- 1Animal Disease Prevention and Food Safety Key Laboratory of Sichuan Province, Key Laboratory of Bio-Resources and Eco-Environment, Ministry of Education, College of Life Sciences, Sichuan University, Chengdu, China
- 2Sichuan Academy of Grassland Sciences, Chengdu, China
Yaks provide necessities such as meat and milk for Tibetans living at high altitudes on and around the Qinghai-Tibetan Plateau. Enterococci are ubiquitous members of the animal gut microbiota that can cause biofilm-associated opportunistic infections. Meanwhile, multidrug-resistant Enterococcus also poses a serious threat to public health. This study aims to characterize antibiotic resistance, virulence genes, and biofilm formation of enterococci from yaks. From April 2018 to July 2019, we collected 395 fecal samples of yaks in Aba Tibetan Autonomous Prefecture, China. Enterococci isolated from the samples were identified and classified according to the 16S rDNA sequence. The antibiotic resistance of each isolate was detected according to the Kirby-Bauer disk diffusion method, and antibiotic resistance genes were detected by polymerase chain reaction (PCR) and sequencing. Enterococcal biofilms were assessed using standard procedures. Different virulence genes were detected by PCR and sequencing. In total, 381 enterococci strains were recovered, with Enterococcus faecalis (41.99%) and Enterococcus faecium (37.80%) being the predominant species. Many isolates were multidrug- resistant (60.37%) and showed a high resistance rate to rifampicin (64.30%) and tetracycline (61.54%). We also detected various antimicrobial resistance (AMR) genes in the tested strains. The E. faecalis strains had higher frequency of biofilm formation and virulence genes than other enterococcal species. This is the first report that shows yaks are repositories for drug-resistant enterococci with virulent determinants and biofilms that may spread into humans and to environment. This study also provides useful data suggesting that enterococci may pose a potential health risk to yaks. Therefore, active surveillance of AMR and pathogenesis in enterococci from yaks is urgently warranted.
Introduction
The yaks (Bos grunniens) are part of the genus Bos within the family Bovidae. As an iconic symbol of high altitude and of Tibet, more than 14 million yaks reside in high-altitude environments of China, Nepal, India, Kyrgyzstan, Pakistan, Russian Federation and Mongolia, mainly across the Qinghai-Tibet Plateau (above 2500–6000 m) in China (Mi et al., 2013). Yaks are essential animals for Tibetans and other nomadic pastoralists in high-altitude environments owing to their provision of the basic necessities (such as milk, meat, transportation, hides for tented accommodation and dung for fuel) (Qiu et al., 2015). However, frequent outbreaks of bacterial diseases in yaks cause serious economic losses and healthy concern to local people (Bandyopadhyay et al., 2012).
Enterococci are the members of Gram-positive lactic acid-producing bacteria widely distributed in the gut microbiota of humans and other animals (García-Solache and Rice, 2019). Enterococci belong to facultative anaerobes and are highly tolerant to diverse environmental conditions, such as extreme PH, salt concentrations and a wide range of temperature (from 10 to >45°C) (Arias and Murray, 2012). The genus Enterococcus includes Enterococcus faecalis, Enterococcus faecium, Enterococcus hirae, Enterococcus mundtii, and Enterococcus casseliflavus, etc. (Ahmed and Baptiste, 2018). Both E. faecium and E. faecalis are the predominant species that are easily isolated from feces of healthy organisms (Landete et al., 2018). Commensal enterococci are generally not pathogenic in healthy hosts; however, in susceptible hosts, they can cause infections in the wounds, dysbiotic gastrointestinal tract, urinary tract, and endocarditis. More importantly, these infections are associated with biofilms (Ch’ng et al., 2019). Enterococcal biofilms increase their hardiness and contribute to both persistence during infection and contamination of the food industry and the environment (Piggot et al., 2012; Da-Silva-Fernandes et al., 2017). The ability of enterococci to form biofilms also increases their intrinsic tolerance to antibiotics and thus is a serious obstacle for the treatment of infections (Ch’ng et al., 2019).
Global public health is severely threatened by antimicrobial resistance (AMR), the major source of which is composed of the “ESKAPE” pathogens (E. faecium, Staphylococcus aureus, Klebsiella spp., Acinetobacter baumannii, Pseudomonas aeruginosa, and Enterobacter spp.) (Woksepp et al., 2014). Among them, E. faecium is considered as a typical multidrug-resistant (MDR) pathogen owing to both its enormous capacity to develop acquired resistance during antimicrobials chemotherapy and its intrinsic resistance to various antibiotics such as aminoglycosides and β-lactam-based antibiotics (Dale et al., 2015). The World Health Organization (WHO) recently released a list of drug-resistant “priority pathogens” that pose a significant threat to public health and urgently need new therapies (World Health Organization [WHO], 2017). Vancomycin-resistant E. faecium is classified as a Priority 2 pathogen in this list. Other enterococcal species that frequently occur in animals, such as E. durans, E. hirae, and E. casseliflavus, are also appropriate indicators of AMR in Gram-positive bacteria and their appearance can constitute a ‘pool’ of resistant genes, which may be transferred either to other commensal bacteria or even to pathogenic bacteria (Hamed et al., 2018).
As enterococci potentially transfer resistant genes from enteric bacteria in animals to humans via the food chain and they are potential pathogens, they have become a global public health concern. In previous reports, available data showed MDR Enterococcus with a variety of virulent determinants isolated from commensal animals, such as chickens, pigs and cattle (Diarra et al., 2010; Beshiru et al., 2017; De Jong et al., 2019; Igbinosa and Beshiru, 2019). However, there has been no available data on enterococci isolated from yaks. During the sampling process, we consulted local Tibetans and teachers of the Sichuan Academy of Grassland Sciences about the use of antibiotics in yaks. Some Tibetans used tetracycline antibiotics and cephalosporins, many Tibetans did not know the specific drug used to treat yak diseases. The level of antibiotic resistance and virulence determinants in enterococci isolated from yaks can reflect the selection pressure as a result of the use of antimicrobials and the potential pathogenesis, respectively.
Some enterococcal infections are associated with the production of biofilms, which increase enterococci’s intrinsic resistance to antimicrobials. Also, enterococci have the ability to horizontally transfer antibiotic resistance genes in biofilms at high rates, and the transfer is promoted by Epa, Ebp, PrgABC (Bhatty et al., 2015; Dale et al., 2015; La-Rosa et al., 2016). Both antibiotic resistance and biofilms of enterococci can increase the difficulty to treat bacterial diseases in yaks. It is therefore important to monitor AMR, virulence genes, and biofilm formation capacity of enterococci in yaks. In this study, we provided the first comprehensive analysis of antibiotic resistance, virulence genes and biofilm formation of Enterococcus species isolated from yaks.
