- 1Key Laboratory of Agricultural Microbiology of Heilongjiang Province, Northeast Agricultural University, Harbin, China
- 2State Key Laboratory of Phytochemistry and Plant Resources in West China, Kunming Institute of Botany, Chinese Academy of Sciences, Kunming, China
- 3State Key Laboratory for Biology of Plant Diseases and Insect Pests, Institute of Plant Protection, Chinese Academy of Agricultural Sciences, Beijing, China
Actinobacteria associated with insects represent one potentially rich source of novel natural products with antifungal activity. Here, we investigated the phylogenetic diversity and community composition of actinobacteria associated with ants using a combination of culture-dependent and -independent methods. Further, we assessed the antagonistic activity against phytopathogenic fungi and identified the secondary metabolites from isolates with bioactivity. A total of 416 actinobacterial isolates were obtained from three ant species (Camponotus japonicus, Lasius fuliginosus, and Lasius flavus) located in five nests. The largest amount of isolates were observed in the head samples. 16S rRNA gene sequencing showed that the isolates were diverse and belonged to ten genera within the phylum Actinobacteria, with Streptomyces and Micromonospora comprising the most abundant genera. High-throughput sequencing analyses revealed that the actinobacterial communities were more diverse and dominated by the families Nocardioidaceae, Nocardiaceae, Dermacoccaceae, Intrasporangiaceae, and Streptomycetaceae. In addition, 52.3% of the representative isolates had inhibitory properties against phytopathogenic fungi. Chemical analysis of one Streptomyces strain led to the discovery of two known compounds and one new compound. These results demonstrated that ant-derived actinobacteria represented an underexplored bioresource library of diverse and novel taxa that may be of potential interest in the discovery of new agroactive compounds.
Introduction
The phylum Actinobacteria consists of a wide range of Gram-positive bacteria with high guanine+cytosine (G+C) content. Members of the phylum are the most important sources of antimicrobials, and have generally been isolated from terrestrial and marine habitats (Qin et al., 2016; Lee et al., 2018). Recently, insect-associated actinobacteria have been intensely studied for their capacity to produce structurally novel natural products with antimicrobial properties. For example, dentigerumycin A, a new depsipeptide that contains unusual amino acids (β-hydroxyleucine, N-hydroxyalanine, piperazic acid and γ-hydroxypiperazic acid) and a pyran side chain, isolated from an symbiotic Pseudonocardia sp. associated with fungus-growing ant, Acromyrmex octospinosus, shows selective antifungal activity (Oh et al., 2009a). Selvamicin, a new polyene macrolide with antifungal activity, was produced by two Pseudonocardia isolates recovered from nests of fungus-growing ant (Acromyrmex dentigerum) (Van Arnam et al., 2016). Mycangimycin, a structurally novel polyene peroxide with pronounced antifungal and antimalarial activity, was secreted by a symbiotic Streptomyces sp. associated with the southern pine beetle (Dendroctonus frontalis) (Oh et al., 2009b). Further studies of the antinobacterial symbionts of the southern pine beetle led to the discovery of antifungals frontalamides A and B, two novel polycyclic tetramate macrolactams (Blodgett et al., 2010). Macrotermycins A and C, two novel glycosylated polyketide macrolactams with selective antifungal activity, were produced by Amycolatopsis sp. M39 isolated from the fungus-growing termite species Macrotermes natalensis (Beemelmanns et al., 2017). Sceliphrolactam, a novel polyene macrocyclic lactam with antifungal activity against amphotericin B-resistant Candida albicans, was isolated from a wasp-associated Streptomyces sp. (Oh et al., 2011). Further, a recent study assessed insect-associated Streptomyces strains as a source of new antimicrobials by application of genomics, metabolomics, and bioactivity assays. Their results showed that Streptomyces from insects harbored many of uncharacterized biosynthetic gene clusters which led to the discovery of new molecules, and exhibit notably greater activity against fungi than soil-derived Streptomyces (Chevrette et al., 2019). These findings highlighted that chemical studies of insect-associated actinomycetes would be an effective strategy for the discovery of new chemotypes with antimicrobial activity.
Insect-associated actinobacteria have been isolated from diverse insects, including fungus-growing ants, fungus-growing termites, southern pine beetle, dung beetle, beewolf digger wasps, honeybee and grasshoppers (Currie et al., 1999; Scott et al., 2008; Patil et al., 2010; Kroiss et al., 2010; Visser et al., 2012; Kim et al., 2013). Streptomyces and Pseudonocardia are the most predominant genera cultivated. Other genera, including Amycolatopsis, Nocardiopsis, Nocardia, Saccharothrix, Kitassatospora, and Propionicimonas, were also identified (Patil et al., 2010; Zucchi et al., 2011; Matarrita-Carranza et al., 2017; Van Arnam et al., 2018). With over five million species, insects spread to nearly all terrestrial niches on our planet, and host numerous chemically prolific bacteria (Stork et al., 2015; Van Arnam et al., 2018). However, there is still limited information related to the chemical ecology of insect-associated actinobacteria. Thus, insects can be regarded as a huge and underexplored reservoir for unusual microorganisms with antimicrobial potential.
Fungi are the oldest enemies of terrestrial organism. For example, the earliest terrestrial plants probably developed chemicals to counteract their assault (Swain, 1978). Ants are widespread in most terrestrial habitats. However, the damp underground nests in which they live were also suitable for fungal growth (Bailey, 1920), which leads to a high risk of fungal infestation for their larvae and food. To counteract these threats, they have developed a range of defensive strategies. For example, fungus-growing ants cultivate actinobacteria that produce antibiotic substances, which can protect the fungal gardens against the antagonistic fungus (Oh et al., 2009a; Carr et al., 2012). Further, Sánchez-Peña et al. (2008) found that antinobacteria associated with fungus-growing ants showed significant inhibitory activity against plant pathogenic fungi, which broadens the studies of antinobacteria insect associates to control economically important plant pathogens.
Ants are an very diverse group of insects with largely untapped antinobacterial communities (Matarrita-Carranza et al., 2017). Here, we investigated the diversity of actinobacteria from three ant species (Camponotus japonicas, Lasius fuliginosus, and Lasius flavus) using culture-dependent and culture-independent methods, assessed the anti-phytopathogenic fungal activity of cultured isolates, and identified the secondary metabolites produced by one Streptomyces strain with broad-spectrum antifungal activity.
Materials and Methods
Sample Collection
Ant colonies were collected from the campus of Northeast Agriculture University located in Harbin, Heilongjiang, north China (45°44′ N, 126°43′ E) in September 2015. Three ant species, including C. japonicas (Formicinae, Figure 1A), L. fuliginosus (Formicinae, Figure 1B) and L. flavus (Formicinae, Figure 1C), were sampled from five nests. C. japonicas colonies were caught from nests 1, 2, and 4. Nests 1 and 2 were constructed under pine trees, whereas nest 4 was on the oak tree. L. fuliginosus and L. flavus colonies were obtained from nests 3 and 5, which were built under willow tree and oak tree, respectively. Samples from each nest were collected using sterile forceps and deposited into a pre-sterilized container. Then, they were brought to the lab and processed immediately.
