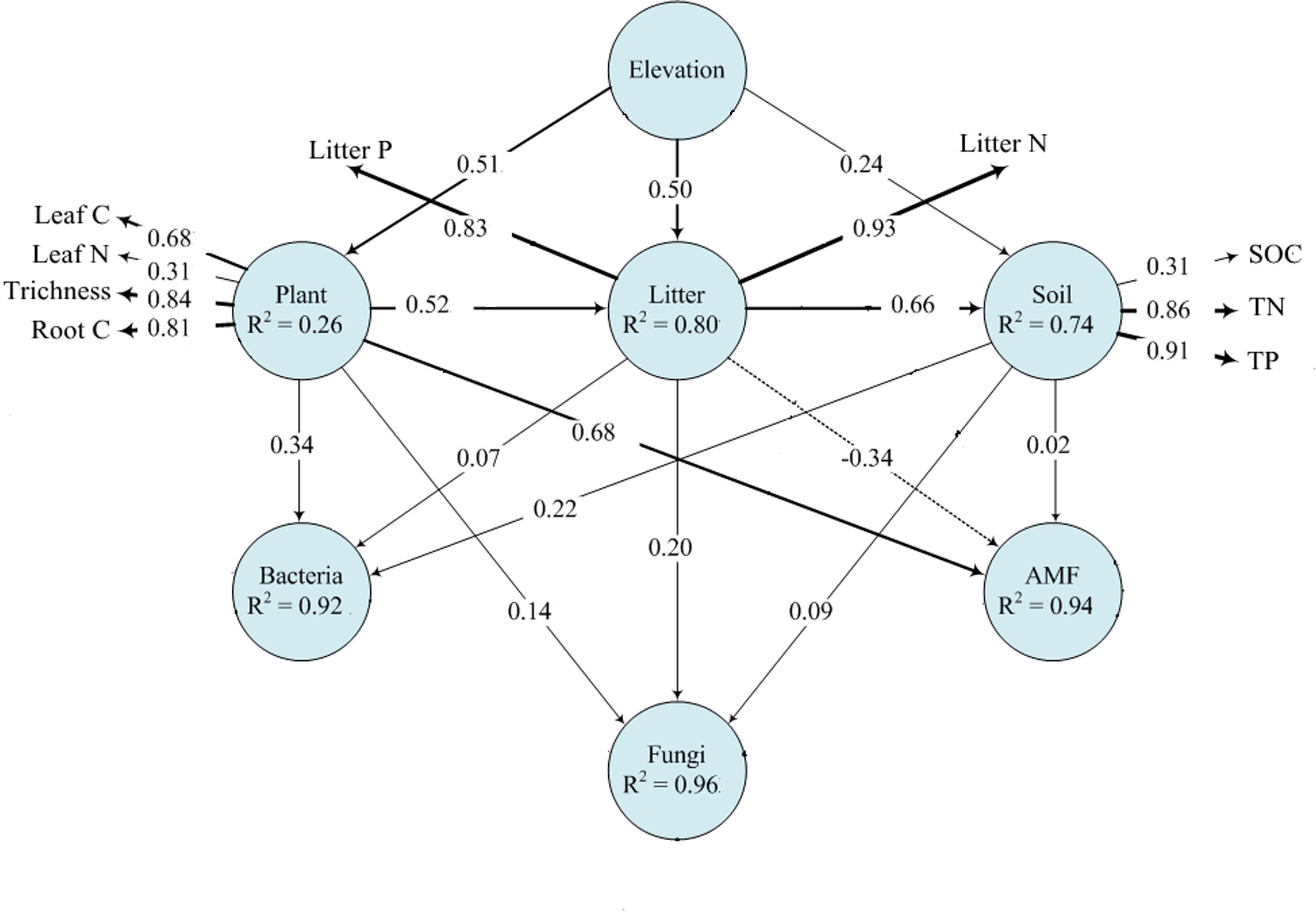
Figure 5. Effects of elevation on microbial communities by plant and soil properties based on PLS-PM analysis. The width of arrows is proportional to the strength of path coefficients. Continuous and dashed arrows indicate positive and negative relationships, respectively. R2 denotes the proportion of variance explained.
RDA demonstrated that the composition of the rhizosphere microbial community at the phylum level was largely affected by plant properties rather than soil properties (Figure 6). Plant properties, particularly litter P, were responsible for much of the variations in the composition of all rhizosphere microbial taxa (bacteria, fungi, and AMF). Litter N, litter P, and tree Simpson index were significantly related to changes of Chloroflexi, Nitrospirae, Acidobacteria, Actinobacteria, Gemmatimonadetes, and Planctomycetes in the bacterial community. Litter P and shrub richness were associated with changes of Basidiomycota, Ascomycota, and Zygomycota in the fungal community, and litter P was correlated with Glomeromycota and other phylum in the AMF community.
