- 1Co-Innovation Center for Sustainable Forestry in Southern China, College of Forestry, Nanjing Forestry University, Nanjing, China
- 2Jiangsu Key Laboratory for Prevention and Management of Invasive Species, Nanjing Forestry University, Nanjing, China
The use of volatile organic compounds (VOCs) produced by microorganisms for the biological control of plant diseases has attracted much attention in recent years. In this study, the antifungal activity and identity of VOCs produced by Rahnella aquatilis JZ-GX1 isolated from the rhizosphere soil of pine were determined and analyzed. The effect of the VOCs on the mycelial growth of Colletotrichum gloeosporioides, the pathogen of Liriodendron chinense × tulipifera black spot, was determined by a joined-petri dish fumigation method. An in vitro leaf inoculation method was used to determine the fumigation effect of the VOCs on Liriodendron black spot. VOCs with antifungal activity were collected by headspace solid-phase microextraction (SPME), and their components were analyzed by gas chromatography-mass spectrometry (GC-MS). The results showed that the VOCs secreted by JZ-GX1 inhibited the mycelial growth of the tested pathogen. The VOCs destroyed the morphology of the mycelium, significantly increased the permeability of the cell membrane and downregulated the expression of pathogenicity-related genes during mycelial infection, thus inhibiting the expansion of anthracnose disease spots in leaves. In the volatile compound profile, 3-methyl-1-butanol and 2-phenylethyl methyl ether significantly inhibited the mycelial growth and spore germination of C. gloeosporioides. This work provides a new strategy for the research and application of microorganisms and bioactive compounds to control plant anthracnose.
Introduction
Biological control of plant diseases is an important measure to reduce the use of chemical pesticides and improve plant health (Eva et al., 2019). Research based on the interaction between antagonistic microorganisms and pathogens has always been the focus of biocontrol research. Studies have shown that biocontrol agents such as Bacillus, Pseudomonas, Burkholderia, and Streptomyces play important roles in plant pathogen inhibition (Jiang et al., 2018; Roxane et al., 2018; Randa et al., 2019; Jeon et al., 2019). Biocontrol mechanisms mainly include the production of antibiotics, occupation of active sites, and nutrient or mineral competition, of which the most common strategy is the secretion of antifungal metabolites (Huang et al., 2017). At present, the most well-studied antifungal metabolites are antibiotics, cell wall-degrading enzymes and volatile organic compounds (VOCs), and the VOCs produced during microbial metabolism are particularly important biocontrol factors (Tagele et al., 2019).
In recent years, attention to VOC research has increased. Compared with other secondary metabolites of microorganisms, VOCs have many desirable properties, such as low molecular weight, low polarity, high vapor pressure, low boiling point, and lipophilicity (Sharifi and Ryu, 2018). VOCs at low concentrations can be sensed and can be transmitted over long distances, mediating indirect interactions between organisms; thus, VOCs have been used for the biological control of plant diseases (Sharifah et al., 2019). Wan et al. (2008) detected phenylethyl alcohol in the volatile profile of Streptomyces platensis F-1, and the application of phenylethyl alcohol slowed the growth of Botrytis cinerea and maintained the aroma in strawberry. The VOCs produced by Pseudomonas aureofaciens SPS-41 can be used as a biological fumigant to control disease in sweet potato tuber roots (Zhang et al., 2019). The volatiles produced by Enterobacter asburiae Vt-7 inhibited aflatoxin production in peanut during storage (Gong et al., 2019).
Colletotrichum gloeosporioides is widely distributed in tropical and subtropical regions and can infect mango, peaches, Liriodendron chinense × tulipifera, Cunninghamia lanceolata, and Camellia sinensis, causing anthracnose in leaves and fruits (Huang et al., 2018; Tang et al., 2019; Wu et al., 2019; Zhu et al., 2019; Shang et al., 2020). The conidia of this pathogen can infect the tender leaves, twigs, flowers and fruits of the host, causing leaves, flowers and fruits to fall, and fruit to decay during storage, resulting in serious economic losses. At present, chemical application is the main control strategy for plant anthracnose. However, with the widespread application of chemical pesticides, the selection pressure on pathogens increases. Anthracnose in many areas also has varying degrees of drug resistance. Wang et al. (2019) found significant differences in the sensitivity of 13 Colletotrichum anthracnose isolates from walnut fruits and leaves on walnut plantations in China to the same fungicide. Mancozeb, which is used to control anthracnose in mango orchards, has had similar effects (Chanchaichaovivat et al., 2007). Therefore, reducing the drug resistance of pathogens through biological control has become an important issue in the prevention and control of agricultural and forestry diseases.
Rahnella aquatilis JZ-GX1 is a plant growth-promoting bacterium isolated from the rhizosphere of Pinus massoniana in our laboratory. Previous studies have shown that it has strong inhibitory activity against poplar canker pathogen (Cytospora chrysosperma) and seedling quenching pathogen (Rhizoctonia solani) (Song et al., 2017; Kong et al., 2019b) and can promote the growth of Cinnamomum camphora and Pinus massoniana (Li et al., 2013; Kong et al., 2019a). However, it is not clear whether the strain can produce volatile compounds and whether its VOCs have inhibitory effects on plant pathogens. In this study, the antagonistic effect of the VOCs emitted by the JZ-GX1 strain against the anthracnose pathogen Liriodendron chinense × tulipifera was evaluated to isolate and identify individual volatile compounds with antifungal activity, to reveal the antagonistic mechanism behind the effect of the volatile compounds against plant pathogens, and to develop new microbial resources to control plant diseases caused by C. gloeosporioides.
Materials and Methods
Tested Bacterial and Fungal Strains
Rahnella aquatilis JZ-GX1 is a plant growth-promoting bacterium isolated from the rhizosphere soil of a 28-year-old P. massoniana in Nanning, Guangxi. It is now stored in the typical Culture Preservation Center of China (CCTCC, No: M2012439). After activation, the JZ-GX1 strain was cultured overnight on LB liquid medium at 28°C.
The pathogen C. gloeosporioides was isolated from a susceptible Liriodendron chinense × tulipifera on the campus of Nanjing Forestry University. The strain was cultured on potato glucose agar (PDA) medium at 25°C for 7 days.
Determination of the Antagonistic Effect of VOCs Produced by Strain JZ-GX1 on C. gloeosporioides
The antifungal activity of VOCs produced by JZ-GX1 was detected after culturing on two sealed petri dishes (Gao et al., 2017). One petri dish contained 20 mL LB medium, and the other 20 mL PDA medium. The LB medium was coated with 100 μL JZ-GX1 suspension, and a 6 mm diameter plug of C. gloeosporioides was placed on the PDA plate. Then, the bottoms of the two petri dishes were sealed with Parafilm and cultured in a constant-temperature incubator at 25°C for 5 days. Each experiment was repeated three times. The inhibition rate = (Cd - Td) × 100%/Cd, where Cd is the colony diameter on the control PDA plate and Td is the colony diameter on the treated PDA plate.
Scanning Electron Microscopy (SEM) Observation of the Mycelium of C. gloeosporioides
The mycelium morphology of C. gloeosporioides was observed and analyzed by SEM (Quanta 200FEIJI, United States) in C. gloeosporioides after 5 days of VOC treatment and in C. gloeosporioides that had not been exposed to the JZ-GX1 strain. A mycelium sample was fixed in 4% glutaraldehyde solution for 24 h and washed with pH = 7.2 phosphate buffer (Edgar et al., 2019). The mycelium samples were dehydrated with an ethanol gradient (70, 80, 90%, and anhydrous ethanol). Then, the gold layer was sputtered with liquid CO2 in a critical point dryer (EMITECH K850) for 15 min. The specimens were subsequently mounted on stubs and sputtered with gold (HITACH E-1010). The scanning voltage was 20 kV (Li et al., 2013).
