- 1Department of Dermatology and Venereology, Peking University First Hospital, Beijing, China
- 2Research Center for Medical Mycology, Peking University, Beijing, China
- 3National Clinical Research Center for Skin and Immune Diseases, Beijing, China
- 4Department of Laboratory Medicine, The First Affiliated Hospital of Zunyi Medical University, Zunyi, China
- 5Department of Laboratory Medicine, Peking University First Hospital, Beijing, China
To determine the dynamic changes of pathogenic yeast prevalence and antifungal susceptibility patterns in tertiary hospitals in China, we analyzed 527 yeast isolates preserved in the Research Center for Medical Mycology at Peking University, Beijing, China, between Jan 2010 and Dec 2019 and correctly identified 19 yeast species by matrix-assisted laser desorption ionization time-of-flight mass spectrometry (MALDI-TOF-MS) and ribosomal DNA sequencing. Antifungal susceptibility testing was performed following a Sensititre YeastOne colorimetric microdilution panel with nine clinically available antifungals. The Clinical and Laboratory Standards Institute (CLSI)-approved standard M27-A3 (S4) and newly revised clinical breakpoints or species-specific and method-specific epidemiological cutoff values were used for the interpretation of susceptibility test data. In this study, although Candida albicans was the predominant single species, non-C. albicans species constituted >50% of isolates in 6 out of 10 years, and more rare species were present in the recent 5 years. The non-C. albicans species identified most frequently were Candida parapsilosis sensu stricto, Candida tropicalis, and Candida glabrata. The prevalence of fluconazole and voriconazole resistance in the C. parapsilosis sensu stricto population was <3%, but C. tropicalis exhibited decreased susceptibility to fluconazole (42, 57.5%) and voriconazole (31, 42.5%), and 22 (30.1%) C. tropicalis isolates exhibited wild-type minimum inhibitory concentrations (MICs) to posaconazole. Furthermore, fluconazole and voriconazole cross-resistance prevalence in C. tropicalis was 19 (26.1%). The overall prevalence of fluconazole resistance in the C. glabrata population was 14 (26.9%), and prevalence of isolates exhibiting voriconazole non-wild-type MICs was 33 (63.5%). High-level echinocandin resistance was mainly observed in C. glabrata, and the prevalence rates of isolate resistance to anidulafungin, micafungin, and caspofungin were 5 (9.6%), 5 (9.6%), and 4 (7.7%), respectively. Moreover, one C. glabrata isolate showed multidrug resistant to azoles, echinocandins, and flucytosine. Overall, the 10-year surveillance study showed the increasing prevalence of non-C. albicans species over time; the emergence of azole resistance in C. tropicalis and multidrug resistance in C. glabrata over the years reinforced the need for epidemiological surveillance and monitoring.
Introduction
A growing population of immunocompromised patients has resulted in frequent diagnoses of invasive fungal infections (IFIs), including those caused by unusual yeasts (Dabas et al., 2017). Invasive candidiasis is a growing concern worldwide with high morbidity and mortality; it affects patients of all ages, including patients with malignancies, HIV-negative immunocompromised (IC) patients, and non-immunocompromised (NIC) patients among those who are critically ill, often admitted to an intensive care unit (ICU), or diagnosed with diabetes mellitus or uncontrolled hyperglycemia requiring invasive mechanical ventilation (Berdal et al., 2014; Bitar et al., 2014; Epelbaum and Chasan, 2017; Alves et al., 2018; Ding et al., 2018; Mantadakis et al., 2018). Although Candida albicans is still the leading cause of fungemia, epidemiological switch and species distribution have shifted toward non-C. albicans in recent decades, especially in patients with severe health conditions (Canton et al., 2012; Pfaller et al., 2015; Taei et al., 2019; Xiao et al., 2020). There are considerable geographic and population variability in the prevalence of pathogenic Candida species; for example, Candida tropicalis is frequently isolated in Asia and South America, and Candida glabrata has a high frequency in North and Central Europe and the United States, specially among elderly people (Castanheira et al., 2016; Pappas et al., 2018). C. glabrata is also generally more common among individuals >60 years of age and among recipients of solid organ transplant (Cleveland et al., 2015; McCarty and Pappas, 2016). The growing number of non-C. albicans species might be connected to former exposure to polyenes and azoles, use of indwelling catheters, malignancies, age, the improved biochemical and molecular diagnostic methods in laboratories, and geographical regions (Diekema et al., 2012; Pfaller et al., 2012; Kullberg and Arendrup, 2015; McCarty and Pappas, 2016; Perlin et al., 2017; Pappas et al., 2018).
The incidence of non-C. albicans species infection is increasing compared with that of C. albicans, and antifungal resistance was generally uncommon; however, azole resistance occurs in several species, such as C. glabrata, C. tropicalis, and Candida krusei (Berdal et al., 2014; Chapman et al., 2017). There have been reports of outbreaks of azole-resistant Candida parapsilosis sensu stricto in adult ICUs in Brazil and in African neonatal ICUs (NICUs) (Neji et al., 2017; Thomaz et al., 2018). In addition, susceptibility to antifungals is variable among species of the C. parapsilosis complex (Brilhante et al., 2018). Moreover, in 2009, a new fluconazole-resistant species, Candida auris, was identified in East Asia and has now been isolated on five continents, showing high morbidity and mortality (Spivak and Hanson, 2018). Echinocandins have been used as preferred antifungals for most candidemia and invasive candidiasis (Mora-Duarte et al., 2002; Andes et al., 2012; Pappas et al., 2016), but echinocandin resistance among Candida species is rising. C. glabrata shows cross-resistance to azoles, limiting therapeutic options. The recent emergence of multidrug-resistant Candida species further complicates the selection of antifungal therapy owing to the lack of data that can guide therapy (Pfaller et al., 2011a, b; Alexander et al., 2013; Lewis et al., 2013; Castanheira et al., 2014). These findings highlight the importance of epidemiological and laboratory studies of invasive candidiasis in hospitals (Canela et al., 2018).
Rapid species identification and antifungal susceptibility tests are essential for the treatment of IFIs. Matrix-assisted laser desorption ionization time-of-flight mass spectrometry (MALDI-TOF-MS) combined with nucleotide sequencing is a reliable approach for identifying yeast isolates (Maldonado et al., 2018; Kal Çakmaklıoğulları et al., 2019). The commercial Sensititre YeastOne (SYO) test is widely used to determine the susceptibility of Candida spp., and it provides an easy and affordable alternative to the Clinical and Laboratory Standards Institute (CLSI) or the European Committee on Antimicrobial Susceptibility Testing (EUCAST) standard broth microdilution methods (Cuenca-Estrella and Rodriguez-Tudela, 2010; Pfaller, 2012; Posteraro and Sanguinetti, 2014; Posteraro et al., 2015). For Candida spp., the results of the SYO test are generally in agreement with those obtained by EUCAST and CLSI reference methods, and some method-dependent epidemiological cutoff values (ECVs) were also defined following the criteria recently published by the CLSI (Eschenauer et al., 2014; Espinel-Ingroff et al., 2015, 2019; Posteraro et al., 2015; Perlin et al., 2017).
We believe that knowledge of invasive yeast infection epidemiology in a tertiary hospital in China, including geographical variability and dynamics of susceptibility to the clinical available antifungal drugs, is essential for guiding antifungal treatment protocol development. The objectives of this study were to determine the species distribution and in vitro antifungal susceptibility patterns of yeast species collected successively for 10 years from a tertiary teaching hospital at Beijing, China.
Materials and Methods
Study Design
This study was a retrospective, laboratory-based study of invasive yeast infections with its inception in Jan 2010 and end in Dec 2019. The 527 yeast isolates came from 514 patients, with more than one episode of candidiasis in some patients. The isolates were preserved at the Research Center for Medical Mycology at Peking University First Hospital, Beijing, China. It is a “rank-A tertiary” hospital with 64 wards and has a total of 1,835 beds and admits more than 86,000 patients per year and provides care for different types of patients across the country. Surgical patients account for approximately 51.2% of the hospital population.