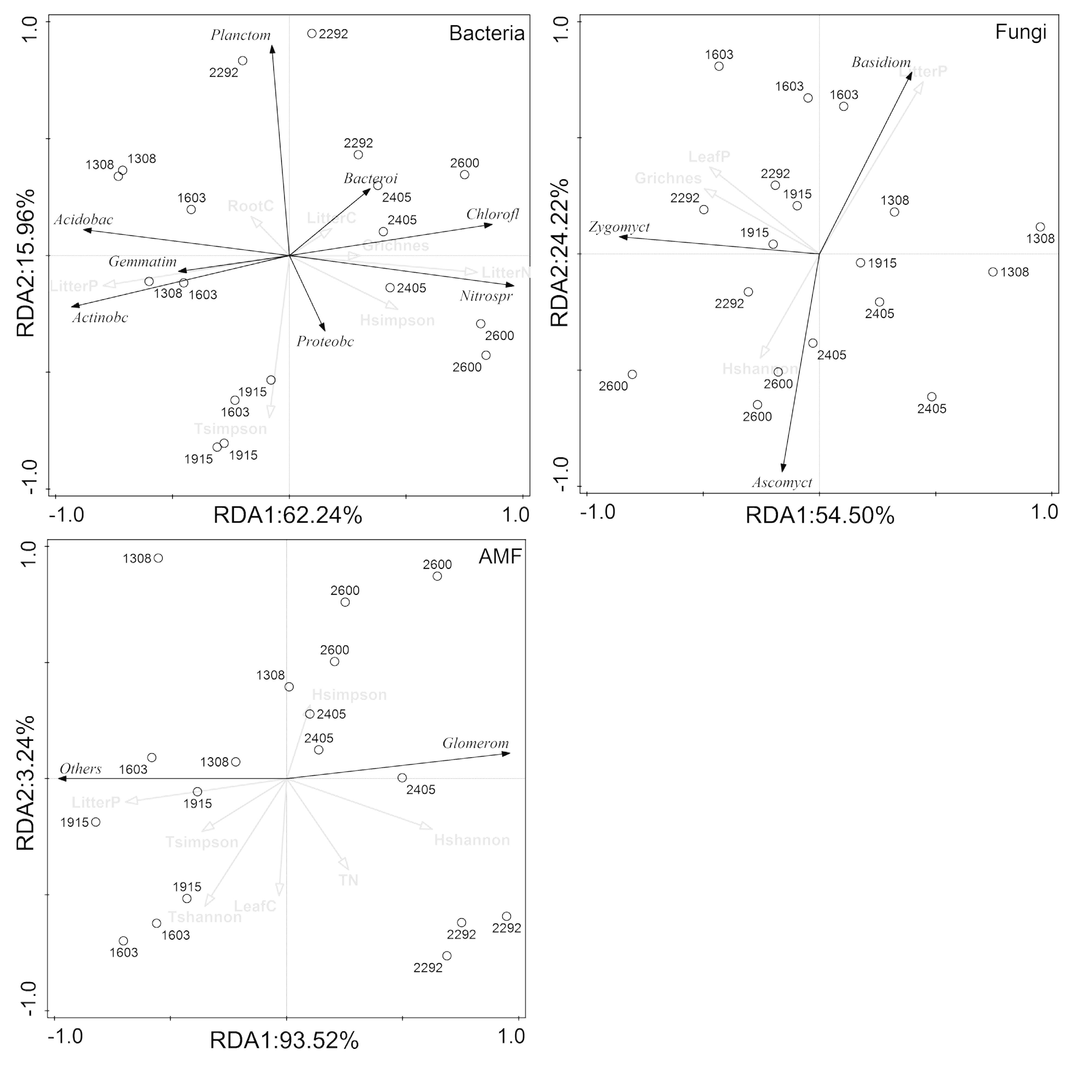
Figure 6. Redundancy analysis (RDA) ordination to identify the relationships between microbial phyla and plant and soil properties.
Discussion
Differences in Rhizosphere Bacterial, Fungal, and AMF Diversity Along the Elevation Gradient
Elevational gradients lead to shifts in plant and soil properties, and then cause the microbial diversity in rhizosphere soils to change (Berg and Smalla, 2009). However, the effects of plant and soil properties on diversity patterns of rhizosphere microbes along the elevation remain unclear. By studying the rhizosphere microbial community and corresponding plant and soil properties along a 1300 m elevational range on Taibai Mountain, a global biodiversity hot spot, we found that rhizosphere microbial diversity changed significantly along the elevational gradient depending on plant and soil properties.
In this study, both rhizosphere bacterial and fungal alpha diversity were higher at mid-elevation. The diversity of bacterial community observed was consistent with the pattern reported by Ren et al. (2018a), which investigated bulk soil microorganisms on Taibai Mountain. However, Ren et al. (2018a) found that the elevational gradient had no effects on fungal alpha diversity in bulk soil. This suggested that rhizosphere fungal diversity, compared with rhizosphere bacterial diversity, was more influenced by aboveground vegetation. In general, rhizosphere soil has a higher nutrient and moisture content than bulk soil due to root activities (Kielak et al., 2008; Ai et al., 2012). Guo et al. (2016) found that the diversity of the rhizosphere fungal community was generally higher than in bulk soil. In addition, most fungi are dependent on a particular host plant species or genera (Grayston et al., 1998). A great number of studies have shown a positive relationship between fungal richness and plant richness (Hiiesalu et al., 2014; Tedersoo et al., 2014, 2016). For example, Saitta et al. (2018) found that fungal richness was strongly associated with tree richness along an elevational gradient. In agreement with these studies, our study also found that tree alpha diversity was the most significant attribute affecting fungal alpha diversity among the plant and soil properties (Table 2).