Determination of Nucleic Acid Exosmosis by Pathogenic Mycelium
To evaluate the effect of VOCs on the nucleic acid leakage of C. gloeosporioides, the OD260 of a mycelial cell suspension was determined by ultraviolet spectrophotometry. LB medium was added to one petri dish, onto which 100 μL JZ-GX1 bacterial suspension was coated, and PDA medium was poured into another petri dish. After cooling, sterilized cellophane was spread on the PDA medium, and 100 μL of a spore suspension (108 cfu/mL) of C. gloeosporioides was evenly coated on the cellophane. Then, the bottoms of the two dishes were sealed with Parafilm. The plates were cultured at 25°C, and mycelial cells were removed at 12, 24, 36, 48, and 60 h and washed with 5 mL aseptic water. After centrifugation, the OD260 value of the supernatant was determined by an ultraviolet spectrophotometer (Zhang, 2015). The experiment was carried out three times in parallel and repeated three times.
Determination of Malondialdehyde (MDA) and Soluble Sugar in Pathogenic Mycelium
A total of 0.5 g mycelium of C. gloeosporioides fumigated with VOCs, 0.1 g quartz sand and 2 mL 10% trichloroacetic acid were fully ground into a homogenate with a mortar and pestle. Then, 8 mL 10% trichloroacetic acid was added, and grinding continued. The homogenate was centrifuged at 4000 rpm for 10 min, and MDA was recovered in the supernatant. Next, 2 mL supernatant was added to a clean test tube, and a control tube was filled with 2 mL distilled water; 2 mL 0.6% thiobarbituric acid solution was added to each tube. The tubes were shaken and allowed to react in a boiling water bath for 15 min. The tubes were quickly cooled and then centrifuged. The absorbance (A) of the final supernatant was determined at 532, 600, and 450 nm, and the MDA concentration was calculated as follows: c (MDA) (μM) = 6.45 (A532 - A600) - 0.56A450; c (soluble sugar) (μM) = 11.71A450 (Song et al., 2017).
RNA Extraction and RT-qPCR Analysis of Pathogenic Mycelium
The mycelium of C. gloeosporioides was inoculated in CMC liquid medium and shaken at 25°C and 150 rpm for 48 h. The suspension was filtered through monolayer gauze and diluted to obtain a 106 cfu/mL fresh spore suspension. Then, 100 μL conidial suspension was coated on a PDA plate. The LB plate inoculated with JZ-GX1 was joined with the PDA plate containing the conidial suspension of C. gloeosporioides. The bottoms of the two culture dishes were sealed with Parafilm and cultured in the dark at 28°C. Conidia on PDA without JZ-GX1 treatment were used as a control. C. gloeosporioides was collected 24 h later for RNA extraction. Total RNA was extracted with TRIzol reagent according to the manufacturer’s instructions. After DNaseI treatment, 2 μg ribonucleic acid was added to the 20 μL reaction system, and first-strand cDNA was synthesized by reverse transcription according to the manufacturer’s instructions. HiScript II Q Select RT Supermix was used to prepare cDNA samples for qPCR (China). Using 1.0 μL cDNA diluted 1:10 as the template, the whole reaction system was carried out in an ABI 7500 system (Applied Biosystems, United States). Three pathogenicity-related genes, polyketone synthase (PKS), endotoxin galacturonase (PG), and cysteine dehydratase (SCD), were selected for RT-qPCR analysis (Table 1). The actin gene was used as an internal reference gene because of its relatively stable expression level. The relative quantification of gene expression changes was carried out by the 2–Δ Δ CT method (Li Z.Q. et al., 2017; Su et al., 2018).
Determination of Anthracnose Spot on Liriodendron chinense × tulipifera Treated With VOCs
A petri dish with a diameter of 15 cm was coated with JZ-GX1 bacterial solution, and a blank LB plate was used as the control. Medium-sized hybrid Liriodendron leaves were placed at the bottom of another petri dish, and the petioles were wrapped with wet cotton balls for moisturization. Then, the leaf surfaces were sprayed and disinfected with 75% alcohol, and two inoculation points were selected on each side of the main vein. After puncture of the leaf surface, the wound was inoculated with C. gloeosporioides plugs (Φ = 6 mm), with 10 dishes per treatment and three replicates. Each petri dish inoculated with JZ-GX1 and each petri dish inoculated with pathogenic fungal leaves were joined together and sealed with Parafilm. After 2 and 4 days of confrontation culture at 25°C, the expansion of lesions on the leaves was observed, and the diameter of each lesion was measured (Xu et al., 2017; Wang et al., 2018).
Identification of VOCs and Inhibitory Analysis
The analysis of VOCs emitted by JZ-GX1 was performed with headspace solid-phase microextraction (HS-SPME) coupled with gas chromatography-mass spectrometry (GC-MS). A single colony of JZ-GX1 was inserted into a 200 mL flask containing 50 mL of liquid LB medium and fermented at 28°C with shaking at 180 rpm for 2 days. LB liquid medium without bacterial inoculation was used as the control. A 65 μm PDMS/DVB fiber was selected for the determination of VOCs. The SPME fiber was inserted into the flask and cultured at 40°C for 30 min. After the extraction, the fiber was quickly retracted, and the needle was pulled out and immediately inserted into the GC inlet (TraceISQ, Thermo Fisher Scientific, United States). The fiber was pushed down into the inlet at 230°C desorption for 3 min. The CK and JZ-GX1 treatments were tested three times.
Gas chromatographic conditions: DB-5MS capillary column (30 m × 0.25 mm × 0.25 μm), He carrier gas; injector port temperature of 230°C; initial temperature of 40°C, maintained for 3 min, raised to 95°C at 10°C/min, then to 230°C at 3°C/min, and maintained for 5 min.
Mass spectrometry conditions: EI ion source; electron energy of 70 eV; ion source temperature of 250°C; interface temperature of 250°C; data acquisition rate, 0.2 s/time and spectrum retrieval using the NIST 05 and NIST 05s libraries (Zhang, 2013).
To test the antifungal activity of JZ-GX1 VOCs, we used authentic reference standard compounds purchased from Rongshide Trading Co., Ltd. (Nanjing, China). PDA medium (20 ml) was added to a petri dish; 7-day-old anthracnose plugs (6 mm) and conidia (100 μL of conidia at 108 cfu/mL) were inoculated in the center of the PDA medium with a punch. Another petri dish was added with authentic standard to final concentrations of 10, 20, 100, and 200 μL/L (compound volume to airspace volume). The bottoms of the two petri dishes were joined together and sealed with film. After culturing at 25°C for 3 days, the inhibition by the standard was observed; anthrax was inoculated alone as the control. Each process was repeated three times.
Statistical Analysis
The data were analyzed by analysis of variance and Duncan’s multiple comparison with SPSS 22.0 software, and the standard errors of all mean values were calculated (P < 0.05).
Results
Antagonistic Effect of VOCs Produced by JZ-GX1 Against C. gloeosporioides
The determination of the mycelial growth inhibition rate showed that the VOCs produced by JZ-GX1 had a good inhibitory effect on the colony growth of C. gloeosporioides. Compared with that of the control group, the colony diameter of C. gloeosporioides treated with JZ-GX1 was significantly inhibited (Figure 1A). With extension of the culture time, the relative rate of inhibition by the VOCs produced by JZ-GX1 to C. gloeosporioides gradually increased (Figure 1B), reaching 63.16% after 5 days of culture.
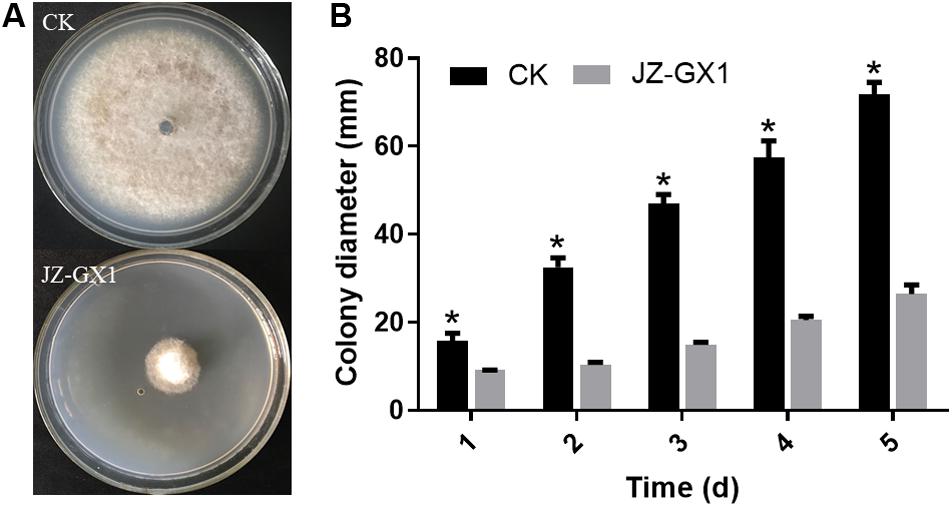
Figure 1. Effect of R. aquatilis JZ-GX1 on the mycelial growth of C. gloeosporioides. (A) Colony morphology of C. gloeosporioides in vitro. (B) Colony diameter of C. gloeosporioides. Vertical bars represent the standard deviation of the average. One-way ANOVA analysis was performed and Duncan’s post hoc test was applied. Asterisks indicate statistically significant differences (p < 0.05) among treatments.