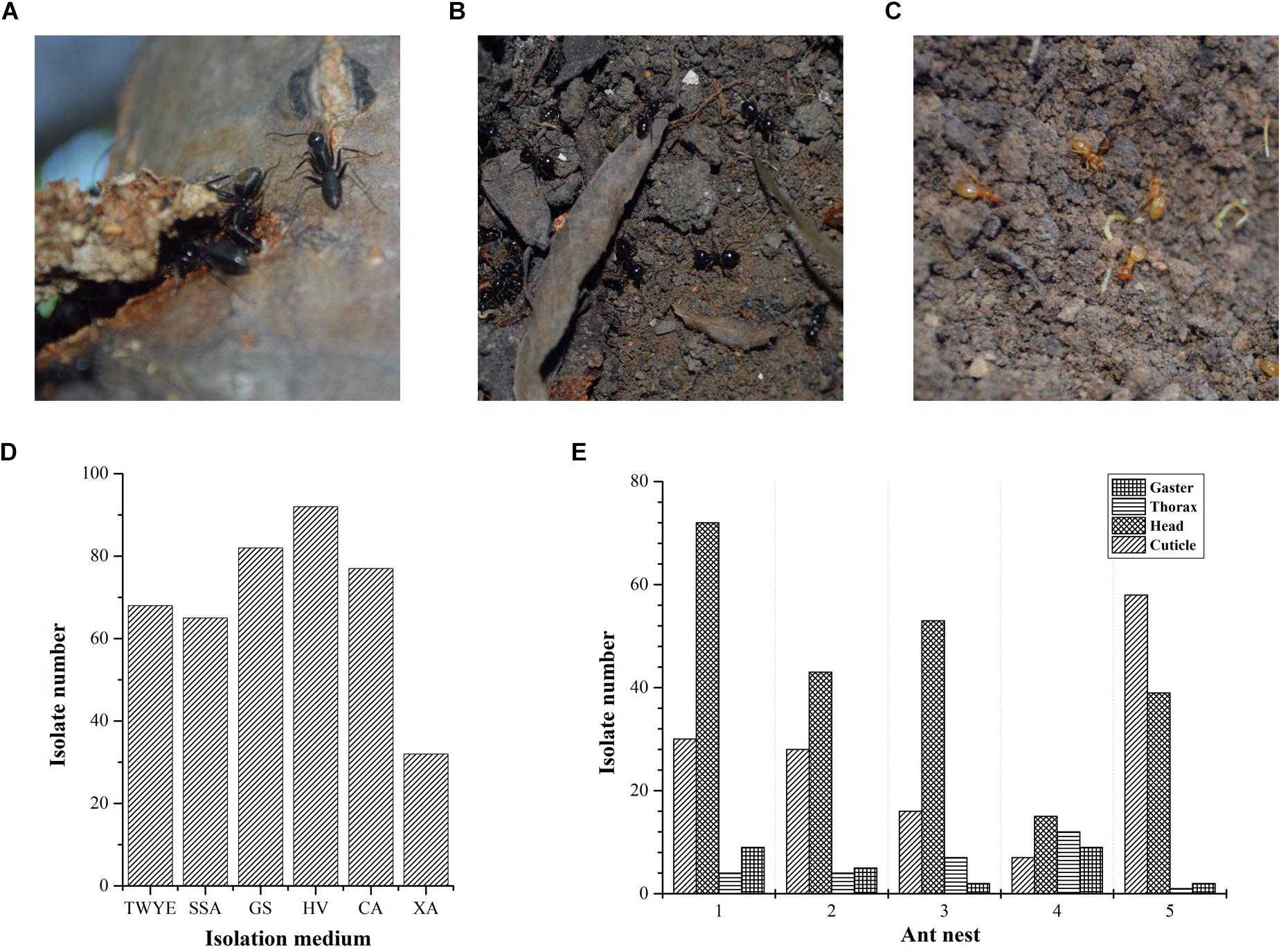
Figure 1. Ant species and actinobacteria isolation. (A) Camponotus japonicas; (B) Lasius fuliginosus; (C) Lasius flavus; (D) Compositions of the six different media used for the isolation of actinobacteria from ant samples; (E) Actinobacteria isolated from different body parts of ants from five nests.
Actinobacteria Isolation
For large sized ant (C. japonicas), five individuals were placed in an autoclaved 1.5 mL Eppendorf tube for actinobacteria isolation. For medium (L. fuliginosus) and small sized ants (L. flavus), ten and twenty individuals were used, respectively. Each sample was rinsed twice with sterile water to remove the surface soil and adherent epiphytes, followed by immersion in 1.2 mL sterile water and vortexed for 30 s. The suspension was transferred to a new autoclaved Eppendorf tube for actinobacteria isolation from the cuticle. Subsequently, the ant samples were surface sterilized by 70% ethanol for 1 min. Ethanol waste was removed and ant specimens were rinsed three times with sterile water. After external sterilization, the specimens were divided into head, thorax and gaster, and each body part was separately immersed in 1.2 mL sterile water and cultivated for 30 min at 28°C with shaking at 180 rpm. Finally, an aliquot of 200 μL suspension was plated onto six different actinobacteria-selective media types: humic acid-vitamin (HV) agar (Hayakawa and Nonomura, 1987); Gause’s synthetic (GS) agar no. 1 (Atlas, 1993); chitin agar (CA) (Hanshew et al., 2015); tap water-yeast extract (TWYE) agar (Qin et al., 2009); sodium succinate-asparagine (SSA) agar (Li et al., 2012); and xylan-arginine (XA) agar (Qin et al., 2012). All media were amended with nalidixic acid (20 mg/L) and cycloheximide (50 mg/L) to suppress the growth of gram-negative bacteria and fungi. Cultures were incubated at 28°C for 2–3 weeks. Colonies obtained after incubation were transferred onto oatmeal agar (ISP3) (Shirling and Gottlieb, 1966), and then maintained on slants at 4°C or as glycerol suspensions (20%, v/v) at −80°C.
Taxonomic Identification of Isolates
Isolates were cultivated on ISP 3 medium at 28°C for 2 weeks, and then preliminarily identified according to their phenotypic characteristics including the characteristics of colonies on plates, color of aerial and substrate mycelium, and production of diffusible pigment. Genomic DNA was extracted from isolates using lysozyme-SDS-phenol/chloroform method (Maniatis et al., 1982). PCR amplifications for the 16S rRNA gene sequence were performed using the universal bacterial primers, 27F and 1492R (Lane, 1991). The reaction conditions were 95°C for 5 min, followed by 30 cycles of 95°C for 30 s, 55°C for 45 s, and 72°C for 1 min, with a final extension at 72°C for 10 min. The PCR products were purified and cloned into the vector pMD19-T (Takara) for sequencing. The almost full-length 16S rRNA gene sequences (∼1500 bp) were obtained and neighbor-joining phylogenetic tree was constructed using the molecular evolutionary genetics analysis (MEGA) software version 7.0 (Kumar et al., 2016). Bootstrap replication (1000 replications) was used to assess the topology of the phylogenetic tree (Felsenstein, 1985). The obtained gene sequences were deposited in the GenBank database under accession numbers KP784763-KP784808, KX777578-KX777633, KX977397-KX977399, and KR261651- KR261652, respectively.
Culture-Independent Community Analysis
Five ant individuals (C. japonicas) were rinsed three times with sterile water, and then ground-up using a sterile pestle inside the tube. Total community DNA was extracted using FastDNA®SPIN for soil kit refering to the manufacturers’ specifications (MP Biomedicals, Solon, CA, United States). The V6 hypervariable region of the 16S rRNA gene was targeted for amplification by PCR with primers 967F and 1046R (Huse et al., 2008). The reaction conditions were 94°C for 2 min, followed by 30 cycles of 94°C for 30 s, 57°C for 30 s, and 72°C for 30 s, with a final extension at 72 for 5 min. PCR amplicon was purified using GeneJET Gel Extraction Kit (Thermo Scientific, Fermentas, Germany). Sequencing library was generated using NEB Next Ultra DNA Library Prep Kit for Illumina (New England Biolabs, Ipswich, MA, United States) according to the manufacturer’s instructions and index codes were added. The library quality was evaluated on the Qubit@ 2.0 Fluorometer and Agilent Bioanalyzer 2100 system (Agilent Technologies, Palo Alto, CA, United States). Finally, the library was sequenced with an Illumina HiSeq 2500 using 100 bp paired-end reads.
Raw reads were demultiplexed and quality-filtered using QIIME version 1.17 (Caporaso et al., 2010). Clean reads obtained were spliced using the PEAR software (Zhang et al., 2014). The merged sequences were then removed chimeras and clusterd in operational taxonomic units (OTUs) with the software UCLUST (Edgar, 2010) based on 97% nucleotide similarity. Taxonomy of the representative sequence for each OTU was assessed by RDP_Classifier and comparison against the SILVA 16S rRNA gene database (Quast et al., 2013). Raw sequencing data can be retrieved from the NCBI Short Read Archive under accession number SRR8061509.
In vitro Antifungal Assays
Antifungal screening was performed against 13 different phytopathogenic fungi: Rhizoctonia solani, Fusarium oxysporum, Curvularia lunata, Corynespora cassiicola, Setosphaeriaturcica turcicaf, Colletotrichum orbiculare, Alternaria solani, Helminthosporium maydis, Sphacelotheca reiliana, Sclerotinia sclerotiorum, Phytophthora sojae, Phytophthora capsici, and Phytophthora infestans. All pathogens were maintained at the Key Laboratory of Agricultural Microbiology within the Heilongjiang Province, China. P. sojae, P. capsici, and P. infestans were incubated on carrot agar (Cao et al., 2017) at 20°C, whereas the others were incubated on potato dextrose agar (PDA) (Cao et al., 2017) at 28°C except S. sclerotiorum at 20°C. Antifungal activity of isolates were assessed using the dual culture plate assay (Hamzah et al., 2018). When a clear inhibitory zone had formed, typically within 1–2 weeks after fungal inoculation, the inhibition diameters were measured, and then the inhibition rates were calculated according to the formula described by Liu et al. (2019). The assay was performed three replicates.
Secondary Metabolite Characterization
Five isolates with broad-spectrum antifungal activity, including three novel species Streptomyces capitiformicae 1H-SSA4, Streptomyces amphotericinicus 1H-SSA8 and Streptomyces lasiicapitis 3H-HV17(2), as well as strains Streptomyces sp. 1H-GS5 and Streptomyces sp. 1H-XA2, were performed for secondary metabolites characterization. The isolation and identification of secondary metabolites from strains 1H-SSA4, 1H-SSA8, 3H-HV17(2) and 1H-GS5 have been reported by our previous studies (Liu et al., 2016; Cao et al., 2017; Liu et al., 2017; Ye et al., 2017; Jiang et al., 2018). Here, we describe the detailed isolation and structural determination of the compounds from strain 1H-XA2.
