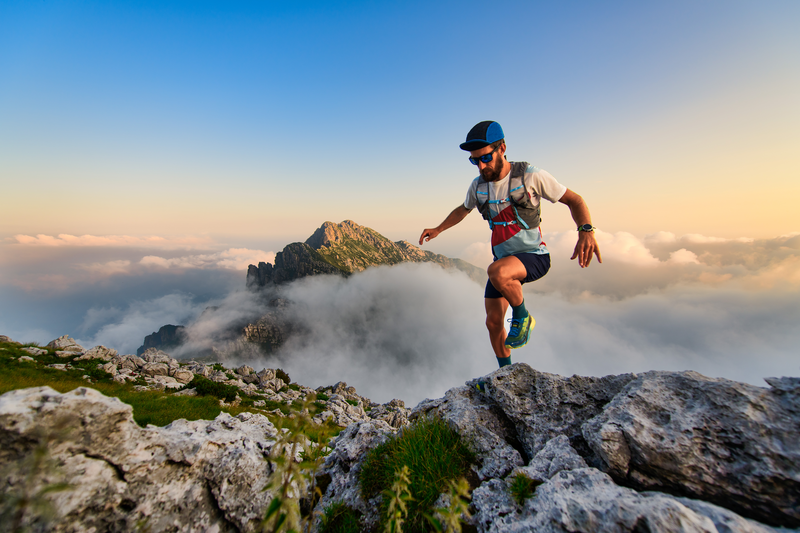
94% of researchers rate our articles as excellent or good
Learn more about the work of our research integrity team to safeguard the quality of each article we publish.
Find out more
ORIGINAL RESEARCH article
Front. Microbiol. , 03 June 2020
Sec. Infectious Agents and Disease
Volume 11 - 2020 | https://doi.org/10.3389/fmicb.2020.00998
The primary reason for skin graft failure and the mortality of burn wound patients, particularly those in burn intensive care centers, is bacterial infection. Several animal models exist to study burn wound pathogens. The most commonly used model is the mouse, which can be used to study virulence determinants and pathogenicity of a wide range of clinically relevant burn wound pathogens. However, animal models of burn wound pathogenicity are governed by strict ethical guidelines and hindered by high levels of animal suffering and the high level of training that is required to achieve consistent reproducible results. In this study, we describe for the first time an invertebrate model of burn trauma and concomitant wound infection. We demonstrate that this model recapitulates many of the hallmarks of burn trauma and wound infection seen in mammalian models and in human patients. We outline how this model can be used to discriminate between high and low pathogenicity strains of two of the most common burn wound colonizers Pseudomonas aeruginosa and Staphylococcus aureus, and multi-drug resistant Acinetobacter baumannii. This model is less ethically challenging than traditional vertebrate burn wound models and has the capacity to enable experiments such as high throughput screening of both anti-infective compounds and genetic mutant libraries.
Burn wound infection is one of the main clinical complications associated with burn wound care and is a leading cause of mortality among burn wound patients (Branski et al., 2009; Calum et al., 2017; Akers et al., 2019). Burn wound infections are also associated with autograft failure and prolonged treatment regimes, placing a significant burden on global health care systems. Specific nosocomial pathogens have established the burn unit as a particular niche in which they thrive; this is seen with clonal outbreaks occurring in burn units around the world. The primary burn wound pathogens include Pseudomonas aeruginosa, Acinetobacter baumannii, and Staphylococcus aureus (Branski et al., 2009; Azzopardi et al., 2011; Fransen et al., 2016; Amissah et al., 2017). Due to the complexity of the damage induced by thermal injuries, the insights offered by in vitro or ex vivo models are limited (Abdullahi et al., 2014). This has led to the development of different animal models to study burn wound infections. These models primarily use mammals such as mice, rats, dogs, and pigs. Murine models have become well established as the principal model to study burn wound infections (Everett et al., 2017; Lenzmeier et al., 2019). These murine models can offer robust insights into the host/pathogen processes involved in burn wound colonization and can play a key role in the validation of novel therapeutic strategies. However, they are governed by strict ethical guidelines, associated with high levels of suffering and are limited by the numbers of animals that can be used in a given study. Another complication with mouse burn models is that mice are classified as “loose-skin animals,” meaning that they do not have a similar skin structure to humans and the process of wound healing is different. The process of burn healing in mice is contraction, whereas in humans, it is granulation and re-epithelization (Wong et al., 2011; Abdullahi et al., 2014). The porcine model has emerged as a viable alternative to the mouse model particularly when studying wound healing as there is considerable similarity of skin structure, immune response, and healing pattern (re-epithelization) between pigs and humans (Abdullahi et al., 2014; Roy et al., 2016; Tsai D. M. et al., 2016). However, studies are limited to eight to nine experimental animals, due to high maintenance costs and strict ethical procedures, which limits statistical power and infection output kinetics. Although mammalian models have indisputable advantages, they are ethically challenging and not amenable to large cohort studies or drug discovery strategies.