Morphological Changes of Mycelium After Coculture of C. gloeosporioides and Strain JZ-GX1
To observe the effect of the VOCs produced by JZ-GX1 on the mycelium morphology of C. gloeosporioides, changes in mycelium morphology were observed by SEM. The results showed that the surface of the pathogenic mycelium treated with JZ-GX1 VOCs was rough, with obvious wrinkles and collapsed areas, while the surface of the mycelium without JZ-GX1 VOCs treated was neat, plump and smooth (Figure 2).
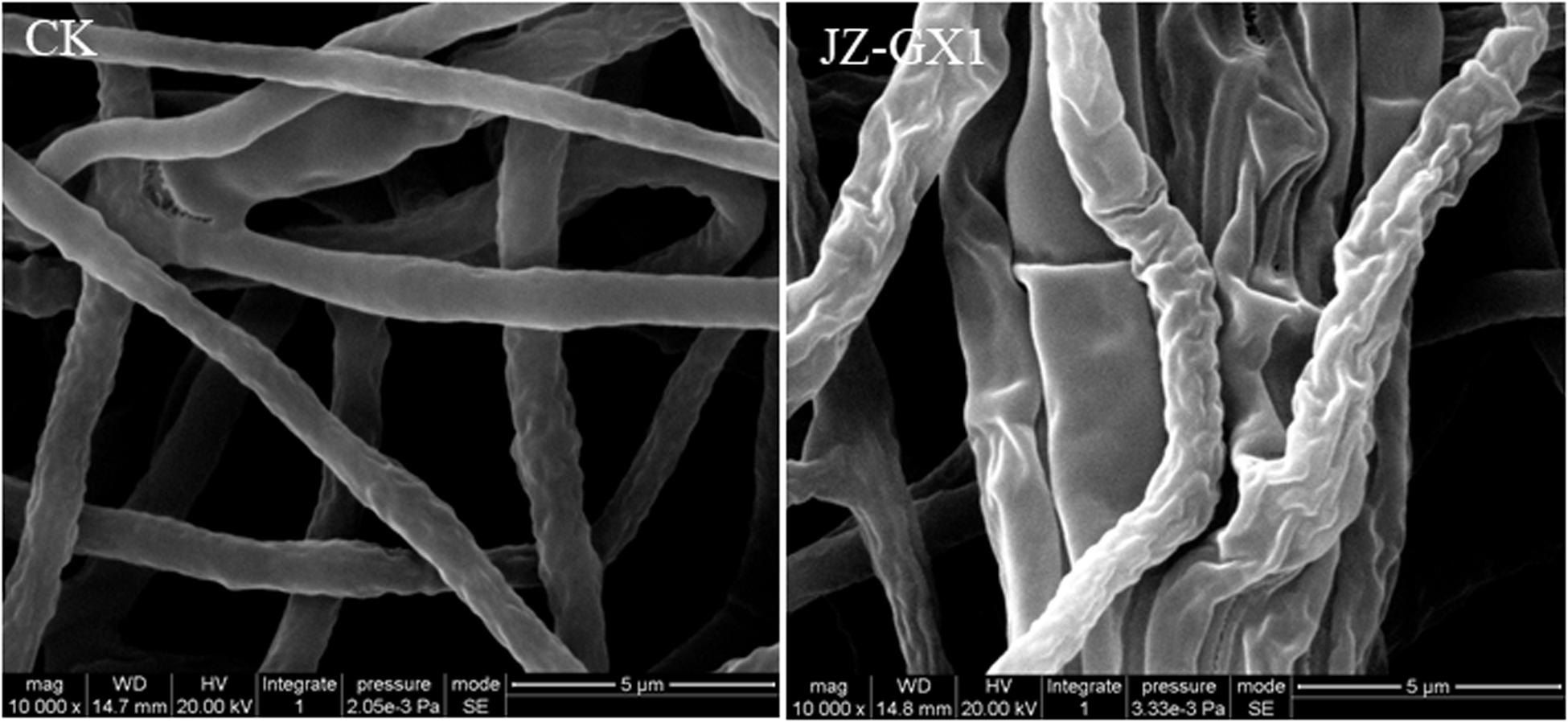
Figure 2. Morphological observation of C. gloeosporioides via SEM. CK: untreated control group; JZ-GX1: treated with VOCs produced by R. aquatilis JZ-GX1.
Effect of VOCs Released by JZ-GX1 on the Cell Membrane Permeability of C. gloeosporioides
After the VOCs produced by JZ-GX1 were incubated with C. gloeosporioides for 36 h, the OD260 of the centrifuged mycelial suspension was significantly higher than that of the blank control group (Figure 3A). The longer the incubation time with the VOCs produced by JZ-GX1 was, the higher the OD260 value of the centrifuged mycelial suspension, indicating that the cell membrane of C. gloeosporioides was greatly damaged, that is, high leakage of nucleic acids, by the VOCs.
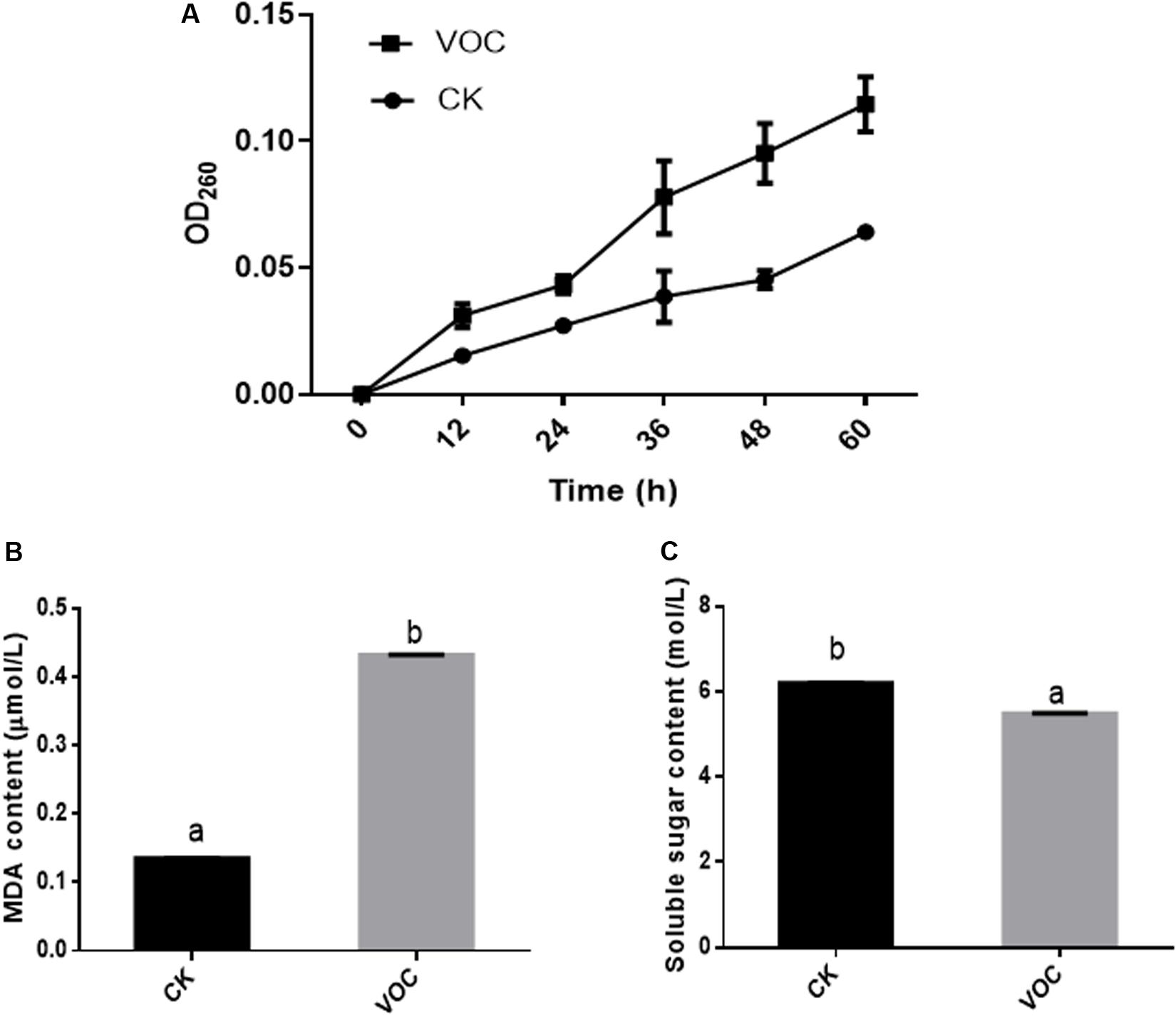
Figure 3. Effects of R. aquatilis JZ-GX1 on nucleic acid leakage (A), the MDA content (B), and the soluble sugar content (C) in the mycelia of C. gloeosporioides. The vertical bars represent the standard deviation of the average. One-way ANOVA analysis was performed and Duncan’s post hoc test was applied. Different letters indicate statistically significant differences (p < 0.05) among treatments.