For each episode of yeast isolation (see the criteria for study inclusion below), the information collected included the patient’s age and gender, the patient’s classification (inpatient or outpatient), and the ward location [e.g., emergency department (ED), surgical, medical, and ICU] of the patient at the time of collection of the sample. The date of sample collection, the specimen type, the body site of isolation, and the initial species identification made by the referring laboratory were also recorded. In this study, all isolates were isolated from normally sterile clinical samples of inpatients.
Criteria for Study Inclusion
We collected all Candida, Cryptococcus, and other yeast isolates recovered from blood; other sterile body fluids, including ascitic fluid and peritoneal dialysate fluid, pus, and tissue from patients with invasive yeast infections (De Pauw et al., 2008) were included in this study. Yeast isolates from bronchoalveolar lavage (BAL) fluid samples, central venous catheter (CVC) tips, and the gastrointestinal tracts [e.g., biliary tract fluid (aseptically collected)] of patients with invasive infections were tested; however, yeast isolates from sputum, urine, and the genital tract and others considered colonizers were excluded. Isolates of the same species and of the same susceptible or resistant biotype profile from the same site of a given patient isolated at a different time were considered duplicates and also excluded.
Species Identification
All yeast isolates were reidentified to the species level by MALDI-TOF-MS using the MALDI Biotyper RTC 4.0 software (Bruker Daltonik) by rastering the target position. For any isolate with no identification or uncertain identification (e.g., low confidence value) results by MALDI-TOF-MS and for all isolates identified within the C. parapsilosis complex (i.e., C. parapsilosis sensu stricto, Candida metapsilosis, and Candida orthopsilosis) and C. glabrata, sequencing of the internal transcribed spacer (ITS) rDNA (ITS1/ITS4) region was performed for definitive species identification.
Antifungal Susceptibility Testing
An SYO YO10 panel tray (Thermo Scientific, Cleveland, OH, United States) was used for antifungal susceptibility tests. The plate contains serial twofold dilutions of amphotericin B (0.12–8 mg/L), flucytosine (0.06–64 mg/L), fluconazole (0.12–256 mg/L), itraconazole (0.015–16 mg/L), voriconazole (0.008–8 mg/L), posaconazole (0.008–8 mg/L), anidulafungin (0.015–8 mg/L), micafungin (0.008–8 mg/L), and caspofungin (0.008–8 mg/L).
Antifungal susceptibility testing was performed following the manufacturer’s instructions. C. parapsilosis ATCC 22019 and C. krusei ATCC 6258 from the American Type Culture Collection were included as control isolates in all experiments. Minimum inhibitory concentrations (MICs) were determined after 24 h of incubation at 35°C for Candida spp. and Trichosporon spp. and after 72 h of incubation for Cryptococcus spp. MIC was defined as the lowest concentration of an antifungal at which the color in the well changed from red (positive, indicating growth) to blue (negative, indicating no growth).
Interpretation of MIC Results
MIC data were determined using the CLSI-approved standard M27-S4, and interpretation of susceptibility was performed by applying the updated species-specific CLSI clinical breakpoints (CBPs) (CLSI, 2017, Wayne, PA, United States), or epidemiological cutoff values (ECVs) where CBPs were not available (CLSI, 2018, Wayne, PA, United States; Canton et al., 2012; Pfaller and Diekema, 2012; Espinel-Ingroff et al., 2019) were applied (Supplementary Table 1). In the absence of CBPs, isolates were defined as having a wild-type (WT) or a non-WT (NWT) drug susceptibility phenotype according to the ECVs as determined by the CLSI broth microdilution methods and colorimetric SYO method.
Ethical Approval
This retrospective study was approved by the ethics committee of Peking University First Hospital. The need for informed consent was waived by the Clinical Research Ethics Committee.
Statistical Analysis
The data were analyzed using SPSS software version 22 for Windows (SPSS, Chicago, IL, United States). Categorical variables were analyzed using the chi-square test or Fisher’s exact test, and continuous variables were analyzed by the Mann–Whitney U test. Data were processed using the Kruskal–Wallis test to avoid random significance when comparing several groups. Significance was set as a P value of <0.05 (two-tailed). GraphPad Prism version 8.0 (GraphPad, San Diego, CA, United States) was used to perform the analyses.
Results
Patient Demographics
A total of 527 yeast isolates were isolated from inpatient wards and preserved at the Research Center for Medical Mycology in a tertiary teaching hospital at Beijing, China. Of the yeast isolates investigated, 201 (38.1%) were isolated from female patients and 326 (61.9%) were from male patients. Patient age ranged from 1 h to 101 years (median, 66.0 years; interquartile range, 48.0–78.0 years). More than half of isolates (400, 75.9%) were isolated from ICU patients, of which 366 (69.4%) were detected in a respiratory ICU, 24 (4.6%) were from a surgery ICU, and the remaining were from a cardiothoracic ICU, a coronary ICU, and a comprehensive ICU. Fifty-eight (11.0%) patients were hospitalized in surgical wards, and four (0.8%) were bone marrow transplant patients. The percentages of the different age groups were as follows: 0–1 year, 39 (7.4%); 2–14 years, 28 (5.3%); 15–49 years, 74 (14.0%); 50–65 years, 119 (22.6%); and over 65 years, 267 (50.7%). There was variation in species with age group. C. albicans was most common in patients 0–14 years of age (43, 63.6%), and there were 7 (10.4%)with C. parapsilosis complex in this age group. In patients aged over 65 years, C. albicans dominated, but the frequency rates of the C. parapsilosis complex (53, 19.9%) and C. glabrata (30, 11.2%) were slightly higher compared to the overall average (17.3%, 9.9%; P > 0.05). Among these, 138 (51.7%) were from ICU patients.
Species Distribution Over 10 Years
A total of 19 yeast species were identified within the 527 isolates. Our study showed a decrease in the isolation of C. albicans and an increasing prevalence of non-C. albicans species over time. Although C. albicans remained the most frequently recovered species, it comprised less than half of all isolates (259, 49.2%). C. parapsilosis complex isolates were 92 (17.5%) (of which 91 were C. parapsilosis sensu stricto and 1 was C. metapsilosis). C. tropicalis (73, 13.9%) was the third most common species, followed by C. glabrata (52, 9.9%), Cryptococcus neoformans (14, 2.7%), C. krusei (12, 2.3%), Trichosporon asahii (4, 0.8%), and Rhodotorula glutinis (4, 0.8%). The prevalence rates of rare species, such as Candida lusitaniae, Candida guilliermondii, Candida inconspicua, Pichia anomalus, Candida carpophila, Candida utilis, Candida kefyr, Candida lipolytica, Cryptococcus curvatus, and Trichosporon inkin, were collectively <1% (Supplementary Table 2).
Overall, yeast species isolated varied across years. More rare species were isolated in the recent 5 years; although C. albicans was the predominant single species, non-C. albicans species constituted >50% of isolates in 6 out of 10 years. The non-C. albicans species identified most frequently were C. parapsilosis sensu stricto, C. tropicalis, and C. glabrata (Figure 1).