Galleria mellonella, larvae of the greater wax moth, have become an increasingly popular non-mammalian infection model (Andrea et al., 2019). This is largely due to the exclusion of invertebrates from the Animals (Scientific Procedures) Act 1986, simplifying the ethical approval for research. G. mellonella are now well established as a powerful model to study pathogenicity in a range of bacterial and fungal pathogens (Fedhila et al., 2010; Tsai C. J. et al., 2016; McCarthy et al., 2017a,b). In comparison to other mammalian models, G. mellonella models of infection have a number of distinct advantages: the larvae are easy to use without special equipment, are inexpensive, and can be maintained at a range of temperatures including 37°C. Larvae are also readily available from a range of sources including facilities where they are reared under controlled conditions (genotype, age, weight, diet) specifically for scientific use. G. mellonella also have a similar innate immunity to mammalian models with primitive immune cells called hemocytes, which are capable of phagocytosis and producing a range of comparable innate immune effectors (Lange et al., 2018). In this study, we outline for the first time a G. mellonella burn wound model that can be used to study burn trauma and wound infection.
Pseudomonas aeruginosa (PA14, PA14ΔesxA), Staphylococcus epidermidis ATCC 12228 (American Tissue Culture Collection), methicillin resistant S. aureus (MRSA, National Collection of Type Cultures 12493), and A. baumannii (AB5075) were used. Single colonies were inoculated in lysogeny broth (LB) and incubated at 37°C for 16–18 h at 180 r/min before the experiment.
Galleria mellonella were sourced from Biosystems Technology (Exeter, United Kingdom) or LiveFood UK Ltd. (Somerset, United Kingdom). The animals were at a stage in their life cycle where they do not need to be fed. They are stored at 4°C. Prior to use, larvae were sorted into Petri dishes lined with Whatman filter paper (Fisher, United Kingdom), 10 larvae per plate and stored at 4°C until use. The larval bodies were sterilized with 70% ethanol. The burn was induced with a heated steel instrument to achieve a burn consistent burn area of approximately 2 mm2. The metal instruments used were of low thermal conductivity and specifically selected to generate reproducible burn wounds. The instrument was heated until it was red/white-hot and applied to the middle section of the back of larvae for a fixed time of 4 s (Figure 1). This location was chosen so the wound could be easily visualized without having to physically manipulate the larvae. For total burn surface area experiments, the number of times the burn instrument was applied to the larval back depended on the size of burn wound desired. For infection studies, immediately post burn, the wound was inoculated with 10 μl of 1:10 dilution of bacterial over-night culture. For assays using A. baumannii, a single colony was applied to the wound. Any larvae who showed distress or leakage of hemolymph after the burn process were immediately euthanized by incubating at -20°C for 20 min to minimize suffering. Post wounding, larvae were incubated at 37°C and monitored over the course of 72–120 h (3–5 days). Mortality was recorded by complete melanization of the larval body and complete loss of motility (McCarthy et al., 2017b).
Figure 1. Schematic outline of burn procedure: (1) Heating up the burn device in the middle of the flame of a Bunsen burner until the metal element is red/white-hot. (2) Cooling down the burn device near the flame for 4 s. (3) Applying the hot metal element to the middle segments of Galleria mellonella back for 4 s and slowly lifting it. (4) Applying 10 μl of 1:10 dilution of over-night bacterial culture onto the fresh wound. (5) Larvae are incubated at 37°C for 120 h and monitored every 24 h. The darkness of Galleria signifies the intensity of infection (Wojda, 2017). Created with BioRender.
Larvae were burned three times as previously described. 15 min post burn wound, larvae were injected in the right-hand side of the first set of prolegs with 100 μl of sterile phosphate buffered saline (PBS) solution using Micro Fine Insulin syringe 0.5 ml 29 G × 12.7 mm. Larvae were incubated at 37°C and monitored over the course of 72 h (3 days). Mortality was recorded by complete melanization of the larval body and complete loss of motility.
To determine bacterial dissemination from the burn wound site into the circulation (hemolymph), immediately after death, 10 μl of hemolymph was aspirated from larvae. This hemolymph was serially diluted in PBS solution to a dilution of 10–6 and then 20 μl from each dilution was plated on Pseudomonas Isolation Agar (Thermo Fisher®). Plates were incubated at 37°C overnight and colony forming units enumerated.