Materials and Methods
Sample Collection
From April 2018 to July 2019, 395 non-repeated fecal samples were collected from healthy yaks from 37 different farms in the Aba TAP Tibetan Autonomous Prefecture (Sichuan), Sichuan Province, China. During the sampling process, we used sterile swabs to collect fresh feces, and then placed them into 10 mL aseptic tubes. We placed the collected samples with ice packs and later shipped them to the laboratory within 24 h of collection for isolating bacteria.
Isolation and Identification of Enterococcus Species
At the stage of pre-enrichment, we placed the samples into 10 mL aseptic tubes containing 4 mL BHI (Brain Heart Infusion) broth, before incubating them at 37°C for 18–24 h with a rotation speed of 200 rpm. The enriched cultures were inoculated to Bile Esculin Azide agar (Enterococcus selective agar). One colony was selected for each sample. Subsequently, we used the BD Phoenix-100 Automated Microbiology System (BD Dignostic System, Sparks, MD, United States) to identify all the isolated strains. The confirmed isolates were stored in 25% glycerol containing BHI broth at −80°C. According to the instructions of the TIANamp Column Bacteria Genomic DNA Purification Kit (Beijing Tiangen Biotech, Beijing, China), total bacterial DNA was extracted from all the Enterococcus strains for PCR templates. The genomic DNA solution was stored at −20°C.
To identify the enterococcal species, all the isolates were screened for the presence of 16S rDNA gene by PCR. The PCR products were then directly sent to a sequencing company (Chengdu Sangon Biological Engineering Technology & Services, Co., Ltd.) and DNA sequences were analyzed online using BLAST1.
Antimicrobial Sensitivity Test
The antibiotic sensitivity profile of all enterococci was determined according to Clinical and Laboratory Standards Institute [CLSI] (2017) guidelines. The following antimicrobials (all purchased from Oxoid, Thermo Fisher Scientific, Basingstoke, United Kingdom) were used: ampicillin (AMP, 10 μg), penicillin (PEN, 10 units), erythromycin (ERY, 15 μg), tetracycline (TET, 30 μg), rifampicin (RIF, 5 μg), ciprofloxacin (CIP, 5 μg), chloramphenicol (CHL, 30 μg), vancomycin (VAN, 30 μg), linezolid (LZD, 30 μg), fosfomycin (FOS, 50 μg) and nitrofurantoin (NIT, 300 μg). E. faecalis ATCC 29212 was used as a quality control strain.
Detection of Antimicrobial Resistance Genes
The emergence of drug-resistant genes associated with tetracycline (tetA, tetB, tetM, tetL), erythromycin (ermA, ermB), ciprofloxacin (qnrA, qnrB, qnrS, qepA), linezolid (optrA, poxtA), chloramphenicol (cat) and vancomycin (vanA, vanB) was determined by PCR using specific primers (Supplementary Table S1). Positive PCR products were sequenced by Chengdu Sangon Biological Engineering Technology & Services, Co., Ltd.
Biofilm Formation
Biofilm assays were performed according to the guidance of a method (Stepanović et al., 2007). First, the purified Enterococcus colonies were resuspended in 10 mL of Tryptic Soy Broth (TSB) supplemented with 1% glucose, incubated at 37°C for 18–20 h. Then, for each strain tested, 20 μl of bacterial suspensions were transferred to three wells of sterile 96-well polystyrene microtiter plates containing 180 μl of TSB supplemented with 1% glucose. E. faecalis (ATCC 29212) was used as the positive control, and 200 μl of broths (TSB with 1% glucose) were used as the negative control. The microtiter plates were incubated for 24 h at 37°C, washed with sterile phosphate-buffered solution (PBS), dried at 28 ± 2°C, and stained with crystal violet for 30 min. The wells were washed twice with sterile deionized water and dried. Crystal violet dye bound to adherent cells was resuspended in 150 mL of 99% ethanol. The OD readings from respective wells were determined at 570 nm. Each assay was determined three times. The formation of biofilm was classified as negative, weak, moderate, or strong. The cut-off value (ODc) was defined as the mean OD value above three standard deviations (SD) of the negative control: ODc = average OD of negative control + (3 × SD of negative control). Each Enterococcus isolate was classified as follows: OD < ODc = non-biofilm producers (category 0); ODc < OD < 2ODc = weak biofilm producers (category 1); 2ODc < OD < 4ODc = moderate biofilm producers (category 2); and OD > 4ODc = strong biofilm producers (category 3).
Screening for Virulence Associated Genes
Specific primers (Supplementary Table S2) were used for PCR testing of all Enterococcus isolates to detect virulence genes (selected according to their functional characteristics, including secreted factors and cell surface determination cluster) encoding cylA, gelE, agg, ace, ebpA, ebpB, ebpC, efaA, hyl, and srtA. Appropriate virulence genes were used as positive controls and sterile water as negative controls in all tests.
Statistical Analysis
All statistical analyses were performed using GraphPad Prism 8. Fisher’s exact test for samples were used. P < 0.05 was considered statistically significant.
Results
Sample Collection and the Species of Enterococci
In this study, we collected 395 non-duplicated fresh samples in 37 farms. Out of the samples, a collection of 381 enterococci were identified. Hence, the isolation rate of Enterococcus isolates was 96.46% (381/395) in the current investigation. The number of isolated strains of all enterococcal species is shown in Table 1, and the most frequent Enterococcus spp. was E. faecalis (n = 160), followed by E. faecium (n = 144).