In contrast to the bacterial and fungal communities, the AMF community exhibited a monotonically decreasing pattern along the elevational gradient. This result was consistent with other published studies, such as Lugo et al. (2008) and Gai et al. (2012), which showed similar trends in AMF diversity along elevational gradients. However, these studies did not investigate the drivers of these changes in the AMF assemblages. In our study, the change in AMF diversity along the elevational gradient was highly related to litter P (Table 2). In forest ecosystems, most phosphorus is sequestrated in plant litter, which limits its availability for plants. In this situation, AMF can improve phosphorus availability for plants and simultaneously obtain carbon from root exudates (Landis and Fraser, 2008; Lugo et al., 2008). Therefore, litter P plays a more important role in regulating AMF diversity than other properties.
Moreover, elevational gradients also showed significant effects on rhizosphere microbial beta diversity (Figure 2). For the bacterial and fungal community, their beta diversity was most closely correlated with litter N and P (Table 2). This correlation probably arises because changes in nutrient availability, due to differing return rates and litter nutrient quality along the climate and vegetation gradient, ultimately lead to differences in the rhizosphere bacterial and fungal community (Jacob et al., 2009). In addition, rhizosphere soil TN and TP were also responsible for differences in bacterial and fungal beta diversity, which further indicated that the availability of N and P drive the changes in the rhizosphere bacterial and fungal communities along the elevational gradient. In contrast, AMF beta diversity responded significantly to leaf N and P. This response is probably due to the selective effects of host plant root exudates on a specific AMF population (Hartmann et al., 2009). Leaf N and P reflect the nutrient uptake and metabolic strategies of plants (Richardson et al., 2009). Previous studies have suggested that AMF was correlated with plant metabolic type (Hetrick et al., 1990; Lugo and Cabello, 2002). Therefore, leaf N and leaf P can explain the change in AMF beta diversity.
In addition, analysis of the community alpha and beta diversity response to elevational gradient revealed that bacteria varied more than fungi and AMF (Figure 2). This result agreed with other previous reports that bacterial communities is more sensitive to changes in climate, such as rainfall and temperature (Yuste et al., 2011), than fungal communities (Ren et al., 2018b). The different responses were associated with plant and soil properties. Compared with rhizosphere bacteria, rhizosphere fungi and AMF were more affected by plant properties, which may offset the direct effect of elevational gradients (Susan et al., 2015). Overall, these results satisfied our hypothesis that different rhizosphere microbial taxa respond differently to elevational gradients. Plant properties had more significant effects on rhizosphere microbial diversity than soil properties, particularly for the AMF community.
Differences in Rhizosphere Bacterial, Fungal, and AMF Community Compositions Along the Elevation Gradient
In addition to the effect of plant and soil characteristics on rhizosphere microbial diversity, the influence of these properties on the community composition of rhizosphere microbial taxa has also been seldom reported. Here, we found that elevational gradients had different effects on the compositions of bacterial, fungal, and AMF communities. Such differential responses can be largely explained by plant litter N and litter P rather than soil properties (Figure 4), which further indicate that plant properties were the main driver for the changes of the rhizosphere microbial structure.
For the bacterial community, Proteobacteria was most abundant at mid-elevation and was largely affected by tree Simpson index (Figures 2, 4), which might be because Proteobacteria prefers available carbon (Fierer et al., 2007). Highly diverse tree communities have substantially greater rhizosphere resource input by root exudation, and therefore may promote Proteobacteria. In addition, Proteobacteria was one of the most diverse phyla in the bacterial community, and higher soil resource concentrations and diversity can modulate competitive species interactions within the phylum and thus promote their abundance (Schlatter et al., 2015). Acidobacteria and Actinobacteria were most abundant at low-elevation and were mostly related to changes in litter N and litter P (Figures 2, 4). This relationship is probably because higher litter P and lower litter N at lower elevation restrict litter decomposition and nutrient return, and result in lower resource availability (Aponte et al., 2012; Saura-Mas et al., 2012). Acidobacteria and Actinobacteria have been found to adapt to acidic and resource-limited conditions (Stroobants et al., 2014; Siles and Margesin, 2016). Therefore, higher litter P and lower litter N promote their abundance. Other specific taxa, mainly Nitrospirae and Chloroflexi, were also most closely related to litter N and litter P (Figure 5), and these patterns were likely due to their ecological strategies (Sorokin et al., 2012; Siles and Margesin, 2016). Therefore, these results suggested that the different responses of rhizosphere bacterial community composition to the elevational gradients were more dependent on plant characteristics than soil characteristics.
