- 1Department of Pharmacology, Shanghai Tenth People’s Hospital, Tongji University School of Medicine, Shanghai, China
- 2Department of Laboratory Diagnosis, Shanghai East Hospital, Tongji University School of Medicine, Shanghai, China
- 3Department of Rheumatology, Renji Hospital, School of Medicine, Shanghai Jiao Tong University, Shanghai, China
- 4Department of Critical Care Medicine, Renji Hospital, School of Medicine, Shanghai Jiao Tong University, Shanghai, China
- 5Department of Anesthesiology, Changzheng Hospital, Second Military Medical University, Shanghai, China
Invasive candidiasis (IC) is one of the leading causes of death among immunocompromised patients. Because of limited effective therapy treatment options, prevention of IC through vaccine is an appealing strategy. However, how to induce the generation of direct candidacidal antibodies in host remains unclear. Gpi7 mutant C. albicans is an avirulent strain that exposes cell wall β-(1,3)-glucans. Here, we found that vaccination with the gpi7 mutant strain could protect mice against invasive candidiasis caused by C. albicans and non-albicans Candida spp. The protective effects induced by gpi7 mutant relied on long-lived plasma cells (LLPCs) secreting protective antibodies against C. albicans. Clinically, we verified a similar profile of IgG antibodies in the serum samples from patients recovering from IC to those from gpi7 mutant-vaccinated mice. Mechanistically, we found cell wall β-(1,3)-glucan of gpi7 mutant facilitated Dectin-1 receptor dependent nuclear translocation of non-canonical NF-κB subunit RelB in macrophages and subsequent IL-18 secretion, which primed protective antibodies generation in vivo. Together, our study demonstrate that Dectin-1 engagement could trigger RelB activation to prime IL-18 expression and established a new paradigm for consideration of the link between Dectin-1 mediated innate immune response and adaptive humoral immunity, suggesting a previously unknown active vaccination strategy against Candida spp. infection.
Introduction
Invasive candidiasis (IC) is one of leading death causes among hospitalized individuals, especially immunocompromised patients (Kullberg and Arendrup, 2015; McCarty and Pappas, 2016). It is the fourth most common nosocomial bloodstream infection with a mortality rate about 40–50% (Arendrup, 2010; Yang et al., 2017). Anticandidal therapy is often of limited effectiveness in these patients, resulting in high rates of mortality and morbidity (Arendrup, 2010; Kullberg and Arendrup, 2015; McCarty and Pappas, 2016; Yang et al., 2017). The diagnosis of IC is not always possible due to difficulties in isolating the Candida cells from clinical samples, but clearly defined risk factors, such as immunocompromise, have been established for IC (Clancy and Nguyen, 2013; Candel et al., 2017). Therefore, the prevention of IC through a proven, effective vaccine is an appealing strategy.
Although recent studies have highlighted the crucial roles of TH1 and TH17 cell-mediated immunity in host defense against infection caused Candida spp., both protective immune serum and antibodies have shown remarkable efficacy (Bugli et al., 2013; Drummond et al., 2014; Verma et al., 2014). These findings have special relevance for vaccination, especially in partly or totally immunocompromised individuals. In general, vaccination can induce antibody generation in at-risk patients before they become immunocompromised. In addition, because of the longevity (weeks to months depending on the IgG subclass), these IgG antibodies might persist at a protective titer even during prolonged immunosuppression. However, strategies for directly inducing candidacidal antibody generation in the host have not been developed.
Dectin-1, a spleen tyrosine kinase (Syk)-coupled C-type lectin receptors (CLR) expressed on myeloid cells, recognizes β-(1,3)-glucan on cell surface of various fungi (Taylor et al., 2007). Dectin-1 engagement by β-(1,3)-glucan induces phosphorylation of the immunoreceptor tyrosine-based activation motif-like sequence within the cytoplasmic domain of Dectin-1 (Rogers et al., 2005; Underhill et al., 2005). The subsequent phosphorylation of Syk induces the assembly of caspase recruitment domain 9 (CARD9) protein, the adaptor proteins Bcl-10 and MALT1 (Rogers et al., 2005; Underhill et al., 2005; Gross et al., 2006). The CARD9-Bcl-10-MALT1 scaffold contributes to NF-kB pathway activation and thus helps prime the T helper (TH) cell immune response (Gross et al., 2006; Gringhuis et al., 2009; Drummond et al., 2014; Verma et al., 2014; Xu et al., 2018). Dectin-1 mediated TH1 and TH17 cell immune responses are effective in host defense against fungal infection (Verma et al., 2014). Previous studies suggested that Dectin-1 could prime TH2 cell response by inducing non-canonical NF-kB subunit RelB activation (Gringhuis et al., 2009; Xu et al., 2018). However, little is known about whether TH2 cell response mediated by Dectin-1 can trigger humoral immunity and protective antibodies production in host.
Candida albicans is the most common Candida spp. that can cause invasive infection (Arendrup, 2010; Kullberg and Arendrup, 2015; McCarty and Pappas, 2016; Yang et al., 2017). C. albicans shields surface β-(1,3)-glucan, except in the region between the mature bud and parent yeast cell, for evading host Dectin-1 binding (Gantner et al., 2005; Wheeler et al., 2008). Most cell wall proteins (CWPs) of C. albicans are glycosylphosphatidylinositol (GPI)-anchored proteins (GPI-CWPs), which are linked to cell wall β-(1,6)-glucan through a GPI remnant (Richard and Plaine, 2007; Chaffin, 2008). C. albicans GPI7 is responsible for adding ethanolaminephosphate to the second mannose in GPI anchor biosynthesis and key for the linkage of GPI-anchored protein to the cell wall (Richard et al., 2002; Imhof et al., 2004; Richard and Plaine, 2007). In our previous study, we generated an avirulent C. albicans strain (gpi7 mutant) lacking GPI-CWPs and demonstrated that the surface β-(1,3)-glucan of gpi7 mutant was exposed (Shen et al., 2015). The findings encouraged us to investigate whether vaccination with this avirulent mutant could confer protection against infection caused by wild type C. albicans.
This study found that the gpi7 mutant C. albicans with surface β-(1,3)-glucan exposure could induce hosts to generate protective antibodies against IC in mice and demonstrated that IL-18 plays a central role in the type 2 response contributing to this immunoprotection. The mechanistic analysis revealed that Dectin-1 engagement by surface β-(1,3)-glucan of the gpi7 mutant could facilitate activation of the non-canonical NF-kB subunit RelB, and this activation regulates IL-18 expression to prime the type 2 response. Clinically, we verified a similar profile of IgG antibodies in serum samples from patients recovering from IC to those of gpi7 mutant-vaccinated mice.
Materials and Methods
Reagents, Antibodies and Plasmids
Coomassie brilliant blue, IPTG and DTT were purchased from Sangon Biotech. The p65 inhibitor helenalin was purchased from Abcam. RelB inhibitor 1,25(OH)2D3 and HF-pyridine was purchased from Merck. Zymosan were purchased from sigma. Ni-nitrilotriacetic acid (Ni-NTA) were purchased from QIAGEN. V450-conjugated anti-B220 antibody (Clone RA3-6B2, BioLegend), Phycoerythrin-anti-CD44 antibody (Clone MEM-85, BioLegend), FITC-conjugated anti-MHCII antibody (Clone M5/114.15.2, BioLegend), Allophycocyanin-conjugated anti-CD138 antibody (Clone 281-2, BioLegend), Allophycocyanin-conjugated anti-CD80 antibody (Clone 16-10A1, BioLegend), FITC-conjugated anti-CD273 antibody (Clone 122, Invitrogen), Phycoerythrin-conjugated-CD73 antibody (Clone TY/11.8, BioLegend), Anti-mouse IL-4 Antibody (Cat# ab93503, Abcam), and Alexa-488-labeled goat anti-mouse antibody (Cat# A11059, Life Technologies) were used in flow cytometry (isotype antibodies were used as controls). The neutralizing anti-CD4 antibody was purchased from Invitrogen. And the neutralizing anti-IL-17A antibody and anti-IFN-γ antibody were purchased from BioLegend. The neutralizing anti–IL-18 antibody was purchased from R&D Systems. Antibodies against RelB and PCNA were obtained from Cell Signaling Technologies. pET-21a (+) was obtained from Novagen.
Mice
Female C57BL/6 and BALB/c background athymic nude mice were obtained from Shanghai Laboratory Animal Center (SLAC) of the Chinese Academy of Sciences (Shanghai, China). Dectin-1-deficient (Dectin-1–/–) mice were kindly provided by Dr. Gordon D. Brown (the mice were backcrossed for nine generations on the C57BL/6 background).
Strain Growth Conditions
gpi7 mutant C. albicans was generated in our previous study (Shen et al., 2015; Zhang et al., 2016). C.albicans SN152 was kindly provided by Dr. Noble SM (University of California-San Francisco). C. albicans SC5314 was kindly provided by Sanglard D (Centre Hospitalier Universitaire Vaudois). The clinical isolate C. albicans 0304103 was kindly provided by Dr. Jun Gu (Changhai Hospital, Shanghai, China), and ssa1 mutant C. albicans was kindly provided by Dr. Filler SG (State University of New York) (Sun et al., 2010). C. glabrata ATCC28226, C. parapsilosis ATCC 22019, C. krusei ATCC 6258 and C. tropicalis ATCC 750 were obtained from ATCC.
Sabouraud dextrose agar (SDA) plates (4% dextrose,1% peptone, and 1.8% agar) were used for isolation of individual Candida clones. Individual Candida clones were cultured in yeast peptone dextrose (YPD) liquid medium (2% dextrose, 2% peptone, and 1% yeast extract) with shaking incubator at 30°C. For C. albicans heat-inactivation, C. albicans SN152 (2.5 × 107/ml) were boiled in PBS for 10 min. BL21 (DE3) pLysS was purchased from Tiangen Biotech, and cultured on LB plates (1%NaCl, 1% tryptone, 0.5% yeast extract, 1.5% Agar) for isolation of individual clones and cultured in LB liquid medium at 37°C in a shaking incubator for C. albicans proteins expression.
Serum Samples and Ethics Statement
Ten serum samples were collected from recovering IC patients, and 10 serum samples were taken from deceased IC patients with clinical and microbiological evidence in intensive care unit (ICU) of Shanghai Tenth People’s Hospital and Changzheng Hospital (Shanghai, China) and were saved at −80°C. The patients for serum samples collecting were age 50–70 years old, and the neutropenia patients (absolute neutrophil count <500 cells/mm3) were excluded from our study. The iatrogenic risk factors of the included IC patients included broad-spectrum antibiotics (5, 25%), central venous catheters (4, 20%), parenteral nutrition (6, 30%), and abdominal or thoracic surgery (5, 25%). This study was approved by the Review Board and Ethics Committee (Permit number: 2018-Clinical-Res-172).
Murine Invasive Candidiasis Model
For invasive Candida spp. infection in vivo, C57BL/6 or athymic nude (BALB/c background) female mice were intravenously infected with 200 μl of live Candida cells in sterile saline. The mice were daily monitored for survival or sacrificed at day 2 post-infection for fungal burden assessment and pathological examination. The livers and kidneys were collected and fixed in neutral formalin (10%) for H&E and PAS staining or homogenized in 0.5 ml of PBS for fungal burden assay. Homogenate supernatants of kidney were collected and stored at −80°C until cytokines measurements.
Vaccination and Candida spp. Reinfection Models
C57BL/6 female mice, 6–8 weeks old, were intravenously vaccinated with live gpi7 mutant (5 × 105 CFU per mouse) at day 1 and day 14, and re-infected with wild-type Candida spp. at day 28. UV-inactive parental strain C. albicans SN152-vaccination was used as control. To investigate the role of TH1 and TH17 mediated immune response in gpi7 mutant induced protective effect against Candida spp. infection, gpi7 mutant-vaccinated mice were intravenously injected with anti-IFN-γ antibody (clone XMG1.2, 500 μg per mouse) or anti-IL-17A antibody (clone TC11-18H10.1, 100 μg per mouse).
Cytokines Measurement
Concentrations of TNF-α, IL-6, IL-4, IL-13, and IL-18 in spleen and serum homogenates and concentrations of IL-17 and IFN-γ in kidney homogenates were assayed under manufacturer’s instructions by Ready-Set-Go cytokine kits (Invitrogen) in triplicates.
Flow Cytometry Analysis
The spleen and bone marrow of mice were homogenized in 1 ml PBS and were subsequently passed through a 70-mm filter. For leukocytes isolation, the mixture cells were centrifuged in a 40%/70% percoll gradient (Huang et al., 2016). Cells were counted and blocked with PBS containing 5% heat-inactivated FBS and 1 mM sodium azide at 4°C. The cells were then incubated with V450-conjugated anti-B220, phycoerythrin-anti-CD44, allophycocyanin-conjugated anti-CD138 and FITC-conjugated anti-MHCII antibodies to detect LLPCs or V450-conjugated anti-B220, FITC-conjugated anti-CD273 antibodies, allophycocyanin-conjugated anti-CD80 and phycoerythrin-conjugated-CD73 antibodies to detect Bmem in a binding buffer at room temperature for 20 min. After being fixed with 1% formaldehyde in PBS, a flow cytometry (BD FACSVerse) were used to evaluate LLPCs and Bmem cells.