Data was generated from a minimum of 10 larvae per condition per biological replicate with between two to four biological replicates per experiment. Kaplan–Meier survival curves were used to visualize data and a Log Rank test performed with p < 0.05 considered significant. For CFU counting assays, a Mann–Whitney U-test was performed with p < 0.05 considered significant.
Classical burn wound studies in mammalian models typically use a heated metal instrument to achieve consistency in burn area [TBSA% (percentage total body surface area)] and burn intensity (superficial versus deep) (Tsai D. M. et al., 2016). To determine if G. mellonella were a suitable model to study burn trauma and burn wound infection, a protocol was developed to mimic mammalian models and create a localized area of thermal tissue injury, without causing the excessive loss of hemolymph, the larval equivalent of blood, from the burn wound site (Figure 1). The burn injury procedure is well tolerated by larvae, as in the control group (1x Burn only), the percentage of live larvae was >87% after 52 h at 37°C (Figure 2A), highlighting this invertebrate as a potential model of burn trauma. In human burn treatment, it is recognized that the increase in total burn surface is directly linked to a decrease in survival probability (Kraft et al., 2012). To determine if this paradigm is also true in the G. mellonella model, we tested the impact of increased burn wound surface area on survival. Sequentially increasing the total burn surface area was shown to lead to a corresponding decrease in survival with less than 20% of larvae with double the burn surface area surviving for 52 h (Log Rank, p < 0.001), whereas 0% of larvae with triple the burn surface survived to this time point (Log Rank, p < 0.001) (Figure 2A).
Figure 2. Hallmarks of burn trauma: (A) Total burn surface area (TBSA) correlates with survival: it is well established in burn patients that the larger the TBSA, the greater the risk of mortality. We demonstrate that this central dogma can be recapitulated in the G. mellonella burn wound model. 1x = 2 mm2 Burn, 2x = 2 × 2 mm2 Burn, 3x = 3 × 2 mm2 Burns. 1x vs 2x, Log Rank p < 0.001, 1x vs 3x Log Rank p < 0.001, 2x vs 3x Log Rank p < 0.001. n ≥ 30 per condition. (B) Fluid resuscitation: administering larvae with fluid after burn trauma can significantly increase their survival prognosis Log Rank p < 0.001. n ≥ 30 per condition.
It is widely recognized that treating severe fluid loss is the greatest problem faced by clinicians when dealing with major burn injuries. Therefore, fluid resuscitation is one of the central cornerstones of modern burn treatment regimens (Baxter and Shires, 1968; Baxter, 1979; Haberal et al., 2010). Early observations in our assay suggested that the larvae that did succumb to burn trauma, did so due to severe fluid loss. This suggested that the fluid loss paradigm may hold true in the G. mellonella model. To test this, 15 min after receiving a fatal burn wound, larvae were injected with 100 μl PBS and their survival was monitored. There was a significant difference in survival between larvae that received fluid resuscitation and untreated larvae (Log Rank, p < 0.001), confirming that severe fluid loss is at least partly responsible for mortality in this model (Figure 2B). This event mimics the fluid loss in human burn injury.
Pseudomonas aeruginosa is the most common Gram-negative burn wound pathogen and despite the remarkable advances in human burn patient care over the last century, patients who become infected with P. aeruginosa face a mortality rate of up to 80% (Tredget et al., 2004). To determine if P. aeruginosa could colonize larval burn wounds, larvae were topically infected with P. aeruginosa PA14, a known highly pathogenic burn wound isolate (Mikkelsen et al., 2011; McCarthy et al., 2014). This inoculation method mimics the exposure route that has been shown to occur in both the environment and burn clinics (Kolmos et al., 1993; Calum et al., 2017). Larvae infected with P. aeruginosa PA14 displayed high levels of mortality with only 10% survival observed after 120 h (Log Rank, p < 0.001) (Figures 3A,B and Supplementary Figure S1). The observed infection progression cycle in this model closely matches what is seen in mammalian models and human cases, with local tissue necrosis, biofilm formation, dissemination from the burn wound site, bacteremia, burn sepsis, and ultimately mortality.
Figure 3. Visualization of burn wound pathogenicity over time: (A) Following the burn wound procedure and subsequent infection, the tissue at site of the burn will turn necrotic and evidence of bacterial dissemination is seen through the melanization of the larvae and loss of motility. Representative images of each stage shown. (B) Percentage survival of larvae after infecting the burn site with PA14ΔexsA which has an inactive T3SS and Wild Type PA14 against the uninfected control group burn. PA14 vs Burn Only Log Rank p < 0.001, Burn Only vs PA14ΔexsA Log Rank p < 0.001, PA14ΔexsA vs Burn Only Log Rank p < 0.001. n ≥ 40 per condition. (C) Hemolymph (larval blood) was aspirated and the bacterial numbers enumerated by plating serial dilutions n = 25 larvae, with SEM. Mann–Whitney U-test ***p < 0.001.