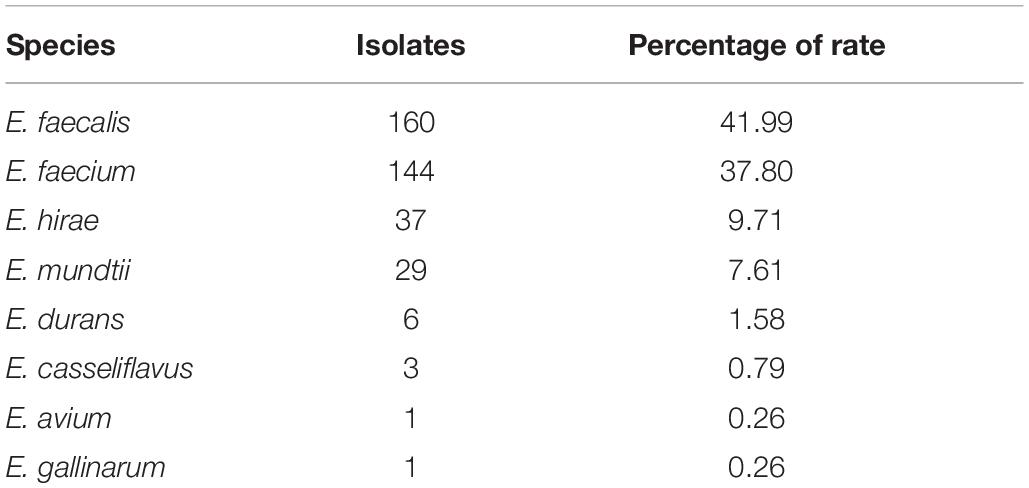
Table 1. Numbers of isolates of enterococcal species from yaks in Aba TAP (from April 2018 to July 2019).
Due to the different scales of the farms, the number of enterococci isolated in each farm varies. On most farms, E. faecalis and E. faecium are the main isolates. However, we isolated 8 E. mundtii strains (accounting for 50%) on farm 3. Moreover, six different kinds of Enterococcus species were detected on farm 5. The details of the isolation of enterococci on each farm are shown in Supplementary Table S3.
Antimicrobial Sensitivity Test
Table 2 shows antibiotic non-susceptible (intermediate and resistant) Enterococcus species isolated from yaks, which exhibit high rates of resistance to RIF (64.30%) and TET (61.54%), with rates of resistance to ERY (35.96%), CIP (35.96%), CHL (26.25%), NIT (24.93%), PEN (17.32%), and AMP (15.22%). However, 10.76, 3.94, and 2.10% of strains were resistant to LZD, VAN, and FOS, respectively. Of the isolates, 60.37% (n = 230) were multi-drug resistant (MDR, resistant to at least three different classes of antibiotics). The various resistance patterns were observed, of which TET-ERY-RIF was more common.
We further analyzed the relationship between resistance phenotypes and enterococcal species. We found that E. faecium was the most antibiotic resistant species, with a multi-drug resistance rate of 75.69%, followed by E. faecalis, whose multi-drug resistance rate was 68.13%. Whereas most of E. mundtii isolates were sensitive to antibiotics, only two strains were MDR. Our data showed that E. faecium was more resistant to AMP, PEN, and NIT than E. faecalis (P < 0.0001; Fisher’s exact test). Moreover, we found that erythromycin and tetracycline had a combined resistance trend, with 137 erythromycin-resistant isolates and 133 Enterococcus strains resistant to tetracycline together.
Detection of Antimicrobial Resistance Genes
Table 3 shows the detection of 15 AMR genes. The highest rate of resistance gene was tetM gene, which accounted for 54.07% of the strains. Other tetracycline resistances tetA, tetB, and tetL were detected in 6.56, 2.62, and 45.14% of the strains, respectively. The erythromycin resistance gene ermB was found in 30.45% of the isolates, and ermA gene was only presented in 15.75%. The cat gene responsible for chloramphenicol resistance, was detected in 25.98% of the strains. Moreover, the oxazolidinone resistance genes (optrA, poxtA) were found in 3.94 and 0.26% of the isolates, respectively. We didn’t detect ciprofloxacin resistance genes (qnrA, qnrB, qnrS, and qepA) in ciprofloxacin resistant strains. The vancomycin resistance genes vanA and vanB were also not detected in this study.
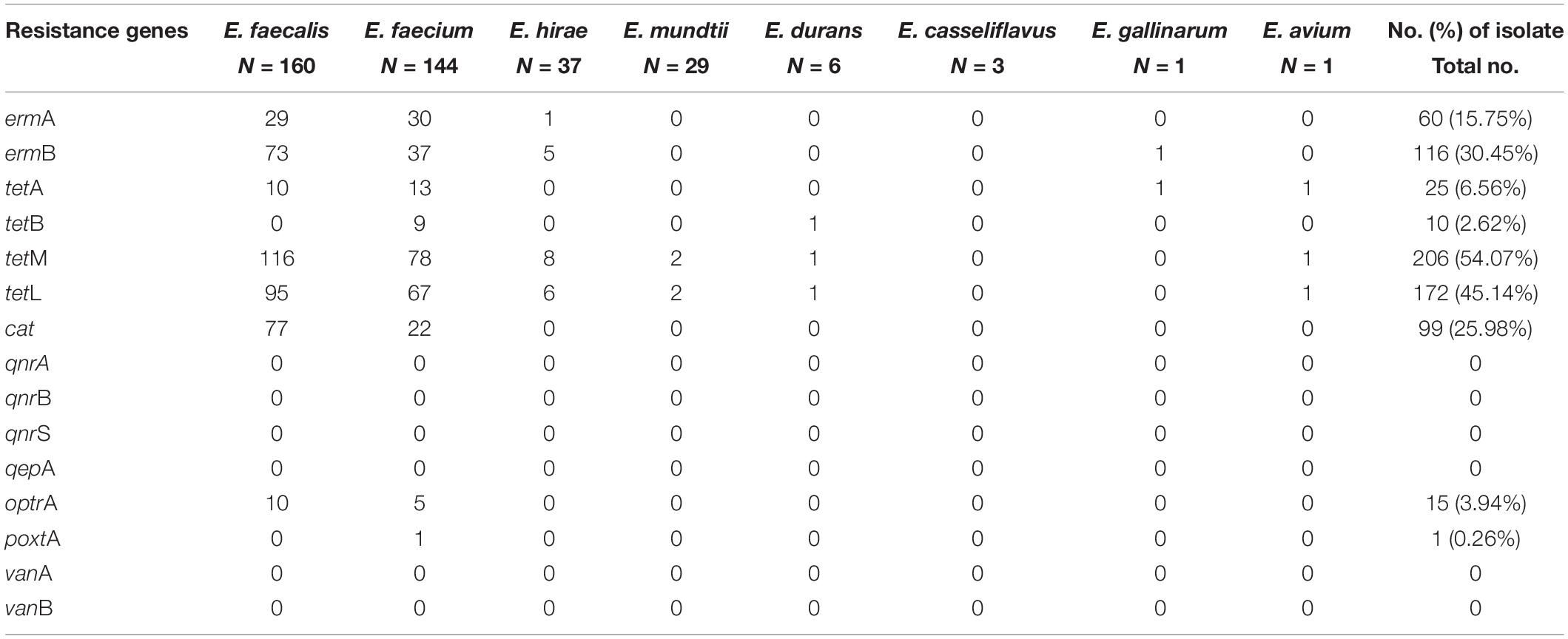
Table 3. Prevalence and distribution antimicrobial resistance genes in Enterococcus isolates recovered from yaks.