Confocal Laser Scanning Microscopy
To analyze antibodies in gpi7 mutant-induced antiserum that bind to epitopes on the surface of C. albicans, exponentially growing C. albicans SC5314 yeast cells were incubated with serum samples from gpi7 mutant-vaccinated mice at 4°C overnight and then stained with alexa-488-labeled goat anti-mouse secondary antibodies (Life Technologies) for 1 h at 30°C. A confocal laser scanning microscope (TCS SP5; Leica) were used to scanned the stained cells (63 × magnification). LAS AF Lite program was used to acquire and analysis micrograph images.
Identification of Immunogenic Proteins on Cell Wall of Gpi7 Mutant
Covalent and non-covalent C. albicans CWPs were isolated according to previously published methods (Zhang et al., 2016). The CWPs of C. albicans mainly included GPI-APs, non-covalent CWPs and Pir proteins (proteins with internal repeats). The cell wall debris were treated by undiluted HF-pyridine at 0°C for 3 h for GPI-CWPs releasing. Then the cell wall debris were treated by 30 mM NaOH at 4°C for 16 h for Pir proteins specifically releasing with. The non-covalent CWPs of C. albicans were extracted in an SDS buffer (10 mM DTT, 100 mM EDTA, 2% SDS, 50 mM Tris–HCl, and; pH 8.0). All the CWPs were then mixed for a subsequent experiment.
To determine whether gpi7-induced antiserum can specifically detect proteins from C. albicans, the whole CWPs mixture from wild-type C. albicans was fracted by SDS-PAGE and then subjected to immunoblotting analysis using gpi7-induced antiserum or wild-type C. albicans SN152-induced serum. Total CWPs from wild-type C. albicans stained with Coomassie brilliant blue on SDS-PAGE was used as a control. Then, the gel bands of different proteins were isolated from the control lane for LC-MS/MS analysis by high-resolution instruments (LTQ-Orbitrap XL and Velos, Thermo Fisher Scientific). MaxQuant software was used for peptide/protein identification from the raw files.
Expression and Purification of Cell Wall-Localized Moonlighting Proteins of C. albicans
The genes encoding cell wall-localized moonlighting proteins were amplified from C. albicans SC5314 genomic DNA (the primers were listed in Supplementary Table S1) and cloned into pET-21a (+), which includes a 6 × His tag. The plasmid was then transformed into BL21 (DE3) pLysS cells to express His-fused moonlighting proteins. The transformants were cultured at 37°C for 12 h and diluted in fresh LB medium (1:100). Once the optical density at 600 nm (OD600) of the medium reached 0.6, the cells were grown for 16 h at 16°C in LB medium containing 0.1 mM IPTG. The Ni-nitrilotriacetic acid (Ni-NTA) (QIAGEN) were used to purified the His-fused moonlighting proteins.
Determination of the IgG Titers in Antiserum
After coating 96-well microtiter plates with a recombinant C. albicans moonlighting protein (0.1 μg per well), the IgG titers in serum samples from gpi7 mutant-vaccinated mice or IC patients were determined by indirect ELISA. The relative amounts of IgG are shown in a heat map produced using Heml 1.0.3.3 (heatmap illustrator).
Thioglycollate-Elicited Peritoneal Neutrophils and Macrophages Preparation
Thioglycollate-elicited peritoneal neutrophils and macrophages were harvested as previously published method (Taylor et al., 2007). Thioglycollate (Merck, 3%, wt/vol) were injected into the abdominal cavity of C57BL/6 mice (2 ml per mouse). Peritoneal cells were harvested through washing with PBS containing 0.5 mM EDTA 14 h later for neutrophils or 72 h later macrophages collection. The neutrophils and macrophages were cultured in RPMI1640 containing FBS (10%, vol/vol).
Serum Opsonophagocytic Phagocytosis and Killing Assay
For phagocytosis assay, C. albicans SC5314 was incubated with 1 × 106 peritoneal macrophages (MOI = 0.4) accompanied with diluted gpi7-induced antiserum at 37°C for 1 h. After washed with PBS, unbound C. albicans SC5314 cells were removed and the mixture was plate on SDA agar at 30°C for live C. albicans counted after 48 h incubation.
For neutrophils killing assay, C. albicans SC5314 (1 × 104 CFU) were incubated with diluted gpi7-induced antiserum for 1 h at 30°C. The cells of C. albicans SC5314 were collected and incubated with thioglycolate-elicited peritoneal neutrophils at MOI = 0.05 for 1 h at 4°C to make the cells settle, and then transferred to 37°C for further 1 h for killing test. Control plates were placed at 4°C in parallel during the incubation, then the mixture was plated on SDA agar at 30°C for 48 h and the live C. albicans were counted.
Quantitative Real-Time RT-PCR
Total RNA was isolated using TRIzol (Invitrogen) and reverse transcribed using SuperScript III (Invitrogen). Power SYBR Green PCR Master Mix (TaKaRa, RR420A) was then used for quantitative real-time PCR. The amounts of transcript were normalized to those for Gapdh. The primers used in this assay are listed in Supplementary Table S2.
Immunoblotting Assays
Total cell lysates were extracted by (250 mM NaCl, 1 mM EDTA, 1% NP-40, 50 mM HEPES, protease inhibitors, pH 7.4). Nuclear extracts were extracted by lysis buffer (0.1 mM EDTA, 10 mM HEPES, 10 mM KCl, 0.4% NP-40, protease inhibitors, pH 7.9). Nuclear extracts or total cell lysates were subjected to SDS-PAGE, blotted with the indicated primary antibodies and secondary antibodies, and then developed using an ECL detection system (GE Healthcare).
Quantification and Statistical Analysis
All experiments performed three biological replicates unless otherwise indicated. Log-rank test was used for survival data analysis. For parametric data, two groups were analyzed by two-tailed Student’s t-test and multiple groups were analyzed by one-way analysis of variance (ANOVA). For non-parametric data, the non-parametric t-test or ANOVA was used. Statistical significance was set at a P-value of 0.05, 0.01 or 0.001.
Results
gpi7 Mutant-Vaccination Protects Mice From IC
In previous studies, we constructed C. albicans gpi7 mutant of which cell surface β-(1,3)-glucan was exposed (Shen et al., 2015). A series of vaccination experiments were performed according to the strategies shown in Figure 1A. Gpi7 mutant-vaccinated mice or parental strain SN152 (UV-inactivated)-vaccinated mice were subsequently systemically infected with a lethal dose of C. albicans SC5314 (Figures 1B–F) or clinical isolate C. albicans 0304103 (Figure 1G). When challenged with a lethal dose of C. albicans SC5314, the gpi7 mutant-vaccinated mice presented a markedly higher survival rate than those vaccinated with the parental strain SN152 (Figures 1B,C). Over a 40-day observation period, two gpi7 mutant-vaccinated mice infected by 5 × 105 CFU C. albicans SC5314 died (Figure 1B), and half of the gpi7 mutant-vaccinated mice infected by 1 × 106 CFU C. albicans SC5314 died (Figure 1C). In contrast, all the mice vaccinated with the parental strain SN152 and challenged with C. albicans SC5314 died within 20 days (Figures 1B,C). At day 2 and 7 post-infection, gpi7 mutant-vaccinated mice had significantly lower kidney and liver fungal burdens than those vaccinated with parental strain SN152 (Figures 1D,E and Supplementary Figure S1). Moreover, hematoxylin and eosin (H&E) staining identified fewer inflammatory cell influx and ameliorated tissue necrosis in the kidneys in the gpi7 mutant-vaccinated mice (Figure 1F, top panel). Periodic acid-Schiff (PAS) staining also revealed fewer hyphae in the kidneys of the gpi7 mutant-vaccinated mice than in those of the parental strain SN152-vaccinated mice (Figure 1F, bottom panel). In terms of systemically infection by the clinically isolated C. albicans 0304103 strain which is more virulent than C. albicans SC5314, gpi7 mutant-vaccination could significantly extent the median survival time of mice, while did not significantly improve the long-term survival (Figure 1G). This result suggested that the level of protection elicited by gpi7 mutant-vaccination is strain-dependent (mainly related with the pathogenicity of the infected strain).
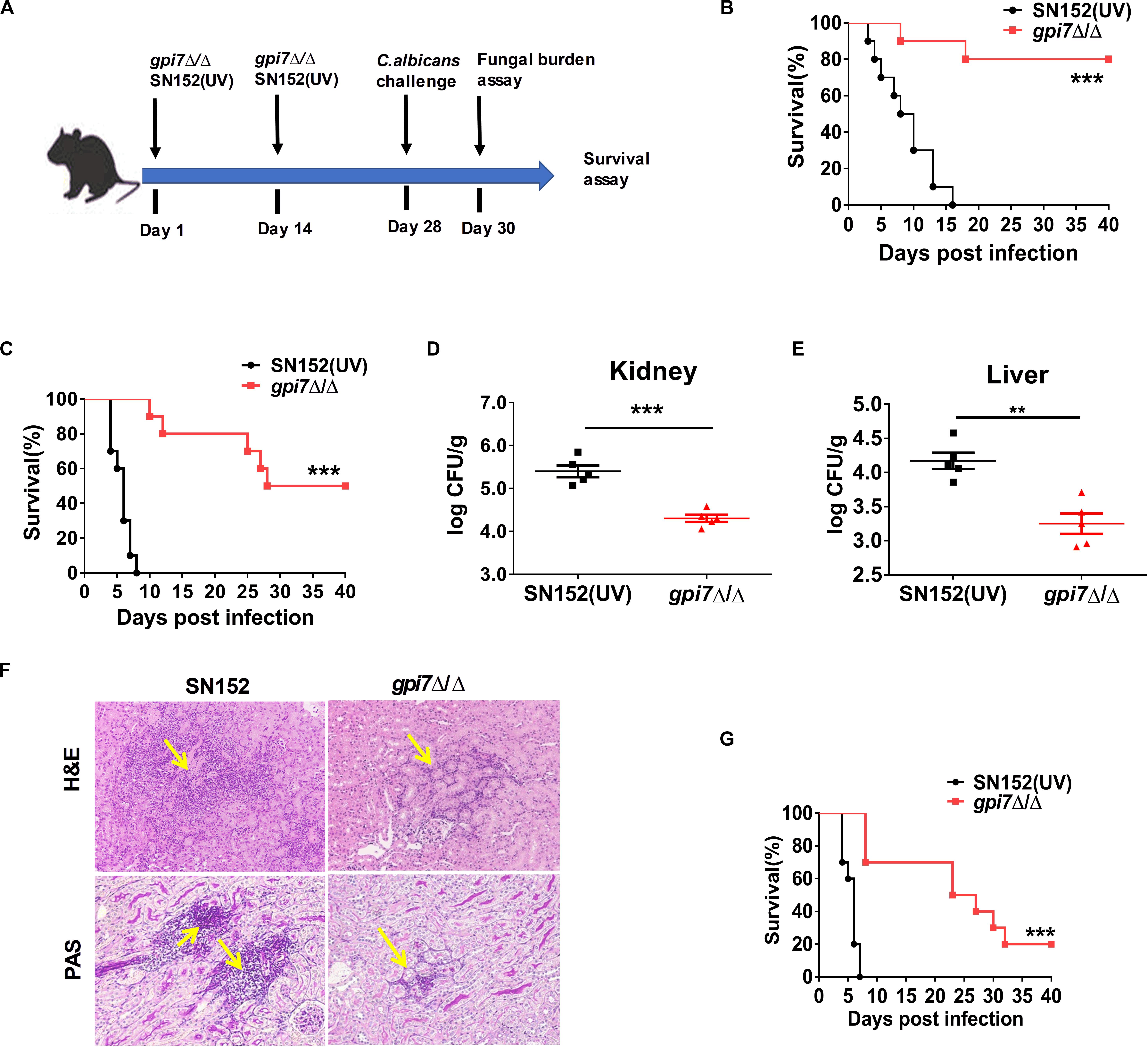
Figure 1. Gpi7 mutant-vaccination protects mice from invasive C. albicans infection. (A) The strategy for gpi7 mutant-vaccination and wild-type C. albicans reinfection. C57BL/6 mice were intravenously vaccinated with live gpi7 mutant (5 × 105 CFU per mouse) at day 1 and day 14, and re-infected with wild-type C. albicans at day 28. UV-inactive parental strain C. albicans SN152 vaccination was used as control. The kidney and liver fungal burden was determined at day 2 post-infection and survival was surveyed for 40 days’ post-infection. (B,C) The survival of gpi7 mutant-vaccinated C57BL/6 mice were monitored for 40 days’ post systemically infected with C. albicans SC5314 (B, 5 × 105 CFU per mouse; C, 1 × 106 CFU per mouse) (n = 10 per group). Data are representative of three independent experiments. (D,E) The kidney fungal burden (D) and liver fungal burden (E) of gpi7 mutant-vaccinated C57BL/6 mice at day 2 post systemically infected with C. albicans SC5314 (5 × 105 CFU per mouse) (n = 5 per group). Data are representative of three independent experiments. (F) Representative H&E (for the inflammatory cells influx and the extent of tissue necrosis) and PAS (for C. albicans) staining of kidneys from gpi7 mutant-vaccinated C57BL/6 mice at day 2 post systemically infected with C. albicans SC5314 (5 × 105 CFU per mouse). Arrows indicate inflammatory cells influx and tissue necrosis (H&E staining), and C. albicans filaments in the tissues (PAS staining). Magnification = 200 ×. (G) Survival of gpi7 mutant-vaccinated C57BL/6 mice were monitored for over 40 days post systemically infected with clinical isolated C. albicans 0304103 (1 × 106 CFU per mouse) (n = 10 per group). Data are representative of three independent experiments. **P < 0.01; ***P < 0.001 [Log-rank test (B,C,G) and non-parametric t-test (D,E)].