The fungal community composition was largely explained by litter P (Figure 5), which is in line with Marschner et al. (2006), who reported that fungal community composition in the rhizosphere was affected by P availability since P availability in the forest largely depended on the return of litter P. In addition, He et al. (2016) suggested that available P was a key parameter that determines the diversity and composition of the fungal community, and other soil parameters played secondary roles. However, the dominant fungal phyla, Basidiomycota and Ascomycota, responded oppositely to the change in litter P (Figure 4), which might be caused by their different responses to elevational change. Basidiomycota decreased with the elevational gradient, Ascomycota while increased with the elevational gradient (Supplementary Table S3). The different response between Basidiomycota and Ascomycota might be attributed to their different ability to decompose litter. As the primary decomposers of dead plant biomass, many studies have found that fungal communities changes during litter decomposition (Osono, 2007; Herzog et al., 2019). In particular, Ascomycota phylum are found to have higher relative abundances during the early stages of decomposition because they have a limited ability to decompose complex organic litter (Osono and Takeda, 2001; Vorísková and Baldrian, 2013),while Basidiomycota phylum are relative more abundant during the later stages of decomposition because of their capability to synthesize enzymes required for the degradation of complex polymers (Hannula et al., 2010; Vorísková and Baldrian, 2013). In montane system, litter decomposition would decrease across the elevation gradient (Wang et al., 2010). Therefore, Basidiomycota have a higher relative abundance in lower elevation, while Ascomycota have a higher relative abundance in higher elevation.
As with the fungal community, the variation in AMF phylum composition can be largely explained by their close relationships with litter P. As the dominant phylum, Glomeromycota was negatively related with litter P, which might be due to the function of Glomeromycota in the plant-AMF interaction. P uptake is considered the main role of AMF symbiosis (Richardson et al., 2009). In order to acquire more P, under P-limiting conditions the plant will invest more C to increase Glomeromycota (Landis and Fraser, 2008). Camenzind et al. (2014) found that elevated P availability reduced AMF abundance significantly. Thus, Glomeromycota was more abundant in the low litter P environment. Together with our findings on bacterial and fungal community composition responses, our results showed that the rhizosphere microbial community composition was primarily driven by changes in litter N and P along the elevational gradients. In light of the importance of litter properties for rhizosphere microbial assembly, and litter chemistry rather than the plant species composition per se, should receive more attention in future studies that investigate plant-microbe interactions in the rhizosphere.
Conclusion
We found different responses of rhizosphere microbial diversity and community composition to elevational gradient depending on plant and soil properties. In particular, the changes of rhizosphere microbes along the elevational gradient were more explained by plant properties than soil properties. Rhizosphere bacterial and fungal alpha diversity was higher at mid-elevation sites and was mainly dependent on the dynamics of tree diversity and SOC. Rhizosphere AMF diversity was higher at the low-elevation sites and was mainly dependent on the dynamics of litter N and P. The changes in bacterial community composition were mainly dependent on litter N and P, while the changes in fungal and AMF community composition were mainly dependent on litter P. These results highlight the importance of plant litter nutrition in regulating rhizosphere microbial communities in montane forest, and provide insights into the elevational pattern and drivers of rhizosphere microbial community in montane ecosystems.
Data Availability Statement
All datasets generated for this study are included in the article/Supplementary Material.
Author Contributions
YG and FZ conceived and designed the experiments. FZ, YG, CR, and JY performed field work and the experiments. YG analyzed the data and wrote the manuscript. RD and CR revised the manuscript. All authors contributed to the article and approved the submitted version.
Funding
This study was financially supported by the National Natural Science Foundation of China (41601578) and the National Natural Science Foundation of China (31600337).
Conflict of Interest
The authors declare that the research was conducted in the absence of any commercial or financial relationships that could be construed as a potential conflict of interest.
Acknowledgments
We are grateful to Peng Zhao and Xiuxiu Feng (Northwest University) for field work and the logistical support from Mount Taibai National Nature Reserve.
Supplementary Material
The Supplementary Material for this article can be found online at: https://www.frontiersin.org/articles/10.3389/fmicb.2020.02042/full#supplementary-material
Footnotes
References
Ai, C., Liang, G., Sun, J., Wang, X., and Zhou, W. (2012). Responses of extracellular enzyme activities and microbial community in both the rhizosphere and bulk soil to long-term fertilization practices in a fluvo-aquic soil. Geoderma 173, 330–338. doi: 10.1016/j.geoderma.2011.07.020
Aponte, C., García, L. V., and Maranon, T. (2012). Tree species effect on litter decomposition and nutrient release in mediterranean oak forests changes over time. Ecosystems 15, 1204–1218. doi: 10.1007/s10021-012-9577-4
Aponte, C., García, L. V., Pérez-Ramos, I. M., Gutiérrez, E., and Marañón, T. (2011). Oak trees and soilinteractions in Mediterranean forests: a positive feedback model. J. Veg. Sci. 22, 856–867. doi: 10.1111/j.1654-1103.2011.01298.x
Bardgett, R. D., and Wardle, D. A. (2010). Abovegroundbelowground Linkages: Biotic Interactions, Ecosystem Processes, and Global Change. New York, NY: Oxford University Press.