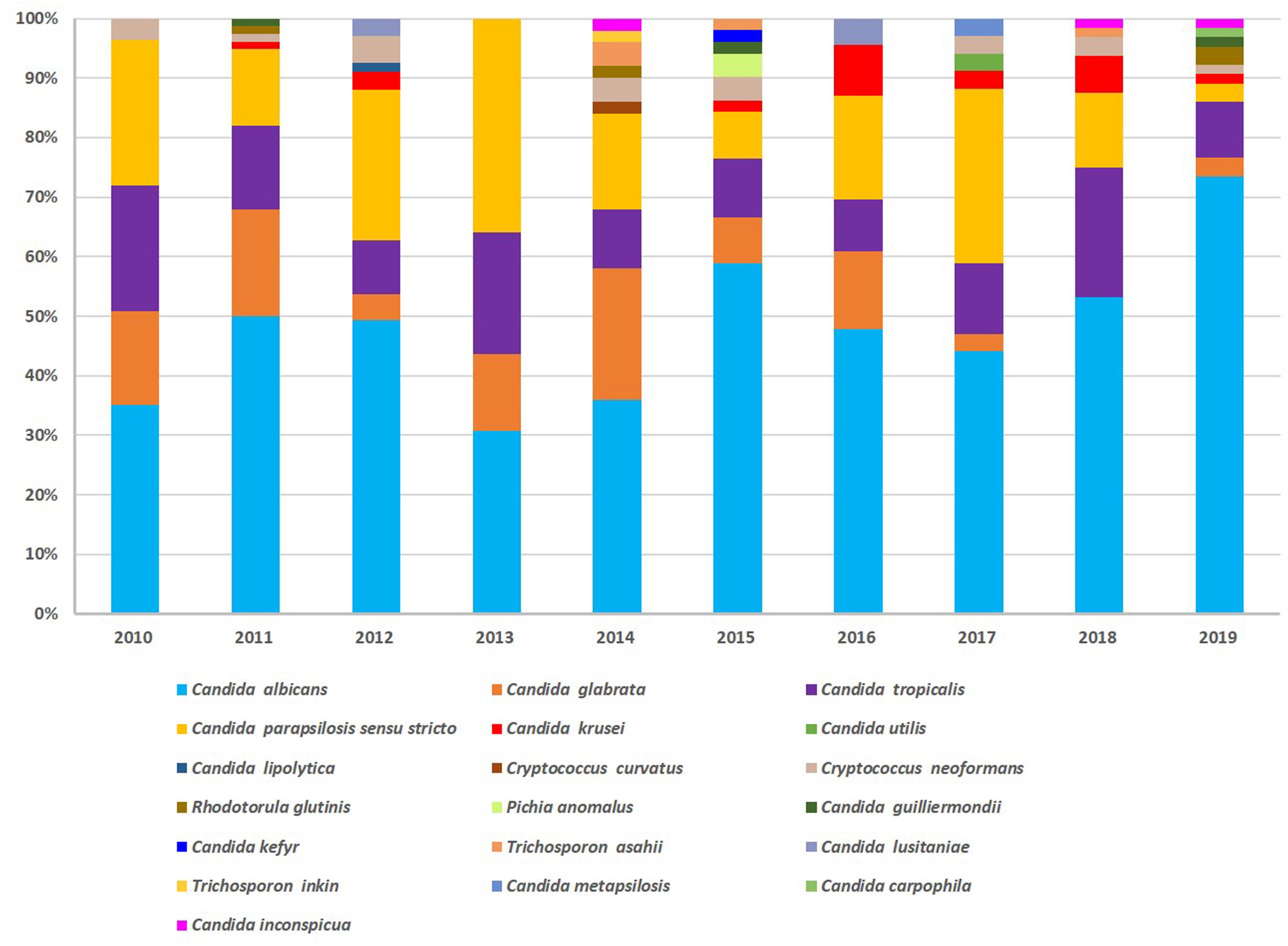
Figure 1. Species distribution over 10 years of surveillance. Yeast species varied across years. More rare species were isolated in the recent 5 years; C. albicans was the predominant single species. Non-C. albicans species identified most frequently were C. parapsilosis sensu stricto, C. tropicalis, and C. glabrata.
Specimen Sources of Yeast Isolates
Concerning sample type, all yeast isolates were isolated from clinical samples, of which blood culture isolates made up 184 (34.9%) and lower respiratory BAL fluid (BALF) accounted for 143 (27.1%). Eighty (15.2%) were detected in ascitic fluid, 39 (7.4%) in pleural fluid, 22 (4.2%) in pus, 19 (3.6%) in cerebrospinal fluid, and 16 (3.0%) in peritoneal dialysis. While CVC tips, bile, tissue, hydrarthrosis, and bone marrow specimens yielded yeast isolates uncommonly (<5%), blood, BALF, and ascitic fluid were the top three most common specimens over 10 years, and yeast species isolated from blood were more frequent than those from other specimens (χ2 = 527.000, P = 0.000) (Supplementary Table 3).
Candida species isolates were broadly distributed and made up the majority of the blood culture isolates (174, 94.6%). Cryptococcus and non-Candida yeast isolates accounted for 5.4% of the yeast isolates from blood cultures. The majority of the yeast isolates from ascitic fluid (78, 97.5%) and pleural fluid (37, 94.9%) were Candida spp., and Candida spp. were the only yeast isolates from CVC, bile, hydrarthrosis, and tissue. Thirteen (68.4%) of the yeast isolates from CSF samples were Candida spp. and exceeded C. neoformans. There was a single isolate of T. asahii from an ascitic fluid sample (Supplementary Table 3).
Thirteen yeast species were involved in fungemia, of which C. albicans was 61 (33.2%). C. parapsilosis sensu stricto (48, 26.1%) was the leading species of non-C. albicans in candidemia patients, followed by C. glabrata (31, 16.8%) and C. tropicalis (26, 14.1%), and C. krusei (4, 2.2%). The frequency of R. glutinis, C. neoformans, T. asahii, and P. anomalus was collectively <1% (Supplementary Table 4).
In vitro Susceptibility to Azoles
The susceptibility of the 527 yeast isolates to antifungals is presented in Table 1. Generally, C. albicans and C. parapsilosis complex isolates were susceptible or exhibited WT MICs to all four azoles (prevalence of isolates exhibiting resistance or NWT MICs was <5%) during the 10 years. Azole susceptibility was less common among C. tropicalis isolates, fluconazole and voriconazole susceptibility was exhibited by only 42 (57.5%) isolates and 31 (42.5%) isolates, respectively; furthermore, 19 (26.1%) isolates were cross-resistant to fluconazole and voriconazole, and 51 (69.9%) C. tropicalis isolates exhibited NWT MICs to posaconazole (Supplementary Table 5). The overall prevalence of fluconazole resistance in the C. glabrata population was 14 (26.9%), and the prevalence of C. glabrata isolates exhibiting NWT to voriconazole was 33 (63.5%). Among C. krusei, all isolates showed the WT phenotype to itraconazole, while resistance was more prevalent (8.3%) to voriconazole and posaconazole. However, the remaining non-C. albicans species (those with >2.5% prevalence), including C. guilliermondii, C. lusitaniae, C. inconspicua, C. lipolytica, C. utilis, C. kefyr, and C. carpophila, exhibited reduced azole susceptibility, and their 50% MIC (MIC50), MIC90, and geometric mean MIC were twofold to 15-fold higher than those of C. albicans. The overall cross-resistant prevalence rates in common Candida species were 23 (44.2%) in C. glabrata, 24 (32.9%) in C. tropicalis, 1 (8.3%) in C. krusei, and 12 (4.6%) in C. albicans (Supplementary Table 5).
The overall prevalence of antifungal agents resistance in 184 blood culture isolates was 25 (13.6%). For C. tropicalis and C. glabrata, resistance was more prevalent to at least one triazole [19 (73.1%) and 23 (74.2%), respectively]. Triazole cross-resistance prevalence rates were also high in C. tropicalis (10, 38.5%) and C. glabrata (19, 61.3%) isolates. Resistance to echinocandins was detected in two (3.3%) C. albicans isolates and one (3.8%) C. tropicalis isolate (Supplementary Table 6).
In vitro Susceptibility to Echinocandins, Amphotericin B, and Flucytosine
Resistance to one or more of the echinocandins was distinctly uncommon among isolates of C. albicans (0.8–1.6%), C. parapsilosis (0.0%), C. tropicalis (1.4–2.7%), and C. krusei (0.0%). The prevalence rates of resistance to anidulafungin (5, 9.6%), caspofungin (4, 7.7%), and micafungin (5, 9.6%) were most prominent among C. glabrata isolates. No trend toward increasing resistance was seen over time for any of these species, although a higher prevalence of resistant isolates was detected in C. tropicalis in 2013–2014 and in C. glabrata in 2017–2019. Cross-resistance to two or three echinocandins was observed in three (1.2%) C. albicans isolates, five (9.6%) C. glabrata isolates, and one (1.4%) C. tropicalis isolates.
Amphotericin B also showed good overall activity against common Candida species, with WT rates of 100% (Table 1). The MIC50 and MIC90 values of amphotericin B were 1 and 2 μg/ml for C. krusei and C. neoformans, respectively, and were either 0.5 and 1 μg/ml for C. lipolytica, C. guilliermondii, C. carpophila, and C. kefyr species (Table 1). Rare species exhibited amphotericin B MICs of 0.12–2 mg/L (Table 1), which are considered WT for other Candida species. Less than 6% of common Candida species were of NWT phenotype to flucytosine, while C. tropicalis and C. krusei were 100% susceptible to flucytosine (Table 1).