Although C. albicans is the most common Candida spp., there has been an increase of infections caused by non-albicans Candida spp. (Parmeland et al., 2013; Fu et al., 2017). Therefore, gpi7 mutant-vaccinated mice were also subsequently systemically infected with non-albicans Candida spp., including Candida glabrata, Candida krusei, Candida parapsilosis and Candida tropicalis. At day 2 post-infection, the gpi7 mutant-vaccinated mice had significantly lower fungal burdens in the kidneys than those mice vaccinated with parental strain SN152, which indicated that the gpi7 mutant provided markedly improved immunoprotection (Figure 2).
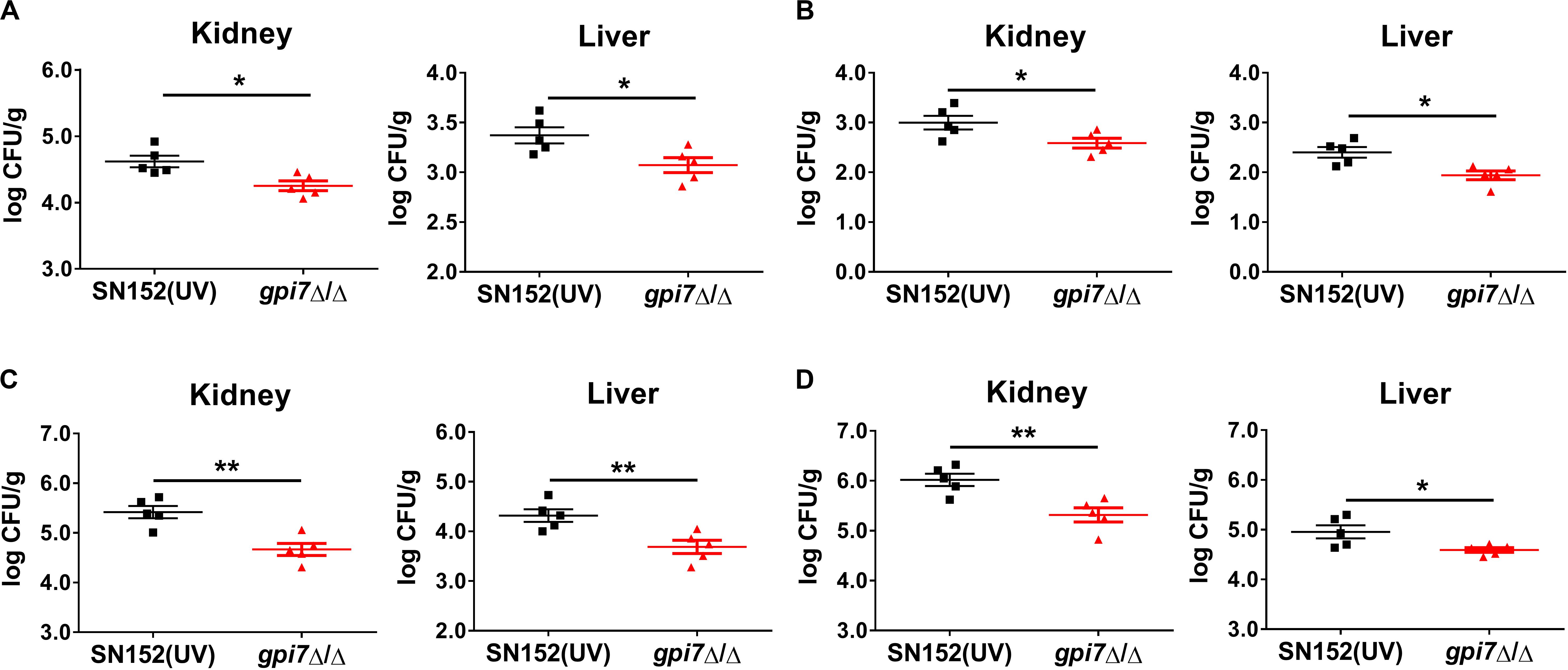
Figure 2. Gpi7 mutant-vaccination protects mice from invasive infections caused by non-albicans Candida spp. Gpi7 mutant-vaccinated C57BL/6 mice were systemically infected with non-albicans Candida spp. (n = 5 per group). And the kidney fungal burden was determined at day 2 post-infection. (A) Kidney and liver fungal burden of gpi7 mutant-vaccinated mice infected with C. glabrata ATCC 28226 (1 × 107 CFU per mouse). (B) Kidney and liver fungal burden of gpi7 mutant-vaccinated mice infected with C. krusei ATCC 6258 (1 × 107 CFU per mouse). (C) Kidney and liver fungal burden of gpi7 mutant-vaccinated mice infected with C. parapsilosis ATCC 22019 (1 × 106 CFU per mouse). (D) Kidney and liver fungal burden of gpi7 mutant-vaccinated mice infected with C. tropicalis ATCC 750 (1 × 106 cells per mouse). Data are representative of three independent experiments. *P < 0.05; **P < 0.01 (Non-parametric t-test).
Furthermore, we examined whether prior exposure to another avirulent ssa1 mutant without cell surface β-(1,3)-glucan exposure (Sun et al., 2010) could confer protection to infection caused by wild type C.albicans. However, after challenge with C. albicans SC5314, the fungal burdens in the kidneys and liver of ssa1 mutant-vaccinated mice were similar to those of mice vaccinated with its complemental strain (Supplementary Figure S2). These results demonstrated that cell surface β-(1,3)-glucan exposure is critical for C. albicans induced host immunoprotection.
LLPC Mediated Humoral Immunity Is Involved in the Immunoprotection Induced by Gpi7 Mutant
To determine whether innate or adaptive immunity plays a central role in the protective effect of gpi7 mutant vaccination, we performed vaccination experiments with athymic nude mice. After infected with a lethal dose of C. albicans SC5314, the fungal burdens in the kidney and liver of the gpi7 mutant-vaccinated athymic nude mice were similar to those of the parental strain SN152-vaccinated mice, which suggested that immunoprotection is dependent on the thymus and that adaptive immunity plays a necessary role in this process (Figure 3A).
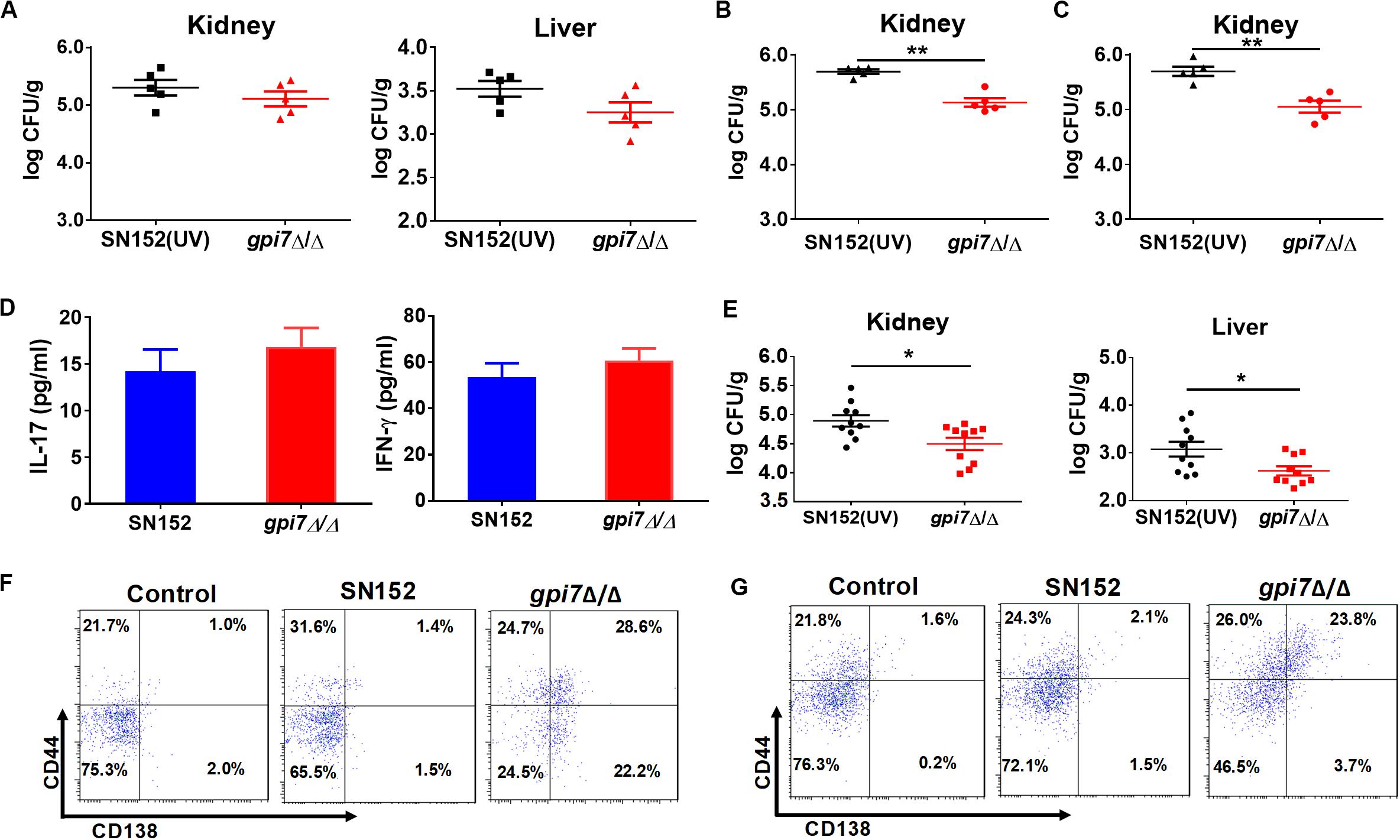
Figure 3. LLPCs mediated humoral immunity is involved in the immunoprotection induced by gpi7 mutant. (A) Gpi7 mutant-vaccinated athymic nude mice were systemically infected with C. albicans SC5314 (5 × 105 CFU per mouse, n = 5 per group). And the kidney and liver fungal burdens were determined at day 2 post-infection. Data are representative of three independent experiments. (B,C) Gpi7 mutant-vaccinated C57BL/6 mice treated with anti-IFN-γ antibody (500 μg per mouse) (B) or of anti-IL-17A (100 μg per mouse) (C) were systemically infected with C. albicans SC5314 (5 × 105 CFU per mouse, n = 5 per group). And the kidney burden was determined at day 2 post-infection. Data are representative of three independent experiments. (D) ELISA assays for IL-17 and IFN-γ in homogenized kidneys from gpi7 mutant-vaccinated C57BL/6 mice at day 2 post systemically infected with C. albicans SC5314 (n = 8 per group). Data are means ± SD and are representative of three independent experiments. (E) Two hours before systemically infected with C. albicans SC5314 (5 × 105 cells per mouse), C57BL/6 mice were treated by 100 μl gpi7 mutant-induced antiserum. And the kidney and liver fungal burdens were determined at day 2 post-infection (n = 10 per group). Data are means ± SD and are representative of three independent experiments. (F,G) Flow cytometry for B220+CD44+CD138+MHCII– LLPCs in the spleen (F) and bone marrow (G) of gpi7 mutant-vaccinated C57BL/6 mice. Data are representative images of 5 mice. *P < 0.05; **P < 0.01 (Non-parametric t-test).