Strain 1H-XA2 was grown on ISP 3 slant medium for 1 week at 28°C. Then it was inoculated into 250 mL baffled Erlenmeyer flasks containing 50 mL of sterile seed medium (TSB) and cultivated for 2 days at 30°C with shaking at 250 rpm. After that, aliquots (12.5 mL) of the culture were transferred into 1 L baffled Erlenmeyer flasks filled with 250 mL of the production medium (soluble starch 2%, tryptone 2%, glycerol 1%, NaCl 0.05%, K2HPO4⋅3H2O 0.05%, MgSO4⋅7H2O 0.05%, FeSO4⋅7H2O 0.05%, KNO3 0.1%, pH 7.2–7.4) and cultured at 30°C for 1 week with shaking at 250 rpm. The fermentation broth (25 L) was centrifuged (4000 rev/min, 20 min), and the supernatant was extracted with ethylacetate three times. The ethylacetate extract was subsequently evaporated in vacuo to afford 10.0 g of oily crude extract. The mycelia were extracted with methanol (1 L), and then concentrated in vacuo to remove the methanol to yield the aqueous concentrate. This aqueous concentrate was finally extracted with EtOAc (1 L × 3) to give 1.0 g of oily crude extract after removing the ethylacetate. Both extracts revealed an identical set of metabolites based on HPLC and TLC analyses, and therefore, they were combined for further purification.
The crude extract (11 g) was subjected to silica gel using a successive elution of petroleum ether/ethylacetate (1:0, 10:1, 5:1, 1:1 and 0:1, v/v) (1-7). Fraction 6 was subjected to Sephadex LH-20 column elution with methanol to collect three fractions (F6A, F6B, and F6C). F6B was purified by semipreparative HPLC (YMC-Triart C18 column, 250 × 10 mm i.d., 5 μm, 3.0 mL min–1, 0–26.0 min CH3OH: H2O = 44:56, 26.1–39.0 min CH3OH: H2O = 62:38, 39.1–43 min CH3OH: H2O = 100:0) to obtain compound 1 (tR = 38.2, 5.4 mg). Fraction 7 was subjected to Sephadex LH-20 column elution with MeOH to collect two fractions (F7A and F7B). Compound 2 (tR = 11.5 min, 33.7 mg) and 3 (tR = 18.5 min, 24.8 mg) were obtained from the F7A by semipreparative HPLC (YMC-Triart C18 column, 250 mm × 10 mm i.d., 5 μm, CH3OH: H2O = 48:52, 0.1% acetic acid, 3 mL/min).
Structures of compounds 1, 2, and 3 were determined using spectroscopic analysis. NMR spectra were measured with a Bruker Avance III-400 spectrometer in DMSO using TMS as internal standard. HR-ESI-MS or ESI-MS data were obtained using an UPLC-IT-TOF mass instrument (Shimadzu, Japan).
Results
Isolation of Ant-Derived Actinobacteria
A total of 416 isolates were obtained from three different ant species sampled and the most frequently isolated genus based on phenotypic characteristics was Streptomyces (∼80% of isolates). Of the five ant nests sampled, nest 1 yielded the most isolates (115), followed by nest 5 (100), nest 2 (80), nest 3 (78), and nest 4 (43). HV medium yielded the highest number of isolates (Figure 1D). The majority of isolates were cultured from head samples (222 isolates, 53.4%), followed by cuticle (139 isolates, 33.4%), thorax (28 isolates, 6.7%) and gaster (27 isolates, 6.5%) (Figure 1E). Based on morphological and cultural characteristics, 107 strains with different phenotypic characteristics were chosen for further investigation.
Diversity of Cultured Actinobacteria
16S rRNA gene sequence analysis of the 107 isolates revealed considerable diversity, which distributed among six family: Streptomycetaceae, Micromonosporaceae, Promicromonosporaceae, Nocardiaceae, Sreptosporangiaceae, and Thermomonosporaceae within the phylum Actinobacteria, including ten known genera. Streptomyces was the most frequently isolated genus (73%, 77 isolates), followed by Micromonospora (19 isolates) and Nocardia (3 isolates). Some rare genera, including Promicromonospora, Non-omuraea, Verrucosispora, Phytohabitans, Streptosporangium, Microbispora, and Actinocorallia were isolated less frequently and were represented by only one strain (Figure 2 and Supplementary Table S1). Furthermore, EzTaxon analysis of the 16S rRNA gene sequences revealed that many strains showed relatively low similarities to the type strains of the corresponding genera. Finally, 13 strains, including 1C-GS8, 2C-SSA16(2), 1C-HV12, 1H-GS9, 1H-HV4, 1H-SSA4, 1H-SSA8, 2C-HV3, 2H-TWYE14, 3C-HV12, 3H-GS17, 3H-HV17(2), and 5H-CA11, were chosen for detailed identification using standard polyphasic taxonomic identification methods, and have been proposed to represent novel species of the genera Streptomyces, Nocardia, Promicromonospora, Microbispora, and Actinocorallia (Bai et al., 2016; Guo et al., 2016; Han et al., 2016; Liu et al., 2016a,b,c, 2018; Li et al., 2016; Cao et al., 2017; Piao et al., 2017; Ye et al., 2017; Jiang et al., 2018).
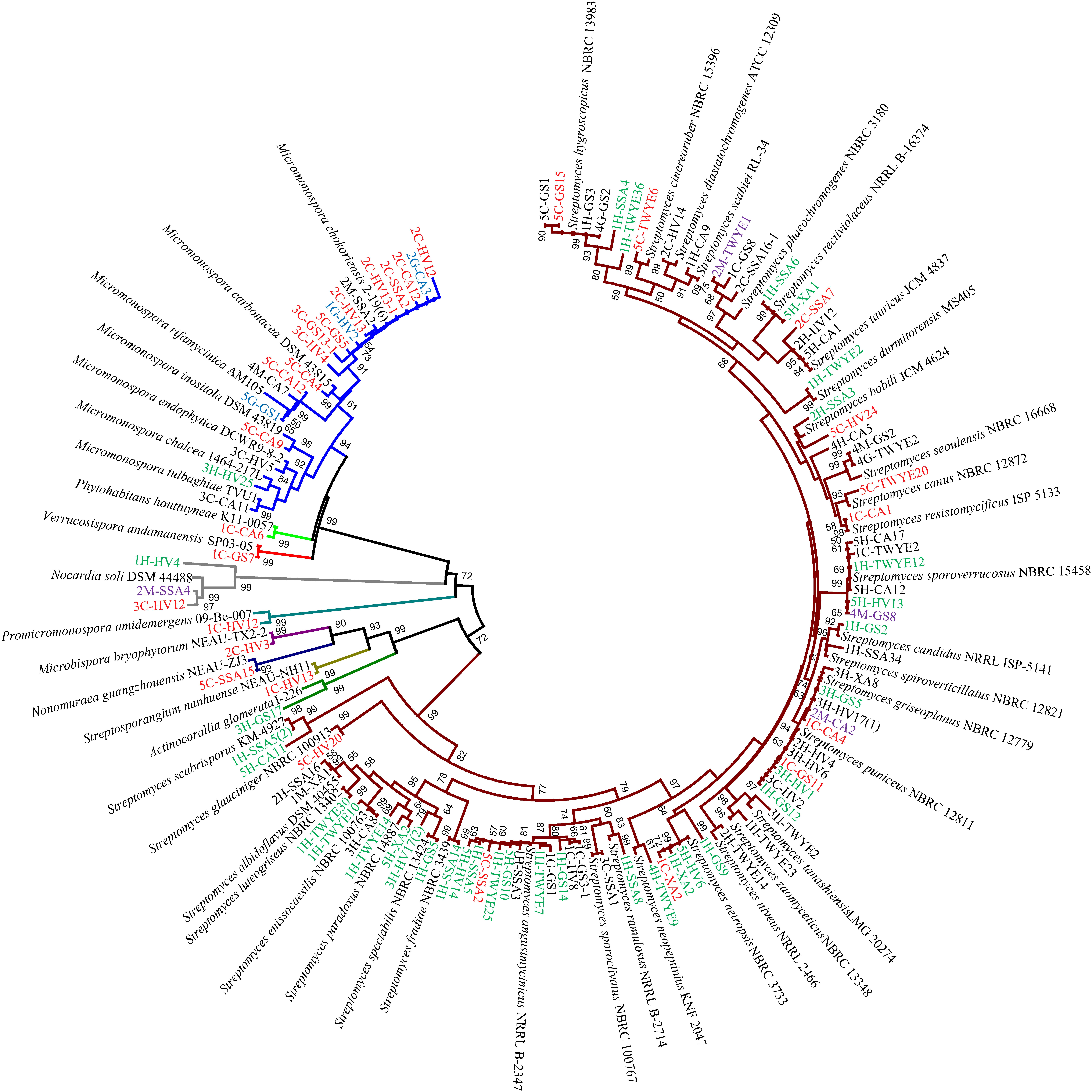
Figure 2. Neighbor-joining phylogenetic tree of 16S rRNA gene sequences from 107 actinobacteria in this study and their phylogenetic neighbors. Numbers at nodes are bootstrap values (percentages of 1000 replications); only values >50% are shown. A branch indicated by the same color belongs to the same genus. Isolates indicated by green from head, red from curticle, purple from thorax, and blue from gaster.