Multidrug Resistance
For species with established ECVs and CBPs, we analyzed antifungal multidrug resistance (i.e., isolates that were resistant or NWT to at least two classes of antifungal agents tested).
Only six isolates (two C. albicans, two C. glabrata, and two C. tropicalis) showed multidrug resistance to two classes of antifungal agents (<3% for each species), of which one C. albicans and one C. glabrata isolates were multidrug resistant to azoles, echinocandins, and flucytosine.
Trends in Fluconazole and Voriconazole Resistance Over Time
Trends in fluconazole and voriconazole resistance/NWT for common Candida species over time are shown in Table 2. Generally, fluconazole resistance in C. albicans slightly increased from 2012 (3.0%) to 2018 (5.9%), but this increase was not statistically significant (P > 0.05; Table 2). A significantly higher prevalence of resistant isolates was detected in C. tropicalis to fluconazole and voriconazole (frequency of resistance from <10 to 75%) over 10 years, C. glabrata to voriconazole (NWT phenotype increased from <25 to 40%; Table 2), and C. parapsilosis complex to fluconazole (from <6 to 9%). There were no trends in resistance for other species in this study (Table 2).
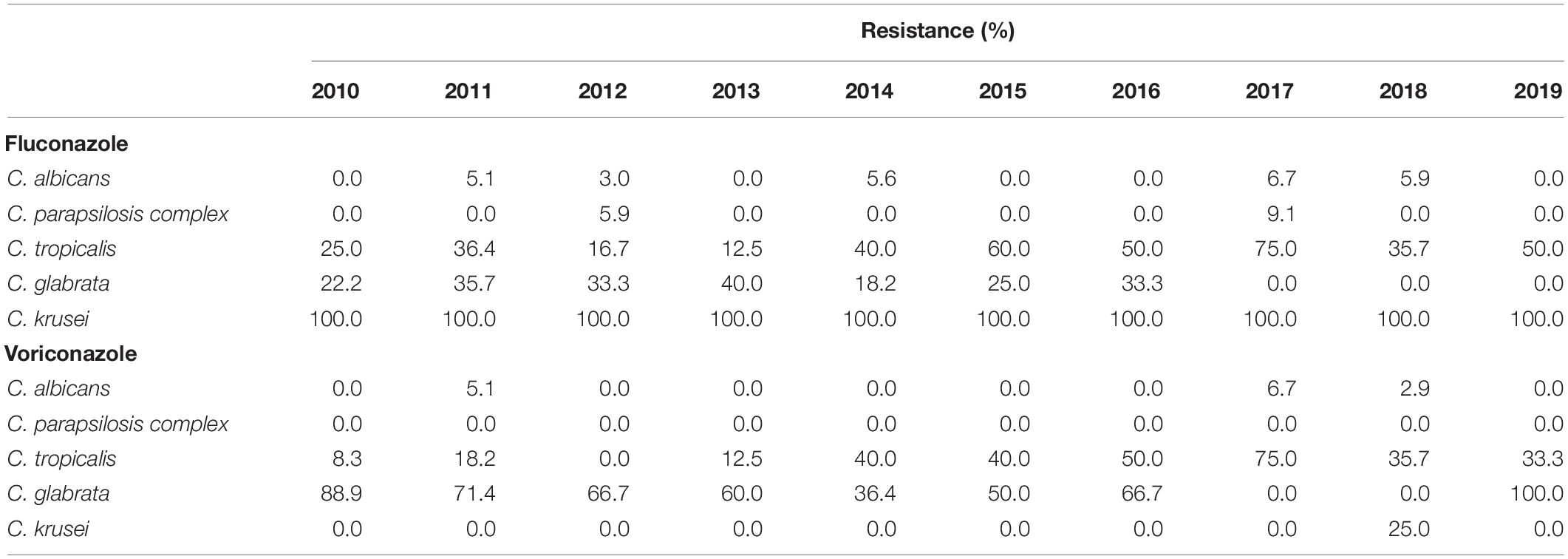
Table 2. Trends of fluconazole and voriconazole resistance or non-wild-type phenotype in Candida species over 10 years.
Discussion
The landscape of invasive mycoses is in a continuous evolution with important implications for their diagnosis and treatment. The overall burden remains high, particularly in neonates and the elderly; patients admitted to intensive care units and using prostheses, catheters, or other intravenous devices; those receiving different immunosuppressant treatments or antineoplastic chemotherapy; or transplant recipients (Lortholary et al., 2014; Quindós, 2018; Pfaller et al., 2019). In this 10-year surveillance study, the etiology of invasive candidiasis progressively shifts from C. albicans to other species of Candida, and the proportion of non-C. albicans was over 50% in 6 out of 10 years, of which the C. parapsilosis complex, C. tropicalis, C. glabrata, and C. krusei accounted for 43.4% collectively, while C. albicans represents more than 50% of isolates in 2018 and more than 70% in 2019 due to the high frequency of C. albicans isolated from BALF, with 12 (35.3%) in 2018 and 31 (66.0%) in 2019. The prevalence of C. parapsilosis sensu stricto is much higher than C. tropicalis and C. glabrata (17.3% versus 13.9 and 9.9%). In addition, large nosocomial outbreaks due to C. parapsilosis sensu stricto have been reported in NICU patients and adult wards from tertiary hospitals in China (Wang et al., 2016a, b; Qi et al., 2018). Rarer species accounted for 4.5% in this study, including C. inconspicua, C. utilis, C. lipolytica, C. kefyr, C. curvatus, C. carpophila, and T. inkin. The species varied gradually over time, and more species were present in the recent 5 years. This increasing prevalence of non-C. albicans species over time has been noted in other studies, which is generally considered to be associated with reduced antifungal susceptibilities (Kullberg and Arendrup, 2015; Perlin et al., 2017; Lamoth et al., 2018).
Variation in species distribution was noted among different clinical services and age groups; for example, C. parapsilosis sensu stricto was predominant in ICU admissions and among patients aged 65 years and older. This may be because potential risk factors for candidiasis were more common in such patient population, regarding invasive procedures in ICU, the aging of the population, and previous antibiotic exposure (Kullberg and Arendrup, 2015). The trend for increasing C. parapsilosis was seen in tertiary university-affiliated hospitals located in southwest China as well (Jia et al., 2018). The picture is somewhat different in a teaching hospital in central China, where the predominant species was C. tropicalis (25.1%), followed by the C. parapsilosis complex (17.3%) and C. glabrata (9%) (Xu et al., 2019). The trend for increasing C. glabrata was reported in Australia and in some Latin American and African countries (Nucci et al., 2013; Doi et al., 2016; Govender et al., 2016). The difference showed the geographic and temporal variations in the frequency of Candida species.
The epidemiology of candidemia has been described in numerous single-center and population-based surveys conducted worldwide (Guinea, 2014; Yang et al., 2014; Astvad et al., 2018; Lamoth et al., 2018). As our results showed, in the 184 isolates from candidemia patients, the proportion of non-C. albicans species exceeded that of C. albicans to become the most common among patients infected with candidemia, in accordance with some previous studies from local China, Asia-Pacific regions, and European countries (Liu et al., 2014; Tan et al., 2016; Wang et al., 2016a; Guo et al., 2017; Pappas et al., 2018; Zeng et al., 2019). In addition, the C. parapsilosis complex (26.1%) was the second most prevalent Candida spp. isolated in our study, the frequencies of which were much higher than those reported in Southern Europe and Southeast Asia (Siri et al., 2017; Teo et al., 2017). Another report analyzed patients with candidemia in the ICU in a teaching hospital in Beijing, with similar trends of the C. parapsilosis complex (19.5%), C. glabrata (15.9%), and C. tropicalis (14.6%) (Xiao et al., 2019), while the prevalence of C. tropicalis is much higher in some other reports (Ma et al., 2013; Pfaller et al., 2019; Xiao et al., 2020).