CD4+ TH1 and TH17 cell-mediated immunity plays important roles in host defense against fungal infection (Drummond et al., 2014; Verma et al., 2014). Interferon gamma (IFN-γ) and IL-17 are the key cytokines produced by Th1 and Th17 cells, respectively. Therefore, we abolished TH1 and TH17 cell-mediated immunity in gpi7 mutant-vaccinated mice by neutralizing anti-IFN-γ and anti-IL-17 antibodies. And the efficacy of anti-IFN-γ antibody and anti-IL-17A antibody were confirmed by detecting serum IFN-γ and IL-17A by ELISA (Supplementary Figure S3).
The results demonstrated that neutralizing anti-IFN-γ antibody (Figure 3B) or anti-IL-17 antibody (Figure 3C) did not affect the immunoprotection against C. albicans infection induced by gpi7 mutant vaccination. Next, TH1 and TH17 cell mediated immune responses were also evaluated by measuring IFN-γ and IL-17 levels in the kidneys of gpi7 mutant-vaccinated mice at day 2 post C. albicans SC5314 challenge, and no difference was found between the gpi7 mutant- and parental strain SN152-vaccinated mice (Figure 3D). The above results suggested that TH1 and TH17 cell-mediated immunity were not involved in the protective effect induced by gpi7 mutant vaccination.
We subsequently investigated the role of B cell-mediated humoral immunity in the protective effects inducted by gpi7-mutant vaccination. An adoptive immunization experiment demonstrated that the serum from gpi7 mutant-vaccinated mice could protect mice from systemic C. albicans infection (Figure 3E). This result suggested that B cell-mediated humoral immunity played a critical role in gpi7 mutant induced protective effect. Memory in humoral immunity depends on the differentiation and maintenance of memory B cells (Bmem) or LLPCs. Our results revealed that the number of B220+CD44+CD138+MHCII– LLPCs in the bone marrow (Figure 3F) and spleen (Figure 3G) were higher in the gpi7 mutant-vaccinated mice than in the parental strain SN152-vaccinated mice. However, no difference in B220+ CD273+CD80+CD73+ Bmem cells were found between the gpi7 mutant- and parental strain SN152-vaccinated mice (Supplementary Figure S4). These results suggested that maintenance of the protective effect against infection induced by gpi7 mutant vaccination can be mainly attributed to LLPCs.
Gpi7 Mutant Vaccination Induces IgG Antibody Production Targeting Cell Wall-Localized Moonlighting Proteins of C. albicans in Mice
To investigate the mechanism though which gpi7 mutant-vaccination induced immunoprotection, we determined whether gpi7 mutant vaccination could induce specific anti-C. albicans antibodies in mice. Indeed, we found that serum samples from gpi7 mutant-vaccinated mice contained specific antibodies that recognized the surface of C. albicans, as demonstrated by confocal laser scanning microscopy, whereas serum samples from parental strain SN152-vaccinated mice only contained very low levels of anti-C. albicans antibodies (Figure 4A).
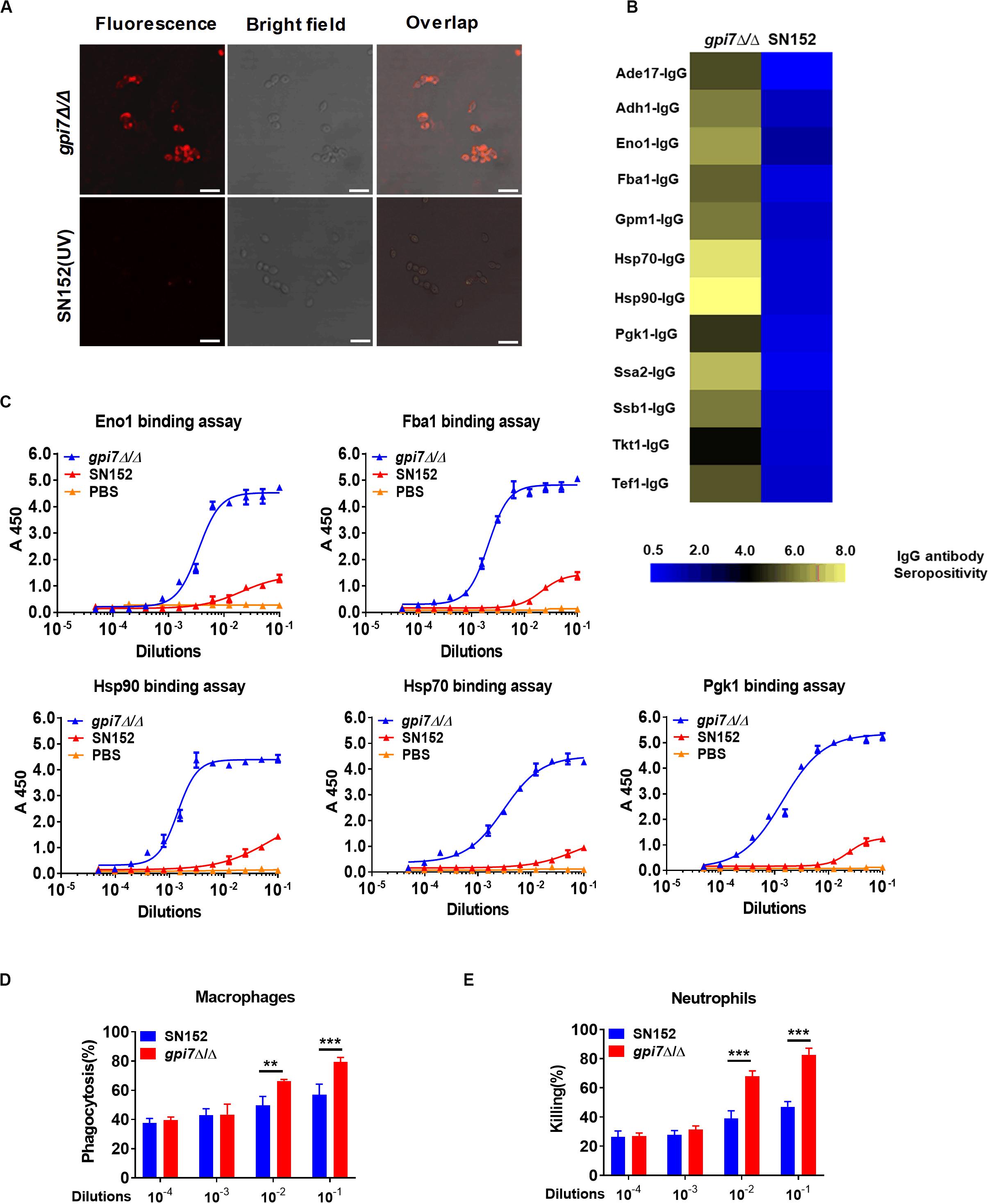
Figure 4. Gpi7 mutant-vaccination induces IgG antibodies production targeting cell wall-localized moonlighting proteins of C. albicans. (A) Representative confocal microscopic analysis for IgG antibodies in gpi7 mutant-induced antiserum from mice binding epitopes on the surface of C. albicans SC5314. Scale bar represents 10 μm. (B) Heat map of the signal intensity of IgG antibodies in gpi7 mutant-induced antiserum binding to cell wall moonlighting proteins of C. albicans SC5314 was shown by Heml 1.0.3.3 (Heatmap illustrator). The IgG antibody response signals were defined as the ratio of gpi7 mutant or parental strain SN152 induced antiserum antibody titers versus normal serum from mice. The response for each IgG antibody is color-coded, as described in the color bar. (C) The represent IgG antibody titers in gpi7 mutant or parental SN152-induced antiserum were evaluated by ELISA method. (D) Thioglycolate-induced peritoneal macrophage phagocytosis of C. albicans. C. albicans SC5314 was co-cultured with peritoneal macrophages (MOI = 0.4) and the indicated dilution of gpi7-induced antiserum at 37°C for 1 h. The suspension was then plated on SDA agar for 48 h, after which C. albicans colonies were counted. (E) Thioglycolate-induced peritoneal neutrophil-mediated killing of C. albicans. C. albicans SC5314 were co-cultured with peritoneal neutrophils (MOI = 0.05) with the indicated dilution of gpi7-induced antiserum at 37°C for 1 h. The suspension was then plated on SDA agar for 48 h, after which C. albicans colonies were counted. **P < 0.01; ***P < 0.001 [(One-way ANOVA (D,E)].
To determine whether gpi7 mutant-induced antibodies in mice can specifically recognize proteins from C. albicans, lysates from C. albicans SC5314 cell wall were fracted by SDS-PAGE and then subjected to immunoblotting analysis using gpi7 mutant or parental strain SN152-induced antiserum. Cell wall lysates from C. albicans SC5314 stained with coomassie brilliant blue staining on SDS-PAGE were used as a control. We found that the gpi7 mutant-induced antiserum detected proteins in cell wall lysates from C. albicans SC5314 were different from those detected by SN152-induced antiserum (Figure 5). LC-MS/MS analysis for the gel bands of the different proteins which isolated from the control lane identified 22 different representative cell wall proteins of C. albicans (Supplementary Table S3). And we found that most of these proteins were cell wall-localized moonlighting proteins, including glycolytic enzymes on the cell wall (Fba1, Pgk1, and Eno1), heat shock proteins (Hsp90, Hsp70, and the HSP70 family chaperones Ssa2 and Ssb1), oxidative stress proteins (Cat1), and general metabolic enzymes (Ade17, Adh1, Ach1, and Tkt1) (Supplementary Table S3). The results indicated that gpi7 mutant-vaccination could protect against C. albicans or non-albicans Candida spp. caused systematic infection, suggesting that the proteins targeted by the antibodies produced after gpi7 mutant-vaccination may share similarity among Candida spp. The sequence alignment results in Supplementary Figure S5 validated the high similarity of the protective antibody targeting moonlighting proteins (Eno1, Hsp90, Fba1, Hsp70, Pgk1, Ssa2, Ssb1, Ade17, Ach1).
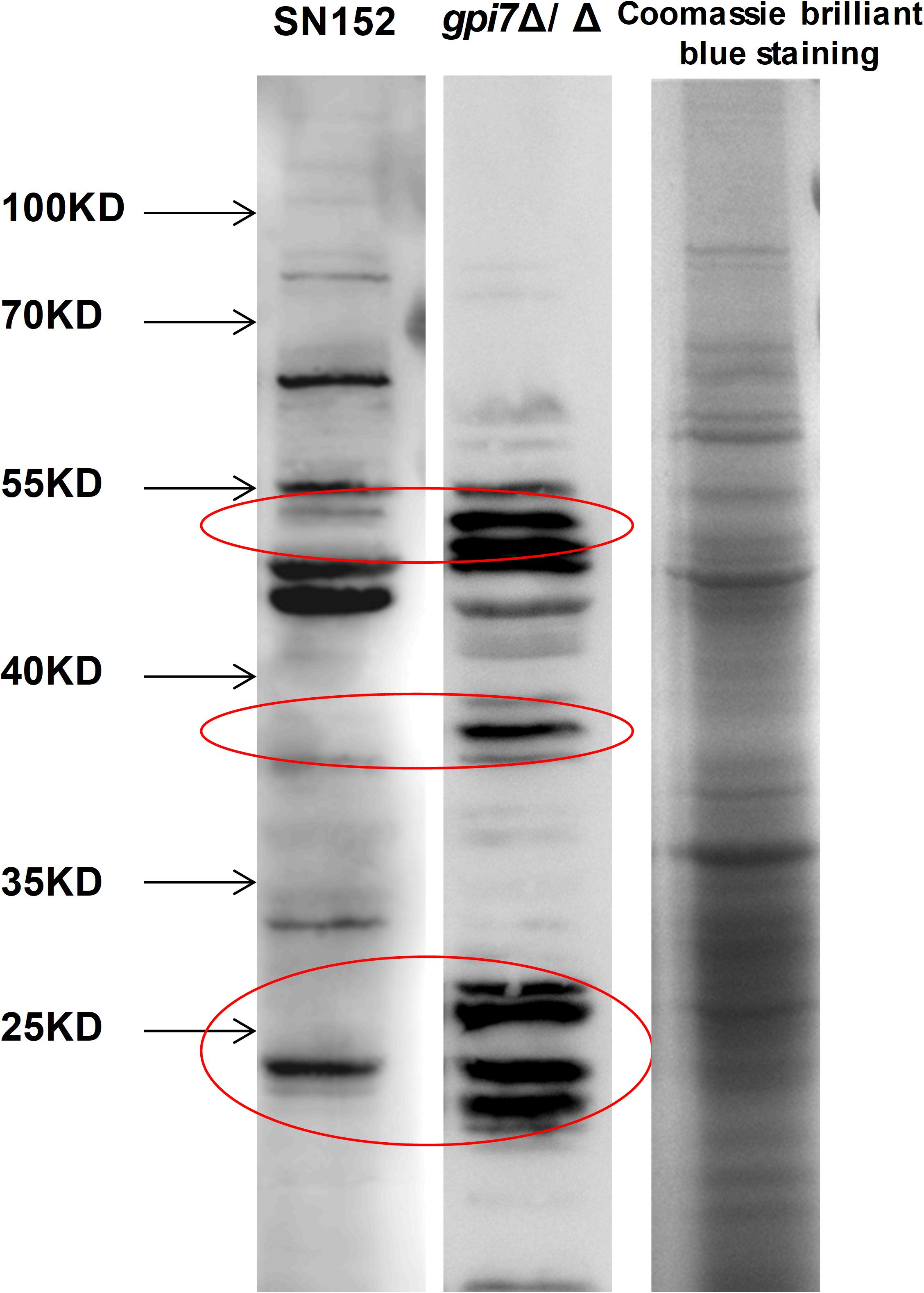
Figure 5. The whole CWP mixture from wild-type C. albicans was fracted by SDS-PAGE and then subjected to immunoblotting analysis using gpi7 mutant or parental strain SN152-induced antiserum. Cell wall lysates from C. albicans SC5314 with coomassie brilliant blue staining on SDS-PAGE were used as a control. Parental strain SN152-induced antiserum detection (Band 1) or gpi7-induced antiserum detection (Band 2). Total CWPs from wild-type C. albicans fracted by SDS-PAGE and stained with Coomassie brilliant blue (Band 3).