The damage to C. gloeosporioides lipids by JZ-GX1 VOCs was determined by measuring the MDA content. Compared with that in the control, the content of MDA in the mycelium of C. gloeosporioides increased after treatment with JZ-GX1 VOCs for 60 h (Figure 3B), indicating that the VOCs produced by JZ-GX1 enhanced the oxidative damage in the mycelium of C. gloeosporioides. In addition, the soluble sugar concentration in C. gloeosporioides in the treatment group was significantly lower than that in the control group (Figure 3C), which also reflected the hydrolysis of the mycelial cell wall of C. gloeosporioides.
R. aquatilis JZ-GX1 Inhibited the Expression of Pathogenicity-Related Genes in C. gloeosporioides
To better understand the effect of JZ-GX1 VOCs on the infection of Liriodendron chinense × tulipifera leaves by C. gloeosporioides, the differential expression of pathogenicity-related genes in C. gloeosporioides was analyzed. Compared with the expression in the control, the selected genes were downregulated in the presence of JZ-GX1 VOCs. In particular, the expression of the PG gene was downregulated 19.61-fold. The expression of PKS and SCD decreased 3.50- and 2.35-fold, respectively, which was significantly different from their expression in the control (Figure 4).
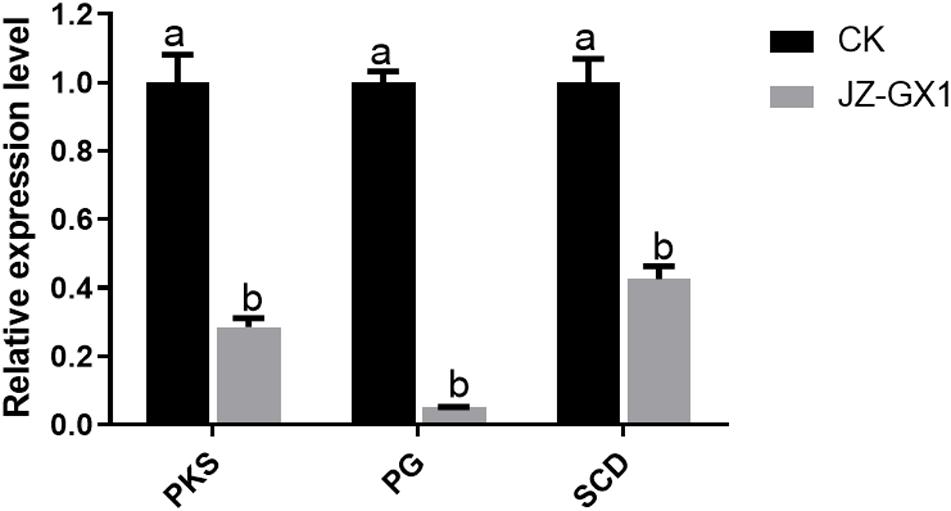
Figure 4. Relative expression of pathogenicity-related genes in C. gloeosporioides treated with R. aquatilis JZ-GX1 VOCs. The vertical bars represent the standard deviation of the average. One-way ANOVA analysis was performed and Duncan’s post hoc test was applied. Different letters indicate statistically significant differences (p < 0.05) among treatments.
Inhibition by the VOCs Produced by R. aquatilis JZ-GX1 of Infection by C. gloeosporioides
The VOCs produced by JZ-GX1 significantly inhibited leaf anthracnose on Liriodendron chinense × tulipifera caused by C. gloeosporioides (Figure 5A). Two days after inoculation with C. gloeosporioides, the control leaves of Liriodendron chinense × tulipifera were completely infected, while the incidence in leaves treated with the JZ-GX1 strain was 45.7% (Figure 5B). After 2 and 4 days of inoculation, the average spot diameters of leaves treated with the JZ-GX1 strain were 5.67 and 15 mm, respectively, while those of control leaves were 28.33 and 66 mm, respectively (Figure 5C). These results showed that VOCs from the JZ-GX1 strain could significantly inhibit the expansion of anthracnose spots in Liriodendron chinense × tulipifera leaves.
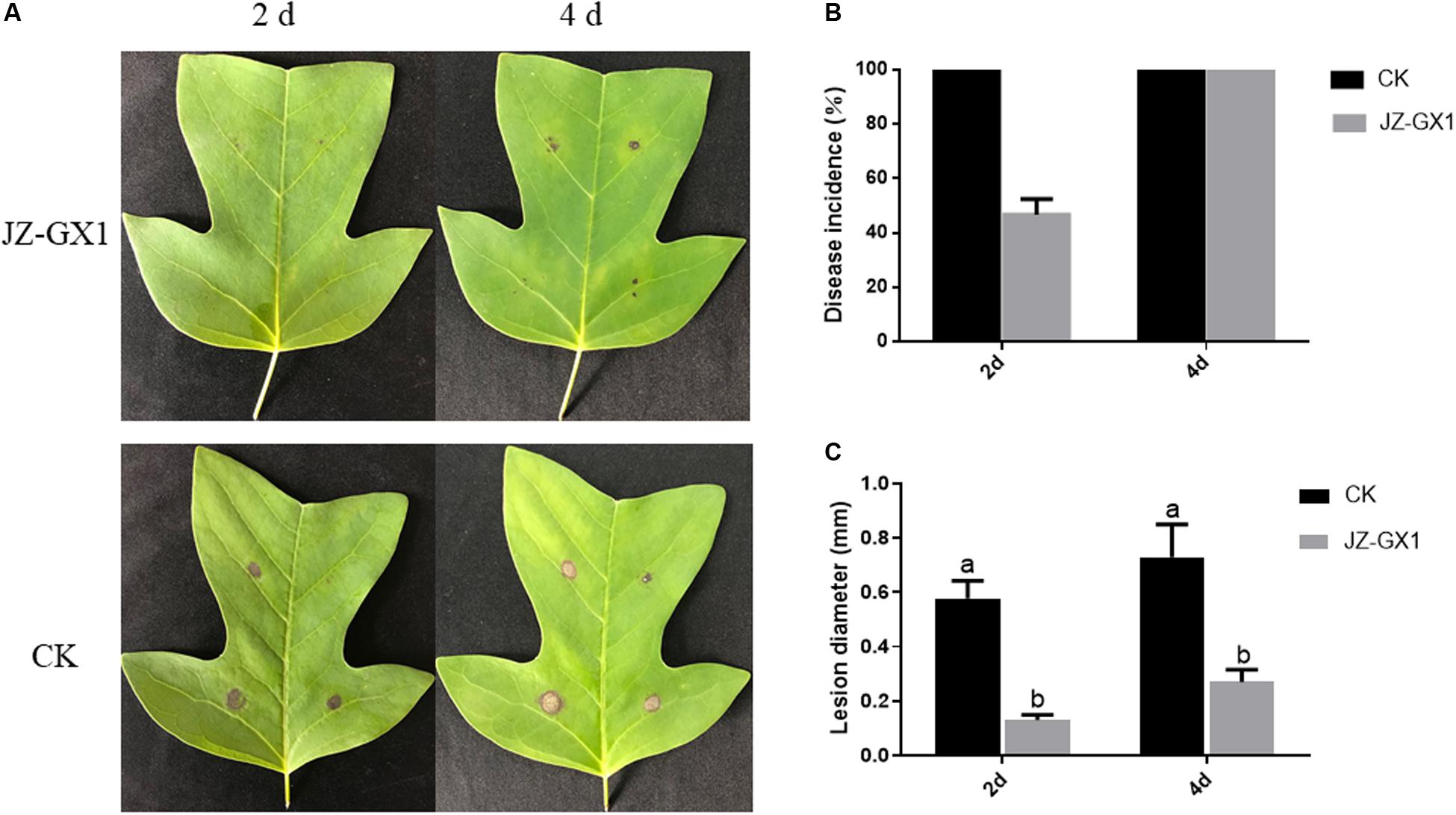
Figure 5. Inhibition by JZ-GX1 of spot expansion on Liriodendron chinense × tulipifera leaves. (A) Symptoms of anthracnose in leaves, (B) incidence of anthracnose in leaves, and (C) spot diameter of anthracnose. One-way ANOVA analysis was performed and Duncan’s post hoc test was applied. The vertical bars represent the standard deviation of the mean. Different letters indicate statistically significant differences (p < 0.05) among treatments.