Culture-Independent Community
The pyrosequencing of V6 hypervariable region was performed to analyze the actinobacterial community within C. japonicas sample from nest 1. A total of 332440 high-quality reads that distributed across 1515 OTUs were generated. Taxonomic assignment of OTUs revealed the presence of 16 known phyla, wherein the Proteobacteria was the most abundant phylum, followed by Actinobacteria as the second largest phylum (Figure 3A and Supplementary Table S2). At the family level, 40 known actinobacterial families were observed, with Nocardioidaceae, Nocardiaceae, Dermacoccaceae, Intrasporangiaceae, and Streptomycetaceae representing the most abundant taxa (Figure 3B and Supplementary Table S3). Cultivation-independent analyses showed more diverse actinobacterial communities than did the cultivation-dependent method. Of the 40 families detected by cultivation-independent sequencing, six families were also obtained by cultivation.
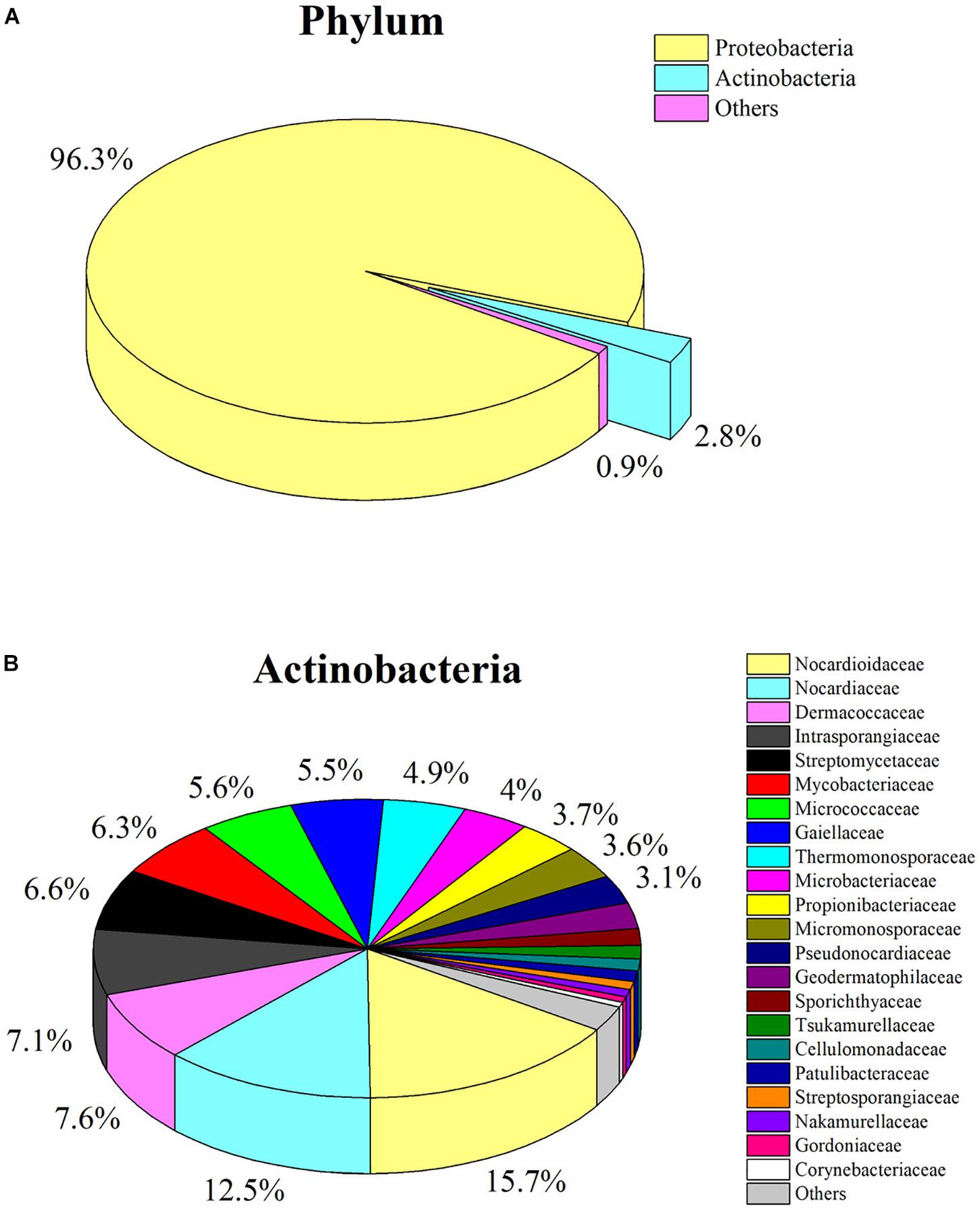
Figure 3. Analysis of culture-independent microbial communities. (A) Pie chart showing relative abundance of OTUs (>0.5%) at phylum level; (B) Pie chart showing relative abundance of OTUs (>0.5%) at family level from the phylum Actinobacteria.
Antifungal Activity Evaluation
Fifty-six of the 107 strains (52.3%) exhibited antifungal activity against at least one of the phytopathogenic fungi tested (Figure 4). All the active strains belong to the genus Streptomyces. Eleven strains were found to inhibit half of the pathogens. Four strains, 1H-XA2, 1H-GS5, 1H-SSA8, and 3H-HV17(2), showed the inhibitory capability against all tested fungi. The antifungal activity against Colletotrichum orbiculare was the most prevalent (32 isolates, 29.9%).
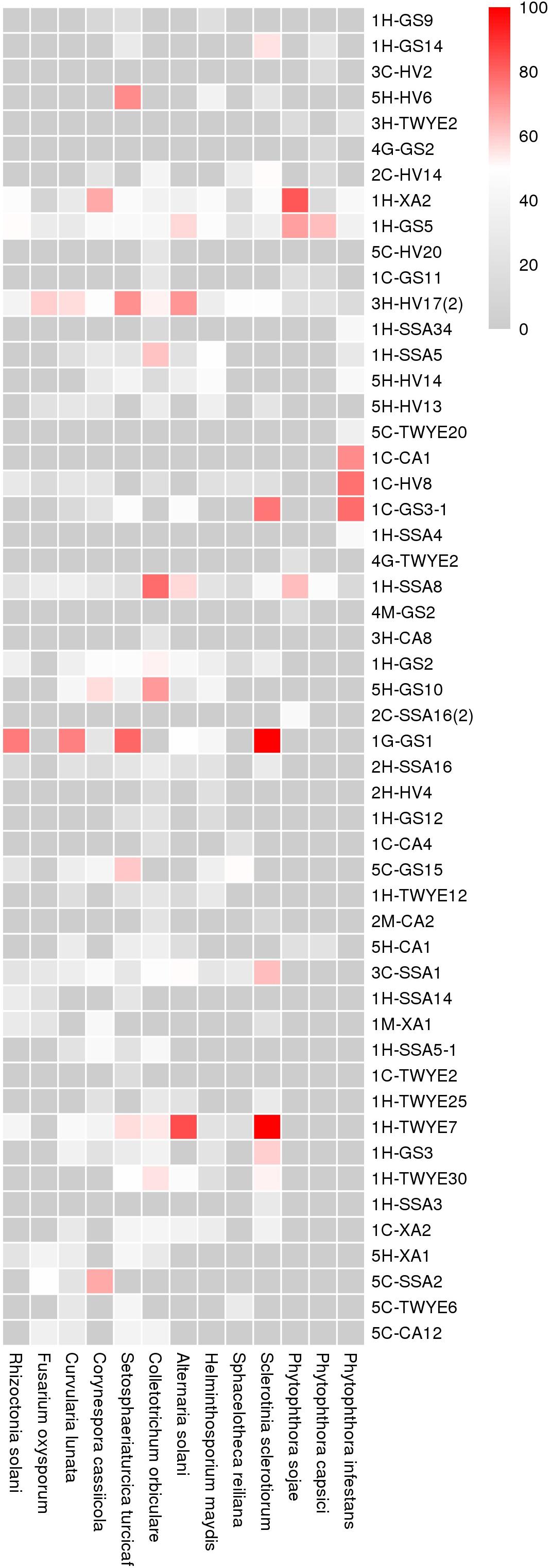
Figure 4. Bioassay evaluation of the antifungal activity of ant-derived actinobacteria. Boxes represent the inhibition rates (0–100%) and different colors indicate the degree of inhibition.
Identification of Secondary Metabolites
In view of the broad-spectrum antifungal activity of strain 1H-XA2 (Figure 5A), we investigated its secondary metabolites, and obtained a novel polyene amide compound (1), together with two known compounds, furamycins I and II (2 and 3). The structures were determined on the basis of spectroscopic data in this paper.