To verify that this observed mortality was due to bacterial pathogenicity, a mutant strain of PA14 was used in which the master regulator of the Type 3 Secretion System (T3SS), exsA was deleted. Previous studies have demonstrated that inactivating the T3SS significantly diminishes the capacity of P. aeruginosa to infect murine burn wounds (Holder et al., 2001). Exposure of PA14ΔexsA to the larval burn wound resulted in significantly lower levels of mortality compared to the Wild Type PA14 (Log Rank, p < 0.001) (Figure 3B and Supplementary Figure S1), replicating the scenario seen in the mouse burn wound model (Holder et al., 2001).
To demonstrate bacterial dissemination from the burn wound site and bacteremia, hemolymph, the larval equivalent of blood, was aspirated from larvae immediately post-mortem. P. aeruginosa was recovered from the hemolymph of these larvae in 92% of cases whereas in the burn only control, P. aeruginosa was never recovered (Figure 3C). This further demonstrates that the infection progression cycle mimics what is observed in mammalian burn wound models and human patients. To determine if this model could be used to study other Gram-negative burn wound pathogens such as A. baumannii, the assay was repeated using a highly virulent multidrug resistant strain of A. baumannii AB5075 (Jacobs et al., 2014). As expected, larvae infected with A. baumannii AB5075 demonstrated a significant drop in survival compared to burn only controls (Log Rank, p < 0.001) (Figure 4A).
Figure 4. Burn wound pathogenicity: (A) Percentage survival rate of larvae infected with A. baumannii AB5075 and the burn only control. A. baumannii AB5075 vs Burn Only Log Rank p < 0.001. (B) Percentage survival rate of larvae infected with MRSA and the non-burn wound pathogen S. epidermidis. MRSA vs Burn Only Log Rank p < 0.001, MRSA vs S. epidermidis Log Rank p < 0.001, S. epidermidis vs Burn Only Log Rank p < 0.14. n ≥ 30 per condition.
To further explore the application of this model to the study of burn wound infections, we tested the capacity of a common Gram-positive pathogen MRSA to cause mortality in this model (Nishiguchi et al., 2017). MRSA is known to be a common burn wound pathogen and is frequently identified in burn care centers (Amissah et al., 2017). Although not as virulent as P. aeruginosa, MRSA could cause a significant decrease in burn wound survival compared to the uninfected controls with only 34% survival after 120 h (Log Rank, p < 0.001) (Figure 4B). To further interrogate the capacity of Staphylococcus spp. to cause mortality in this model and demonstrate the role of biofilm formation in infection progression, a strain of S. epidermidis (ATCC 12228) that does not readily form biofilm and has not previously been reported to cause burn wound infections was tested. Exposure of this strain to the burn site did not result in a significant increase in mortality compared to the uninfected burn wound control (Figure 4B).
Burn wounds are complex microenvironments where colonization by bacterial pathogens such as P. aeruginosa, A. baumannii, and S. aureus represents the primary clinical complication. Infection is the leading cause of mortality among burn wound patients (Branski et al., 2009; Calum et al., 2017). Burn wound infections are also associated with increased autograft failure and prolonged treatment regimes. This places a significant burden on burn treatment centers. The problem of burn wound infection is exacerbated by the emergence of multidrug resistance (MDR) among bacterial isolates, commonly found in the hospital setting, with one study reporting that 73% of septic deaths in a pediatric burn center were due to MDR organisms (Williams et al., 2009). Current in vivo models to study burn trauma and infection are exclusively based on mammalian models such as mice, rats, and pigs. The ability to gain in vivo insights into burn wound infection or test novel therapeutic options is hampered by the difficulty in gaining ethical approval for these mammalian models. This is due to the severity of the procedures involved and the limited quantities of data that can be realistically retrieved from these experiments. We describe for the first time an invertebrate model of burn trauma and infection. We demonstrate that this model closely mimics the hallmarks of burn trauma seen in patients such as a decreased survival prognosis with increased burn surface area and the importance of rapid fluid resuscitation (Figures 2A,B), hallmarks which cannot be reproduced in in vitro burn wound models (Figures 2A,B).