The phenotypic and genotypic patterns of the AMR detected in the isolates are shown in Supplementary Material S4.
Analyze the Capacity of Biofilm Formation
Biofilm formation of the Enterococci includes the following: non-formers, 124 (32.55%); weak formers, 112 (29.40%); moderate formers, 113 (29.66%); and strong formers, 32 (8.40%). Overall, 257 (67.45%) were biofilm formers. Biofilm formation of the tested E. faecalis was statistically significantly higher than that of E. mundtii strains (P = 0.0089; Fisher’s exact test). Table 4 summarizes the biofilm-forming strength in Enterococcus species isolated from yaks.

Table 4. Association between biofilm-forming strength in TSB Broth with 1% glucose and Enterococcus species (no. of strains/%).
Prevalence of Virulence Genes in Enterococci
Table 5 shows the prevalence of virulence genes detected in all isolates, as follows: cylA, 73 (19.16%); gelE, 160 (41.99%); agg, 162 (42.52%); ace, 246 (64.57%); ebpA, 150 (39.37%); ebpB, 61 (16.01%); ebpC, 175 (45.93%); esp, 122 (32.02%); efaA, 300 (78.74%); hyl, 143 (37.53%); and srtA, 202 (53.02%). Only three E. faecalis isolates were simultaneously positive to all tested virulence genes, while 24 E. mundtii strains were negative to all tested virulence genes. These results indicate that E. faecalis had the potential for higher virulence and E. mundtii the potential for lower virulence among enterococcal species.
We then analyzed the relationship between virulence genes and biofilm formation, which shows there was a correlation between biofilm formation and the presence of the ace gene in E. faecium (P < 0.0001; Fisher’s exact test). The presence of gelE and cylA genes were mainly found in E. faecalis (P < 0.0001; Fisher’s exact test), and these genes did not have a statistically correlation for the formation of biofilms in E. faecalis (PgelE = 0.7348, PcylA = 0.6894; Fisher’s exact test).
The genotypic patterns of the virulence factors and biofilms detected in the isolates are shown in Supplementary Material S5.
Discussion
Compared with findings about enterococci of other commensal animals, available data on enterococci isolated from yaks is still inadequate. The yak is vital to the production system of the Tibetans and other nomadic herders in high-altitude regions (Xiao-Yun et al., 2006). Here, we provide the comprehensive analysis of the antimicrobial susceptibility, biofilm formation, and virulence genes of several enterococcal species isolated from yaks. We observed a high proportion of multidrug-resistant enterococci with virulence factors in yaks.
In current study, 381 Enterococci were isolated. Unlike previous studies, the isolation rate of E. faecalis was slightly higher than that of E. faecium (De Jong et al., 2018, 2019; Lei et al., 2019). Moreover, the isolation rate of E. mundtii was 7.61% (n = 29), which contradicts the findings from previous studies that show a very low isolation rate of E. mundtii in other commensal animals (Kim et al., 2016; De Jong et al., 2018; Osman et al., 2019). These results indicate that Enterococcus species composition varies in different host environments.
Enterococcal resistance and tolerance to antimicrobials is a serious threat to global health that needs to be addressed as a priority. Enterococci are intrinsically resistant to various antibiotics (for example, aminoglycosides, clindamycin, and β-lactam-based antibiotics). Due to the low permeability of enterococcal cell wall to large aminoglycoside molecules, enterococci are moderately resistant to aminoglycosides and are more prevalence in E. faecium than E. faecalis (Aslangul et al., 2006; Abat et al., 2016). Enterococci have the ability to overexpress penicillin-binding proteins with low affinity for β-lactams, which allows enterococci intrinsically resistant to penicillin (Murray, 1997; Duez et al., 2001). Compared with E. faecalis, E. faecium is more intrinsically resistant to antimicrobials. However, E. faecalis can show tolerance to antibiotics by forming a thicker biofilm. Biofilms of E. faecalis isolated from clinic show increased tolerance to vancomycin and tigecycline (Hashem et al., 2017). In the present study, we found that 15 E. faecalis strains were resistant to vancomycin. However, vancomycin-resistant genes were not detected in these strains. It may be due to the formation of biofilms that enhance tolerance to vancomycin.
Enterococci can readily acquire resistance to antimicrobials during antibiotics chemotherapies. In this study, 60.37% of the Enterococcus isolates were MDR. We observed that erythromycin and tetracycline had a combined resistance trend, which was in line with the findings of previous study (Kim et al., 2019). High antibiotic resistance in the study area may be a marker for differences in multiple factors such as antimicrobials use, disease control measures, or genetic mutations leading to multidrug-resistant phenotypes (Jadhav et al., 2011). Our study reveal that local Tibetan people’s inappropriate use of antibiotics caused MDR enterococci to appear in yaks. Furthermore, yak is a free-grazing animal that can move around in a large geographic area, and thus yak feces containing MDR bacteria can also cause pollution to the local ecological environment. Therefore, robust monitoring programs are needed to control the spread of AMR in yaks.
The association among enterococcal species and resistance phenotypes varied in this study. Overall, E. faecium strains had a higher rate of MDR, whereas most of E. mundtii isolates were sensitive to antibiotics. Similarly, the higher prevalence of MDR E. faecium among Enterococcus spp. isolated from other animals has been documented previously (Novais et al., 2013; De Jong et al., 2018). Moreover, enterococcal species also had significant differences in antibiotic resistance among various antibiotics, for we observed that E. faecium was more resistant to penicillin and ampicillin than E. faecalis. This was in line with the findings of other study (Novais et al., 2013). Since enterococci that produce β-lactamase are extremely rare, the acquired resistance of E. faecium to ampicillin is mainly due to the penicillin-binding protein5 (pbp5) mutation, which has a lower affinity for β-lactam antibiotics, while the fact that E. faecalis has acquired resistance to ampicillin is mainly due to pbp4 mutation (Rice et al., 2004; Ono et al., 2005). Therefore, our results indicate that pbp5 of E. faecium may be more susceptible to mutations than pbp4 of E. faecalis under antibiotic stress.