The above-identified moonlighting proteins were produced by a bacterial expression system and were further used to investigate their IgG antibody titers in gpi7 mutant-induced antiserum samples through ELISA. The results demonstrated that the titers of IgG antibodies targeting 12 cell wall-localized moonlighting proteins were markedly higher in gpi7 mutant-induced antiserum samples than in those induced by SN152 strain (Figure 4B). In addition, the titers of representative IgG antibodies targeting cell wall-localized moonlighting proteins (Eno1, Fba1, Hsp90, Hsp70, and Pgk1) were shown in Figure 4C. Furthermore, we found the representative IgG antibodies could maintain higher titers until 56 days post vaccination (Supplementary Figure S6).
Neutrophils and macrophages are the primary leukocytes responsible for phagocytosis and killing of invasive fungal cells. Antibody binding to invading fungal cells can induce neutrophil or macrophage-mediated opsono-phagocytosis and opsono-killing. Serum samples from gpi7 mutant-vaccinated mice mediated fungal opsono-phagocytosis and opsono-killing, as demonstrated by incubating C. albicans with macrophages and neutrophils. We found that treatment with gpi7 mutant-induced antiserum exhibited dose-dependent opsono-phagocytosis and opsono-killing activity when macrophages and neutrophils challenged by C. albicans (Figures 4D,E).
The Reactivity Differences Among Serum IgG Antibodies Targeting Cell Wall-Localized Moonlighting Proteins Are Associated With the Prognosis of IC Patients
Ten serum samples were collected from recovered IC patients with clinical and microbiological evidence of C. albicans infection, and 10 serum samples were collected from deceased IC patients. We detected the IgG antibody response targeting cell wall-localized moonlighting proteins in serum samples from IC patients. The results indicated that the titers of 12 immunodominant antibodies targeting cell wall-localized moonlighting proteins of C. albicans in the serum samples from recovering IC patients were markedly higher than those from the deceased IC patients (Figure 6A). The titers of the representative IgG antibodies targeting cell wall-localized moonlighting proteins (Eno1, Fba1, Hsp90, Hsp70, and Pgk1) in serum samples from the recovering IC patients were shown in Figure 6B. Meanwhile, control serum from healthy people did not show significant responsiveness to the above wall-localized moonlighting proteins (Supplementary Figure S7). These data showed that the IgG antibody response targeting cell wall-localized moonlighting proteins in the serum samples of recovering IC patients was similar to that in the gpi7 mutant-vaccinated mice.
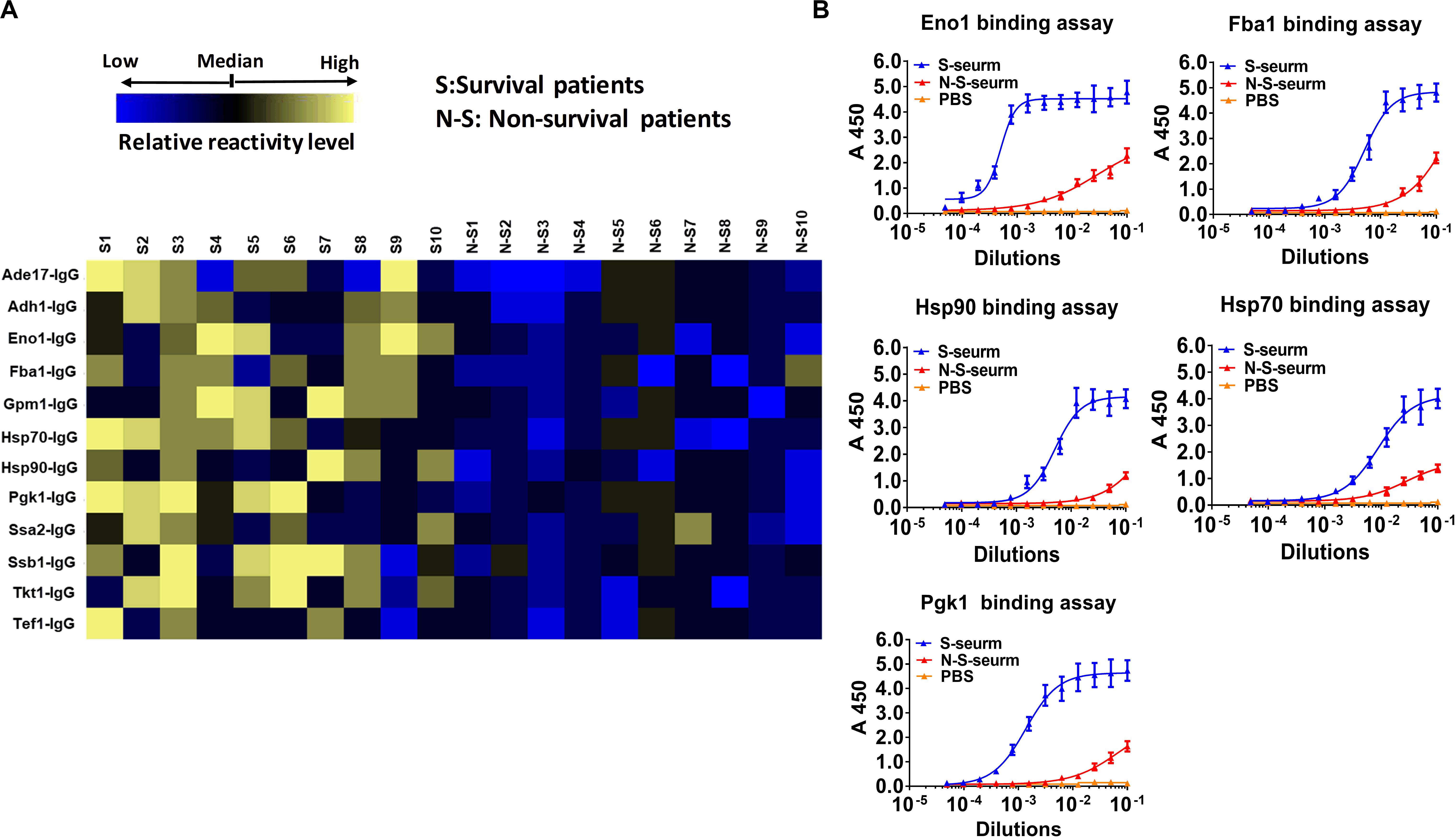
Figure 6. Reactivity differences among serum IgG antibodies targeting cell wall-localized moonlighting proteins of C. albicans are associated with the prognosis of IC patients. (A) Heat map of signal intensity of IgG antibodies in serum IC patients targeting cell wall-localized moonlighting proteins of C. albicans SC5314 was shown by Heml 1.0.3.3 (Heatmap illustrator) (n = 10 per group). The IgG antibody response signals were defined as the ratio of IgG antibody titers of serum from recovery IC patients or deceased IC patients versus that from healthy volunteers. The response for each IgG antibody is color-coded, as described in the color bar. (B) The represent IgG antibody titers in serum of recovery IC patients and deceased IC patients were evaluated by ELISA method.
TH2 Cell Response Is Involved in Gpi7 Mutant Vaccination Induced Immunoprotection Against Candidiasis in Mice
Cytokines play crucial roles in the initiation and amplification of B cell-mediated humoral immunity (Paul and Zhu, 2010). Our results indicated that the levels of TH1-type effector cytokines, such as tumor necrosis factor (TNF)-α and IL-6, in the spleen and serum of gpi7 mutant-vaccinated mice after challenged by C. albicans SC5314 were significantly lower than those in the spleen and serum of the parental strain SN152-vaccinated mice (Figure 7A). However, we found that gpi7 mutant vaccination could significantly increase the levels of TH2-type effector cytokines, such as IL-4 and IL-13, in the spleen and serum of mice when challenged by C. albicans SC5314, which suggests that these play a role in the immunoprotection against candidiasis (Figure 7B).
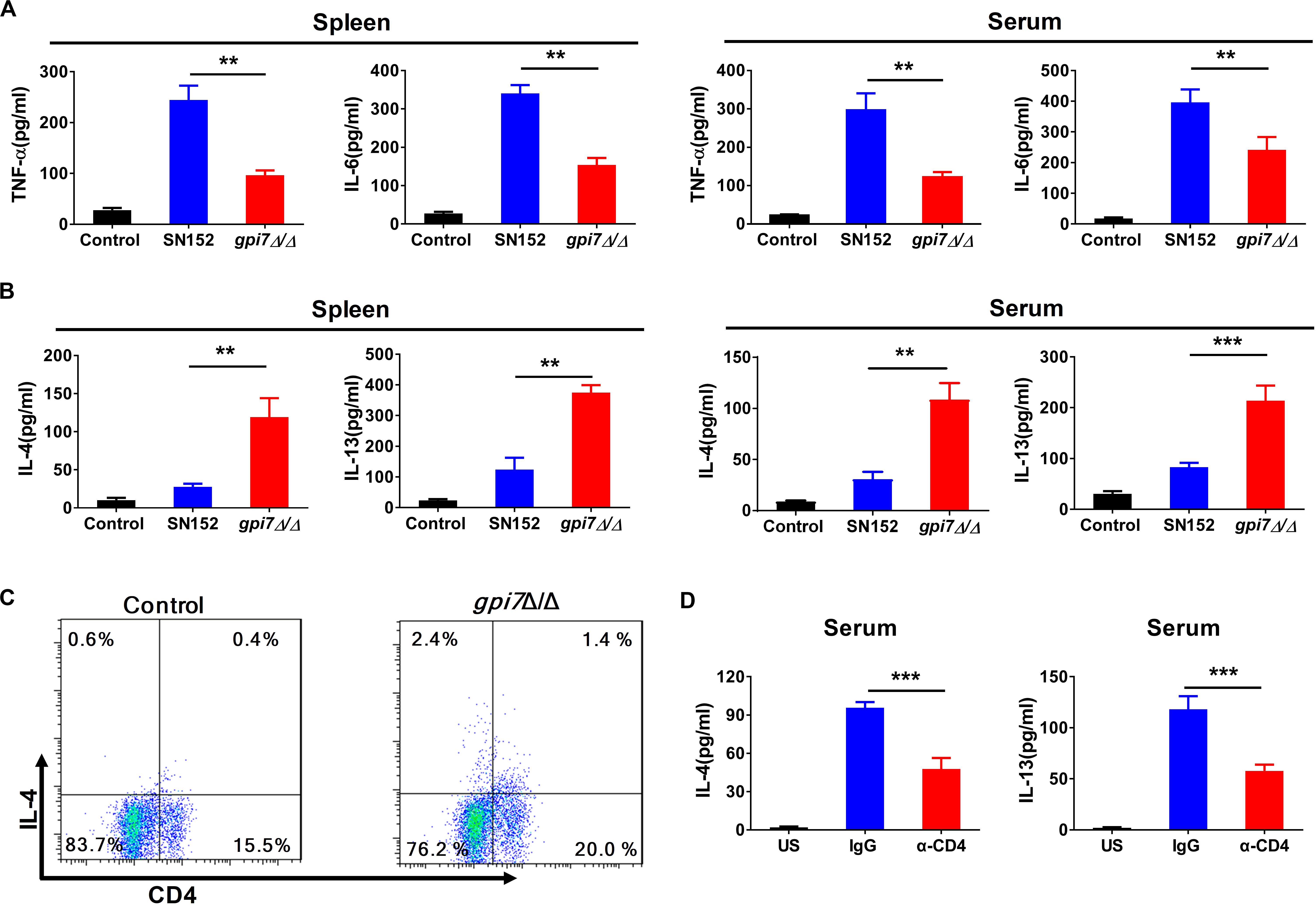
Figure 7. TH2 cell response is involved in gpi7 mutant vaccination induced immunoprotection against candidiasis in mice. Gpi7 mutant-vaccinated C57BL/6 mice were systemically infected with C. albicans SC5314 (5 × 105 CFU per mouse). (A) ELISA results for cytokines TNF-α, IL-6 in the spleen and serum of gpi7 mutant-vaccinated mice at day 2 post-infection (n = 5 per group). (B) ELISA results for cytokines IL-4 and IL-13 in the spleen and serum of gpi7 mutant-vaccinated mice at day 2 post-infection (n = 5 per group). (C) Flow cytometry analysis for CD4+IL-4+ T cells in the spleen of gpi7 mutant-vaccinated mice at day 2 post-infection. Data are representative images of 5 mice. (D) IL-4 and IL-13 levels in gpi7 mutant-vaccinated mice given intravenous injection of control IgG and neutralizing anti-CD4 antibody (200 μg per mouse) twice (n = 5 per group). **P < 0.01; ***P < 0.001 (Non-parametric one-way ANOVA).