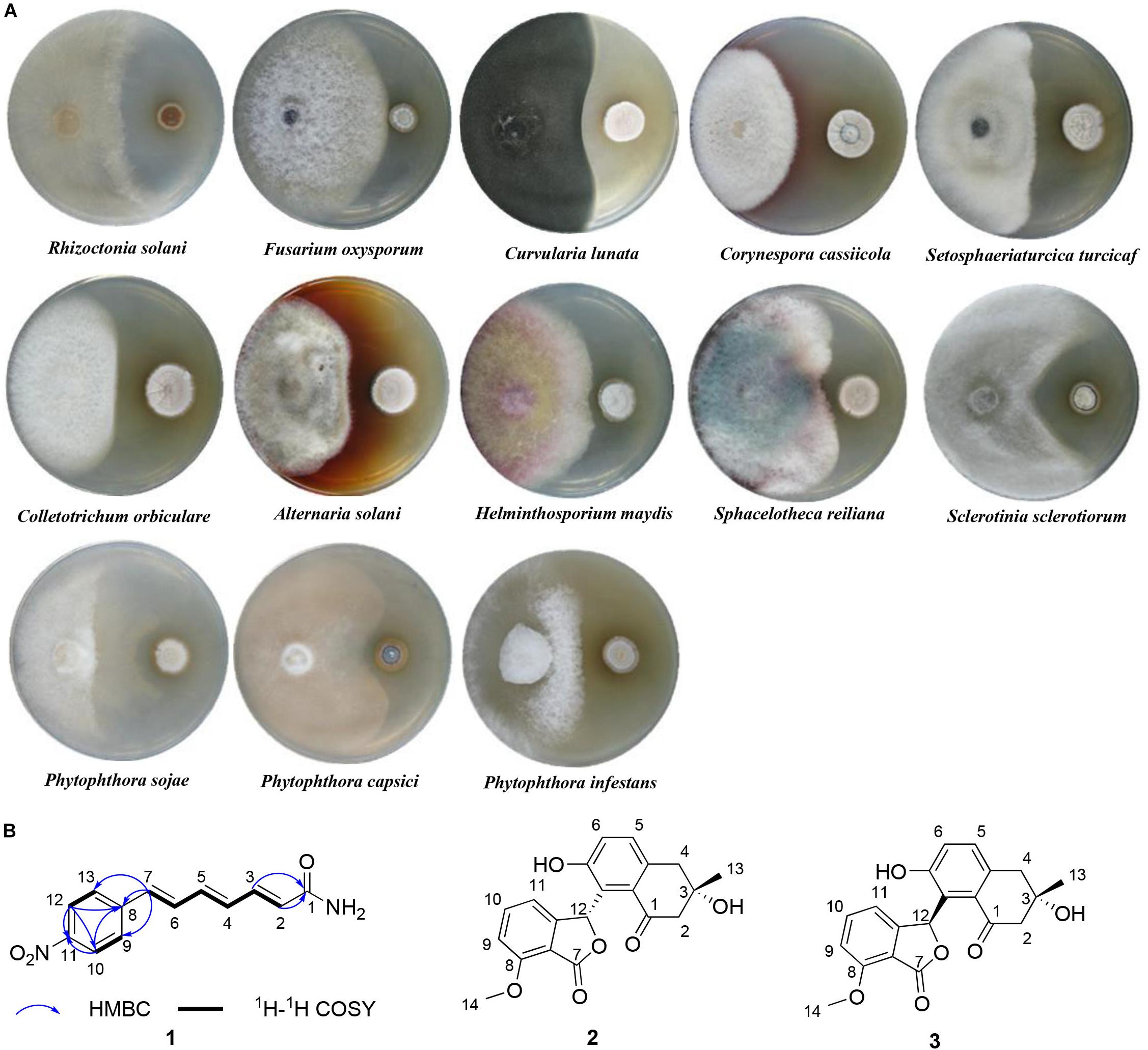
Figure 5. The antifungal activity and secondary metabolites of strain 1H-XA2. (A) The antifungal activity of strain 1H-XA2 against phytopathogenic fungi; (B) The structures of compounds 1–3.
Compound 1 was obtained as yellow amorphous powder, its molecular formula C13H12N2O3 was determined by high resolution electrospray ionization mass spectrometry (HRESIMS) data (m/z 267.0740, [M+Na]+, calcd for 267.0740), corresponding to nine degrees of unsaturation. The 1H NMR and 1H-1HCOSY data (Table 1) indicated an 1,4-disubstituted phenyl group with signals at δH 7.76 (d, J = 8.7 Hz, 2H), 8.20 (d, J = 8.7 Hz, 2H). In addition, the 1H NMR data of 1 (Table 1) also revealed the presence of three trans double bonds at δH 7.30 (dd, J = 15.6, 10.8 Hz, H-6), 7.14 (dd, J = 15.0, 11.3 Hz, H-3), 6.90 (d, J = 15.6 Hz, H-7), 6.66 (dd, J = 14.7, 11.3 Hz, H-4), 6.81 (dd, J = 14.7, 10.8 Hz, H-5), 6.12 (d, J = 15.1 Hz, H-2), along with two active protons signals [δH 7.51 (brs) and 7.05 (brs)]. The 13C and HSQC spectra of 1 suggested thirteen carbons, which were classified into one carbonyl carbons, ten sp2 methine carbons and two sp2 quaternary carbons.
The 1H-1H COZY and HSQC spectrum (Supplementary Figure S1) of 1 showed one spin-coupling systems, H-2/H-3/H-4/H-5/H-6/H-7 (Figure 5B). Cross-peaks from H-7 to C-8/C-9/C-13, H-9/H-13 to C-7 were observed in the HMBC spectrum (Supplementary Figure S1), which suggested 1, 4-disubstituted phenyl group connected with olefin side chain at C-8. The HMBC cross-peaks from H-2/H-3 to C-1 (δC 166.64) revealed the presence of an amide residue, respectively. The remaining nitro functional group was determined to be substituted at C-11 by comparison of the chemical shifts with polypropionate aureothin (Ueda et al., 2007) and based on high resolution electrospray ionization mass spectrometry. The configurations of double bonds as 2E, 4E, and 6E were confirmed by the vicinal proton typical coupling constants (∼15 Hz). Consequently, compound 1 was elucidated as shown in Figure 5B.
Compound 2 was isolated as colorless powder, its ESI-MS spectrum showed a molecular ion peak at m/z 377 [M+Na]+ and 731 [2M+Na]+ (Supplementary Figure S2). 1H (400 MHz) and 13C (100 MHz) NMR data were shown in Table 2. Compound 2 was proved to be furammycins I by direct comparison of these data with those from the literature (Zhang S. et al., 2018).
Compound 3 was isolated as colorless powder. Its ESI-MS spectrum showed a molecular ion peak at m/z 377 [M+Na]+ and 731 [2M+Na]+ (Supplementary Figure S2). 1H (400 MHz) and 13C (100 MHz) NMR data were shown in Table 2. Compound 3 was proved to be furammycins II by direct comparison of these data with those from the literature (Zhang S. et al., 2018).
Discussion
In this study, the diversity of culturable ant–associated actinobacteria obtained from three ant species is investigated. Our results have shown that the actinobacterial community is diverse and that the most abundant genus recovered is Streptomyces, which is consistent with previous reports (Matarrita-Carranza et al., 2017). It is worth noting that such large amounts of rare actinobacterial genera and novel taxa isolated from ant samples have not been previously reported. Instead, a single genus of actinobacteria (Streptomyces or Pseudonocardia) has often been reported to be associated with different ant species (Seipke et al., 2011; Caldera and Currie, 2012). It can be considered that the different approach employed in this study led to the successful isolation of various actinobacteria. Firstly, six different type of isolation media were used in this study. Besides CA medium that was widely used to isolate insect–associated actinobacteria (Visser et al., 2012; Matarrita-Carranza et al., 2017), several media (TWYE, SSA, XA, and HV) with relative simple nutrients that were proved to be effective for actinobacteria isolation were used in this study (Qin et al., 2012). Especially, HV medium significantly increased the recovery of isolates as well as rare actinobacterial genera, which resemble with soil-derived or endophytic actinobacteria isolation (Matsukawa et al., 2007; Kaewkla and Franco, 2013). Moreover, actinobacteria from different body segments of the ants (cuticle, head, thorax, and gaster) were investigated. Previous studies have focused on actinobacteria from the cuticle (Currie et al., 1999; Zucchi et al., 2011; Caldera and Currie, 2012). Although large amounts of isolates were also obtained in this segment, interestingly, the head segment yielded the highest number of isolates in the present study. This finding could potentially be due to the infrabuccal pocket, a specialized pouch in the oral cavity of the ants, which is known to be used for cleaning themselves, their nestmates and brood (Hölldobler and Wilson, 1990). Early results indicated that the pocket of C. japonicas harbored a high abundance of actinobacteria strains (Zhang et al., 2016; Zhang K.X. et al., 2018).
Out of the five nests sampled, the ant sample from nest 4 yielded the fewest number of isolates. The ant species from nests 1, 2, and 4 were the same. The only difference is that nests 1 and 2 located in the soil environment, whereas nest 4 was built on a tree. This result could be owing to the fact that solid food and other materials with environmental microorganisms will be accumulated in the infrabuccal pocket, which led to the potential for some of the isolates we obtained being environmental contaminants. Therefore, fewer isolates from nest 4 observed here could be explained by less abundant microorganisms existing in plant tissue compared with soil environment.