According to the results of the antibiotic resistance phenotype, various resistance genes were detected in Enterococcus strains. Among the tetracycline resistance genes, tetM and tetL were significantly present in tetracycline-resistant enterococci. Similarly, ermB was more than ermA present in erythromycin-resistant enterococci. These results were in line with the previous studies (Schwaiger et al., 2009; Kim et al., 2019). We also detected oxazolidinone resistance genes optrA and poxtA. Oxazolidinones (tedizolid and linezolid) are effective antibiotics for the treatment of multidrug resistant Gram-positive bacterial (including vancomycin-resistant Enterococcus) infections, and these resistant genes can usually be linked with mobile genetic elements for horizontal transmission (Hao et al., 2019; Lei et al., 2019), so we should standardize the use of oxazolidinone and strengthen the detection of oxazolidinone-resistant enterococci to prevent the spread of resistant genes to the environment and humans. Enterococci resistance to ciprofloxacin is usually due to the mutations in the gyrA and parC quinolone resistance-determining region (Onodera et al., 2002; Leavis et al., 2016), so we did not detect plasmid-mediated quinolone resistance genes in isolated strains.
Enterococcal biofilms have been observed in many infections. Moreover, Biofilm is an important source of pollution in the food processing industry and enterococci isolated from food have the ability to form biofilms (Ch’ng et al., 2019; Igbinosa and Beshiru, 2019). Therefore, we determined biofilm formation capacity among Enterococcus species from yaks and its correlation with virulence genes. We found that many of the E. faecalis isolates showed higher-level biofilm formation and virulence genes, whereas most of the tested E. mundtii strains were negative to biofilm and all tested of virulence genes. Our findings corroborate the results reported that E. faecalis was the first-most frequent Enterococcus species associated with disease (Gawryszewska et al., 2017), while E. mundtii was low in virulence and infrequently associated to infections (Repizo et al., 2014).
We found that ace had a correlation with biofilm formation in E. faecium. Compared with many factors involved in E. faecalis biofilm formation, several E. faecium genes are involved in the development of biofilm, including esp, ebpABC, and ace (Sava et al., 2010; Lim et al., 2017). However, we observed the low prevalence of ebpA, ebpB, ebpC, and esp genes in E. faecium. Hemolysin–cytolysin (Cyl) and the proteases gelatinase (GelE) are important secreted factors and play a key role in enterococcal pathogenesis. These genes (cylA and gelE) were more often found in E. faecalis strains. Similar findings were also reported that E. faecalis more often carried cylA and gelE genes among enterococcal species (Golob et al., 2019; Stępień-Pyśniak et al., 2019).
Most biofilm-associated infections are caused by a combination of multiple microorganisms, and usually two or more of species can be found at the site of infection. Enterococci can account for a large proportion of the population in wound infections identified by next generation sequencing technology (Holá et al., 2010; Dworniczek et al., 2012). Isolation of E. faecalis is often accompanied by other bacteria. For example, Proteus mirabilis was recovered from nearly 40% of E. faecalis biofilms (Macleod and Stickler, 2007). It was also found on catheters together with Klebsiella pneumoniae and Escherichia coli (Galvan et al., 2016). Meanwhile, enterococci can act as gene pool to spread antibiotic resistance within and between species. This may lead to the aggravation of clinical symptoms and increase the difficulty of diagnosis and control of yak diseases. For the local Tibetans, yak milk and meat are not only the main protein food, but also an important economic source by exporting for high-end consumption (Yue et al., 2013), and thus AMR genes of enterococci in yaks can transfer to humans via the food chain. Therefore, it is altogether fitting and proper that we analyze the antibiotic resistance and virulence of enterococci isolated from yaks.
Conclusion
The results of this study reveal that 60.37% isolated strains were MDR and 9 antibiotic-resistant genes were detected. Likewise, great frequency of biofilm formation and virulence genes were observed among the isolated enterococci. The emergence of AMR genes and virulence genes in enterococci from yaks is a serious concern because they could be transmitted to humans through the food chain and the spread of these genes could significantly limit the treatment options for MDR bacteria. Finally, the study demonstrate that yaks are reservoirs of antimicrobial resistant enterococci with potential virulence. Therefore, we should initiate robust surveillance programs to control and monitor the use of antibiotics.
Data Availability Statement
All datasets generated for this study are included in the article/Supplementary Material.
Ethics Statement
The animal study was reviewed and approved by the College of Life Science, Sichuan University affiliation ethics committee, and all efforts were made to minimize animal suffering.
Author Contributions
PC designed the study and wrote the manuscript. LF, XF, TA, XZ, HuL, and XL collected the samples. PC, JH, LZ, CLi, YZ, HaL, and WY performed the experiments. HW, CLe, and XY supervised the research.
Funding
This research was supported by the Project for Science and Technology Support Program of Sichuan Province (2016KJT0057).
Conflict of Interest
The authors declare that the research was conducted in the absence of any commercial or financial relationships that could be construed as a potential conflict of interest.
Acknowledgments
We would like to sincerely thank XZ, HuL and the local Tibetans for collecting samples.
Supplementary Material
The Supplementary Material for this article can be found online at: https://www.frontiersin.org/articles/10.3389/fmicb.2020.01250/full#supplementary-material
Footnotes
References
Abat, C., Raoult, D., and Rolain, J. M. (2016). Low level of resistance in Enterococci isolated in four hospitals, Marseille, France. Microb. Drug. Resist. 22, 218–222. doi: 10.1089/mdr.2015.0121
Ahmed, M. O., and Baptiste, K. E. (2018). Vancomycin-resistant enterococci: a review of antimicrobial resistance mechanisms and perspectives of human and animal health. Microb. Drug. Resist. 24, 590–606. doi: 10.1089/mdr.2017.0147
Arias, C. A., and Murray, B. E. (2012). The rise of the Enterococcus: beyond vancomycin resistance. Nat. Rev. Microbiol. 10, 266–278. doi: 10.1038/nrmicro2761
Aslangul, E., Massias, L., Meulemans, A., Chau, F., Andremont, A., Courvalin, P., et al. (2006). Acquired gentamicin resistance by permeability impairment in Enterococcus faecalis. Antimicrob. Agents. Chemother. 50, 3615–3621. doi: 10.1128/AAC.00390-06
Bandyopadhyay, S., Lodh, C., Sarkar, M., Ghosh, M. K., Bera, A. K., Bhattacharyya, D., et al. (2012). Prevalence molecular fingerprinting and drug resistance profile of enterovirulent Escherichia coli isolates from free ranging yaks of Tawang district, Arunachal Pradesh, India. Trop. Anim. Health. Prod. 44, 1063–1072. doi: 10.1007/s11250-011-0041-9
Beshiru, A., Igbinosa, I. H., Omeje, F. I., Ogofure, A. G., Eyong, M. M., and Igbinosa, E. O. (2017). Multi-antibiotic resistant and putative virulence gene signatures in Enterococcus species isolated from pig farms environment. Microb. Pathog. 104, 90–96. doi: 10.1016/j.micpath.2017.01.020
Bhatty, M., Cruz, M. R., Frank, K. L., Gomez, J. A., Andrade, F., Garsin, D. A., et al. (2015). Enterococcus faecalis pCF10-encoded surface proteins PrgA, PrgB (aggregation substance) and PrgC contribute to plasmid transfer, biofilm formation and virulence. Mol. Microbiol. 95, 660–677. doi: 10.1111/mmi.12893
Ch’ng, J. H., Chong, K. K. L., Lam, L. N., Wong, J. J., and Kline, K. A. (2019). Biofilm-associated infection by enterococci. Nat. Rev. Microbiol. 17, 82–94. doi: 10.1038/s41579-018-0107-z
Choi, J. M., and Woo, G. J. (2015). Transfer of tetracycline resistance genes with aggregation substance in food-borne Enterococcus faecalis. Curr. Microbiol. 70, 476–484. doi: 10.1007/s00284-014-0742-1
Clinical and Laboratory Standards Institute [CLSI] (2017). Performance Standards for Antimicrobial Susceptibility Testing M02-A12, M07-A10, and M11-A8, 27th Edn, Philadelphia, PA: Clinical and Laboratory Standards Institute.