In our study, most Candida species isolates were susceptible to the antifungal agents. For example, C. albicans and the C. parapsilosis complex have low incidences of fluconazole resistance, at 5.0 and 4.3%, respectively. The low fluconazole resistance prevalence among C. albicans isolates was consistent with previous reports (Liu et al., 2014; Guo et al., 2017; Brilhante et al., 2018; Zeng et al., 2019). But a trend toward increased resistance or the emergence of naturally resistant species was observed among other Candida spp. Our study confirmed that azole resistance was mainly observed in C. tropicalis and C. glabrata isolates over 10 years. Moreover, the resistance prevalence rates to fluconazole (35.6%) and voriconazole (26.0%) in C. tropicalis isolates were much higher than those reported in the single-center study in southwest China (Jia et al., 2018) and CHIF-NET study in China (Guo et al., 2017; Xiao et al., 2020). Notably, 32.9% of C. tropicalis isolates were cross-resistant to azoles, but no isolate was multidrug resistant to azoles, flucytosine, and amphotericin B. In C. glabrata species, the prevalence rates of fluconazole (26.9%) and voriconazole (63.5%) resistance in this study were much higher than those reported in the United States, Australia, and several European countries, which also have increasing trends in prevalence of C. glabrata over time, with 6–15% of isolates being resistant to fluconazole (Chapman et al., 2017; Lamoth et al., 2018; Pfaller et al., 2019). There has been high fluconazole and azole cross-resistance prevalence (>30%) of C. glabrata in this study. This 10-year surveillance study identified a sharp increase in fluconazole and voriconazole resistance prevalence of C. tropicalis (from <10 to 75%) over 10 years and C. glabrata to voriconazole (from <25 to 40%). The C. parapsilosis complex showed a slightly increase to fluconazole and voriconazole resistance prevalence (from <6% in 2012 to 9% in 2017), and fluconazole resistance in C. albicans increased from 3.0% in 2012 to 5.9% in 2018. C. glabrata and C. tropicalis are considered to exhibit moderate- to high-level intrinsic azole resistance, with the prevalence and resistance prevalence varying with geographic region (Kullberg and Arendrup, 2015; Fan et al., 2017; Perlin et al., 2017).
Another noteworthy finding of our study was the emergence of echinocandin resistance. High-level echinocandin resistance was mainly observed in C. glabrata, with resistance prevalence rates of 9.6% to anidulafungin, 9.6% to micafungin, and 7.7% to caspofungin, all higher than those seen in large-scale surveillance studies in China and Europe (Pham et al., 2014; Klotz et al., 2016; Xiao et al., 2020), and a higher prevalence rate close to or more than 10% in C. glabrata is also reported by some institutional studies in North America (Alexander et al., 2013; Farmakiotis et al., 2014). Whether this difference is because of strain types, clinical practice issues, or both is unclear. Notably, one C. glabrata isolate was multidrug resistant to azoles, echinocandins, and flucytosine in this study. Previous studies reported that echinocandin resistance in susceptible Candida species arises after repeated or long-term exposure (Lortholary et al., 2011; Fekkar et al., 2014; Perlin et al., 2017), and an increase in echinocandin resistance among C. glabrata is often accompanied by azole resistance, resulting in multidrug-resistant isolates (Alexander et al., 2013; Pham et al., 2014).
From our study, the increasing prevalence of non-C. albicans species over time has been noted. The emergence of azole resistance among non-C. albicans is particularly concerning, followed by echinocandin and multidrug resistance among some Candida species, especially C. tropicalis and C. glabrata. Notably, C. glabrata can acquire resistance to azoles and echinocandins as single-drug classes, as well as multidrug resistance involving all major drug classes. Azole resistance among Candida spp. involves several well-defined mechanisms, including upregulation of drug transporters, overexpression or alteration of the drug target, and cellular changes caused, in some cases, by nontarget effects induced by stress responses (Cowen et al., 2015; Perlin et al., 2017). Genetic factors, such as DNA repair, and chromosomal abnormalities help to induce drug-resistant phenotypes. Drug exposure also drives the emergence of resistance (Perlin et al., 2017). Numerous point mutations in ERG11 have been reported in response to fluconazole. Investigation has shown that the ERG11 mutation A395T was the major resistance mechanism, which was responsible for >83% of azole resistance in China (Fan et al., 2019). Echinocandin resistance is uncommon but is conferred by hot spot amino acid substitutions in glucan synthase. Candida isolates that are potentially echinocandin resistant or less susceptible often harbor amino acid substitutions in the Fks1p (and/or Fks2p in C. glabrata) gene (Alexander et al., 2013; Pham et al., 2014). Therefore, it is essential to screen the isolates with drug MIC values in the intermediate or resistant range to determine the mutations in FKS1 and FKS2 genes based on our surveillance data and compare the changes in MIC values caused by those mutations. Susceptibility testing of azoles and echinocandins, detection of the molecular mechanism of resistance, and combined antifungal treatment outcomes will allow more informed determinations of the value of these drugs in the antifungal armamentarium.
Our study has some limitations. First, this was a single-center retrospective study, so our results may not be generalizable to all patients with candidiasis and extrapolated to other institutions as the epidemiology of candidiasis can be highly institution specific. Second, this was a laboratory-based study, more detailed demographic and clinical characteristics were not collected, but it is the intention to include the collection of such data prospectively from this time forward. Nevertheless, this study provides important epidemiological findings which are instrumental in designing strategies for better management of candidiasis in our hospital.
In conclusion, our study has provided important updated information at a tertiary hospital in China on species distribution and antifungal susceptibility of yeast species. Over the 10-year surveillance, non-C. albicans species have become the predominant cause of nosocomial candidemia, and azole resistance is notable among C tropicalis and C. glabrata isolates. Multidrug resistance has emerged in C. glabrata, while overall resistance to echinocandins and flucytosine remains rare. Amphotericin B demonstrated excellent activity against all Candida species over the years. Continued surveillance, especially of national antifungal susceptibility trends, is warranted. The epidemiological findings in our study will pave the way for more in-depth studies and help us establish better antifungal stewardship in our hospital.
Data Availability Statement
All datasets generated for this study are included in the article/Supplementary Material.
Ethics Statement
All the strains isolated from patients were preserved in the Research Center for Medical Mycology at Peking University First Hospital. We conducted a retrospective study on the isolates and patient data collection including age and gender from electronic laboratory records. The study was approved by the ethics committee of Peking University First Hospital. The need for informed consent was waived by the Clinical Research Ethics Committee.
Author Contributions
RL designed the experiments and supervised the data analysis. YS performed the antifungal susceptibility testing and wrote the manuscript. YS, XC, ZW, YY, and WL collected and analyzed the data. All authors discussed the results and commented on the manuscript.
Funding
This study was supported by Major Infectious Diseases Such as AIDS and Viral Hepatitis Prevention and Control Technology Major Projects (2018ZX10712001-011).
Conflict of Interest
The authors declare that the research was conducted in the absence of any commercial or financial relationships that could be construed as a potential conflict of interest.