Considering the limitations of isolation methods, the culture-independent approach was employed to accurately evaluate the composition of ant-derived actinobacterial community. A total of 40 known actinobacterial families were detected in the ant sample, whereas only six families were isolated by culturing. This result provides the impetus for the development of new methods or strategies to obtain more diverse antinobacteria from ants in the future studies. Taxonomic classification of the OTU-representative sequences to family level showed that Nocardioidaceae (15.7%) and Nocardiaceae (12.5%) largely dominate the ant host actinobacterial community, whereas Streptomycetaceae observed as the most dominant cultivated family was found in lower abundance (6.6%). Similar results were also reported in the soil environment, where Streptomyces belonging to the family Streptomycetaceae was the predominant genus of culturable actinobacteria, but it was not the largest community detected by culture-independent technique (Lundberg et al., 2012). It might be because the isolation media used in this study presents a bias that favored the growth of Streptomyces strains. Other studies also demonstrated that Streptomyces was the most commonly isolated genus from soil, plant and insect environments using these media, although many rare actinobacteria genera were also recovered (Matsukawa et al., 2007; Qin et al., 2012; Kaewkla and Franco, 2013; Matarrita-Carranza et al., 2017). The interaction between members of the genus Pseudonocardia in the family Pseudonocardiaceae and fungus-growing ants has been extensively reported (Currie et al., 1999; Andersen et al., 2013). Members of the family Pseudonocardiaceae were not obtained by cultivation. However, the relatively high abundance of the family (3.1%) was detected by high-throughput sequencing method.
To validate that ant-derived actinobacteria represent a source of antimicrobials with activity against phytopathogenic fungi, 107 different strains were conducted in vitro antagonism assays using 13 different phytopathogenic fungi. Our results indicated that a high percentage of strains (52.3%) possessed the capacity to inhibit fungi. There is also evidence that insect-associated actinobacteria showed significantly greater antifungal activity compared to soil and plant-associated actinobacteria (Chevrette et al., 2019). All the active strains belong to the genus Streptomyces through 16S rRNA gene sequence analysis. Indeed, it is not surprising that members of this taxon encompassed the most active strains, as revealed by previous studies (Verma et al., 2009; Liu et al., 2019). Notably, high proportions of active strains (52%, 29 strains) were obtained from the head sample. This result could potentially be correlated with the infrabuccal pocket, where fungus-growing ants have a symbiotic association with actinobacteria that inhibit the parasitic fungus (Escovopsis sp.) (Little et al., 2006).
Actinobacteria from insect microbiomes are a rich source of antifungal natural products and a number of compounds with unique structures were identified (Van Arnam et al., 2018; Chevrette et al., 2019). Our chemical investigation of four ant-derived Streptomyces strains led to the discovery of eight known compounds and two new polyketide compounds (Liu et al., 2016, 2017; Cao et al., 2017; Ye et al., 2017; Jiang et al., 2018). Among the ten compounds, amphotericin, and kanchanamycin are known as excellent antifungal agents (Fiedler et al., 1996; Hamill, 2013; Zhang et al., 2019). In this report, we investigated the secondary metabolites from ant-derived Streptomyces strain 1H-XA2 with broad-spectrum antifungal activity, which resulted in the discovery of furamycins I and II (2 and 3), as well as a novel polyene amide compound (1). Furamycins I and II have been reported to be produced by a marine Streptomyces species and to show strong antifungal activity against Candida albicans, Colletortrichum gloeosporiodes, and Penicillium marneffei (Zhang S. et al., 2018). Polyene compounds with antifungal activity were also recovered from insect host Streptomyces (Oh et al., 2009b, 2011). However, compound 1 did not show any antifungal or antibacterial activity (data not shown). Previous study showed that its structural analogs, trichostatin A and BL1521, could inhibit proliferation and induce apoptosis in neuroblastoma cells (de Ruijter et al., 2004). Therefore, further bioactive assays to define its functional role is under way.
Conclusion
Ant-derived actinobacterial community structure was analyzed using both culture-dependent and -independent methods. Ants harbored abundant and diverse communities of actinobacteria, including many rare actinobacteria and novel taxa. Antifungal activity assays showed that high percentage of the isolates had antagonistic activity agaist phytopathogenic fungi. In addition, two known compounds and one new compound were identified. These results suggest that ant-derived actinobacteria represent a promising and underexplored resource for exploring novel agricultural antifungals.
Data Availability Statement
Publicly available datasets were analyzed in this study. This data can be found here: The obtained 16S rRNA gene sequences were deposited in the GenBank database under accession numbers KP784763–KP784808, KX777578–KX777633, KX977397–KX977399, and KR261651–KR261652, respectively. High-throughput sequencing data can be retrieved from the NCBI Short Read Archive under accession number SRR8061509.
Author Contributions
CL and JZ designed the experiments. CL, ZW, ZY, JZ, XZ, PC, and XG performed the experiments. CL analyzed the data and prepared the manuscript. WX reviewed the manuscript.
Funding
This work was supported by the National Natural Science Foundation of China (Grant Nos. 31500010, 31701858, and 31872037) and the Academic Backbone Project of Northeast Agricultural University (Grant No. 17XG17).
Conflict of Interest
The authors declare that the research was conducted in the absence of any commercial or financial relationships that could be construed as a potential conflict of interest.
Acknowledgments
We are grateful to Prof. Shanyi Zhou for the identification of ant species.
Supplementary Material
The Supplementary Material for this article can be found online at: https://www.frontiersin.org/articles/10.3389/fmicb.2020.00201/full#supplementary-material
References
Andersen, S. B., Hansen, L. H., Sapountzis, P., Sørensen, S. J., and Boomsma, J. J. (2013). Specificity and stability of the Acromyrmex-Pseudonocardia symbiosis. Mol. Ecol. 22, 4307–4321. doi: 10.1111/mec.12380
Atlas, R. M. (1993). Handbook of Microbiological Media, ed. L. C. Parks (Boca Raton, FL: CRC Press).
Bai, L., Liu, C. X., Guo, L. F., Piao, C. Y., Li, Z. L., Li, J. S., et al. (2016). Streptomyces formicae sp. nov., a novel actinomycete isolated from the head of Camponotus japonicas Mayr. Antonie van Leeuwenhoek 109, 253–261. doi: 10.1007/s10482-015-0628-7
Bailey, I. W. (1920). Some relations between ants and fungi. Ecology 1, 174–189. doi: 10.2307/1929134
Beemelmanns, C., Ramadhar, T. R., Kim, K. H., Klassen, J. L., Cao, S., Wyche, T. P., et al. (2017). Macrotermycins A-D, glycosylated macrolactams from a termite-associated Amycolatopsis sp. M39. Org. Lett. 19, 1000–1003. doi: 10.1021/acs.orglett.6b03831
Blodgett, J. A. V., Oh, D. C., Cao, S., Currie, C. R., Kolter, R., and Clardy, J. (2010). Common biosynthetic origins for polycyclic tetramate macrolactams from phylogenetically diverse bacteria. Proc. Natl. Acad. Sci. U.S.A. 107, 11692–11697. doi: 10.1073/pnas.1001513107
Caldera, E. J., and Currie, C. R. (2012). The population structure of antibiotic-producing bacterial symbionts of Apterostigma dentigerumants: impacts of coevolution and multipartite symbiosis. Am. Nat. 80, 604–617. doi: 10.1086/667886
Cao, T. T., Mu, S., Lu, C., Zhao, S. S., Li, D. M., Yan, K., et al. (2017). Streptomyces amphotericinicus sp. nov., an amphotericin-producing actinomycete isolated from the head of an ant (Camponotus japonicas Mayr). Int. J. Syst. Evol. Microbiol. 67, 4967–4973. doi: 10.1099/ijsem.0.002382