Berg, G., and Smalla, K. (2009). Plant species and soil type cooperatively shape the structure and function of microbial communities in the rhizosphere. FEMS Microbiol. Ecol. 68, 1–13. doi: 10.1111/j.1574-6941.2009.00654.x
Biddle, J., Fitz-Gibbon, S., Schuster, S., Brenchley, J., and House, C. (2008). Metagenomic signatures of the Peru Margin subseafloor biosphere show a genetically distinct environment. Proc. Natl. Acad. Sci. U.S.A. 105, 10583–10588. doi: 10.1073/pnas.0709942105
Borriello, R., Lumini, E., Girlanda, M., Bonfante, P., and Bianciotto, V. (2012). Effects of different management practices on arbuscular mycorrhizal fungal diversity in maize fields by a molecular approach. Biol. Fert. Soils 48, 911–922. doi: 10.1007/s00374-012-0683-4
Bragazza, L., Bardgett, R. D., Mitchell, E. A. D., and Buttler, A. (2015). Linking soil microbial communities to vascular plant abundance along a climate gradient. New Phytol. 205, 1175–1182. doi: 10.1111%2Fnph.13116
Bremner, J., and Mulvaney, C. (1982). “Nitrogen total,” in Methods of Soil Analysis. Part 2. Chemical and Microbiological Properties (Madison, WI: Soil Sci. Soc. Am. Inc. Publisher).
Camenzind, T., Hempel, S., Homeier, J., Horn, S., Velescu, A., Wilcke, W., et al. (2014). Nitrogen and phosphorus additions impact arbuscular mycorrhizal abundance and molecular diversity in a tropical montane forest. Global Change Biol. 20, 3646–3659. doi: 10.1111/gcb.12618
Caporaso, J. G., Lauber, C. L., Walters, W. A., Berg-Lyons, D., Huntley, J., Fierer, N., et al. (2012). Ultra-high-throughput microbial community analysis on the Illumina HiSeq and MiSeq platforms. ISME J. 6, 1621–1624. doi: 10.1038/ismej.2012.8
Clarke, K., Gorley, R., Somerfield, P., and Warwick, R. (2014). Change in Marine Communities: An Approach to Statistical Analysis and Interpretation, 3nd Edn. Plymouth: PRIMER-E.
Dang, H., Jiang, Q., and Zhang, Y. (2007). Growth responses of subalpine fir (Abies fargesii) to climate variability in the Qinling Mountain. China. For. Ecol. Manag. 240, 143–150. doi: 10.1016/j.foreco.2006.12.021
Delgado-Baquerizo, M., Maestre, F. T., Reich, P. B., Jeffries, T. C., Gaitan, J. J., Encinar, D., et al. (2016). Microbial diversity drives multifunctionality in terrestrial ecosystems. Nat. Commun. 7:10541. doi: 10.1038/ncomms10541
Fierer, N., Bradford, M., and Jackson, R. (2007). Toward an ecological classification of soil bacteria. Ecology 88, 1354–1364. doi: 10.1890/05-1839
Fierer, N., McCain, C. M., Meir, P., Zimmermann, M., Rapp, J. M., Silman, M. R., et al. (2011). Microbes do not follow the elevational diversity patterns of plants and animals. Ecology 92, 797–804. doi: 10.1890/10-1170.1
Gai, J. P., Tian, H., Yang, F. Y., Christie, P., Li, X. L., and Klironomos, J. N. (2012). Arbuscular mycorrhizal fungal diversity along a Tibetan elevation gradient. Pedobiologia 55, 145–151. doi: 10.1016/j.pedobi.2011.12.004
Grayston, S. J., Wang, S., Campbell, C. D., and Edwards, A. C. (1998). Selective influence of plant species on microbial diversity in the rhizosphere. Soil Biol. Biochem. 30, 369–378. doi: 10.1016/S0038-0717(97)00124-7
Guo, J., McCulley, R. L., Phillips, T. D., and McNear, D. H. Jr. (2016). Fungal endophyte and tall fescue cultivar interact to differentially affect bulk and rhizosphere soil processes governing C and N cycling. Soil Biol. Biochem. 101, 165–174. doi: 10.1016/j.soilbio.2016.07.014
Guo, Y., Zhao, P., and Yue, M. (2019). Canopy disturbance and gap partitioning promote the persistence of a pioneer tree population in a near-climax temperate forest of the Qinling Mountains. China. Ecol. Evol. 9, 7676–7687. doi: 10.1002/ece3.5319
Hannula, S. E., de Boer, W., and van Veen, J. A. (2010). In situ dynamics of soil fungal communities under different genotypes of potato, including a genetically modified cultivar. Soil Biol. Biochem. 42, 2211–2223. doi: 10.1016/j.soilbio.2010.08.020
Hartmann, A., Schmid, M., Van Tuinen, D., and Berg, G. (2009). Plant-driven selection of microbes. Plant Soil 321, 235–257. doi: 10.1007/s11104-008-9814-y
He, D., Xiang, X., He, J. S., Wang, C., Cao, G., Adams, J., et al. (2016). Composition of the soil fungal community is more sensitive to phosphorus than nitrogen addition in the alpine meadow on the Qinghai-Tibetan Plateau. Biol. Fert. Soils 52, 1059–1072. doi: 10.1007/s00374-016-1142-4
Herold, N., Schöning, I., Gutknecht, J., Alt, F., Boch, S., Müller, J., et al. (2014). Soil property and management effects on grassland microbial communities across a latitudinalgradient in Germany. Appl. Soil Ecol. 73, 41–50. doi: 10.1016/j.apsoil.2013.07.009