CD4+T cells are important sources of TH2-type effector cytokines. The analysis of CD4+ lymphocytes revealed a significantly greater frequency of IL-4 producing CD4+ lymphocytes in the spleen of gpi7 mutant-vaccinated mice than the parental strain SN152-vaccinated mice (Figure 7C). However, we observed a similar frequency of IL-17A-producing CD4+T cells and IFN-γ producing CD4+T cells (Supplementary Figure S5). Depletion CD4+ T cells in gpi7 mutant-vaccinated mice by a neutralizing anti-CD4 antibody prior to challenge with C. albicans SC5314 significantly reduced the concentrations of IL-4 and IL-13 in the serum of gpi7 mutant-vaccinated mice (Figure 7D). These data indicated that CD4+ TH2 cells mediated immune responses were involved in the immunoprotection conferred by gpi7 mutant vaccination.
Dectin-1 Mediated IL-18 Production Is Required for the Generation of Candidacidal Antibodies in Gpi7 Mutant-Vaccinated Mice
Dectin-1 engagement by fungal β-(1,3)-glucan activates innate immune cells to respond and renders antigen-presenting cells competent to prime T cells differentiation (Rogers et al., 2005; Taylor et al., 2007). Our previous study indicated that β-(1,3)-glucan on the surface of gpi7 mutant was exposed and could engage host Dectin-1 (Shen et al., 2015). Therefore, we investigated whether Dectin-1 engagement by β-(1,3)-glucan involved in the immunoprotection of gpi7 mutant-vaccinated mice. We vaccinated Dectin-1-deficient mice with the gpi7-mutant strain and found that gpi7 mutant vaccination could not induce protective antibodies such as anti-Hsp90 and anti-Eno1 antibodies (Figure 8A). In addition, vaccination of Dectin-1-deficient mice with the gpi7-mutant strain could not protect against lethal invasive C. albicans infection, as indicated by fungal burdens in the kidneys and survival time (Figures 8B,C). Experiments with athymic nude mice found that gpi7 mutant vaccination also could not induce the generation of protective antibodies such as anti-Hsp90 and anti-Eno1 antibodies (Figure 8D). Therefore, we hypothesized that the TH2 cell-mediated protective effect induced by the gpi7-mutant strain is dependent on Dectin-1.
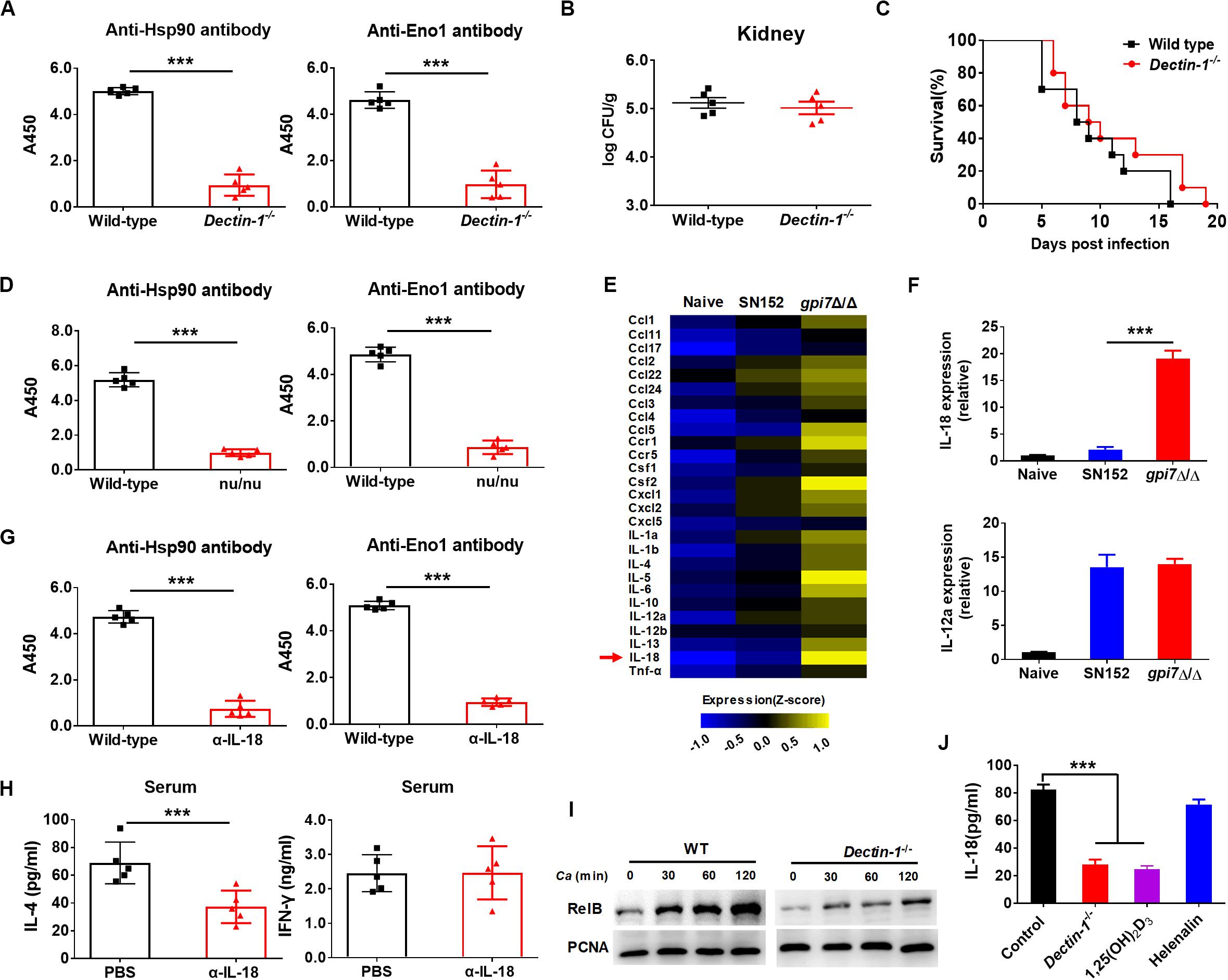
Figure 8. Gpi7 mutant induced antibodies generation in mice was Dectin-1 dependent and relied on IL-18. (A) Anti-Hsp90 and Anti-Eno1 antibodies assay by ELISA in gpi7 mutant-vaccinated wild-type C57BL/6 mice or Dectin-1-deficient C57BL/6 mice (n = 5 per group). (B,C) Gpi7 mutant-vaccinated wild-type C57BL/6 mice or Dectin-1-deficient C57BL/6 mice were systemically infected with C. albicans SC5314 (5 × 105 CFU per mouse). The kidney fungal burden was determined at day 2 post-infection (B, n = 5 per group), and the survival was monitored for 20 days’ post-infection (C, n = 10 per group). (D) Anti-Hsp90 and Anti-Eno1 antibodies assay by ELISA in gpi7 mutant-vaccinated wild-type BALB/c mice or athymic nude mice (BALB/c background) (n = 5 per group). (E) Peritoneal macrophages were stimulated with gpi7 mutant for 24 h and blank medium was used as a control. Total RNA was extracted, and real-time RT-PCR assays on inflammatory cytokines was performed. Data shown are heat maps for assayed gene panels. Arrows highlight the upregulated IL-18. (F) Real-time RT-PCR analysis of IL-18 and IL-12a expression in peritoneal macrophages as in (E), results were normalized to those of the control gene Gapdh. (G) Anti-Hsp90 and Anti-Eno1 antibodies assay by ELISA in gpi7 mutant-vaccinated C57BL/6 mice given intravenous injection of control IgG and neutralizing anti-IL-18 antibody (100 μg per mouse) twice (n = 5 per group). (H) ELISA results for cytokines IL-4 and IFN-γ in serum of mice as in (G) challenged by C. albicans (5 × 105 CFU per mouse, n = 5 per group). (I) Immunoblot analysis of RelB in the nucleus of peritoneal macrophages isolated from wild-type mice (Dectin-1+/+) and Dectin-1-deficient mice (Dectin-1– /–) and assessed at 0–2 h after challenge with gpi7 mutant (MOI = 5). (J) Peritoneal macrophages were pretreated with P65 inhibitor helenalin (20 μmol/L), RelB inhibitor 1,25(OH)2D3 (0.1 μmol/L) or PBS, and then stimulated with gpi7 mutant. The production of IL-18 was determined by ELISA. ***P < 0.001 [Non-parametric t-test (A,B,D,G,H); Log-rank test (C); One-way ANOVA (F,J)].
Dectin-1 receptor triggers innate immune cells to secret cytokines to prime naive CD4+ T cells differentiation. Therefore, a real-time RT-PCR assay was performed on peritoneal macrophages challenged by gpi7 mutant or parental SN152 strain to identify the potential cytokines initiation naive CD4+ T cell differentiation. Compared to other inflammatory related cytokines, we found that peritoneal macrophages challenged by gpi7 mutant had relative higher expression of the gene encoding IL-18 than those challenged by parent strain SN152 (Figures 8E,F). In addition, neutralization of endogenous IL-18 significantly reduced protective antibodies generation and serum concentration of IL-4 in gpi7 mutant-vaccinated mice after challenged by C. albicans (Figures 8G,H). However, neutralization of IL-18 had no significant influence on the serum concentration of IFN-γ (Figure 8H). Thus, we hypothesized that β-(1,3)-glucan on the surface of the gpi7 mutant activated the Dectin-1 receptor to facilitate the secretion of IL-18 from host innate immune cells, and then induced type 2 responses in gpi7 mutant-vaccinated mice.
Dectin-1 induces cytokines expression requires NF-κB signaling. Our previous studies indicated that gpi7 mutant could activate classical NF-κB (p65) by Dectin-1 receptor in macrophages (Shen et al., 2015). Here we show that gpi7 mutant also facilitated nuclear translocation of non-canonical NF-κB subunit RelB in a Dectin-1 dependent manner (Figure 8I). Furthermore, we found that RelB inhibitor 1,25-dihydroxyvitamin D3 [1,25(OH)2D3] (Mineva et al., 2009) and Dectin-1 deficiency could markedly impair the gpi7 mutant-induced production of IL-18 in peritoneal macrophages (Figure 8J). However, P65 inhibitor helenalin (Widen et al., 2017) had no significant influence on IL-18 production (Figure 8J). Thus, these data suggested that the Dectin-1 dependent activation of non-canonical NF-κB subunit RelB is required for the gpi7 mutant-induced priming of IL-18 production.
Discussion
Invasive infections caused by Candida spp. represents the fourth leading cause of healthcare-associated infections (Arendrup, 2010; Kullberg and Arendrup, 2015; McCarty and Pappas, 2016; Yang et al., 2017). The limited ability of antifungal therapy renewed the interest for effective vaccines research. In this study, we reported that the avirulent gpi7 mutant C. albicans could induce generation of candidacidal antibodies. We found that the generation of protective antibodies in gpi7 mutant-vaccinated mice was dependent on Dectin-1 and that IL-18 plays a central role in this process. Mechanistically, we showed that Dectin-1 engagement by gpi7 mutant was associated with translocation of non-canonical NF-κB subunit RelB to nucleus for facilitating IL-18 production. Our study not only suggests a new active vaccination strategy for Candida spp. infection but also provides evidence showing that the Dectin-1 receptor facilitates IL-18 production for the host generation of candidacidal antibodies.