Caporaso, J. G., Kuczynski, J., Stombaugh, J., Bittinger, K., Bushman, F. D., Costello, E. K., et al. (2010). QIIME allows analysis of high-throughput community sequencing data. Nat. Methods 7, 335–336. doi: 10.1038/nmeth.f.303
Carr, G., Derbyshire, E. R., Caldera, E., Currie, C. R., and Clardy, J. (2012). Antibiotic and antimalarial quinones from fungus-growing ant-associated Pseudonocardia sp. J. Nat. Prod. 75, 1806–1809. doi: 10.1021/np300380t
Chevrette, M. G., Carlson, C. M., Ortega, H. E., Thomas, C., Ananiev, G. E., Barns, K. J., et al. (2019). The antimicrobial potential of Streptomyces from insect microbiomes. Nat. Commun. 10:516. doi: 10.1038/s41467-019-08438-0
Currie, C. R., Scott, J. A., Summerbell, R. C., and Malloch, D. (1999). Fungus-growing ants use antibiotic-producing bacteria to control garden parasite. Nature 398, 701–704. doi: 10.1038/19519
de Ruijter, A. J., Kemp, S., Kramer, G., Meinsma, R. J., Kaufmann, J. O., Caron, H. N., et al. (2004). The novel histone deacetylase inhibitor BL1521 inhibits proliferation and induces apoptosis in neuroblastoma cells. Biochem. Pharmacol. 68, 1279–1288. doi: 10.1016/j.bcp.2004.05.010
Edgar, R. C. (2010). Search and clustering orders of magnitude faster than BLAST. Bioinformatics 26, 2460–2461. doi: 10.1093/bioinformatics/btq461
Felsenstein, J. (1985). Confidence limits on phylogenies: an approach using the bootstrap. Evolution 39, 783–791. doi: 10.1111/j.1558-5646.1985.tb00420.x
Fiedler, H. P., Nega, M., Pfefferle, C., Groth, I., Kempter, C., Stephan, H., et al. (1996). Kanchanamycins, new polyol macrolide antibiotics produced by Streptomyces olivaceus Tü 4018. I. Taxonomy, fermentation, isolation and biological activities. J. Antibiot. 49, 758–764. doi: 10.7164/antibiotics.49.758
Guo, L. F., Liu, C. X., Zhao, J. W., Li, C., Guo, S., Fan, J. L., et al. (2016). Promicromonospora alba sp. nov., an actinomycete isolated from the cuticle of Camponotus japonicas Mayr. Int. J. Syst. Evol. Microbiol. 66, 1340–1345. doi: 10.1099/ijsem.0.000885
Hamill, R. J. (2013). Amphotericin B formulations: a comparative review of efficacy and toxicity. Drugs 73, 919–934. doi: 10.1007/s40265-013-0069-4
Hamzah, T. N. T. H., Lee, S. Y., Hidayat, A., Terhem, R., Faridah-Hanum, I., and Mohamed, R. (2018). Diversity and characterization of endophytic fungi isolated from the tropical mangrove species, Rhizophora mucronata, and identification of potential antagonists against the soil-borne fungus, Fusarium solani. Front. Microbiol. 9:1707. doi: 10.3389/fmicb.2018.01707
Han, C. Y., Liu, C. X., Zhao, J. W., Guo, L. F., Lu, C., Li, J. S., et al. (2016). Microbispora camponoti sp. nov., a novel actinomycete isolated from the cuticle of Camponotus japonicus Mayr. Antonie van Leeuwenhoek 109, 215–223. doi: 10.1007/s10482-015-0625-x
Hanshew, A. S., McDonald, B. R., Díaz Díaz, C., Djiéto-Lordon, C., Blatrix, R., and Currie, C. R. (2015). Characterization of actinobacteria associated with three ant–plant mutualisms. Microb. Ecol. 69, 192–203. doi: 10.1007/s00248-014-0469-3
Hayakawa, M., and Nonomura, H. (1987). Humic acid-vitamin agar, a new medium for the selective isolation of soil actinomycetes. J. Ferment. Technol. 65, 501–509. doi: 10.1016/0385-6380(87)90108-7
Huse, S. M., Dethlefsen, L., Huber, J. A., Mark Welch, D., Relman, D. A., and Sogin, M. L. (2008). Exploring microbial diversity and taxonomy using SSU rRNA hypervariable tag sequencing. PLoS Genet. 4:e1000255. doi: 10.1371/journal.pgen.1000255
Jiang, S. W., Piao, C. Y., Yu, Y., Cao, P., Li, C. X., Yang, F., et al. (2018). Streptomyces capitiformicae sp. nov., a novel actinomycete producing angucyclinone antibiotics isolated from the head of Camponotus japonicas Mayr. Int. J. Syst. Evol. Microbiol. 68, 118–124. doi: 10.1099/ijsem.0.002468
Kaewkla, O., and Franco, C. M. (2013). Rational approaches to improving the isolation of endophytic actinobacteria from Australian native trees. Microb. Ecol. 65, 384–393. doi: 10.1007/s00248-012-0113-z
Kim, S. H., Kwon, S. H., Park, S. H., Lee, J. K., Bang, H. S., and Nam, S. J. (2013). Tripartin, a histone demethylase inhibitor from a bacterium associated with a dung beetle larva. Org. Lett. 15, 1834–1837. doi: 10.1021/ol4004417
Kroiss, J., Kaltenpoth, M., Schneider, B., Schwinger, M. G., Hertweck, C., and Maddula, R. K. (2010). Symbiotic Streptomycetes provide antibiotic combination prophylaxis for wasp offspring. Nat. Chem. Biol. 6, 261–263. doi: 10.1038/nchembio.331
Kumar, S., Stecher, G., and Tamura, K. (2016). MEGA7: molecular evolutionary genetics analysis version 7.0 for bigger datasets. Mol. Biol. Evol. 33:1870. doi: 10.1093/molbev/msw054
Lane, D. (1991). “16S/23S rRNA sequencing,” in Nucleic Acid Techniques in Bacterial Systematics, eds E. Stackebrandt and M. Goodfellow (Chichester: John Wiley & Sons), 115–147.
Lee, L.-H., Chan, K.-G., Stach, J., Wellington, E. M. H., and Goh, B.-H. (2018). Editorial: the search for biological active agent(s) from actinobacteria. Front. Microbiol. 9:824. doi: 10.3389/fmicb.2018.00824
Li, J., Zhao, G. Z., Huang, H. Y., Qin, S., Zhu, W. Y., and Zhao, L. X. (2012). Isolation and characterization of culturable endophytic actinobacteria associated with Artemisia annua L. Antonie van Leeuwenhoek 101, 515–527. doi: 10.1007/s10482-011-9661-3
Li, Y., Ye, L., Wang, X. J., Zhao, J. W., Ma, Z. X., Yan, K., et al. (2016). Streptomyces camponoticapitis sp. nov., an actinomycete isolated from the head of an ant (Camponotus japonicas Mayr). Int. J. Syst. Evol. Microbiol. 66, 3855–3859. doi: 10.1099/ijsem.0.001276
Little, A. E., Murakami, T., Mueller, U. G., and Currie, C. R. (2006). Defending against parasites: fungus-growing ants combine specialized behaviours and microbial symbionts to protect their fungus gardens. Biol. Lett. 2, 12–16. doi: 10.1098/rsbl.2005.0371
Liu, C., Zhuang, X., Yu, Z., Wang, Z., Wang, Y., Guo, X., et al. (2019). Community structures and antifungal activity of root-associated endophytic actinobacteria of healthy and diseased soybean. Microorganisms 7:E243. doi: 10.3390/microorganisms7080243
Liu, C. X., Bai, L., Ye, L., Zhao, J. W., Yan, K., Xiang, W. S., et al. (2016a). Nocardia lasii sp. nov., a novel actinomycete isolated from the cuticle of an ant (Lasius fuliginosus L). Antonie van Leeuwenhoek 109, 1513–1520. doi: 10.1007/s10482-016-0753-y
Liu, C. X., Guan, X. J., Li, Y., Li, W. C., Ye, L., Kong, X. X., et al. (2016b). Nocardia camponoti sp. nov., an actinomycete isolated from the head of an ant (Camponotus japonicas Mayr). Int. J. Syst. Evol. Microbiol. 66, 1900–1905. doi: 10.1099/ijsem.0.000963
Liu, C. X., Han, C. Y., Jiang, S., Zhao, X. L., Tian, Y. Y., Yan, K., et al. (2018). Streptomyces lasii sp. nov., a novel actinomycete with antifungal activity isolated from the head of an ant (Lasius flavus). Curr. Microbiol. 75, 353–358. doi: 10.1007/s00284-017-1388-6
Liu, C. X., Li, Y., Ye, L., Zhao, J. W., Piao, C. Y., Li, Z. L., et al. (2016c). Actinocorallia lasiicapitis sp. nov., an actinomycete isolated from the head of an ant (Lasius fuliginosus L.). Int. J. Syst. Evol. Microbiol. 66, 2172–2177. doi: 10.1099/ijsem.0.001005
Liu, C. X., Liu, S. H., Zhao, J. W., Zhang, J., Wang, X. J., Li, J. S., et al. (2017). A new spectinabilin derivative with cytotoxic activity from ant-derived Streptomyces sp. 1H-GS5. J. Asian. Nat. Prod. Res. 19, 924–929. doi: 10.1080/10286020.2016.1254200
Liu, S. H., Xu, M. D., Zhang, H., Qi, H., Zhang, J., Liu, C. X., et al. (2016). New cytotoxic spectinabilin derivative from ant-associated Streptomyces sp. 1H-GS5. J. Antibiot. 69, 128–131. doi: 10.1038/ja.2015.99
Lundberg, D. S., Lebeis, S. L., Paredes, S. H., Yourstone, S., Gehring, J., Malfatti, S., et al. (2012). Defining the core Arabidopsis thaliana root microbiome. Nature 488, 86–90. doi: 10.1038/nature11237
Maniatis, T., Fritsch, E. F., and Sambrook, J. (1982). Molecular Cloning: A Laboratory Manual. New York, NY: Cold Spring Harbor Laboratory.