Supplementary Material
The Supplementary Material for this article can be found online at: https://www.frontiersin.org/articles/10.3389/fmicb.2020.01401/full#supplementary-material
References
Alexander, B. D., Johnson, M. D., Pfeiffer, C. D., Jiménez-Ortigosa, C., Catania, J., Booker, R., et al. (2013). Increasing echinocandin resistance in Candida glabrata: clinical failure correlates with presence of FKS mutations and elevated minimum inhibitory concentrations. Clin. Infect. Dis. 56, 1724–1732. doi: 10.1093/cid/cit136
Alves, J., Palma, P., Azevedo, D., and Rello, J. (2018). Candidemia in the patient with malignancy. Hosp. Pract. 46, 246–252. doi: 10.1080/21548331.2018.1508290
Andes, D. R., Safdar, N., Baddley, J. W., Playford, G., Reboli, A. C., Rex, J. H., et al. (2012). Impact of treatment strategy on outcomes in patients with candidemia and other forms of invasive candidiasis: a patient-level quantitative review of randomized trials. Clin. Infect. Dis. 54, 1110–1122. doi: 10.1093/cid/cis021
Astvad, K. M. T., Johansen, H. K., Røder, B. L., Rosenvinge, F. S., Knudsen, J. D., Lemming, L., et al. (2018). Update from a 12-year nationwide fungemia surveillance: increasing intrinsic and acquired resistance causes concern. J. Clin. Microbiol. 56, e1564–e1567. doi: 10.1128/JCM.01564-17
Berdal, J. E., Haagensen, R., Ranheim, T., and Bjørnholt, J. V. (2014). Nosocomial candidemia; risk factors and prognosis revisited; 11 years experience from a Norwegian secondary hospital. PLoS One 9:e103916. doi: 10.1371/journal.pone.0103916
Bitar, D., Lortholary, O., Le Strat, Y., Nicolau, J., Coignard, B., Tattevin, P., et al. (2014). Population-based analysis of invasive fungal infections, France, 2001-2010. Emerg. Infect. Dis. 20, 1149–1155. doi: 10.3201/eid2007.140087
Brilhante, R. S. N., Sales, J. A., da Silva, M. L. Q., de Oliveira, J. S., Pereira, L. D. A., Pereira-Neto, W. A., et al. (2018). Antifungal susceptibility and virulence of Candida parapsilosis species complex: an overview of their pathogenic potential. J. Med. Microbiol. 67, 903–914. doi: 10.1099/jmm.0.000756
Canela, H. M. S., Cardoso, B., Vitali, L. H., Coelho, H. C., Martinez, R., and Ferreira, M. E. D. S. (2018). Prevalence, virulence factors and antifungal susceptibility of Candida spp. isolated from bloodstream infections in a tertiary care hospital in Brazil. Mycoses 61, 11–21. doi: 10.1111/myc.12695
Canton, E., Peman, J., Hervas, D., Iñiguez, C., Navarro, D., Echeverría, J., et al. (2012). Comparison of three statistical methods for establishing tentative wild-type population and epidemiological cutoff values for echinocandins, amphotericin B, flucytosine, and six Candida species as determined by the colorimetric Sensititre YeastOne method. J. Clin. Microbiol. 50, 3921–3926. doi: 10.1128/JCM.01730-12
Castanheira, M., Messer, S. A., Rhomberg, P. R., and Pfaller, M. A. (2016). Antifungal susceptibility patterns of a global collection of fungal isolates: results of the SENTRY antifungal surveillance program (2013). Diagn. Microbiol. Infect. Dis. 85, 200–204. doi: 10.1016/j.diagmicrobio.2016.02.009
Castanheira, M., Woosley, L. N., Messer, S. A., Diekema, D. J., Jones, R. N., and Pfaller, M. A. (2014). Frequency of fks mutations among Candida glabrata isolates from a 10-year global collection of bloodstream infection isolates. Antimicrob. Agents Chemother. 58, 577–580. doi: 10.1128/AAC.01674-13
Chapman, B., Slavin, M., Marriott, D., Halliday, C., Kidd, S., Arthur, I., et al. (2017). Changing epidemiology of candidaemia in Australia. J. Antimicrob. Chemother. 72, 1103–1108. doi: 10.1093/jac/dkw422
Cleveland, A. A., Harrison, L. H., Farley, M. M., Hollick, R., Stein, B., Chiller, T. M., et al. (2015). Declining incidence of candidemia and the shifting epidemiology of Candida resistance in two US metropolitan areas, 2008-2013: results from population-based surveillance. PLoS One 10:e0120452. doi: 10.1371/journal.pone.0120452
CLSI, (2017). M60. Performance Standards for Antifungal Susceptibility Testing of Yeasts, 1st Edn. Wayne, PA: Clinical and Laboratory Standards Institute.
CLSI, (2018). M59. Epidemiological Cutoff Values for Antifungal Susceptibility Testing, 2nd Edn. Wayne, PA: Clinical and Laboratory Standards Institute.
Cowen, L. E., Sanglard, D., Howard, S. J., Rogers, P. D., and Perlin, D. S. (2015). Mechanisms of antifungal drug resistance. Cold. Spring. Harb. Perspect. Med. 5, a019752–a019773. doi: 10.1101/cshperspect.a019752
Cuenca-Estrella, M., and Rodriguez-Tudela, J. L. (2010). The current role of the reference procedures by CLSI and EUCAST in the detection of resistance to antifungal agents in vitro. Expert. Rev. Anti. Infect. Ther. 8, 267–276. doi: 10.1586/eri.10.2
Dabas, Y., Xess, I., and Kale, P. (2017). Molecular and antifungal susceptibility study on trichosporonemia and emergence of Trichosporon mycotoxinivorans as a bloodstream pathogen. Med. Mycol. 55, 518–527. doi: 10.1093/mmy/myw100
De Pauw, B., Walsh, T. J., Donnelly, J. P., Stevens, D. A., Edwards, J. E., Calandra, T., et al. (2008). Revised definitions of invasive fungal disease from the European Organization for Research and Treatment of Cancer/Invasive Fungal Infections Cooperative Group and the National Institute of Allergy and Infectious Diseases Mycoses Study Group (EORTC/MSG) Consensus Group. Clin. Infect. Dis. 46, 1813–1821. doi: 10.1086/588660
Diekema, D., Arbefeville, S., Boyken, L., Kroeger, J., and Pfaller, M. (2012). The changing epidemiology of healthcare-associated candidemia over three decades. Diagn. Microbiol. Infect. Dis. 73, 45–48. doi: 10.1016/j.diagmicrobio.2012.02.001
Ding, Y. L., Shen, N., Zhou, Q. T., He, B., Zheng, J. J., and Zhao, X. M. (2018). Clinical analysis of candidemia in immunocompetent patients. Beijing Da Xue Xue Bao Yi Xue Ban 50, 1063–1069. doi: 10.1016/j.diagmicrobio.2012.02.001
Doi, A. M., Pignatari, A. C., Edmond, M. B., Marra, A. R., Camargo, L. F. A., Siqueira, R. A., et al. (2016). Epidemiology and microbiologic characterization of nosocomial candidemia from a brazilian national surveillance program. PLoS One 11:e0146909. doi: 10.1371/journal.pone.0146909
Epelbaum, O., and Chasan, R. (2017). Candidemia in the intensive care unit. Clin. Chest. Med. 38, 493–509. doi: 10.1016/j.ccm.2017.04.010
Eschenauer, G. A., Nguyen, M. H., Shoham, S., Vazquez, J. A., Morris, A. J., Pasculle, W. A., et al. (2014). Real-world experience with echinocandin MICs against Candida species in a multicenter study of hospitals that routinely perform susceptibility testing of bloodstream isolates. Antimicrob. Agents Chemother. 58, 1897–1906. doi: 10.1128/AAC.02163-13
Espinel-Ingroff, A., Alvarez-Fernandez, M., Cantón, E., Carver, P. L., Chen, S. C. A., Eschenauer, G., et al. (2015). Multicenter study of epidemiological cutoff values and detection of resistance in Candida spp. to anidulafungin, caspofungin, and micafungin using the sensititre yeastone colorimetric method. Antimicrob. Agents Chemother. 59, 6725–6732. doi: 10.1128/AAC.01250-15
Espinel-Ingroff, A., Turnidge, J., Alastruey-Izquierdo, A., Botterel, F., Canton, E., Castro, C., et al. (2019). Method-dependent epidemiological cutoff values for detection of triazole resistance in Candida and Aspergillus species for the sensititre yeastone colorimetric broth and etest agar diffusion methods. Antimicrob. Agents Chemother. 63:e1651-18. doi: 10.1128/AAC.01651-18