Collection and Identification of VOCs From R. aquatilis JZ-GX1
The VOCs from JZ-GX1 were collected by an SPME syringe and analyzed by a GC-MS/MS system. VOCs that were also detected in the LB medium and VOCs with relative contents less than 0.5% were filtered out. As shown in Figure 6, there is a very obvious difference between the control and strain JZ-GX1 (Supplementary Data Sheets S1, S2). Eight VOCs released by JZ-GX1 were identified: two acids, two alcohols, two ketones, one ester, and one ether. The most abundant VOCs were 2-Phenylethyl methyl ether (22.87 ± 12.34% by GC), followed by phenylethyl alcohol (16.67 ± 6.61%). To evaluate their potential biological activity, we purchased the reference materials in Table 2 and determined their antagonistic activities against mycelium and conidia of C. gloeosporioides.
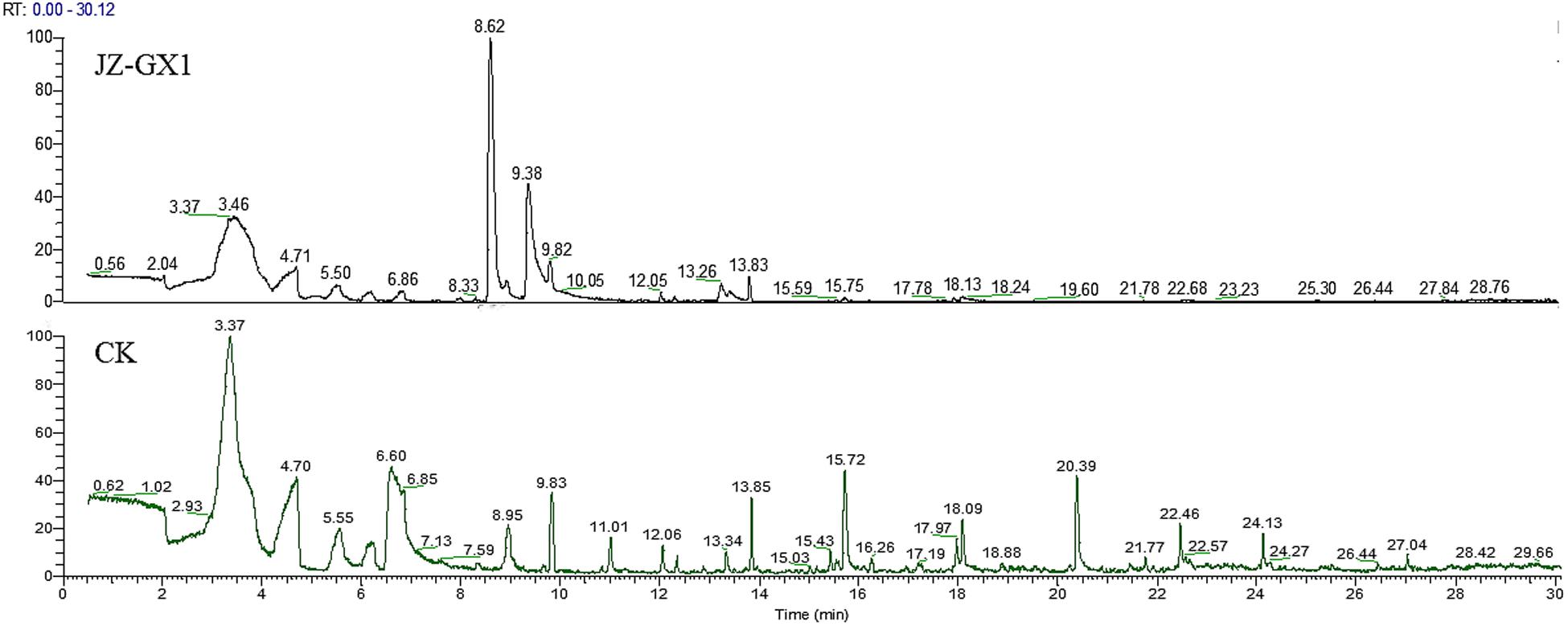
Figure 6. GC-MS/MS spectra of the VOCs emitted from JZ-GX1 incubated in LB medium for 48 h (JZ-GX1) and non-inoculated LB medium (CK).
Determination of Antifungal VOCs and Analysis of the Minimum Inhibitory Concentration (MIC) of JZ-GX1 Against C. gloeosporioides
Standards for 8 VOCs released by JZ-GX1 were used to determine the antifungal activity against C. gloeosporioides. None of the standards except phenylethyl alcohol, 3-methyl-1-butanol and 2-phenylethyl methyl ether exhibited antifungal activity. These three standards were diluted to different concentrations and cocultured with C. gloeosporioides in a sealed petri dish to detect their antagonistic activity. In the concentration range of 10–200 μL/L, all three standards easily volatilized and inhibited mycelium growth and conidia germination. Among them, 3-methyl-1-butanol showed the best antagonistic activity, with MICs against anthracnose mycelium and conidia of 100 and 10 μL/L, respectively, followed by 2-phenylethyl methyl ether, with MICs of 100 and 50 μL/L, respectively. For phenylethyl alcohol, the inhibitory effect on C. gloeosporioides mycelium growth and germination was not ideal, as its MIC was above 200 μL/L (Figure 7).
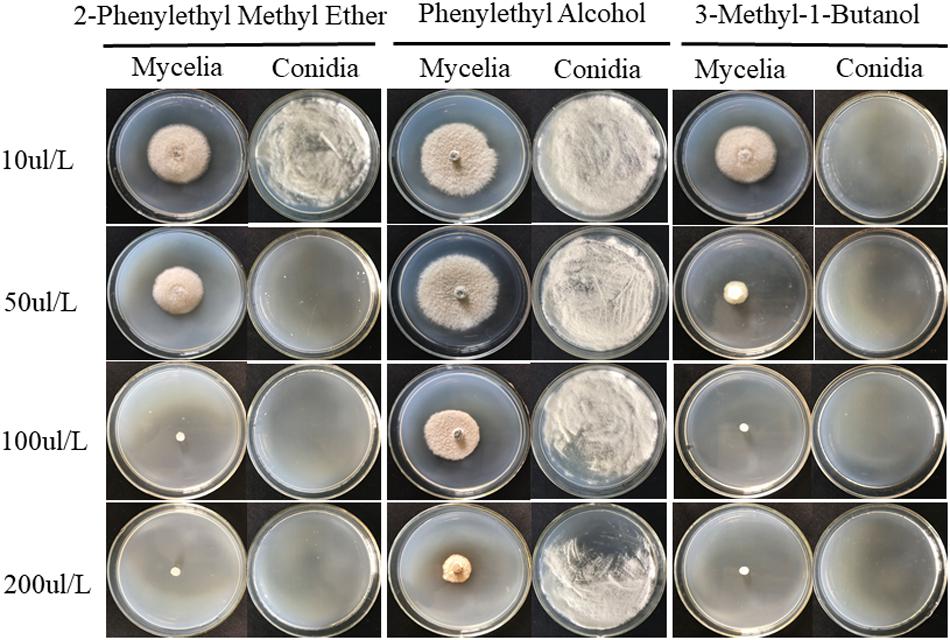
Figure 7. Three individual compounds, 2-phenylethyl methyl ether, phenylethyl alcohol and 3-methyl-1-butanol, at different concentrations were assayed for their inhibitory activity against the mycelial growth and conidial germination of C. gloeosporioides.