Matarrita-Carranza, B., Moreira-Soto, R. D., Murillo-Cruz, C., Mora, M., Currie, C. R., and Pinto-Tomas, A. A. (2017). Evidence for widespread associations between neotropical hymenopteran insects and actinobacteria. Front. Microbiol. 8:2016. doi: 10.3389/fmicb.2017.02016
Matsukawa, E., Nakagawa, Y., Iimura, Y., and Hayakawa, M. (2007). A new enrichment method for the selective isolation of streptomycetes from the root surfaces of herbaceous plants. Actinomycetologica 21, 66–69. doi: 10.3209/saj.saj210202
Oh, D. C., Poulsen, M., Currie, C. R., and Clardy, J. (2009a). Dentigerumycin: a bacterial mediator of an ant-fungus symbiosis. Nat. Chem. Biol. 5, 391–393. doi: 10.1038/nchembio.159
Oh, D. C., Poulsen, M., Currie, C. R., and Clardy, J. (2011). Sceliphrolactam, a polyene macrocyclic lactam from a wasp-associated Streptomyces sp. Org. Lett. 13, 752–755. doi: 10.1021/ol102991d
Oh, D. C., Scott, J. J., Currie, C. R., and Clardy, J. (2009b). Mycangimycin, a polyene peroxide from a mutualist Streptomyces sp. Org. Lett. 11, 633–636. doi: 10.1021/ol802709x
Patil, P. B., Zeng, Y., Coursey, T., Houston, P., Miller, I., and Chen, S. (2010). Isolation and characterization of a Nocardiopsis sp. From honeybee guts. FEMS Microbiol. Lett. 312, 110–118. doi: 10.1111/j.1574-6968.2010.02104.x
Piao, C. Y., Zheng, W. W., Li, Y., Liu, C. X., Jin, L. Y., Song, W., et al. (2017). Two new species of the genus Streptomyces: Streptomyces camponoti sp. nov. and Streptomyces cuticulae sp. nov. isolated from the cuticle of Camponotus japonicas Mayr. Arch. Microbiol. 199, 963–970. doi: 10.1007/s00203-017-1353-6
Qin, S., Chen, H. H., Zhao, G. Z., Li, J., Zhu, W. Y., Xu, L. H., et al. (2012). Abundant and diverse endophytic actinobacteria associated with medicinal plant Maytenus austroyunnanensis in Xishuangbanna tropical rainforest revealed by culture-dependent and culture-independent methods. Environ. Microbiol. Rep. 4, 522–531. doi: 10.1111/j.1758-2229.2012.00357.x
Qin, S., Li, J., Chen, H. H., Zhao, G. Z., Zhu, W. Y., Jiang, C. L., et al. (2009). Isolation, diversity, and antimicrobial activity of rare actinobacteria from medicinal plants of tropical rain forests in Xishuangbanna China. Appl. Environ. Microbiol. 75, 6176–6186. doi: 10.1128/AEM.01034-09
Qin, S., Li, W. J., Dastager, S. G., and Hozzein, W. N. (2016). Editorial: actinobacteria in special and extreme habitats: diversity, function roles, and environmental adaptations. Front. Microbiol. 7:1415. doi: 10.3389/fmicb.2016.01415
Quast, C., Pruesse, E., Yilmaz, P., Gerken, J., Schweer, T., Yarza, P., et al. (2013). The SILVA ribosomal RNA gene database project: improved data processing and web-based tools. Nucleic Acids Res. 41, D590–D596. doi: 10.1093/nar/gks1219
Sánchez-Peña, S. R., Ovalle, M. R., Gallegos-Morales, G., and Sánchez-Arizpe, A. (2008). Note: In vitro antagonism of actinomycetes isolated from fungus-growing ants against plant pathogenic fungi. Phytoparasitica 36, 322–325. doi: 10.1007/BF02980811
Scott, J. J., Oh, D. C., Yuceer, M. C., Klepzig, K. D., Clardy, J., and Currie, C. R. (2008). Bacterial protection of beetle-fungus mutualism. Science 322:63. doi: 10.1126/science.1160423
Seipke, R. F., Barke, J., Brearley, C., Hill, L., Yu, D. W., Goss, R. J., et al. (2011). A single Streptomyces symbiont makes multiple antifungals to support the fungus farming ant Acromyrmex octospinosus. PLoS One 6:e22028. doi: 10.1371/journal.pone.0022028
Shirling, E. B., and Gottlieb, D. (1966). Methods for characterization of Streptomyces species. Int. J. Syst. Bacteriol. 16, 313–340. doi: 10.1099/00207713-16-3-313
Stork, N. E., McBroom, J., Gely, C., and Hamilton, A. J. (2015). New approaches narrow global species estimates for beetles, insects, and terrestrial arthropods. Proc. Natl Acad. Sci. U.S.A. 112, 7519–7523. doi: 10.1073/pnas.1502408112
Swain, T. (1978). “Plant-animal coevolution. a synoptic view of the Paleozoic and Mesozoic,” in Biochemical Aspects of Plant and Animal Coevolution, ed. J. B. Harbourne (Oxfiord: Blackwell), 3–19.
Ueda, J. Y., Hashimoto, J., Nagai, A., Nakashima, T., Komaki, H., Anzai, K., et al. (2007). New aureothin derivative, alloaureothin, from Streptomyces sp. MM23. J. Antibiot. 60, 321–324. doi: 10.1038/ja.2007.41
Van Arnam, E. B., Currie, C. R., and Clardy, J. (2018). Defense contracts: molecular protection in insect-microbe symbioses. Chem. Soc. Rev. 47, 1638–1651. doi: 10.1039/c7cs00340d
Van Arnam, E. B., Ruzzini, A. C., Sit, C. S., Horn, H., Pinto-Tomás, A. A., Currie, C. R., et al. (2016). Selvamicin, an atypical antifungal polyene from two alternative genomic contexts. Proc. Natl. Acad. Sci. U.S.A. 113, 12940–12945. doi: 10.1073/pnas.1613285113
Verma, V. C., Gond, S. K., Kumar, A., Mishra, A., Kharwar, R. N., and Gange, A. C. (2009). Endophytic actinomycetes from Azadirachta indica A. Juss.: isolation, diversity, and anti-microbial activity. Microb. Ecol. 57, 749–756. doi: 10.1007/s00248-008-9450-3
Visser, A. A., Nobre, T., Currie, C. R., Aanen, D. K., and Poulsen, M. (2012). Exploring the potential for actinobacteria as defensive symbionts in fungus-growing termites. Microb. Ecol. 63, 975–985. doi: 10.1007/s00248-011-9987-4
Ye, L., Zhao, S. S., Li, Y., Jiang, S. W., Zhao, Y., Li, J. M., et al. (2017). Streptomyces lasiicapitis sp. nov., an actinomycete that produces kanchanamycin, isolated from the head of an ant (Lasius fuliginosus L.). Int. J. Syst. Evol. Microbiol. 67, 1529–1534. doi: 10.1099/ijsem.0.001756
Zhang, J., Kobert, K., Flouri, T., and Stamatakis, A. (2014). PEAR: a fast and accurate Illumina Paired-End reAd mergeR. Bioinformatics 30, 614–620. doi: 10.1093/bioinformatics/btt593
Zhang, J., Nan, X. N., Wei, C., Wang, Y. G., and He, H. (2016). Bacterial composition of the infrabuccal pocket of Camponotus japonicus Mayr. Chinese J. Appl. Entomol. 53, 164–173. doi: 10.7679/j.issn.2095-1353.2016.021
Zhang, S., Fang, H., Yin, C., Wei, C., Hu, J., and Zhang, Y. (2019). Antimicrobial metabolites produced by Penicillium mallochii CCH01 isolated from the gut of Ectropis oblique, cultivated in the presence of a histone deacetylase inhibitor. Front. Microbiol. 10:2186. doi: 10.3389/fmicb.2019.02186
Zhang, S., Yang, X., Guo, L., Xie, Z., Yang, Q., and Gong, S. (2018). Preparing Method and Application of Furamycins I and II. CN Patent: 108084126A.
Zhang, K. X., Wei, C., Nan, X. N., Wang, Y. G., and He, H. (2018). Composition and diversity of microbes in the infrabuccal pocket of Camponotus japonicus (Hymenoptera: Formicidae). Acta Entomol. Sin. 61, 686–697. doi: 10.16380/j.kexb.2018.06.007
Keywords: ant-derived actinobacteria, actinobacterial community, high throughput sequencing, antifungal activity, phytopathogenic fungi, agroactive compound
Citation: Wang Z, Yu Z, Zhao J, Zhuang X, Cao P, Guo X, Liu C and Xiang W (2020) Community Composition, Antifungal Activity and Chemical Analyses of Ant-Derived Actinobacteria. Front. Microbiol. 11:201. doi: 10.3389/fmicb.2020.00201
Received: 04 November 2019; Accepted: 28 January 2020;
Published: 11 February 2020.
Edited by:
Martin Kaltenpoth, Johannes Gutenberg University Mainz, GermanyReviewed by:
Cameron Robert Currie, University of Wisconsin-Madison, United StatesRyan Seipke, University of Leeds, United Kingdom
Copyright © 2020 Wang, Yu, Zhao, Zhuang, Cao, Guo, Liu and Xiang. This is an open-access article distributed under the terms of the Creative Commons Attribution License (CC BY). The use, distribution or reproduction in other forums is permitted, provided the original author(s) and the copyright owner(s) are credited and that the original publication in this journal is cited, in accordance with accepted academic practice. No use, distribution or reproduction is permitted which does not comply with these terms.
*Correspondence: Chongxi Liu, xizi-ok@163.com; Wensheng Xiang, xiangwensheng@neau.edu.cn
†These authors have contributed equally to this work