Fan, X., Xiao, M., Liao, K., Kudinha, T., Wang, H., Zhang, L., et al. (2017). Notable increasing trend in azole non-susceptible Candida tropicalis causing invasive candidiasis in China (August 2009 to July 2014): molecular epidemiology and clinical azole consumption. Front. Microbiol. 8:464. doi: 10.3389/fmicb.2017.00464
Fan, X., Xiao, M., Zhang, D., Huang, J. J., Wang, H., Hou, X., et al. (2019). Molecular mechanisms of azole resistance in Candida tropicalis isolates causing invasive candidiasis in China. Clin. Microbiol. Infect. 25, 885-891. doi: 10.1016/j.cmi.2018.11.007
Farmakiotis, D., Tarrand, J. J., and Kontoyiannis, D. P. (2014). Drug-resistant Candida glabrata infection in cancer patients. Emerg. Infect. Dis. 20, 1833–1840. doi: 10.3201/eid2011.140685
Fekkar, A., Dannaoui, E., Meyer, I., Imbert, S., Brossas, J. Y., Uzunov, M., et al. (2014). Emergence of echinocandin-resistant Candida spp in a hospital setting: a consequence of 10 years of increasing use of antifungal therapy? Eur. J. Clin. Microbiol. Infect. Dis. 33, 1489–1496. doi: 10.1007/s10096-014-2096-9
Govender, N. P., Patel, J., Magobo, R. E., Naicker, S., Wadula, J., Whitelaw, A., et al. (2016). Emergence of azole-resistant Candida parapsilosis causing bloodstream infection: results from laboratory-based sentinel surveillance in South Africa. J. Antimicrob. Chemother. 71, 1994–2004. doi: 10.1093/jac/dkw091
Guinea, J. (2014). Global trends in the distribution of Candida species causing candidemia. Clin. Microbiol. Infect. 20, 5–10. doi: 10.1111/1469-0691.12539
Guo, L. N., Xiao, M., Cao, B., Qu, F., Zhan, Y. L., Hu, Y. J., et al. (2017). Epidemiology and antifungal susceptibilities of yeast isolates causing invasive infections across urban Beijing, China. Future Microbiol. 12, 1075–1086. doi: 10.2217/fmb-2017-0036
Kal Çakmaklıoğulları, E., Aşgın, N., and Değerli, K. (2019). A comparison of the costs, reliability and time of result periods of widely used methods, new molecular methods and MALDI TOF-MS in the routine diagnosis of Candida strains. Mikrobiyol. Bul. 53, 204–212. doi: 10.5578/mb.67952
Klotz, U., Schmidt, D., Willinger, B., Steinmann, E., Buer, J., Rath, P. M., et al. (2016). Echinocandin resistance and population structure of invasive Candida glabrata isolates from two university hospitals in Germany and Austria. Mycoses 59, 312–318. doi: 10.1111/myc.12472
Kullberg, B. J., and Arendrup, M. C. (2015). Invasive Candidiasis. N. Engl. J. Med. 373, 1445–1456. doi: 10.1056/NEJMra1315399
Jia, X., Li, C., Cao, J., Wu, X., and Zhang, L. (2018). Clinical characteristics and predictors of mortality in patients with candidemia: a six-year retrospective study. Eur. J. Clin. Microbiol. Infect. Dis. 37, 1717–1724. doi: 10.1007/s10096-018-3304-9
Lamoth, F., Lockhart, S. R., Berkow, E. L., and Calandra, T. (2018). Changes in the epidemiological landscape of invasive candidiasis. J. Antimicrob. Chemother. 73, i4-i13. doi: 10.1093/jac/dkx444
Lewis, J. S. II, Wiederhold, N. P., Wickes, B. L., Patterson, T. F., and Jorgensen, J. H. (2013). Rapid emergence of echinocandin resistance in Candida glabrata resulting in clinical and microbiologic failure. Antimicrob. Agents Chemother. 57, 4559–4561. doi: 10.1128/AAC.01144-13
Liu, W., Tan, J., Sun, J., Xu, Z., Li, M., Yang, Q., et al. (2014). Invasive candidiasis in intensive care units in China: in vitro antifungal susceptibility in the China-SCAN study. J. Antimicrob. Chemother. 69, 162–167. doi: 10.1093/jac/dkt330
Lortholary, O., Desnos-Ollivier, M., Sitbon, K., Fontanet, A., Bretagne, S., Dromer, F., et al. (2011). Recent exposure to caspofungin or fluconazole influences the epidemiology of candidemia: a prospective multicenter study involving 2,441 patients. Antimicrob. Agents Chemother. 55, 532–538. doi: 10.1128/AAC.01128-10
Lortholary, O., Renaudat, C., Sitbon, K., Madec, Y., Denoeud-Ndam, L., Wolff, M., et al. (2014). Worrisome trends in incidence and mortality of candidemia in intensive care units (Paris area, 2002-2010). Inten. Care Med. 40, 1303–1312. doi: 10.1007/s00134-014-3408-3
Ma, C. F., Li, F. Q., Shi, L. N., Hu, Y. A., Wang, Y., Huang, M., et al. (2013). Surveillance study of species distribution, antifungal susceptibility and mortality of nosocomial candidemia in a tertiary care hospital in China. BMC Infect. Dis. 13:337. doi: 10.1186/1471-2334-13-337
Maldonado, I., Cataldi, S., Garbasz, C., Relloso, S., Striebeck, P., Guelfand, L., et al. (2018). Identification of Candida yeasts: conventional methods and MALDI-TOF MS. Rev. Iberoam. Micol. 35, 151–154. doi: 10.1016/j.riam.2018.02.002
Mantadakis, E., Pana, Z. D., and Zaoutis, T. (2018). Candidemia in children, epidemiology, prevention and management. Mycoses 61, 614–622. doi: 10.1111/myc.12792
McCarty, T. P., and Pappas, P. G. (2016). Invasive candidiasis. J. Infect. Dis. Clin. North Am. 30, 103–124. doi: 10.1016/j.idc.2015.10.013
Mora-Duarte, J., Betts, R., Rotstein, C., Colombo, A. L., Thompson-Moya, L., Smietana, J., et al. (2002). Comparison of caspofungin and amphotericin B for invasive candidiasis. N. Engl. J. Med. 347, 2020–2029. doi: 10.1056/NEJMoa021585
Neji, S., Hadrich, I., Trabelsi, H., Abbes, S., Cheikhrouhou, F., Sellami, H., et al. (2017). Virulence factors, antifungal susceptibility and molecular mechanisms of azole resistance among Candida parapsilosis complex isolates recovered from clinical specimens. J. Biomed. Sci. 24, 67–82. doi: 10.1186/s12929-017-0376-2
Nucci, M., Queiroz-Telles, F., Alvarado-Matute, T., Tiraboschi, I. R., Cortes, J., Zurita, J., et al. (2013). Epidemiology of candidemia in Latin America: a laboratory-based survey. PLoS One 8:e59373–e59379. doi: 10.1371/journal.pone.0059373
Pappas, P. G., Kauffman, C. A., Andes, D. R., Clancy, C. J., Marr, K. A., Ostrosky-Zeichner, L., et al. (2016). Clinical practice guideline for the management of candidiasis: 2016 update by the Infectious Diseases Society of America. Clin. Infect. Dis. 62, e1–e50. doi: 10.1093/cid/civ933
Pappas, P. G., Lionakis, M. S., Arendrup, M. C., Ostrosky-Zeichner, L., and Kullberg, B. J. (2018). Invasive candidiasis. Nat. Rev. Dis. Primers 4, 18026–18045. doi: 10.1038/nrdp.2018.26
Perlin, D. S., Rautemaa-Richardson, R., and Alastruey-Izquierdo, A. (2017). The global problem of antifungal resistance: prevalence, mechanisms, and management. Lancet Infect. Dis. 17, e383–e392. doi: 10.1016/s1473-3099(17)30316-x
Pfaller, M., Neofytos, D., Diekema, D., Azie, N., Meier-Kriesche, H. U., Quan, S. P., et al. (2012). Epidemiology and outcomes of candidemia in 3648 patients: data from the prospective antifungal therapy (PATH Alliance®) registry, 2004-2008. Diagn. Microbiol. Infect. Dis. 74, 323–331. doi: 10.1016/j.diagmicrobio.2012.10.003
Pfaller, M. A. (2012). Antifungal drug resistance: mechanisms, epidemiology, and consequences for treatment. Am. J. Med. 125, S3–S13. doi: 10.1016/j.amjmed.2011.11.001
Pfaller, M. A., Castanheira, M., Messer, S. A., and Jones, R. N. (2015). In vitro antifungal susceptibilities of isolates of Candida spp. and Aspergillus spp. from China to nine systemically active antifungal agents: data from the SENTRY antifungal surveillance program, 2010 through 2012. Mycoses 58, 209-214. doi: 10.1111/myc.12299