Substantial improvements in our understanding of burn trauma and infection and the development of effective treatment regimens have had a dramatic impact on patient survival. Despite this, the mortality rate for patients who become infected with P. aeruginosa has been unchanged in the last 25 years (Tredget et al., 2004; Calum et al., 2017). P. aeruginosa burn wound infections are notoriously difficult to treat and while new insights are consistently emerging into the molecular mechanisms that control P. aeruginosa burn wound colonization (Gonzalez et al., 2016; Everett et al., 2017), the lack of unrestricted in vivo models has stymied the progress in understanding this pathogen. We show that P. aeruginosa PA14 can colonize G. mellonella burn wounds and that this infection progression cycle is remarkably similar to infection progression in patients and mammalian models (Figures 3A,B). The transition from burn wound colonization to blood stream colonization is a critical event, which significantly reduces the probability of survival (Drake and Montie, 1988; Everett et al., 2017). We demonstrate that this model can also be used to study this event, as P. aeruginosa can be recovered from larval hemolymph to indicate that the bacteria have disseminated from the wound site (Figure 3C). One of the primary virulence mechanisms utilized by P. aeruginosa to establish infection is the T3SS. This system is capable of injecting a range of potent toxins, ExoS, ExoT, ExoY, and ExoU, into eukaryotic cells. Strains of P. aeruginosa with a defective T3SS are severely attenuated for virulence in a wide range of virulence models including pneumonia, neutropenic, corneal, and burn wound infection models (Finck-Barbançon et al., 1997; Sawa et al., 1998; Holder et al., 2001; Koh et al., 2005; Lee et al., 2005; Dortet et al., 2018; Williams McMackin et al., 2019). Critically, all of these infection models are mammalian models, we demonstrate for the first time that burn wound pathogenesis in the G. mellonella infection model is also severely attenuated in a strain with a defective T3SS (Figure 3B).
We demonstrate that this model can also be used to study A. baumannii, one of the leading proponents of the emergent antibiotic resistance crisis and a leading cause of burn wound infections. This pathogen can thrive in the hospital environment due to its remarkable ability to survive desiccation. Uniquely, A. baumannii can survive on inanimate objects such as hospital beds and ventilators without nutrients or water for weeks to months, which promotes transmission throughout health care environments (Farrow et al., 2018; Harding et al., 2018; Lima et al., 2018). We have demonstrated the versatility of this model by showing that it is suitable to also study Gram-positive burn wound pathogens such as MRSA.
Biofilm formation plays a central role in the recalcitrance of burn wound infections, in wound dressing failure and burn wound sepsis (Kennedy et al., 2010; Brandenburg et al., 2019; Moyano et al., 2019). This model can also be used to study biofilm formation at the burn wound site and determine the pathogenicity of high biofilm forming strains (Figure 4B). This invertebrate model could also be used to address the complex question of what represents a burn wound infection and what represents the natural microbial flora of a burn wound. It also has the potential to act as a robust drug discovery platform, by facilitating in vivo testing at a throughput level that cannot be achieved in traditional mammalian models. This invertebrate burn wound model, while not a complete replacement for mammalian models of burn trauma and wound infection, does offer a viable alternative that is low cost, less ethically challenging and can facilitate higher power statistical analysis.
The datasets generated for this study are available on request to the corresponding author.
RM, DW, FS, and HA conceived the study. EM, YS, and RM performed the experiments and data analysis. EM and RM wrote the manuscript with inputs from all other authors. EM and YS have contributed equally to the work.
EM and RM were supported by the Brunel Research Innovation and Enterprise Fund (2018-11143), the British Society for Antimicrobial Chemotherapy (BSAC-2018-0095), Innovate UK (37800), FRAME, and the Young European Research University Network. YS, HA, and DW thank the financial support from the Seed Award in Science (210122/Z/18/Z) granted by the Wellcome Trust.
The authors declare that the research was conducted in the absence of any commercial or financial relationships that could be construed as a potential conflict of interest.
We would like to thank Elentina Gjoni for technical assistance in assay development.