Herzog, C., Hartmann, M., Frey, B., Stierli, B., Rumpel, C., Buchmann, N., et al. (2019). Microbial succession on decomposing root litter in a drought-prone Scots pine forest. ISME J. 13, 2346–2362. doi: 10.1038/s41396-019-0436-6
Hetrick, B. A. D., Wilson, G. W. T., and Todd, T. C. (1990). Differential responses of C3 and C4 grasses to mycorrhizal symbiosis, phosphorus fertilization, and soil microorganisms. Can. J. Bot. 68, 461–467. doi: 10.1139/b90-061
Hiiesalu, I., Pärtel, M., Davison, J., Gerhold, P., Metsis, M., Moora, M., et al. (2014). Species richness of arbuscular mycorrhizalfungi: associationswith grassland plantrichness and biomass. New Phytol. 203, 233–244. doi: 10.1111/nph.12765
Jacob, M., Weland, N., Platner, C., Schaefer, M., Leuschner, C., and Thomas, F. M. (2009). Nutrient release from decomposing leaf litter of temperate deciduous forest trees along a gradient of increasing tree species diversity. Soil Biol. Biochem. 41, 2122–2130. doi: 10.1016/j.soilbio.2009.07.024
Jaikoo, L., Sangsun, L., and Young, J. P. W. (2010). Improved PCR primers for the detection and identification of arbuscular mycorrhizal fungi. Fems Microbiol. Ecol. 65, 339–349. doi: 10.1111/j.1574-6941.2008.00531.x
Kaiser, C., Kilburn, M. R., Clode, P. L., Fuchslueger, L., Koranda, M., Cliff, J. B., et al. (2015). Exploring the transfer of recent plant photosynthates to soil microbes: mycorrhizal pathway vs direct root exudation. New Phytol. 205, 1537–1551. doi: 10.1111/nph.13138
Kielak, A., Pijl, A. S., Van Veen, J. A., and Kowalchuk, G. A. (2008). Differences in vegetation composition and plant species identity lead to only minor changes in soil-borne microbial communities in a former arable field. FEMS microbiol. Ecol. 63, 372–382. doi: 10.1111/j.1574-6941.2007.00428.x
Körner, C. (2007). The use of ‘altitude’ in ecological research. Trends Ecol. Evol. 22, 569–574. doi: 10.1016/j.tree.2007.09.006
Landis, F. C., and Fraser, L. H. (2008). A new model of carbon and phosphorus transfers in arbuscular mycorrhizas. New Phytol. 177, 466–479. doi: 10.1111/j.1469-8137.2007.02268.x
Lang, F., Bauhus, J., Frossard, E., George, E., Kaiser, K., Kaupenjohann, M., et al. (2016). Phosphorus in forest ecosystems: new insights from an ecosystem nutrition perspective. J. Plant Nutr. Soil Sci. 179, 129–135. doi: 10.1002/jpln.201500541
Li, J., Shen, Z., Li, C., Kou, Y., Wang, Y., Tu, B., et al. (2018). Stair-step pattern of soil bacterial diversity mainly driven by pH and vegetation types along the elevational gradients of Gongga Mountain. China. Front. Microbiol. 9:569. doi: 10.3389/fmicb.2018.00569
Lin, C. F., Yang, Y. S., Guo, J. F., Chen, G. S., and Xie, J. S. (2011). Fine root decomposition of evergreen broadleaved and coniferous tree species in mid-subtropical China: dynamics of dry mass, nutrient and organic fractions. Plant Soil 338, 311–327. doi: 10.1007/s11104-010-0547-3
Lugo, M. A., and Cabello, M. N. (2002). Native arbuscular mycorrhizal fungi (AMF) from mountain grassland (Córdoba, Argentina) I. Seasonal variation of fungal spore diversity. Mycologia 94, 579–586. doi: 10.1080/15572536.2003.11833186
Lugo, M. A., Ferrero, M., Menoyo, E., Estévez, M. C., Siñeriz, F., and Antón, A. (2008). Arbuscular mycorrhizal fungi and rhizospheric bacteria diversity along an elevational gradient in South American Puna grassland. Microb. Ecol. 55:705. doi: 10.1007/s00248-007-9313-3
Marriott, C. A., Hudson, G., Hamilton, D., Neilson, R., Boag, B., Handley, L. L., et al. (1997). Spatial variability of soil total C and N and their stable isotopes in an upland Scottish grassland. Plant Soil 196, 151–162. doi: 10.1023/a:1004288610550
Marschner, P., Solaiman, Z., and Rengel, Z. (2006). Rhizosphere properties of Poaceae genotypes under P-limiting conditions. Plant Soil 283, 11–24. doi: 10.1007/s11104-005-8295-5
Meng, H., Li, K., Nie, M., Wan, J. R., Quan, Z. X., Fang, C. M., et al. (2013). Responses of bacterial and fungal communities to an elevation gradient in a subtropical montane forest of China. Appl. Microbial. Biot. 97, 2219–2230. doi: 10.1007/s00253-012-4063-7
Mukherjee, P. K., Chandra, J., Retuerto, M., Sikaroodi, M., Brown, R. E., Jurevic, R., et al. (2014). Oral mycobiome analysis of HIV-infected patients: identification of pichia as an antagonist of opportunistic fungi. PLoS Pathog. 10:e1003996. doi: 10.1371/journal.ppat.1003996
Nelson, D. W., Sommers, L. E., Sparks, D. L., Page, A. L., Helmke, P. A., Loeppert, R. H., et al. (1982). Total carbon, organic carbon, and organic matter. Methods of Soil Anal. 9, 961–1010.