Discussion
The VOCs from microorganisms are generally lipophilic and have low boiling points. Therefore, they can be freely released into the external environment from biofilms. Some VOCs can act as signal transducers to communicate with other organisms (Adam et al., 2017; Avalos et al., 2020). In recent years, an increasing number of studies have shown that VOCs produced by beneficial microorganisms will affect the growth of plant pathogenic fungi. However, to date, there has been no report on the production of VOCs with antifungal activity by Rahnella spp. Moreover, there are few reports regarding the biological activity of Rahnella spp. It has been reported that R. aquatilis ZF7 has a strong rhizosphere colonization ability and broad-spectrum plant growth-promoting activity (Yuan et al., 2019). R. aquatilis HX2 can control grape root cancer by producing bacteriocin (Li et al., 2014). In this study, we reported for the first time that the VOCs produced by R. aquatilis JZ-GX1 can inhibit the mycelium growth and spore germination of C. gloeosporioides.
Understanding the antagonistic characteristics of volatile microbial metabolites is helpful to further reveal the biological mechanism of antagonistic bacteria. The cell membrane is the basis for maintaining cell integrity and normal material and energy metabolism. When the cell membrane is destroyed, some intracellular proteins, phosphates, carbonates, DNA and RNA will be released, and these nuclear substances strongly absorb UV at 260 nm (Vitro et al., 2005). Chen and Cooper (2002) reported that some antimicrobial substances can destroy the cell membrane of microorganisms and increase their cell membrane permeability to achieve bacteriostasis. Therefore, a change in cell membrane integrity can be inferred by detecting the ultraviolet absorption of a suspension. In this study, as the treatment time with R. aquatilis JZ-GX1 VOCs increased, more nucleic acids leaked out of C. gloeosporioides. Similarly, MDA is one of the most important products of membrane lipid peroxidation. The degree of membrane lipid peroxidation can be determined by measuring the content of MDA, and the damage degree of the membrane system can be determined indirectly (Xu et al., 2017). Some studies have shown that the fermentation broth of Trichoderma virens T43 can hydrolyze the proteins and sugars of pathogenic fungi, increase the content of MDA, and eventually lead to cell death (Yin et al., 2014). In this study, the MDA content in the mycelium of C. gloeosporioides treated with JZ-GX1 VOCs for 4 days was significantly higher than that of the control group, and the soluble sugar content decreased in the mycelia of C. gloeosporioides stressed by JZ-GX1 VOCs. Therefore, it is speculated that one of the targets of JZ-GX1 VOCs is a location on the C. gloeosporioides cell membrane, and antagonistic effects can be achieved by destroying the integrity of the cell membrane.
In the process of infecting a host, a pathogen will efficiently regulate the expression of its own pathogenicity-related genes, thus regulating the growth of conidia or hyphae in a direction conducive to its own infection, realizing the smooth colonization of the pathogen and causing the host to be susceptible to disease (Lin et al., 2012; Upadhyay et al., 2013). For this reason, we further discussed the expression of three pathogenicity-related genes, SCD, PG, and PKS. Some studies have shown that the appressorium plays an important role in infection by C. gloeosporioides: melanin synthesis and accumulation can increase the swelling and pressure of the appressorium, thus promoting successful pathogen infection. SCD is one of the key enzymes in the melanin biosynthesis pathway, and the expression of this gene will affect the infection efficiency of C. gloeosporioides (Nosanchuk and Casadevall, 2006). PG in the pathogen can degrade pectin in the cell wall of the host and promote host colonization (Alkan et al., 2015). PKS is a key enzyme that regulates the synthesis of polyketones, secondary metabolites involved in defense or cell-to-cell communication (Noar et al., 2019). In this study, qPCR assays showed that all three key genes were downregulated by VOCs, which suggested that the VOCs of JZ-GX1 interfered with the infection activity, colonization ability and host resistance of C. gloeosporioides, thus reducing the degree of infection in the leaves of Liriodendron chinense × tulipifera.
To determine which VOCs produced by R. aquatilis JZ-GX1 inhibited the growth of C. gloeosporioides, the commonly used headspace SPME and GC-MS techniques were used to analyse individual VOCs. The main VOCs produced by JZ-GX1 included eight compounds: ketones, hydrocarbons, ethers, esters, and alcohols. Among them, 3-methyl-1-butanol and 2-phenylethyl methyl ether had the best antagonistic effects against C. gloeosporioides. Previous studies have reported the antifungal properties of 3-methyl-1-butanol. For example, the VOCs produced by the endophytic fungus Phaeosphaeria nodorum include 3-methyl-1-butanol, which inhibits the growth of the mycelium of peach brown rot and leads to mycelial disintegration (Pimenta et al., 2012). The median effective dose of 3-methyl-1-butanol produced by Muscodor suthepensis CMUCib462 was 250.29 ± 0.29 μL/L against Penicillium digitatum growth (Suwannarach et al., 2016). 2-Phenylethyl methyl ether has a pleasant floral fragrance, so it is widely used as an ingredient and flavoring in the food and cosmetics industries (Pan et al., 2013; Li H. H. et al., 2017). However, there are no reports about this compound in the antifungal volatile components of plants or microorganisms in the existing literature. In this study, we first reported that 2-phenylethyl methyl ether has strong inhibitory activity against C. gloeosporioides, but whether it has antagonistic effects against other pathogens remains to be further studied. Although the relative content of 3-methyl-1-butanol in JZ-GX1 VOCs is relatively low, 10 μL of the pure compound can inhibit the germination of C. gloeosporioides spores. However, phenylethyl alcohol, which accounts for a relatively high percentage of the VOC profile, did not show antifungal activity. The content of each VOC produced by a microorganism is not directly related to its inhibitory effect on pathogenic fungi: some compounds are abundant, but their inhibitory effect on pathogenic fungi is not obvious, while the content of some compounds is low, but their inhibitory effect is significant.
In summary, this study proved for the first time that VOCs produced by Rahnella spp. could directly inhibit the spore germination and mycelial growth of C. gloeosporioides, significantly reduce the contents of nucleic acids and soluble sugars in pathogenic mycelia, increase the content of MDA, destroy the integrity of the cell membrane and decrease the expression of pathogenic genes. As a result, the infection activity and vitality of the pathogen were reduced, and the occurrence and damage degree of black spot in the leaves of Liriodendron chinense × tulipifera were reduced. Two VOCs with antifungal activity in JZ-GX1 were studied and identified. Considering the wide host range of C. gloeosporioides, which can infect the leaves and fruits of many plants, the discovery of these antifungal compounds is of practical significance for the development of new fungicides. Furthermore, the application of strain JZ-GX1 to the storage and preservation of postharvest fruit and the biological fumigation of soil-borne diseases will also have good application prospects.
Data Availability Statement
All datasets generated for this study are included in the article/Supplementary Material.
Author Contributions
W-LK completed the data analysis and the first draft of the manuscript. W-LK and LR were the finishers of the experimental research. HN participated in the experimental result analysis. X-QW directed experimental design, data analysis, manuscript writing and revision. All authors read and agreed on the final text.
Funding
This work was supported by the National Key Research and Development Program of China (2017YFD0600104) and the Priority Academic Program Development of the Jiangsu Higher Education Institutions (PAPD).