The Supplementary Material for this article can be found online at: https://www.frontiersin.org/articles/10.3389/fmicb.2020.00998/full#supplementary-material
Abdullahi, A., Amini-Nik, S., and Jeschke, M. G. (2014). Animal models in burn research. Cell. Mol. Life Sci. 71, 3241–3255. doi: 10.1007/s00018-014-1612-5
Akers, K. S., Schlotman, T., Mangum, L. C., Garcia, G., Wagner, A., Seiler, B., et al. (2019) 653. Diagnosis of burn sepsis using the FcMBL ELISA: A pilot study in critically Ill burn patients. Open Forum Infect. Dis. 6(Supplement_2), S300. doi: 10.1093/ofid/ofz360.721
Amissah, N. A., Buultjens, A. H., Ablordey, A., van Dam, L., Opoku-Ware, A., Baines, S. L., et al. (2017). Methicillin resistant Staphylococcus aureus transmission in a ghanaian burn unit: the importance of active surveillance in resource-limited settings. Front. Microbiol. 8:1906. doi: 10.3389/fmicb.2017.01906
Andrea, A., Krogfelt, K. A., and Jenssen, H. (2019). Methods and challenges of using the greater wax moth (Galleria mellonella) as a model organism in antimicrobial compound discovery. Microorganisms 7:85. doi: 10.3390/microorganisms7030085
Azzopardi, E. A., Azzopardi, S. M., Boyce, D. E., and Dickson, W. A. (2011). Emerging gram-negative infections in burn wounds. J. Burn Care Res. 32, 570–576. doi: 10.1097/BCR.0b013e31822ac7e6
Baxter, C. (1979). Fluid resuscitation, burn percentage, and physiologic age. J. Trauma 19(11 Suppl.), 864.
Baxter, C. R., and Shires, T. (1968). Physiological response to crystalloid resuscitation of severe burns. Ann. N. Y. Acad. Sci. 150, 874–894. doi: 10.1111/j.1749-6632.1968.tb14738.x
Brandenburg, K. S., Weaver, A. J., Karna, S. L. R., You, T., Chen, P., Stryk, S. V., et al. (2019). Formation of Pseudomonas aeruginosa biofilms in full-thickness scald burn wounds in rats. Sci. Rep. 9:13627. doi: 10.1038/s41598-019-50003-8
Branski, L. K., Al-Mousawi, A., Rivero, H., Jeschke, M. G., Sanford, A. P., and Herndon, D. N. (2009). Emerging infections in burns. Surg. Infect. 10, 389–397. doi: 10.1089/sur.2009.024
Calum, H., Høiby, N., and Moser, C. (2017). Mouse model of burn wound and infection: thermal (Hot Air) Lesion-Induced Immunosuppression. Curr. Protoc. Mouse Biol. 7, 77–87. doi: 10.1002/cpmo.25
Dortet, L., Lombardi, C., Cretin, F., Dessen, A., and Filloux, A. (2018). Pore-forming activity of the Pseudomonas aeruginosa type III secretion system translocon alters the host epigenome. Nat. Microbiol. 3:378.
Drake, D., and Montie, T. C. (1988). Flagella, motility and invasive virulence of Pseudomonas aeruginosa. J. Gen. Microbiol. 134, 43–52. doi: 10.1099/00221287-134-1-43
Everett, J., Turner, K., Cai, Q., Gordon, V., Whiteley, M., and Rumbaugh, K. (2017). Arginine is a critical substrate for the pathogenesis of Pseudomonas aeruginosa in burn wound infections. mBio 8:2160. doi: 10.1128/mBio.02160-16
Farrow, J. M. III, Wells, G., and Pesci, E. C. (2018). Desiccation tolerance in Acinetobacter baumannii is mediated by the two-component response regulator BfmR. PLoS One 13:e0205638. doi: 10.1371/journal.pone.0205638
Fedhila, S., Buisson, C., Dussurget, O., Serror, P., Glomski, I. J., Liehl, P., et al. (2010). Comparative analysis of the virulence of invertebrate and mammalian pathogenic bacteria in the oral insect infection model Galleria mellonella. J. Invertebr. Pathol. 103, 24–29. doi: 10.1016/j.jip.2009.09.005
Finck-Barbançon, V., Goranson, J., Zhu, L., Sawa, T., Wiener-Kronish, J. P., Fleiszig, S. M. J., et al. (1997). ExoU expression by Pseudomonas aeruginosa correlates with acute cytotoxicity and epithelial injury. Mol. Microbiol. 25, 547–557. doi: 10.1046/j.1365-2958.1997.4891851.x
Fransen, J., Huss, F. R. M., Nilsson, L. E., Rydell, U., Sjöberg, F., Hanberger, H., et al. (2016). Surveillance of antibiotic susceptibility in a Swedish burn center 1994-2012. Burns 42:1295.