Under normal conditions, C. albicans can shield cell surface β-(1,3)-glucan to avoid host phagocyte Dectin-1 recognition (Gantner et al., 2005; Wheeler et al., 2008). In the previous study, we constructed a gpi7 mutant C. albicans strain with surface β-(1,3)-glucan exposure, and this mutant could stimulate Dectin-1 receptor to drive TH1 and TH17 cell mediated immune responses (Shen et al., 2015). In this study, we found the gpi7 mutant could also elicit type 2 responses with candidacidal antibody generation and protect mice from lethal Candida spp. infection, including those caused by C. albicans and non-albicans Candida spp. (C. glabrata, C. krusei, C. parapsilosis and C. tropicalis) (Figures 1, 2, 4). However, other avirulent C. albicans mutant without surface β-(1,3)-glucan exposure could not confer immunoprotection against wild-type strain reinfection, suggesting the central role of β-(1,3)-glucan (Supplementary Figure S2). In addition to GPI-CWPs, a certain number of moonlighting proteins, which are multifunctional proteins participating in unrelated biological processes and are not the result of gene fusion, have been characterized in the cell wall of C. albicans (Gancedo et al., 2016; Serrano-Fujarte et al., 2016). We found that the main IgG antibodies in gpi7 mutant-induced antiserum were targeted to moonlighting proteins on cell wall of C. albicans (Figure 4). Some studies have reported that the moonlighting proteins of Candida spp. can induce IgG antibodies generation in host and protect against fungal reinfection (Pachl et al., 2006; Clancy et al., 2008; Xin et al., 2008; Li et al., 2011; Masek et al., 2011; Xin and Cutler, 2011). Furthermore, we found that the high titer of IgG antibody was maintained for a long time after gpi7 mutant-vaccination, which suggests the longevity of the protective effects of this vaccination against IC (Supplementary Figure S6). Previous studies have been demonstrated that purified glucan could enhance innate antifungal immune response (Quintin et al., 2012; Cheng et al., 2014) and glucan-based vaccine could increase resistance of experimental animal to invasive candidiasis (Bromuro et al., 2010; Liao et al., 2015, 2016). Heat inactivated cell wall β-glucan of C. albicans was exposed, but the protein on the cell wall surface was denatured. Our results indicated that heat inactivated C. albicans-vaccination and fungal glucan-vaccination did not increase resistance of mice to invasive candidiasis by decreasing fungal burden or improving survival in our study (Supplementary Figure S9). The above results further confirmed the protection of gpi7 mutant-vaccination is mainly mediated by immunity to Candida moonlighting proteins. At last, we further determined that the IgG profiles in serum from recovering IC patients were similar to those of gpi7 mutant-vaccinated mice, which suggests that antibodies that target moonlighting proteins favor the prognosis of IC (Figure 6). However, patients with invasive candidiasis often suffer from multiple co-morbidities along with immunosuppression, which may influence the antibodies response targeting moonlighting proteins. In addition, the antibodies response to candida moonlighting proteins in the patients with other forms of candidiasis, such as oral thrush and vaginal candidiasis, deserves further study in the future.
Host innate immune receptors can sense pathogens and then trigger an effective adaptive immunity. Our results indicated that gpi7 mutant induced protective effect and that antibody generation was depended on Dectin-1 receptor. Dectin-1 triggers Syk kinase-dependent inflammatory cytokines secretion to induce antifungal immunity (Taylor et al., 2007). We found that both the immunoprotection and antibody generation induced by the gpi7 mutant were dependent on Dectin-1 (Figures 8A–C), suggesting a new linkage between Dectin-1 receptor and adaptive humoral immunity. IL-18 was originally identified as a factor that enhances IFN-γ production by TH1 cells, particularly in association with IL-12 (Wawrocki et al., 2016; Kaplanski, 2018; Nakanishi, 2018). Thus, IL-18 is regarded as a proinflammatory cytokine that primes type 1 responses, but whether IL-18 can trigger type the 2 response to facilitate candidacidal antibodies generation is unclear. IL-18 is synthesized as an inactive precursor and is induced by NF-κB pathway (Wawrocki et al., 2016; Nakanishi, 2018). Nlrp3 inflammasome orchestrating Caspase-1 activation is essential for the cleavage of pro-IL-18 into their mature and biologically active forms, and plays a role in host antifungal immune response (Thomas et al., 2009; Karki et al., 2015; Kasper et al., 2018; de Castro-Jorge et al., 2019; Swidergall et al., 2019). In addition, recent study revealed that IL-18 production is essential for host defense against fungus Paracoccidioides brasiliensis (Ketelut-Carneiro et al., 2015). In this study, we found that IL-18 expression in peritoneal macrophages challenged with the gpi7 mutant was significantly increased in a Dectin-1-dependent manner (Figures 8F,J). More notably, neutralization of endogenous IL-18 significantly reduced protective antibodies generation in gpi7 mutant-vaccinated mice (Figure 8G). Therefore, Dectin-1 dependent IL-18 secretion represents the key factor for the type 2 responses with candidacidal antibodies generation in the present study.
The NF-κB transcription factors consist of five members: p52 and its precursor (p100), RelB, p50 and its precursor (p105), p65, and c-Rel (Hayden and Ghosh, 2004; Mitchell et al., 2016). Dectin-1 activates not only the Syk-dependent canonical NF-κB subunits p65 and c-Rel, but also the non-canonical NF-κB subunit RelB (Gringhuis et al., 2009; Xu et al., 2018). p65 and c-Rel activation induced by Dectin-1 can prime cytokines IL-12, IL-6 and IL-1β production and drive the TH1 and TH17 cells mediated responses (Gross et al., 2006; Yang et al., 2011). While orchestration of RelB by Dectin-1 often initiates the secretion of cytokines IL-4 and IL-5 to drive TH2 cell mediated response (Gringhuis et al., 2009; Xu et al., 2018). Our previous study indicated gpi7 mutant could activate canonical NF-κB subunits p65 through Dectin-1 receptor and thereby prime TH1 and TH17 cell responses against fungal infections (Shen et al., 2015). In this study, we found gpi7 mutant also facilitated nuclear translocation of non-canonical NF-κB subunit RelB through activating Dectin-1 receptor (Figure 8I). More notably, we found that the gpi7 mutant induced IL-18 production in peritoneal macrophages also depended on RelB activation, but not p65 (Figure 8J). Therefore, our results suggested that Dectin-1 mediated RelB activation could regulated IL-18 production and then prime candidacidal antibodies generation.
In conclusion, our present study revealed that vaccination with the avirulent C. albicans gpi7 mutant could protect against invasive Candida infection, and thus provides a new active vaccination strategy. The immunoprotection attributed to type 2 immune responses which mediated generation of candidacidal antibodies. We further demonstrated that Dectin-1 engagement by the cell surface β-1,3-glucan of gpi7 mutant could trigger RelB activation to prime IL-18 expression and subsequently regulate the generation of candidacidal antibodies, and these findings establish a new paradigm regarding the link between the Dectin-1 mediated innate immune response and adaptive humoral immunity.
Data Availability Statement
All datasets generated for this study are included in the article/Supplementary Material.
Ethics Statement
The studies involving human participants were reviewed and approved by Institutional Ethical Board of Shanghai Tenth People’s Hospital. The patients/participants provided their written informed consent to participate in this study. The animal study was reviewed and approved by Institutional Animal Care and Use Committee of Tongji University. Written informed consent was obtained from the owners for the participation of their animals in this study.
Author Contributions
M-MA, ZZ, Y-YJ, and HS conceptualized the study. HS and S-MC performed the experiments on vaccination with gpi7 mutant protecting mice from candidiasis, and wrote the manuscript. J-JS, WF, and S-YG performed the vaccination experiments. Y-LC and Y-DW performed flow cytometry analysis. W-TH, X-RQ, and YZ performed analysis on IgG antibodies in serum of gpi7 mutant-vaccinated mice. X-YH and LL performed confocal laser scanning microscopy observation. YY performed the analysis of IgG antibodies in serum of IC patients, and wrote the manuscript. M-MA, ZZ, HS, and Y-YJ supervised the study and wrote the manuscript. All authors contributed to the article and approved the submitted version.
Funding
This study was supported by the National Natural Science Foundation of China (81601745, 81671989, 81471924, and 81903670), Scientific Research Projects of the Shanghai Science and Technology Committee (18411951300), Shanghai Youth Medical Talents Training Program (Laboratory Medicine) [2018] 02, the Key Projects of the 12th 5 Year of the Chinese Army (BWS12J027), and Scientific Research Project of Shanghai Municipal Health Bureau (201840006).
Conflict of Interest
The authors declare that the research was conducted in the absence of any commercial or financial relationships that could be construed as a potential conflict of interest.
Acknowledgments
We thank Dr. Gordon D. Brown (University of Aberdeen) for kindly providing Dectin-1-deficient mice. We thank Dr. Noble SM (University of California-San Francisco) for providing C. albicans SN152. We thank Dr. Sanglard D (Centre Hospitalier Universitaire Vaudois) for kindly providing C. albicans SC5314. We also thank Dr. Filler SG (State University of New York) for kindly providing ssa1 mutant C. albicans and Dr. Jun Gu (Changhai Hospital, Shanghai, China) for kindly providing the C. albicans isolates 0304103.
Supplementary Material
The Supplementary Material for this article can be found online at: https://www.frontiersin.org/articles/10.3389/fmicb.2020.01648/full#supplementary-material
References
Arendrup, M. C. (2010). Epidemiology of invasive candidiasis. Curr. Opin. Crit. Care 16, 445–452. doi: 10.1097/MCC.0b013e32833e84d2
Bromuro, C., Romano, M., Chiani, P., Berti, F., Tontini, M., Proietti, D., et al. (2010). Beta-glucan-CRM197 conjugates as candidates antifungal vaccines. Vaccine 28, 2615–2623. doi: 10.1016/j.vaccine.2010.01.012
Bugli, F., Cacaci, M., Martini, C., Torelli, R., Posteraro, B., Sanguinetti, M., et al. (2013). Human monoclonal antibody-based therapy in the treatment of invasive candidiasis. Clin. Dev. Immunol. 2013:403121. doi: 10.1155/2013/403121
Candel, F. J., Pazos Pacheco, C., Ruiz-Camps, I., Maseda, E., Sanchez-Benito, M. R., Montero, A., et al. (2017). Update on management of invasive candidiasis. Rev. Esp. Quimioter. 30, 397–406.