Osono, T. (2007). Ecology of ligninolytic fungi associatedwith leaf litter decomposition. Ecol. Res. 22, 955–974. doi: 10.1007/s11284-007-0390-z
Osono, T., and Takeda, H. (2001). Organic chemical and nutrient dynamics in decomposing beech leaf litter in relation to fungal ingrowth and succession during 3-year decomposition processes in a cool temperate deciduous forest in japan. Ecol. Res. 16, 649–670. doi: 10.1046/j.1440-1703.2001.00426.x
Pii, Y., Borruso, L., Brusetti, L., Crecchio, C., Cesco, S., and Mimmo, T. (2016). The interaction between iron nutrition, plant species and soil type shapes the rhizosphere microbiome. Plant Physiol. Bioch. 99, 39–48. doi: 10.1016/j.plaphy.2015.12.002
R Core Team (2016). R: A Language and Environment for Statistical Computing. Vienna: R Foundation for Statistical Computing.
Ren, C., Chen, J., Lu, X., Doughty, R., Zhao, F., Zhong, Z., et al. (2018a). Responses of soil total microbial biomass and community compositions to rainfall reductions. Soil Biol. Biochem. 116, 4–10. doi: 10.1016/j.soilbio.2017.09.028
Ren, C., Zhang, W., Zhong, Z., Han, X., Yang, G., Feng, Y., et al. (2018b). Differential responses of soil microbial biomass, diversity, and compositions to elevational gradients depend on plant and soil characteristics. Sci. Total Environ. 610, 750–758. doi: 10.1016/j.scitotenv.2017.08.110
Ren, Y., Liu, M. S., Tian, L. H., Tian, X. H., and Li, Z. J. (2006). Biodiversity, Conversation and Management of Taibaishan Nature Reserve. Beijing: China Forestry Publishing House.
Richardson, A. E., Barea, J. M., McNeill, A. M., and Prigent-Combaret, C. (2009). Acquisition of phosphorus and nitrogen in the rhizosphere and plant growth promotion by microorganisms. Plant Soil 321, 305–339. doi: 10.1007/s11104-009-9895-2
Saitta, A., Anslan, S., Bahram, M., Brocca, L., and Tedersoo, L. (2018). Tree species identity and diversity drive fungal richness and community composition along an elevational gradient in a Mediterranean ecosystem. Mycorrhiza 28, 39–47. doi: 10.1007/s00572-017-0806-8
Saura-Mas, S., Estiarte, M., Penuelas, J., and Lloret, F. (2012). Effects of climate change on leaf litter decomposition across post-fire plant regenerative groups. Environ. Exp. Bot. 77, 274–282. doi: 10.1016/j.envexpbot.2011.11.014
Schlatter, D. C., Bakker, M. G., Bradeen, J. M., and Kinkel, L. L. (2015). Plant community richness and microbial interactions structure bacterial communities in soil. Ecology 96, 134–142. doi: 10.1890/13-.1
Schloss, P. D., Westcott, S. L., Ryabin, T., Hall, J. R., Hartmann, M., Hollister, E. B., et al. (2009). Introducing mothur: open-source, platform-independent, community-supported software for describing and comparing microbial communities. Appl. Environ. Microbiol. 75, 7537–7541. doi: 10.1128/AEM.01541-09
Siles, J. A., and Margesin, R. (2016). Abundance and diversity of bacterial, archaeal, and fungal communities along an elevational gradient in alpine forest soils: what are the driving factors? Microb. Ecol. 72, 207–220. doi: 10.1007/s00248-016-0748-2
Siles, J. A., and Margesin, R. (2017). Seasonal soil microbial responses are limited to changes in functionality at two alpine forest sites differing in altitude and vegetation. Sci. Rep. 7:2204. doi: 10.1038/s41598-017-02363-2
Sorokin, D. Y., Lücker, S., Vejmelkova, D., Kostrikina, N. A., Kleerebezem, R., Rijpstra, W. I. C., et al. (2012). Nitrification expanded: discovery, physiology and genomics of a nitrite-oxidizing bacterium from the phylum Chloroflexi. ISME J. 6, 2245–2256. doi: 10.1038/ismej.2012.70
Stroobants, A., Degrune, F., Olivier, C., Muys, C., Roisin, C., Colinet, G., et al. (2014). Diversity of bacterial communities in a profile of a winter wheat field: known and unknown members. Microb. Ecol. 68, 822–833. doi: 10.1007/s00248-014-0458-6
Sundqvist, M. K., Sanders, N. J., and Wardle, D. A. (2013). Community and ecosystem responses to elevational gradients: processes, mechanisms, and insights for global change. Annu. Rev. Ecol. Evol. Syst. 44, 261–280. doi: 10.1146/annurev-ecolsys-110512-135750