Gonzalez, M. R., Fleuchot, B., Lauciello, L., Jafari, P., Applegate, L. A., Raffoul, W., et al. (2016). Effect of human burn wound exudate on Pseudomonas aeruginosa virulence. mSphere 1:111. doi: 10.1128/mSphere.00111-15
Haberal, M., Sakallioglu Abali, A. E., and Karakayali, H. (2010). Fluid management in major burn injuries. Indian J. Plast. Surg. 43(Suppl.), S29–S36. doi: 10.4103/0970-0358.70715
Harding, C. M., Hennon, S. W., and Feldman, M. F. (2018). Uncovering the mechanisms of Acinetobacter baumannii virulence. Nat. Rev. Microbiol. 16, 91–102. doi: 10.1038/nrmicro.2017.148
Holder, I. A., Neely, A. N., and Frank, D. W. (2001). Type III secretion/intoxication system important in virulence of Pseudomonas aeruginosa infections in burns. Burns 27, 129–130. doi: 10.1016/S0305-4179(00)00142-X
Jacobs, A. C., Thompson, M. G., Black, C. C., Kessler, J. L., Clark, L. P., McQueary, C. N., et al. (2014). AB5075, a highly virulent isolate of Acinetobacter baumannii, as a model strain for the evaluation of pathogenesis and antimicrobial treatments. mBio 5:e01076. doi: 10.1128/mBio.01076-14
Kennedy, P., Brammah, S., and Wills, E. (2010). Burns, biofilm and a new appraisal of burn wound sepsis. Burns 36, 49–56. doi: 10.1016/j.burns.2009.02.017
Koh, A. Y., Priebe, G. P., and Pier, G. B. (2005). Virulence of Pseudomonas aeruginosa in a murine model of gastrointestinal colonization and dissemination in neutropenia. Infect. Immun. 73, 2262–2272. doi: 10.1128/IAI.73.4.2262-2272.2005
Kolmos, H. J., Thuesen, B., Nielsen, S. V., Lohmann, M., Kristoffersen, K., and Rosdahl, V. T. (1993). Outbreak of infection in a burns unit due to Pseudomonas aeruginosa originating from contaminated tubing used for irrigation of patients. J. Hosp. Infect. 24, 11–21.
Kraft, R., Herndon, D. N., Al-Mousawi, A. M., Williams, F. N., and Finnerty, C. C. (2012). Burn size and survival probability in paediatric patients in modern burn care: a prospective observational cohort study. Lancet 379, 1013–1021. doi: 10.1016/S0140-6736(11)61345-7
Lange, A., Beier, S., Huson, D. H., Parusel, R., Iglauer, F., and Frick, J. (2018). Genome sequence of Galleria mellonella (greater wax moth). Genome Announc. 6:e1220-17. doi: 10.1128/genomeA.01220-17
Lee, V. T., Smith, R. S., Tümmler, B., and Lory, S. (2005). Activities of Pseudomonas aeruginosa effectors secreted by the type III secretion system in vitro and during infection. Infect. Immun. 73, 1695–1705. doi: 10.1128/IAI.73.3.1695-1705.2005
Lenzmeier, T. D., Mudaliar, N. S., Stanbro, J. A., Watters, C., Ahmad, A., Simons, M. P., et al. (2019). Application of Lactobacillus gasseri 63 AM supernatant to Pseudomonas aeruginosa- infected wounds prevents sepsis in murine models of thermal injury and dorsal excision. J. Med. Microbiol. 68, 1560–1572. doi: 10.1099/jmm.0.001066
Lima, W. G., Alves, M. C., Cruz, W. S., and Paiva, M. C. (2018). Chromosomally encoded and plasmid-mediated polymyxins resistance in Acinetobacter baumannii: a huge public health threat. Eur. J. Clin. Microbiol. Infect. Dis. 37, 1009–1019. doi: 10.1007/s10096-018-3223-9
McCarthy, R. R., Mazon-Moya, M. J., Moscoso, J. A., Hao, Y., Lam, J. S., Bordi, C., et al. (2017a). Cyclic-di-GMP regulates lipopolysaccharide modification and contributes to Pseudomonas aeruginosa immune evasion. Nat. Microbiol. 2:17027.
McCarthy, R. R., Mooij, M. J., Reen, F. J., Lesouhaitier, O., and OGara, F. (2014). A new regulator of pathogenicity (bvlR) is required for full virulence and tight microcolony formation in Pseudomonas aeruginosa. Microbiology 160, 1488–1500. doi: 10.1099/mic.0.075291-0
McCarthy, R. R., Valentini, M., and Filloux, A. (2017b). Contribution of Cyclic di-GMP in the control of type III and type VI secretion in Pseudomonas aeruginosa. Methods Mol. Biol. 1657, 213–224.