Conflict of Interest
The authors declare that the research was conducted in the absence of any commercial or financial relationships that could be construed as a potential conflict of interest.
Acknowledgments
We would like to thank Dr. Xiao-Yue Ji of the Modern Analysis and Testing Center of Nanjing Forestry University for his technical assistance in gas chromatography analysis.
Supplementary Material
The Supplementary Material for this article can be found online at: https://www.frontiersin.org/articles/10.3389/fmicb.2020.01114/full#supplementary-material
DATA SHEET S1 | Report of GC-MS spectra from CK.
DATA SHEET S2 | Report of GC-MS spectra from JZ-GX1.
References
Adam, O., Sylwia, J., and Paolina, G. (2017). The antimicrobial volatile power of the rhizospheric isolate Pseudomonas donghuensis P482. PLoS One 12:e0174362. doi: 10.1371/journal.pone.0174362
Alkan, N., Friedlander, G., and Ment, D. (2015). Simultaneous transcriptome analysis of Colletotrichum gloeosporioides and tomato fruit pathosystem reveals novel fungal pathogenicity and fruit defense strategies. New Phytol. 205:801. doi: 10.1111/nph.13087
Avalos, M., Garbeva, P., and Raaijmakers, J. M. (2020). Production of ammonia as a low-cost and long-distance antibiotic strategy by Streptomyces species. ISME J. 14, 569–583. doi: 10.1038/s41396-019-0537-2
Chanchaichaovivat, A., Ruenwongsa, P., and Panijpan, B. (2007). Screening and identification of yeast strains from fruits and vegetables: potential for biological control of postharvest chilli anthracnose (Colletotrichum capsica). Biol. Control 42, 326–335. doi: 10.1016/j.biocontrol.2007.05.016
Chen, C. Z., and Cooper, S. L. (2002). Interactions between dendrimer biocides and bacterial membranes. Biomaterials 23, 3359–3368. doi: 10.1016/S0142-9612(02)00036-4
Edgar, G. A., Adriana, B. A., Ana-Luisa, K. M., Mónica, R. V., Alfonso, M. B., Aguirre, W. E., et al. (2019). Avocado rhizobacteria emit volatile organic compounds with antifungal activity against Fusarium solani, Fusarium sp. associated with Kuroshio shot hole borer, and Colletotrichum gloeosporioides. Microbiol. Res. 219, 74–83. doi: 10.1016/j.micres.2018.11.009
Eva, A., Sandra, T., Carmen, V., Antonio, V., and Francisco, C. M. (2019). Fitness features involved in the biocontrol interaction of Pseudomonas chlororaphis with host plants: the case study of PcPCL1606. Front. Microbiol. 10:719. doi: 10.3389/fmicb.2019.00719
Gao, Z. F., Zhang, B. J., Liu, H. P., Han, J. C., and Zhang, Y. J. (2017). Identification of endophytic Bacillus velezensis ZSY-1 strain and antifungal activity of its volatile compounds against Alternaria solani and Botrytis cinerea. Biol. Control 105, 27–39. doi: 10.1016/j.biocontrol.2016.11.007
Gong, A. D., Dong, F. Y., Hu, M. J., Kong, X. W., Wei, F. F., Gong, S. J., et al. (2019). Antifungal activity of volatile emitted from Enterobacter asburiae Vt-7 against Aspergillus flavus and aflatoxins in peanuts during storage. Food Control 106:106718. doi: 10.1016/j.foodcont.2019.106718
Huang, L., Li, Q. C., Hou, Y., Li, G. Q., Yang, J. Y., Li, D. W., et al. (2017). Bacillus velezensis strain HYEB5-6 as a potential biocontrol agent against anthracnose on Euonymus japonicus. Biocontrol Sci. Technol. 27, 636–653. doi: 10.1080/09583157.2017.1319910
Huang, L., Zhu, Y. N., Yang, J. Y., Li, D. W., Li, Y., Bian, L. M., et al. (2018). Shoot blight on Chinese fir (Cunninghamia lanceolata) is caused by Bipolaris oryzae. Plant Dis. 102, 500–506. doi: 10.1094/PDIS-07-17-1032-RE
Jeon, C. W., Kim, D. R., and Kwak, Y. S. (2019). Valinomycin, produced by Streptomyces sp. S8, a key antifungal metabolite in large patch disease suppressiveness. World J. Microbiol. Biotechnol. 35:128. doi: 10.1007/s11274-019-2704-z
Jiang, C. H., Liao, M. J., Wang, H. K., Zheng, M. Z., Xu, J. J., and Guo, J. H. (2018). Bacillus velezensis, a potential and efficient biocontrol agent in control of pepper gray mold caused by Botrytis cinereal. Biol. Control 26, 147–157. doi: 10.1016/j.biocontrol.2018.07.017
Kong, W. L., Wu, X. Q., and Zhao, Y. J. (2019a). Effects of Rahnella aquatilis JZ-GX1 on treat chlorosis induced by iron deficiency in Cinnamomum camphora. J. Plant Growth Regul. doi: 10.1007/s00344-019-10029-8
Kong, W. L., Zhou, M., and Wu, X. Q. (2019b). Characteristics of siderophores production by Rahnella aquatilis JZ-GX1 and its antagonism against forest pathogens, (in Chinese). Microbiol. China 46, 3278–3285. doi: 10.13344/j.microbiol.china.190070
Li, G. E., Wu, X. Q., Ye, J. R., Hou, L., Zhou, A. D., and Zhao, L. (2013). Isolation and identification of phytate-degrading rhizobacteria with activity of improving growth of poplar and Masson pine. World J. Microbiol. Biotechnol. 29, 2181–2193. doi: 10.1007/s11274-013-1384-3
Li, H. H., Hu, X. M., Li, A. J., Sun, J. Y., Huang, M. Q., Sun, X. T., et al. (2017). Comparative analysis of volatile components in gujinggong liquor by headspace solid-phase microextraction and stir bar sorptive extraction, (in Chinese). Food Sci. 38, 155–164. doi: 10.7506/spkx1002-6630-201704025
Li, L., Jiao, Z. W., Hale, L., Wu, W. L., and Guo, Y. B. (2014). Disruption of gene pqqA or pqqB reduces plant growth promotion activity and biocontrol of crown gall disease by Rahnella aquatilis HX2. PLoS One 9:e115010. doi: 10.1371/journal.pone.0115010
Li, Z. Q., Ding, B., Zhou, X. P., and Wang, G. L. (2017). The rice dynamin-related protein OsDRP1E negatively regulates programmed cell death by controlling the release of cytochrome c from mitochondria. PLoS Pathog. 13:e1006157. doi: 10.1371/journal.ppat.1006157
Lin, S. Y., Okuda, S., and Ikeda, K. (2012). LAC2 encoding a secreted laccase is involved in appressorial melanization and conidial pigmentation in Colletotrichum orbiculare. Mol. Plant Microb. Interact. 25, 1552–1561. doi: 10.1094/MPMI-05-12-0131-R
Noar, R. D., Thomas, E., and Daub, M. E. (2019). A novel polyketide synthase gene cluster in the plant pathogenic fungus Pseudocercospora fijiensis. PLoS One 14:e0212229. doi: 10.1371/journal.pone.0212229
Nosanchuk, J. D., and Casadevall, A. (2006). Impact of melanin on microbial virulence and clinical resistance to antimicrobial compounds. Antimicrob. Agents Chemother. 50, 3519–3528. doi: 10.1128/AAC.00545-06
Pan, L. J., Wan, J. J., Huang, Y. F., and Li, X. C. (2013). Study on analysis of Michelia Flower and Michelia leaf oil by GC/MS and their application in Fragrances, (in Chinese). Flav. Frag. Cosmet. 2, 1–6.