Susan, G., Woodward, S., and Taylor, A. F. S. (2015). Strong elevational partitioning in the distributions of ectomycorrhizal fungi along a short (300 m) elevation gradient. New Phytol. 206, 1145–1155. doi: 10.1111/nph.13315
Tang, Z., Fang, J., Chi, X., Feng, J., Liu, Y., Shen, Z., et al. (2012). Patterns of plant beta-diversity along elevational and latitudinal gradients in mountain forests of China. Ecography 35, 1083–1091. doi: 10.1111/j.1600-0587.2012.06882.x
Tedersoo, L., Bahram, M., Põlme, S., Kõljalg, U., Yorou, N. S., Wijesundera, R. et al. (2014). Global diversity and geography of soil fungi. Science 346, 1078–1078. doi: 10.1126/science.1256688
Tedersoo, L., Bahram, M., Cajthaml, T., Põlme, S., Hiiesalu, I., Anslan, S., et al. (2016). Tree diversity and species identity effects on soil fungi, protists and animals are context dependent. ISME J. 10, 346–362. doi: 10.1038/ismej.2015.116
Van der Heijden, M. G. A., Bardgett, R. D., and van Straalen, N. M. (2008). The unseen majority: soil microbes as drivers of plant diversity and productivity in terrestrial ecosystems. Ecol. Lett. 11, 296–310. doi: 10.1111/j.1461-0248.2007.01139.x
Vanalle, R. M., Ganga, G. M. D., Filho, M. G., and Lucato, W. C. (2017). Green supply chain management: an investigation of pressures, practices, and performance within the Brazilian automotive supply chain. J. Clean. Prod. 151, 250–259. doi: 10.1016/j.jclepro.2017.03.066
Vorísková, J., and Baldrian, P. (2013). Fungal community on decomposing leaf litter undergoes rapid successional changes. ISME J. 2013, 477–486. doi: 10.1038/ismej.2012.116
Wang, S., Ruan, H., and Han, O. (2010). Effects of microclimate, litter type, mesh size on leaf litter decomposition along an elevation gradient in the wuyi mountains, china. Ecol. Res. 25, 1113–1120. doi: 10.1007/s11284-010-0736-9
Wang, W., Wang, C., Sardans, J., Tong, C., Jia, R., Zeng, C., et al. (2015). Flood regime affects soil stoichiometry and the distribution of the invasive plants in subtropical estuarine wetlands in China. Catena 128, 144–154. doi: 10.1016/j.catena.2015.01.017
White, T. J., Bruns, T., Lee, S., and Taylor, J. (1990). “Amplification and direct sequencing of fungal ribosomal RNA genes for phylogen-etics,” in PCR Protocols. A Guide to Methods and Applications, eds M. A. Innis, D. H. Gelfand, J. J. Sninsky, and T. J. White (San Diego, CA: Academic Press), 315e322.
Yuste, J. C., Penuelas, J., Estiarte, M., Garcia-Mas, J., Mattana, S., Ogaya, R., et al. (2011). Drought-resistant fungi control soil organic matter decomposition and its response to temperature. Global Change Biol. 17, 1475–1486. doi: 10.1111/j.1365-2486.2010.02300.x
Keywords: elevational gradients, rhizosphere soil, microbial community, plant properties, soil properties
Citation: Guo Y, Ren C, Yi J, Doughty R and Zhao F (2020) Contrasting Responses of Rhizosphere Bacteria, Fungi and Arbuscular Mycorrhizal Fungi Along an Elevational Gradient in a Temperate Montane Forest of China. Front. Microbiol. 11:2042. doi: 10.3389/fmicb.2020.02042
Received: 26 November 2019; Accepted: 03 August 2020;
Published: 20 August 2020.
Edited by:
Sébastien Duplessis, INRA Centre Nancy-Lorraine, FranceReviewed by:
Jianjun Wang, Nanjing Institute of Geography and Limnology (CAS), ChinaAnthony Stuart Amend, University of Hawai’i at Mānoa, United States
Andre Boraks, University of Hawai’i, United States, in collaboration with reviewer ASA
Copyright © 2020 Guo, Ren, Yi, Doughty and Zhao. This is an open-access article distributed under the terms of the Creative Commons Attribution License (CC BY). The use, distribution or reproduction in other forums is permitted, provided the original author(s) and the copyright owner(s) are credited and that the original publication in this journal is cited, in accordance with accepted academic practice. No use, distribution or reproduction is permitted which does not comply with these terms.
*Correspondence: Fazhu Zhao, zhaofazhu@nwu.edu.cn