Mikkelsen, H., McMullan, R., and Filloux, A. (2011). The Pseudomonas aeruginosa reference strain PA14 displays increased virulence due to a mutation in ladS. PLoS One 6:e29113. doi: 10.1371/journal.pone.0029113
Moyano, A. J., Mas, C. R., Colque, C. A., and Smania, A. M. (2019). Dealing with biofilms of Pseudomonas aeruginosa and Staphylococcus aureus: in vitro evaluation of a novel aerosol formulation of silver sulfadiazine. Burns 46, 128–135. doi: 10.1016/j.burns.2019.07.027
Nishiguchi, T., Ito, I., Lee, J. O., Suzuki, S., Suzuki, F., and Kobayashi, M. (2017). Macrophage polarization and MRSA infection in burned mice. Immunol. Cell Biol. 95, 198–206. doi: 10.1038/icb.2016.84
Roy, D. C., Tomblyn, S., Isaac, K. M., Kowalczewski, C. J., Burmeister, D. M., Burnett, L. R., et al. (2016). Ciprofloxacin-loaded keratin hydrogels reduce infection and support healing in a porcine partial-thickness thermal burn: antibiotic-keratin gels for burn infection. Wound Repair Regen. 24, 657–668. doi: 10.1111/wrr.12449
Sawa, T., Ohara, M., Kurahashi, K., Twining, S. S., Frank, D. W., Doroques, D. B., et al. (1998). In vitro cellular toxicity predicts Pseudomonas aeruginosa virulence in lung infections. Infect. Immun. 66, 3242–3249. doi: 10.1128/IAI.66.7.3242-3249.1998
Tredget, E. E., Shankowsky, H. A., Rennie, R., Burrell, R. E., and Logsetty, S. (2004). Pseudomonas infections in the thermally injured patient. Burns. 30, 3–26. doi: 10.1016/j.burns.2003.08.007
Tsai, C. J., Loh, J. M. S., and Proft, T. (2016). Galleria mellonella infection models for the study of bacterial diseases and for antimicrobial drug testing. Virulence 7, 214–229. doi: 10.1080/21505594.2015.1135289
Tsai, D. M., Tracy, L. E., Lee, C. C. Y., Hackl, F., Kiwanuka, E., Minasian, R. A., et al. (2016). Full-thickness porcine burns infected with Staphylococcus aureus or Pseudomonas aeruginosa can be effectively treated with topical antibiotics. Wound Repair Regen. 24, 356–365. doi: 10.1111/wrr.12409
Williams, F. N., Herndon, D. N., Hawkins, H. K., Lee, J. O., Cox, R. A., Kulp, G. A., et al. (2009). The leading causes of death after burn injury in a single pediatric burn center. Crit. Care 13:R183. doi: 10.1186/cc8170
Williams McMackin, E. A., Djapgne, L., Corley, J. M., and Yahr, T. L. (2019). Fitting pieces into the puzzle of Pseudomonas aeruginosa type III secretion system gene expression. J. Bacteriol. 201, e209–e219. doi: 10.1128/JB.00209-19
Wojda, I. (2017). Immunity of the greater wax moth Galleria mellonella. Insect Sci. 24, 342–357. doi: 10.1111/1744-7917.12325
Keywords: burn, infection, Galleria mellonella, Pseudomonas aeruginosa, MRSA, Acinetobacter baumannii, biofilm
Citation: Maslova E, Shi Y, Sjöberg F, Azevedo HS, Wareham DW and McCarthy RR (2020) An Invertebrate Burn Wound Model That Recapitulates the Hallmarks of Burn Trauma and Infection Seen in Mammalian Models. Front. Microbiol. 11:998. doi: 10.3389/fmicb.2020.00998
Received: 17 January 2020; Accepted: 24 April 2020;
Published: 03 June 2020.
Edited by:
Giovanna Batoni, University of Pisa, ItalyReviewed by:
Liliana Scorzoni, São Paulo State University, BrazilCopyright © 2020 Maslova, Shi, Sjöberg, Azevedo, Wareham and McCarthy. This is an open-access article distributed under the terms of the Creative Commons Attribution License (CC BY). The use, distribution or reproduction in other forums is permitted, provided the original author(s) and the copyright owner(s) are credited and that the original publication in this journal is cited, in accordance with accepted academic practice. No use, distribution or reproduction is permitted which does not comply with these terms.
*Correspondence: Ronan R. McCarthy, cm9uYW4ubWNjYXJ0aHlAYnJ1bmVsLmFjLnVr
†These authors have contributed equally to this work
Disclaimer: All claims expressed in this article are solely those of the authors and do not necessarily represent those of their affiliated organizations, or those of the publisher, the editors and the reviewers. Any product that may be evaluated in this article or claim that may be made by its manufacturer is not guaranteed or endorsed by the publisher.
Research integrity at Frontiers
Learn more about the work of our research integrity team to safeguard the quality of each article we publish.