Chaffin, W. L. (2008). Candida albicans cell wall proteins. Microbiol. Mol. Biol. Rev. 72, 495–544. doi: 10.1128/MMBR.00032-07
Cheng, S. C., Quintin, J., Cramer, R. A., Shepardson, K. M., Saeed, S., Kumar, V., et al. (2014). mTOR- and HIF-1alpha-mediated aerobic glycolysis as metabolic basis for trained immunity. Science 345:1250684. doi: 10.1126/science.1250684
Clancy, C. J., and Nguyen, M. H. (2013). Finding the “missing 50%” of invasive candidiasis: how nonculture diagnostics will improve understanding of disease spectrum and transform patient care. Clin. Infect Dis. 56, 1284–1292. doi: 10.1093/cid/cit006
Clancy, C. J., Nguyen, M. L., Cheng, S., Huang, H., Fan, G., Jaber, R. A., et al. (2008). Immunoglobulin G responses to a panel of Candida albicans antigens as accurate and early markers for the presence of systemic candidiasis. J. Clin. Microbiol. 46, 1647–1654. doi: 10.1128/JCM.02018-07
de Castro-Jorge, L. A., de Carvalho, R. V. H., Klein, T. M., Hiroki, C. H., Lopes, A. H., Guimaraes, R. M., et al. (2019). The NLRP3 inflammasome is involved with the pathogenesis of Mayaro virus. PLoS Pathog. 15:e1007934. doi: 10.1371/journal.ppat.1007934
Drummond, R. A., Gaffen, S. L., Hise, A. G., and Brown, G. D. (2014). Innate defense against fungal pathogens. Cold Spring Harb. Perspect. Med. 5:a019620. doi: 10.1101/cshperspect.a019620
Fu, J., Ding, Y., Wei, B., Wang, L., Xu, S., Qin, P., et al. (2017). Epidemiology of Candida albicans and non-C.albicans of neonatal candidemia at a tertiary care hospital in western China. BMC Infect. Dis. 17:329. doi: 10.1186/s12879-017-2423-8
Gancedo, C., Flores, C. L., and Gancedo, J. M. (2016). The expanding landscape of moonlighting proteins in yeasts. Microbiol. Mol. Biol. Rev. 80, 765–777. doi: 10.1128/MMBR.00012-16
Gantner, B. N., Simmons, R. M., and Underhill, D. M. (2005). Dectin-1 mediates macrophage recognition of Candida albicans yeast but not filaments. EMBO J. 24, 1277–1286. doi: 10.1038/sj.emboj.7600594
Gringhuis, S. I., den Dunnen, J., Litjens, M., van der Vlist, M., Wevers, B., Bruijns, S. C., et al. (2009). Dectin-1 directs T helper cell differentiation by controlling noncanonical NF-kappaB activation through Raf-1 and Syk. Nat. Immunol. 10, 203–213. doi: 10.1038/ni.1692
Gross, O., Gewies, A., Finger, K., Schafer, M., Sparwasser, T., Peschel, C., et al. (2006). Card9 controls a non-TLR signalling pathway for innate anti-fungal immunity. Nature 442, 651–656. doi: 10.1038/nature04926
Hayden, M. S., and Ghosh, S. (2004). Signaling to NF-kappaB. Genes Dev. 18, 2195–2224. doi: 10.1101/gad.1228704
Huang, J., Meng, S., Hong, S., Lin, X., Jin, W., and Dong, C. (2016). IL-17C is required for lethal inflammation during systemic fungal infection. Cell Mol. Immunol. 13, 474–483. doi: 10.1038/cmi.2015.56
Imhof, I., Flury, I., Vionnet, C., Roubaty, C., Egger, D., and Conzelmann, A. (2004). Glycosylphosphatidylinositol (GPI) proteins of Saccharomyces cerevisiae contain ethanolamine phosphate groups on the alpha1,4-linked mannose of the GPI anchor. J. Biol. Chem. 279, 19614–19627. doi: 10.1074/jbc.M401873200
Kaplanski, G. (2018). Interleukin-18: biological properties and role in disease pathogenesis. Immunol. Rev. 281, 138–153. doi: 10.1111/imr.12616
Karki, R., Man, S. M., Malireddi, R. K. S., Gurung, P., Vogel, P., Lamkanfi, M., et al. (2015). Concerted activation of the AIM2 and NLRP3 inflammasomes orchestrates host protection against Aspergillus infection. Cell Host Microbe 17, 357–368. doi: 10.1016/j.chom.2015.01.006
Kasper, L., Konig, A., Koenig, P. A., Gresnigt, M. S., Westman, J., Drummond, R. A., et al. (2018). The fungal peptide toxin Candidalysin activates the NLRP3 inflammasome and causes cytolysis in mononuclear phagocytes. Nat. Commun. 9:4260. doi: 10.1038/s41467-018-06607-1
Ketelut-Carneiro, N., Silva, G. K., Rocha, F. A., Milanezi, C. M., Cavalcanti-Neto, F. F., Zamboni, D. S., et al. (2015). IL-18 triggered by the Nlrp3 inflammasome induces host innate resistance in a pulmonary model of fungal infection. J. Immunol. 194, 4507–4517. doi: 10.4049/jimmunol.1402321
Kullberg, B. J., and Arendrup, M. C. (2015). Invasive candidiasis. N. Engl. J. Med. 373, 1445–1456. doi: 10.1056/NEJMra1315399
Li, W., Hu, X., Zhang, X., Ge, Y., Zhao, S., Hu, Y., et al. (2011). Immunisation with the glycolytic enzyme enolase confers effective protection against Candida albicans infection in mice. Vaccine 29, 5526–5533. doi: 10.1016/j.vaccine.2011.05.030
Liao, G., Zhou, Z., Burgula, S., Liao, J., Yuan, C., Wu, Q., et al. (2015). Synthesis and immunological studies of linear oligosaccharides of beta-glucan as antigens for antifungal vaccine development. Bioconjug. Chem. 26, 466–476. doi: 10.1021/bc500575a
Liao, G., Zhou, Z., Liao, J., Zu, L., Wu, Q., and Guo, Z. (2016). 6-O-branched oligo-beta-glucan-based antifungal glycoconjugate vaccines. ACS Infect. Dis. 2, 123–131. doi: 10.1021/acsinfecdis.5b00104
Masek, J., Bartheldyova, E., Turanek-Knotigova, P., Skrabalova, M., Korvasova, Z., Plockova, J., et al. (2011). Metallochelating liposomes with associated lipophilised norAbuMDP as biocompatible platform for construction of vaccines with recombinant His-tagged antigens: preparation, structural study and immune response towards rHsp90. J. Control Release 151, 193–201. doi: 10.1016/j.jconrel.2011.01.016
McCarty, T. P., and Pappas, P. G. (2016). Invasive candidiasis. Infect. Dis. Clin. North Am. 30, 103–124. doi: 10.1016/j.idc.2015.10.013
Mineva, N. D., Wang, X., Yang, S., Ying, H., Xiao, Z. X., Holick, M. F., et al. (2009). Inhibition of RelB by 1,25-dihydroxyvitamin D3 promotes sensitivity of breast cancer cells to radiation. J. Cell Physiol. 220, 593–599. doi: 10.1002/jcp.21765
Mitchell, S., Vargas, J., and Hoffmann, A. (2016). Signaling via the NFkappaB system. Wiley Interdiscip. Rev. Syst. Biol. Med. 8, 227–241. doi: 10.1002/wsbm.1331
Nakanishi, K. (2018). Unique action of Interleukin-18 on T cells and other immune cells. Front. Immunol. 9:763. doi: 10.3389/fimmu.2018.00763
Pachl, J., Svoboda, P., Jacobs, F., Vandewoude, K., van der Hoven, B., Spronk, P., et al. (2006). A randomized, blinded, multicenter trial of lipid-associated amphotericin B alone versus in combination with an antibody-based inhibitor of heat shock protein 90 in patients with invasive candidiasis. Clin. Infect. Dis. 42, 1404–1413. doi: 10.1086/503428
Parmeland, L., Gazon, M., Guerin, C., Argaud, L., Lehot, J. J., Bastien, O., et al. (2013). Candida albicans and non-Candida albicans fungemia in an institutional hospital during a decade. Med. Mycol. 51, 33–37. doi: 10.3109/13693786.2012.686673
Paul, W. E., and Zhu, J. (2010). How are T(H)2-type immune responses initiated and amplified? Nat. Rev. Immunol. 10, 225–235. doi: 10.1038/nri2735
Quintin, J., Saeed, S., Martens, J. H. A., Giamarellos-Bourboulis, E. J., Ifrim, D. C., Logie, C., et al. (2012). Candida albicans infection affords protection against reinfection via functional reprogramming of monocytes. Cell Host Microbe 12, 223–232. doi: 10.1016/j.chom.2012.06.006
Richard, M., Ibata-Ombetta, S., Dromer, F., Bordon-Pallier, F., Jouault, T., and Gaillardin, C. (2002). Complete glycosylphosphatidylinositol anchors are required in Candida albicans for full morphogenesis, virulence and resistance to macrophages. Mol. Microbiol. 44, 841–853. doi: 10.1046/j.1365-2958.2002.02926.x
Richard, M. L., and Plaine, A. (2007). Comprehensive analysis of glycosylphosphatidylinositol-anchored proteins in Candida albicans. Eukaryot. Cell 6, 119–133. doi: 10.1128/EC.00297-06
Rogers, N. C., Slack, E. C., Edwards, A. D., Nolte, M. A., Schulz, O., Schweighoffer, E., et al. (2005). Syk-dependent cytokine induction by Dectin-1 reveals a novel pattern recognition pathway for C type lectins. Immunity 22, 507–517. doi: 10.1016/j.immuni.2005.03.004
Serrano-Fujarte, I., Lopez-Romero, E., and Cuellar-Cruz, M. (2016). Moonlight-like proteins of the cell wall protect sessile cells of Candida from oxidative stress. Microb. Pathog. 90, 22–33. doi: 10.1016/j.micpath.2015.10.001
Shen, H., Chen, S. M., Liu, W., Zhu, F., He, L. J., Zhang, J. D., et al. (2015). Abolishing cell wall Glycosylphosphatidylinositol-Anchored proteins in Candida albicans enhances recognition by host Dectin-1. Infect. Immun. 83, 2694–2704. doi: 10.1128/IAI.00097-15
Sun, J. N., Solis, N. V., Phan, Q. T., Bajwa, J. S., Kashleva, H., Thompson, A., et al. (2010). Host cell invasion and virulence mediated by Candida albicans Ssa1. PLoS Pathog. 6:e1001181. doi: 10.1371/journal.ppat.1001181
Swidergall, M., Khalaji, M., Solis, N. V., Moyes, D. L., Drummond, R. A., Hube, B., et al. (2019). Candidalysin is required for neutrophil recruitment and virulence during systemic Candida albicans infection. J. Infect. Dis. 220, 1477–1488. doi: 10.1093/infdis/jiz322
Taylor, P. R., Tsoni, S. V., Willment, J. A., Dennehy, K. M., Rosas, M., Findon, H., et al. (2007). Dectin-1 is required for beta-glucan recognition and control of fungal infection. Nat. Immunol. 8, 31–38. doi: 10.1038/ni1408
Thomas, P. G., Dash, P., Aldridge, J. R. Jr., Ellebedy, A. H., Reynolds, C., Funk, A. J., et al. (2009). The intracellular sensor NLRP3 mediates key innate and healing responses to influenza A virus via the regulation of caspase-1. Immunity 30, 566–575. doi: 10.1016/j.immuni.2009.02.006
Underhill, D. M., Rossnagle, E., Lowell, C. A., and Simmons, R. M. (2005). Dectin-1 activates Syk tyrosine kinase in a dynamic subset of macrophages for reactive oxygen production. Blood 106, 2543–2550. doi: 10.1182/blood-2005-03-1239
Verma, A., Wuthrich, M., Deepe, G., and Klein, B. (2014). Adaptive immunity to fungi. Cold Spring Harb. Perspect. Med. 5:a019612. doi: 10.1101/cshperspect.a019612
Wawrocki, S., Druszczynska, M., Kowalewicz-Kulbat, M., and Rudnicka, W. (2016). Interleukin 18 (IL-18) as a target for immune intervention. Acta Biochim. Pol. 63, 59–63. doi: 10.18388/abp.2015_1153
Wheeler, R. T., Kombe, D., Agarwala, S. D., and Fink, G. R. (2008). Dynamic, morphotype-specific Candida albicans beta-glucan exposure during infection and drug treatment. PLoS Pathog. 4:e1000227. doi: 10.1371/journal.ppat.1000227
Widen, J. C., Kempema, A. M., Villalta, P. W., and Harki, D. A. (2017). Targeting NF-kappaB p65 with a helenalin inspired bis-electrophile. ACS Chem. Biol. 12, 102–113. doi: 10.1021/acschembio.6b00751
Xin, H., and Cutler, J. E. (2011). Vaccine and monoclonal antibody that enhance mouse resistance to candidiasis. Clin. Vaccine Immunol. 18, 1656–1667. doi: 10.1128/CVI.05215-11
Xin, H., Dziadek, S., Bundle, D. R., and Cutler, J. E. (2008). Synthetic glycopeptide vaccines combining beta-mannan and peptide epitopes induce protection against candidiasis. Proc. Natl. Acad. Sci. U.S.A. 105, 13526–13531. doi: 10.1073/pnas.0803195105
Xu, X., Xu, J. F., Zheng, G., Lu, H. W., Duan, J. L., Rui, W., et al. (2018). CARD9(S12N) facilitates the production of IL-5 by alveolar macrophages for the induction of type 2 immune responses. Nat. Immunol. 19, 547–560. doi: 10.1038/s41590-018-0112-4
Yang, H., He, H., and Dong, Y. (2011). CARD9 Syk-dependent and Raf-1 Syk-independent signaling pathways in target recognition of Candida albicans by Dectin-1. Eur. J. Clin. Microbiol. Infect. Dis. 30, 303–305. doi: 10.1007/s10096-010-1103-z
Yang, Y., Guo, F., Kang, Y., Zang, B., Cui, W., Qin, B., et al. (2017). Epidemiology, clinical characteristics, and risk factors for mortality of early- and late-onset invasive candidiasis in intensive care units in China. Medicine (Baltimore) 96:e7830. doi: 10.1097/MD.0000000000007830
Keywords: C. albicans, invasive candidiasis, vaccine, antibody, dectin-1
Citation: Shen H, Yu Y, Chen S-M, Sun J-J, Fang W, Guo S-Y, Hou W-T, Qiu X-R, Zhang Y, Chen Y-L, Wang Y-D, Hu X-Y, Lu L, Jiang Y-Y, Zou Z and An M-M (2020) Dectin-1 Facilitates IL-18 Production for the Generation of Protective Antibodies Against Candida albicans. Front. Microbiol. 11:1648. doi: 10.3389/fmicb.2020.01648
Received: 12 March 2020; Accepted: 25 June 2020;
Published: 16 July 2020.
Edited by:
Hector Mora Montes, University of Guanajuato, MexicoReviewed by:
David Loyd Williams, East Tennessee State University, United StatesLuis Antonio Pérez-García, Universidad Autónoma de San Luis Potosí, Mexico
Copyright © 2020 Shen, Yu, Chen, Sun, Fang, Guo, Hou, Qiu, Zhang, Chen, Wang, Hu, Lu, Jiang, Zou and An. This is an open-access article distributed under the terms of the Creative Commons Attribution License (CC BY). The use, distribution or reproduction in other forums is permitted, provided the original author(s) and the copyright owner(s) are credited and that the original publication in this journal is cited, in accordance with accepted academic practice. No use, distribution or reproduction is permitted which does not comply with these terms.
*Correspondence: Yuan-Ying Jiang, jiangyy@tongji.edu.cn; Zui Zou, zouzui1980@163.com; Mao-Mao An, anmaomao@tongji.edu.cn
†These authors have contributed equally to this work