Dale, J. L., Cagnazzo, J., Phan, C. Q., Barnes, A. M., and Dunny, G. M. (2015). Multiple roles for Enterococcus faecalis glycosyltransferases in biofilm-associated antibiotic resistance, cell envelope integrity, and conjugative transfer. Antimicrob. Agents. Chemother. 59, 4094–4105. doi: 10.1128/AAC.00344-15
Da-Silva-Fernandes, M., Coelho-Alvares, A. C., Martins-Manoel, J. G., Ramires-Esper, L. M., Kabuki, D. Y., and Kuaye, A. Y. (2017). Formation of multi-species biofilms by Enterococcus faecium, Enterococcus faecalis, and Bacillus cereus isolated from ricotta processing and effectiveness of chemical sanitation procedures. Intern. Dairy J. 72, 23–28. doi: 10.1016/j.idairyj.2017.03.016
De Jong, A., Simjee, S., Garch, F. E., Moyaert, H., Rose, M., Youala, M., et al. (2018). Antimicrobial susceptibility of enterococci recovered from healthy cattle, pigs and chickens in nine EU countries (EASSA Study) to critically important antibiotics. Vet. Microbiol. 216, 168–175. doi: 10.1016/j.vetmic.2018.02.010
De Jong, A., Simjee, S., Rose, M., Moyaert, H., El, Garch, F., et al. (2019). Antimicrobial resistance monitoring in commensal enterococci from healthy cattle, pigs and chickens across Europe during 2004–14 (EASSA Study). J. Antimicrob. Chemother. 74, 921–930. doi: 10.1093/jac/dky537
Diarra, M. S., Rempel, H., Champagne, J., Masson, L., Pritchard, J., and Topp, E. (2010). Distribution of antimicrobial resistance and virulence genes in Enterococcus spp. and characterization of isolates from broiler chickens. Appl. Environ. Microbiol. 76, 8033–8043. doi: 10.1128/AEM.01545-10
Duez, C., Zorzi, W., Sapunaric, F., Amoroso, A., Thamm, I., and Coyette, J. (2001). The penicillin resistance of Enterococcus faecalis JH2-2r results from an overproduction of the low- affinity penicillin-binding protein PBP4 and does not involve a psr -like gene. Microbiology 147, 2561–2569. doi: 10.1099/00221287-147-9-2561
Duprè, I., Zanetti, S., Schito, A. M., Fadda, G., and Sechi, L. A. (2003). Incidence of virulence determinants in clinical Enterococcus faecium and Enterococcus faecalis isolates collected in Sardinia (Italy). J. Med. Microbiol. 52, 491–498. doi: 10.1099/jmm.0.05038-0
Dworniczek, E., Piwowarczyk, J., Bania, J., Kowalska-Krochmal, B., Wałecka, E., Seniuk, A., et al. (2012). Enterococcus in wound infections: virulence and antimicrobial resistance. Acta Microbiol. Immunol. Hung. 59, 263–269. doi: 10.1556/AMicr.59.2012.2.11
Eaton, T. J., and Gasson, M. J. (2001). Molecular screening of Enterococcus virulence determinants and potential for genetic exchange between food and medical isolates. Appl. Environ. Microbiol. 67, 1628–1635. doi: 10.1128/AEM.67.4.1628-1635.2001
Galvan, E. M., Mateyca, C., and Ielpi, L. (2016). Role of interspecies interactions in dual-species biofilms developed in vitro by uropathogens isolated from polymicrobial urinary catheter-associated bacteriuria. Biofouling 32, 1067–1077. doi: 10.1080/08927014.2016.1231300
García-Solache, M., and Rice, L. B. (2019). The Enterococcus: a model of adaptability to its environment. Clin. Microbiol. Rev. 32:e058-18. doi: 10.1128/CMR.00058-18
Gawryszewska, I., Malinowska, K., Kuch, A., Chrobak-Chmiel, D., Trokenheim, L. L., Hryniewicz, W., et al. (2017). Distribution of antimicrobial resistance determinants, virulence-associated factors and clustered regularly interspaced palindromic repeats loci in isolates of Enterococcus faecalis from various settings and genetic lineages. Pathog. Dis. 75:ftx021. doi: 10.1093/femspd/ftx021
Golob, M., Pate, M., Kušar, D., Dermota, U., Avberšek, J., Papić, B., et al. (2019). Antimicrobial resistance and virulence genes in Enterococcus faecium and Enterococcus faecalis from humans and retail red meat. Biomed. Res. Int. 2019:2815279. doi: 10.1155/2019/2815279
Hamed, S. M., Elkhatib, W. F., El-Mahallawy, H. A., Helmy, M. M., Ashour, M. S., and Aboshanab, K. M. A. (2018). Multiple mechanisms contributing to ciprofloxacin resistance among Gram negative bacteria causing infections to cancer patients. Sci. Rep. 8:12268. doi: 10.1038/s41598-018-30756-4
Hao, W., Shan, X., Li, D., Schwarz, S., Zhang, S. M., Li, X. S., et al. (2019). Analysis of a poxt A- and optr A-co-carrying conjugative multiresistance plasmid from Enterococcus faecalis. J. Antimicrob. Chemother. 74, 1771–1775. doi: 10.1093/jac/dkz109
Hashem, Y. A., Amin, H. M., Essam, T. M., Yassin, A. S., and Aziz, R. K. (2017). Biofilm formation in enterococci: genotype- phenotype correlations and inhibition by vancomycin. Sci. Rep. 7:5733. doi: 10.1038/s41598-017-05901-0
Holá, V., Ruzicka, F., and Horka, M. (2010). Microbial diversity in biofilm infections of the urinary tract with the use of sonication techniques. FEMS Immunol. Med. Microbiol. 59, 525–528. doi: 10.1111/j.1574-695X.2010.00703.x
Igbinosa, E. O., and Beshiru, A. (2019). Antimicrobial resistance, virulence determinants, and biofilm formation of Enterococcus species from ready-to-eat seafood. Front. Microbiol. 10:728. doi: 10.3389/fmicb.2019.00728