Pimenta, R. S., Silva, J. F., Buyer, J. S., and Janisiewicz, W. J. (2012). Endophytic fungi from plums (Prunus domestica) and their antifungal activity against Monilinia fructicola. J. Food Prot. 75, 1883–1889. doi: 10.4315/0362-028X.JFP-12-156
Randa, Z., Zahoor, U. H., Roda, A. T., Quirico, M., and Samir, J. (2019). In-vitro application of a Qatari Burkholderia cepacia strain (QBC03) in the biocontrol of Mycotoxigenic fungi and in the reduction of ochratoxin a biosynthesis by Aspergillus carbonarius. Toxins 11:700. doi: 10.3390/toxins11120700
Roxane, R., Amy, N., and Tanya, A. (2018). Transcriptome alteration in Phytophthora infestans in response to phenazine-1-carboxylic acid production by Pseudomonas fluorescens strain LBUM223. BMC Genomics 19:474. doi: 10.1186/s12864-018-4852-1
Shang, J., Liu, B. L., and Xu, Z. (2020). Efficacy of Trichoderma asperellum TC01 against anthracnose and growth promotion of Camellia sinensis seedlings. Biol. Control 143:104205. doi: 10.1016/j.biocontrol.2020.104205
Sharifah, F. S., Lilia, C. C., Elvis, T. C., Fong, Y. C., Peter, M. M., Eladl, G. E., et al. (2019). Soil bacterial diffusible and volatile organic compounds inhibit Phytophthora capsica and promote plant growth. Sci. Total Environ. 692, 267–280. doi: 10.1016/j.scitotenv.2019.07.061
Sharifi, R., and Ryu, C. M. (2018). Revisiting bacterial volatile-mediated plant growth promotion: lessons from the past and objectives for the future. Ann. Bot. 122, 349–358. doi: 10.1093/aob/mcy108
Song, F. X., Wu, X. Q., and Zhao, Q. (2017). Antagonism of plant growth-promoting bacteria Rahnella aquatilis JZ-GX1 to canker in poplar, (in Chinese). J. Nanjing Forest. Univ. 41, 42–48. doi: 10.3969/j.issn.1000-2006.201603054
Su, C. L., Kang, H., Mei, Z. D., Yang, S. Y., Liu, X. M., Pu, J. J., et al. (2018). Expression analysis of pathogenic genes during the infection of Colletotrichum gloeosporioides to mango, (in Chinese). Biotechnol. Bull. 34, 182–186. doi: 10.13560/j.cnki.biotech.bull.1985.2018-0295
Suwannarach, N., Bussaban, B., Nuangmek, W., Pithakpol, W., Jirawattanakul, B., Matsui, K., et al. (2016). Evaluation of Muscodor suthepensis strain CMU-Cib462 as a postharvest biofumigant for tangerine fruit rot caused by Penicillium digitatum. J. Sci. Food Agric. 96, 339–345. doi: 10.1002/jsfa.7099
Tagele, S. B., Lee, H. G., Kim, S. W., and Lee, Y. S. (2019). Phenazine and 1-undecene producing Pseudomonas chlororaphis subsp. aurantiaca Strain KNU17Pc1 for growth promotion and disease suppression in Korean maize cultivars. J. Microbiol. Biotechnol. 29, 66–78. doi: 10.4014/jmb.1808.08026
Tang, L. H., Mo, J. Y., Guo, T. X., Huang, S. P., Li, Q. L., Ning, P., et al. (2019). In vitro antifungal activity of dimethyl trisulfide against Colletotrichum gloeosporioides from mango. World J. Microbiol. Biotechnol. 36:4. doi: 10.1007/s11274-019-2781-z
Upadhyay, S., Torres, G., and Lin, X. R. (2013). Laccases involved in 1,8-Dihydroxynaphthalene melanin biosynthesis in Aspergillus fumigatus are regulated by developmental factors and copper homeostasis. Eukaryot. Cell 12, 1641–1652. doi: 10.1128/EC.00217-13
Vitro, R., Manas, P., and Alvarez, I. (2005). Membrane damage and microbial inactivation by chlorine in the absence and presence of a chlorine-demanding substrate. Appl. Environ. Microbiol. 71, 5022–5028. doi: 10.1128/AEM.71.9.5022-5028.2005
Wan, M., Li, G., Zhang, J., Jiang, D., and Huang, H. C. (2008). Effect of volatile substances of Streptomyces platensis F-1 on control of plant fungal diseases. Biol. Control 46, 552–559. doi: 10.1016/j.biocontrol.2008.05.015
Wang, J., Cao, J. M., Chen, D. X., Qiu, J., Wang, X. Q., Feng, C., et al. (2018). Antimicrobial effect and components analysis of volatile organic compounds from Bacillus pumilus AR03, (in Chinese). Sci. Agric. Sin. 51, 1908–1919. doi: 10.3864/j.issn.0578-1752.2018.10.010
Wang, Q. H., Fan, K., and Li, D. W. (2019). Identification, virulence and fungicide sensitivity of Colletotrichum gloeosporioides s.s. responsible for walnut anthracnose disease in China. Plant Dis. 104, 1358–1368. doi: 10.1094/PDIS-12-19-2569-RE
Wu, S. Y., Zhen, C. Y., Wang, K., and Gao, H. Y. (2019). Effects of Bacillus Subtilis CF-3 VOCs combined with heat treatment on the control of Monilinia fructicola in peaches and Colletotrichum gloeosporioides in litchi fruit. J. Food Sci. 84, 3418–3428. doi: 10.1111/1750-3841.14949
Xu, X. B., Lei, H. H., Ma, X. Y., Lai, T. F., Song, H. M., Shi, X. Q., et al. (2017). Antifungal activity of 1-methylcyclopropene (1-MCP) against anthracnose (Colletotrichum gloeosporioides) in postharvest mango fruit and its possible mechanisms of action. Int. J. Food Microbiol. 241, 1–6. doi: 10.1016/j.ijfoodmicro.2016.10.002
Yin, D. C., Deng, X., Ilan, C. H. E. T., and Song, R. Q. (2014). Inhibitory effect and mechanism of Trichoderma virens T43 on four important forest pathogens, (in Chinese). Chin. J. Ecol. 33, 1911–1919. doi: 10.13292/j.1000-4890.20140429.009
Yuan, L. F., Li, L., Zheng, F., Shi, Y. X., Xie, X. W., Chai, A., et al. (2019). The complete genome sequence of Rahnella aquatilis ZF7 reveals potential beneficial properties and stress tolerance capabilities. Arch. Microbiol. 202, 483–499. doi: 10.1007/s00203-019-01758-1
Zhang, C. H. (2015). Study on the Inhibiting of Volites Produced by Streptomyces Alboflavus TD-1 on Botrytis Cinerea: (in Chinese). Ph.D. thesis, Tianjin University of Science &Technology, Tianjin.
Zhang, P. P. (2013). Effects of Some Plant Growth-Promotion Rhizobacteria Volites on Arabidopsis and Antagonistic Properties: (in Chinese). Ph.D. thesis, Shandong Agricultural University, Taian.
Zhang, Y., Li, T. J., Liu, Y. F., Li, X. Y., Zhang, C. M., Feng, Z. Z., et al. (2019). Volatile organic compounds produced by Pseudomonas chlororaphis subsp. aureofaciens SPS-41 as biological fumigants to control Ceratocystis fimbriata in postharvest sweet potato. J. Agric. Food Chem. 67, 3702–3710. doi: 10.1021/acs.jafc.9b00289
Keywords: Rahnella aquatilis, VOCs, Colletotrichum gloeosporioides, mycelial growth, spore germination
Citation: Kong W-L, Rui L, Ni H and Wu X-Q (2020) Antifungal Effects of Volatile Organic Compounds Produced by Rahnella aquatilis JZ-GX1 Against Colletotrichum gloeosporioides in Liriodendron chinense × tulipifera. Front. Microbiol. 11:1114. doi: 10.3389/fmicb.2020.01114
Received: 12 March 2020; Accepted: 04 May 2020;
Published: 28 May 2020.
Edited by:
Siu Mui Tsai, University of São Paulo, BrazilReviewed by:
Jianqiao Xu, Sun Yat-sen University, ChinaCarsten T. Muller, Cardiff University, United Kingdom
Copyright © 2020 Kong, Rui, Ni and Wu. This is an open-access article distributed under the terms of the Creative Commons Attribution License (CC BY). The use, distribution or reproduction in other forums is permitted, provided the original author(s) and the copyright owner(s) are credited and that the original publication in this journal is cited, in accordance with accepted academic practice. No use, distribution or reproduction is permitted which does not comply with these terms.
*Correspondence: Xiao-Qin Wu, xqwu@njfu.edu.cn; xqwu_njfu@163.com