Pfaller, M. A., and Diekema, D. J. (2012). Progress in antifungal susceptibility testing of Candida spp. by use of Clinical and Laboratory Standards Institute broth microdilution methods, 2010 to 2012. J. Clin. Microbiol. 50, 2846–2856. doi: 10.1128/JCM.00937-12
Pfaller, M. A., Diekema, D. J., Turnidge, J. D., Castanheira, M., and Jones, R. N. (2019). Twenty years of the SENTRY antifungal surveillance program: results for Candida species from 1997-2016. Open Forum Infect. Dis. 6, S79-S94. doi: 10.1093/ofid/ofy358
Pfaller, M. A., Moet, G. J., Messer, S. A., Jones, R. N., and Castanheira, M. (2011b). Geographic variations in species distribution and echinocandin and azole antifungal resistance rates among Candida bloodstream infection isolates: report from the SENTRY antimicrobial surveillance program (2008 to 2009). J. Clin. Microbiol. 49, 396–399. doi: 10.1128/JCM.01398-10
Pfaller, M. A., Messer, S. A., Moet, G. J., Jones, R. N., and Castanheira, M. (2011a). Candida bloodstream infections: comparison of species distribution and resistance to echinocandin and azole antifungal agents in Intensive Care Unit (ICU) and non-ICU settings in the SENTRY Antimicrobial Surveillance Program (2008-2009). Int. J. Antimicrob. Agents 38, 65–69. doi: 10.1016/j.ijantimicag.2011.02.016
Pham, C. D., Iqbal, N., Bolden, C. B., Kuykendall, R. J., Harrison, L. H., Farley, M. M., et al. (2014). Role of FKS mutations in Candida glabrata: MIC values, echinocandin resistance, and multidrug resistance. Antimicrob. Agents Chemother. 58, 4690–4696. doi: 10.1128/AAC.03255-14
Posteraro, B., and Sanguinetti, M. (2014). The future of fungal susceptibility testing. Future microbiol. 9, 947–967. doi: 10.2217/fmb.14.55
Posteraro, B., Spanu, T., Fiori, B., De Maio, F., De Carolis, E., Giaquinto, A., et al. (2015). Antifungal susceptibility profiles of bloodstream yeast isolates by sensititre yeastone over nine years at a large italian teaching hospital. Antimicrob. Agents Chemother. 59, 3944–3955. doi: 10.1128/AAC.00285-15
Qi, L., Fan, W., Xia, X., Yao, L., Liu, L., Zhao, H., et al. (2018). Nosocomial outbreak of Candida parapsilosis sensu stricto fungaemia in a neonatal intensive care unit in China. J. Hosp. Infect. 100, e246-e252. doi: 10.1016/j.jhin.2018.06.009
Quindós, G. (2018). Epidemiología de las micosis invasoras: un paisaje en continuo cambio Epidemiology of invasive mycoses: a landscape in continuous change. Rev. Iberoam. Micol. 35, 171-178. doi: 10.1016/j.riam.2018.07.002
Siri, L., Legarraga, P., García, P., González, T., and Rabagliati, R. (2017). Cambios clínicos y epidemiológicos de candidemias en pacientes adultos desdea 2000 to 2013. Rev. Chilena Infectol. 34, 19-26. doi: 10.4067/S0716-10182017000100003
Spivak, E. S., and Hanson, K. E. (2018). Candida auris: an emerging fungal pathogen. J. Clin. Microbiol. 56, e1588-17. doi: 10.1128/JCM.01588-17
Taei, M., Chadeganipour, M., and Mohammadi, R. (2019). An alarming rise of non-albicans Candida species and uncommon yeasts in the clinical samples; a combination of various molecular techniques for identification of etiologic agents. BMC. Res. Notes 12:779. doi: 10.1186/s13104-019-4811-1
Tan, T. Y., Hsu, L. Y., Alejandria, M. M., Chaiwarith, R., Chinniah, T., Chayakulkeeree, M., et al. (2016). Antifungal susceptibility of invasive Candida bloodstream isolates from the Asia-Pacific region. Med. Mycol. 54, 471-477. doi: 10.1093/mmy/myv114
Teo, J. Q., Candra, S. R., Lee, S. J., Chia, S. Y., Leck, H., Tan, A. L., et al. (2017). Candidemia in a major regional tertiary referral hospital - epidemiology, practice patterns and outcomes [published correction appears in antimicrob resist infect control. 2018 Nov 7;7:130]. Antimicrob. Resist. Infect. Control. 6, 27–37. doi: 10.1186/s13756-017-0184-1
Thomaz, D. Y., de Almeida, J. N. Jr., Lima, G. M. E., Nunes, M. D. O., Camargo, C. H., Grenfell, R. D. C., et al. (2018). An azole-resistant Candida parapsilosis outbreak, clonal persistence in the intensive care unit of a brazilian teaching hospital. Front. microbiol. 9:2997. doi: 10.3389/fmicb.2018.02997
Wang, H., Zhang, L., Kudinha, T., Kong, F. R., Ma, X. J., Chu, Y. Z., et al. (2016b). Investigation of an unrecognized large-scale outbreak of Candida parapsilosis sensu stricto fungaemia in a tertiary-care hospital in China. Sci. Rep. 6, 27099–27109. doi: 10.1038/srep27099
Wang, H., Xu, Y. C., and Hsueh, P. R. (2016a). Epidemiology of candidemia and antifungal susceptibility in invasive Candida species in the Asia-Pacific region. Future Microbiol. 11, 1461–1477. doi: 10.2217/fmb-2016-0099
Xiao, M., Chen, S. C., Kong, F., Xu, X. L., Yan, L., Kong, H. S., et al. (2020). Distribution and antifungal susceptibility of Candida species causing candidemia in china: an update from the CHIF-NET study. J. Infect. Dis. 221, S139-S147. doi: 10.1093/infdis/jiz573
Xiao, Z., Wang, Q., Zhu, F., and An, Y. (2019). Epidemiology, species distribution, antifungal susceptibility and mortality risk factors of candidemia among critically ill patients: a retrospective study from 2011 to 2017 in a teaching hospital in China. Antimicrob. Resist. Infect. Control. 8:89. doi: 10.1186/s13756-019-0534-2
Xu, H., Yu, S. Y., Zhou, M. L., Ning, Y. T., Xiao, M., Li, X. G., et al. (2019). Epidemiology and antifungal susceptibility patterns of invasive fungal infections from 2012 to 2014 in a teaching hospital in central China. Infect. Drug Resist. 12, 3641-3651. doi: 10.2147/IDR.S227839
Yang, Z. T., Wu, L., Liu, X. Y., Zhou, M., Li, J., Wu, J. Y., et al. (2014). Epidemiology, species distribution and outcome of nosocomial Candida spp. bloodstream infection in Shanghai. BMC Infect. Dis. 14:241. doi: 10.1186/1471-2334-14-241
Zeng, Z. R., Tian, G., Ding, Y. H., Yang, K., Liu, J. B., and Deng, J. (2019). Surveillance study of the prevalence, species distribution, antifungal susceptibility, risk factors and mortality of invasive candidiasis in a tertiary teaching hospital in Southwest China. BMC Infect. Dis. 19:939–939. doi: 10.1186/s12879-019-4588-9
Keywords: yeast isolate, Candida spp., invasive fungal infection, candidemia, antifungal susceptibility, Sensititre YeastOne, triazoles, echinocandins
Citation: Song Y, Chen X, Yan Y, Wan Z, Liu W and Li R (2020) Prevalence and Antifungal Susceptibility of Pathogenic Yeasts in China: A 10-Year Retrospective Study in a Teaching Hospital. Front. Microbiol. 11:1401. doi: 10.3389/fmicb.2020.01401
Received: 22 February 2020; Accepted: 29 May 2020;
Published: 03 July 2020.
Edited by:
David Christopher Coleman, Dublin Dental University Hospital, IrelandReviewed by:
Brenda A. McManus, Dublin Dental University Hospital, IrelandGuillermo Quindós, University of the Basque Country, Spain
Copyright © 2020 Song, Chen, Yan, Wan, Liu and Li. This is an open-access article distributed under the terms of the Creative Commons Attribution License (CC BY). The use, distribution or reproduction in other forums is permitted, provided the original author(s) and the copyright owner(s) are credited and that the original publication in this journal is cited, in accordance with accepted academic practice. No use, distribution or reproduction is permitted which does not comply with these terms.
*Correspondence: Ruoyu Li, bXljb2xhYkAxMjYuY29t