Jadhav, S., Hussain, A., Devi, S., Kumar, A., Parveen, S., Gandham, N., et al. (2011). Virulence characteristics and genetic affinities of multiple drug resistant uropathogenic Escherichia coli from a semi urban locality in India. PLoS One 6:e18063. doi: 10.1371/journal.pone.0018063
Kim, D. H., Chung, Y. S., Park, Y. K., Yang, S. J., Lim, S. K., Park, Y. H., et al. (2016). Antimicrobial resistance and virulence profiles of Enterococcus spp. isolated from horses in korea. Comp. Immunol. Microbiol. Infect. Dis. 48, 6–13. doi: 10.1016/j.cimid.2016.07.001
Kim, J., Choi, O., and Kim, T. S. (2012). An outbreak of onion center rot caused by Pantoea ananatis in Korea. Plant Dis. 96:1576. doi: 10.1094/PDIS-03-12-0251-PDN
Kim, Y. B., Seo, K. W., Jeon, H. Y., Lim, S. K., Sung, H. W., and Lee, Y. J. (2019). Molecular characterization of erythromycin and tetracycline-resistant Enterococcus faecalis isolated from retail chicken meats. Poult. Sci. 98, 977–983. doi: 10.3382/ps/pey477
Landete, J. M., Peiroten, A., Medina, M., Arques, J. L., and Rodriguez-Minguez, E. (2018). Virulence and antibiotic resistance of Enterococci isolated from healthy breastfed infants. Microb. Drug. Resist. 24, 63–69. doi: 10.1089/mdr.2016.0320
La-Rosa, S. L., Montealegre, M. C., Singh, K. V., and Murray, B. E. (2016). Enterococcus faecalis Ebp pili are important for cell-cell aggregation and intraspecies gene transfer. Microbiology 162, 798–802. doi: 10.1099/mic.0.000276
Leavis, H. L., Willems, R. J., Top, J., and Bonten, M. J. (2016). High-level ciprofloxacin resistance from point mutations in gyr A and par C confined to global hospital-adapted clonal lineage CC17 of Enterococcus faecium. J. Clin. Microbiol. 44, 1059–1064. doi: 10.1128/JCM.44.3.1059-1064
Lei, C. W., Kang, Z. Z., Wu, S. K., Chen, Y. P., Kong, L. H., and Wang, H. N. (2019). Detection of the phenicol–oxazolidinone–tetracycline resistance gene poxt A in Enterococcus faecium and Enterococcus faecalis of food-producing animal origin in China. J. Antimicrob. Chemother. 74, 2459–2461. doi: 10.1093/jac/dkz198
Lim, S. Y., Teh, C. S. J., and Thong, K. L. (2017). Biofilm-related diseases and omics: global transcriptional profiling of Enterococcus faecium reveals different gene expression patterns in the biofilm and planktonic cells. OMICS 21, 592–602. doi: 10.1089/omi.2017.0119
Lopes-Mde, F., Simões, A. P., Tenreiro, R., Marques, J. J., and Crespo, M. T. (2006). Activity and expression of a virulence factor, gelatinase, in dairy enterococci. Int. J. Food. Microbiol. 112, 208–214. doi: 10.1016/j.ijfoodmicro.2006.09.004
Macleod, S. M., and Stickler, D. J. (2007). Species interactions in mixed-community crystalline biofilms on urinary catheters. J. Med. Microbiol. 56, 1549–1557. doi: 10.1099/jmm.0.47395-0
Mi, R., Wang, X., Li, C., Huang, Y., Zhou, P., Li, Z., et al. (2013). Prevalence and genetic characterization of Cryptosporidium in yaks in Qinghai province of China. PLoS One 8:e74985. doi: 10.1371/journal.pone.0074985
Murray, B. E. (1997). Vancomycin-resistant enterococci. Am. J. Med. 102, 284–293. doi: 10.1016/S0002-9343(99)80270-8
Nam, S., Kim, M. J., Park, C., Park, J. G., and Lee, G. C. (2012). Detection and genotyping of vancomycin-resistant Enterococcus spp. by multiplex polymerase chain reaction in Korean aquatic environmental samples. Int. J. Hyg. Environ. Health 216, 421–427. doi: 10.1016/j.ijheh.2012.12.004
Novais, C., Freitas, A. R., Silveira, E., Antunes, P., Silva, R., Coque, T. M., et al. (2013). Spread of multidrug-resistant Enterococcus to animals and humans: an underestimated role for the pig farm environment. J. Antimicrob. Chemother. 68, 2746–2754. doi: 10.1093/jac/dkt289
Ono, S., Muratani, T., and Matsumoto, T. (2005). Mechanisms of resistance to imipenem and ampicillin in Enterococcus faecalis. Antimicrob. Agents. Chemother. 49, 2954–2958. doi: 10.1128/AAC.49.7.2954-2958.2005
Onodera, Y., Okuda, J., Tanaka, M., and Sato, K. (2002). Inhibitory activities of quinolones against DNA gyrase and topoisomerase IV of Enterococcus faecalis. Antimicrob. Agents. Chemother. 46, 1800–1804. doi: 10.1128/aac.46.6.1800-1804.2002
Osman, K. M., Badr, J., Orabi, A., Elbehiry, A., Saad, A., Ibrahim, M. D. S., et al. (2019). Poultry as a vector for emerging multidrug resistant Enterococcus spp. : first report of vancomycin (van) and the chloramphenicol–florfenicol (cat-fex-cfr) resistance genes from pigeon and duck faeces. Microb. Pathog. 128, 195–205. doi: 10.1016/j.micpath.2019.01.006
Piggot, A. M., Klaus, J. S., Johnson, S., Phillips, M. C., and Solo-Gabriele, H. M. (2012). Relationship between enterococcal levels and sediment biofilms at recreational beaches in South Florida. Appl. Environ. Microbiol. 78, 5973–5982. doi: 10.1128/AEM.00603-12
Qiu, Q., Wang, L., Wang, K., Yang, Y., Ma, T., Wang, Z., et al. (2015). Yak whole-genome resequencing reveals domestication signatures and prehistoric population expansions. Nat. Commun. 22:10283. doi: 10.1038/ncomms10283
Repizo, G. D., Espariz, M., Blancato, V. S., Suárez, C. A., Esteban, L., and Magni, C. (2014). Genomic comparative analysis of the environmental Enterococcus mundtii against enterococcal representative species. BMC Genomics 15:489. doi: 10.1186/1471-2164-15-489
Rice, L. B., Bellais, S., Carias, L. L., Hutton-Thomas, R., Bonomo, R. A., Caspers, P., et al. (2004). Impact of specific pbp 5 mutations on expression of beta-lactam resistance in Enterococcus faecium. Antimicrob. Agents. Chemother. 48, 3028–3032. doi: 10.1128/AAC.48.8.3028-3032.2004
Sava, I. G., Heikens, E., and Huebner, J. (2010). Pathogenesis and immunity in enterococcal infections. Clin. Microbiol. Infect. 16, 533–540. doi: 10.1111/j.1469-0691.2010.03213.x
Schwaiger, K., Harms, K., Hölzel, C., Meyer, K., Karl, M., and Bauer, J. (2009). Tetracycline in liquid manure selects for co-occurrence of the resistance genes tet (M) and tet (L) in Enterococcus faecalis. Vet. Microbiol. 139, 386–392. doi: 10.1016/j.vetmic.2009.06.005
Seputiene, V., Bogdaite, A., Ruzauskas, M., and Suziedeliene, E. (2012). Antibiotic resistance genes and virulence factors in Enterococcus faecium and Enterococcus faecalis from diseased farm animals: pigs, cattle and poultry. Pol. J. Vet. Sci. 15, 431–438. doi: 10.2478/v10181-012-0067-6
Stepanović, S., Vuković, D., Hola, V., Di-Bonaventura, G., Djukić, S., Cirković, I., et al. (2007). Quantification of biofilm in microtiter plates: overview of testing conditions and practical recommendations for assessment of biofilm production by staphylococci. APMIS 15, 891–899. doi: 10.1111/j.1600-0463.2007.apm_630.x
Stępień-Pyśniak, D., Hauschild, T., Kosikowska, U., Dec, M., and Urban-Chmiel, R. (2019). Biofilm formation capacity and presence of virulence factors among commensal Enterococcus spp. from wild birds. Sci. Rep. 9:11204. doi: 10.1038/s41598-019-47602-w
Sutcliffe, J., Grebe, T., Tait-Kamradt, A., and Wondrack, L. (1996). Detection of erythromycin-resistant determinants by PCR. Antimicrob. Agents. Chemother. 40, 2562–2566. doi: 10.1128/AAC.40.11.2562
Vankerckhoven, V., Va-Autgaerden, T., Vael, C., Lammens, C., Chapelle, S., Rossi, R., et al. (2004). Development of a multiplex PCR for the detection of asa 1, gel E, cyl A, esp, and hyl genes in enterococci and survey for virulence determinants among European hospital isolates of Enterococcus faecium. J. Clin. Microbiol. 42, 4473–4479. doi: 10.1128/JCM.42.10.4473-4479.2004
Wang, Y., Lv, Y., Cai, J., Schwarz, S., Cui, L., Hu, Z., et al. (2015). A novel gene, optr A, that confers transferable resistance to oxazolidinones and phenicols and its presence in Enterococcus faecalis and Enterococcus faecium of human and animal origin. J. Antimicrob. Chemother. 70, 2182–2190. doi: 10.1093/jac/dkv116
Woksepp, H., Ryberg, A., Billström, H., Hällgren, A., Nilsson, L. E., Marklund, B. I., et al. (2014). Evaluation of high-resolution melting curve analysis of ligation-mediated real-time PCR, a rapid method for epidemiological typing of ESKAPE (Enterococcus faecium, Staphylococcus aureus, Klebsiella pneumoniae, Acinetobacter baumannii, Pseudomonas aeruginosa, and Enterobacter species) pathogens. J. Clin. Microbiol. 52, 4339–4342. doi: 10.1128/JCM.02537-14
World Health Organization [WHO] (2017). Global Priority List Of Antibiotic-Resistant Bacteria To Guide Research, Discovery, And Development Of New Antibiotics. Geneva: WHO.
Xiao-Yun, S., Guo-Zhen, D., and Hong, L. (2006). Studies of a naturally occurring molybdenum-induced copper deficiency in the yak. Vet. J. 171, 352–357. doi: 10.1016/j.tvjl.2004.11.006
Keywords: Enterococcus, yaks, antibiotic resistance, virulence, biofilm
Citation: Cui P, Feng L, Zhang L, He J, An T, Fu X, Li C, Zhao X, Zhai Y, Li H, Yan W, Li H, Luo X, Lei C, Wang H and Yang X (2020) Antimicrobial Resistance, Virulence Genes, and Biofilm Formation Capacity Among Enterococcus species From Yaks in Aba Tibetan Autonomous Prefecture, China. Front. Microbiol. 11:1250. doi: 10.3389/fmicb.2020.01250
Received: 27 February 2020; Accepted: 15 May 2020;
Published: 12 June 2020.
Edited by:
Shaolin Wang, China Agricultural University, ChinaReviewed by:
Xiaoming Wang, Nanjing Agricultural University, ChinaAna P. Tedim, Institute of Health Sciences Studies of Castilla y León (IECSCYL), Spain
Copyright © 2020 Cui, Feng, Zhang, He, An, Fu, Li, Zhao, Zhai, Li, Yan, Li, Luo, Lei, Wang and Yang. This is an open-access article distributed under the terms of the Creative Commons Attribution License (CC BY). The use, distribution or reproduction in other forums is permitted, provided the original author(s) and the copyright owner(s) are credited and that the original publication in this journal is cited, in accordance with accepted academic practice. No use, distribution or reproduction is permitted which does not comply with these terms.
*Correspondence: Xin Yang, eWFuZ3hpbjA4MjJAMTYzLmNvbQ==