- 1Institute for Novel and Emerging Infectious Diseases, Friedrich-Loeffler-Institut, Greifswald-Insel Riems, Germany
- 2Tierarztpraxis Dr. Kindler, Wiesbaden, Germany
- 3Institute of Epidemiology, Friedrich-Loeffler-Institut, Greifswald-Insel Riems, Germany
Hepatitis E virus (HEV) is the main course for acute hepatitis in humans throughout the world. Human associated genotypes 1 and 2 as well as zoonotic genotypes 3 and 4 are grouped in the species Orthohepevirus A. In addition, a large variety of HEV-related viruses has been found in vertebrates including carnivores, rats, bats, and chickens, which were classified in species Orthohepevirus B-D. In 2015, partial genome sequences of a novel hepevirus were detected in feces of red foxes (Vulpes vulpes). However, no further information about virus circulation and the prevalence in foxes was available. We therefore assayed a unique panel of 880 transudates, which was collected from red foxes over 19 years (1993–2012) in Brandenburg, Germany, for HEV-related viral RNA and antibodies. Our results demonstrate a high antibody prevalence of HEV in red foxes, which oscillated annually between 40 and 100%. Molecular screening of the transudates revealed only a single RNA-positive sample, which was assigned to the carnivore species Orthohepevirus C based on the amplified partial sequence. These data indicate that the virus is circulating widely in the fox population and that foxes are carriers of this virus.
Introduction
HEV is a member of the genus Orthohepevirus of the Hepeviridae family and causes acute liver diseases in humans. The virus consists of a single stranded RNA genome of positive polarity with a length of approximately 7.2 kb, which contains three open reading frames (ORF1-3). ORF1 encodes a nonstructural and further processed protein, which includes the RNA-dependent RNA polymerase (RdRp), ORF2 encodes the viral capsid protein and ORF3 for a small phosphoprotein, which is necessary for viral release (LeDesma et al., 2019).
The human associated as well as zoonotic genotypes are grouped into the species Orthohepevirus A, which includes a total of 8 genotypes, originating from pig, wild boar, rabbit, and camel species. Orthohepevirus B consists of avian hepatitis E virus species causing the “splenomegaly syndrome” as well as the “big liver and spleen disease” in poultry, whereas Orthohepevirus C viruses were isolated from rodents (rats voles and shrew) and carnivores (such as ferrets, mink and foxes). HEV from bats are classed in the species Orthohepevirus D. Finally, fish-related HEV belongs to genus Piscihepevirus (Spahr et al., 2018).
To determine and quantify the circulation of HEV in the animal population, numerous monitoring studies have been performed in Europe in the past. Based on serological and molecular results, pigs and wild boars are considered as the main reservoirs of the virus and a potential source of zoonotic transmissions (Van der Poel, 2014). In Germany, activities mainly focused on zoonotic genotype 3 (species Orthohepevirus A) found in pig, wild boar, deer, and wild rabbits. The resulting seroprevalences ranged from 1% in deer (Neumann et al., 2016), 35–37% in rabbits (Hammerschmidt et al., 2017; Ryll et al., 2018), 41% in wild boar (Schielke et al., 2009) up to 100% in some pig holdings (Dremsek et al., 2013). Molecular studies revealed that especially rabbits, wild boar, and pigs frequently carried or excreted HEV (Kaci et al., 2008; Baechlein et al., 2013; Vina-Rodriguez et al., 2015; Anheyer-Behmenburg et al., 2017; Hammerschmidt et al., 2017; Ryll et al., 2018).
Epidemiological studies in other species including rats, voles, and small carnivores (e.g. mink and ferrets) assigned HEV sequences to the Orthohepevirus C group (Raj et al., 2012; Krog et al., 2013; Ryll et al., 2017, 2019). In Norway rats from Germany, the seroprevalence varied between 14.7 and 41.2% (Johne et al., 2012), while wild carnivores like raccoons and raccoon dogs were seropositive in the range of 37 to 54% (Dähnert et al., 2018). So far, only one red fox (Vulpes vulpes) from the Netherlands carried HEV-derived RNA in feces (Bodewes et al., 2013) and sequencing suggested that the virus clustered to the Orthohepevirus C group. However, no further serological or molecular data were available for foxes, although this species is considered to be a potential virus reservoir. We therefore undertook a comprehensive HEV surveillance study with a unique panel of fox transudate samples, which were collected over 20 years (1993–2012) in the federal state of Brandenburg, Germany.
Materials and Methods
Sample Material
Fox cavity transudate samples were collected during an Echinococcus multilocularis surveillance program conducted in the German federal state of Brandenburg. Data on the hunting date, sex, age, and location were recorded for all samples (Conraths et al., 2003).
Hunting Statistics
The population density of red foxes in Brandenburg was deduced by the number of the yearly hunted foxes (Supplementary Figure S1) using the hunting index of population density (HIPD), which is calculated from number of foxes shot per km2 and per year (Bögel et al., 1974).
Serology
Transudates were analyzed by the species-independent HEV-Ab ELISA (AXIOM, Buerstadt, Germany) according to the manufacturers protocol. This commercial kit is a double-antigen sandwich ELISA, which is based on recombinant HEV ORF2 protein as antigen. It is demonstrated as a species independent assay and can detect all immunoglobulin classes (IgG, IgM, and IgA). The specificity of the assay was checked by a modified strip immunoassay recomLine HEV IgG/IgM (Mikrogen GmbH, Neuried, Germany) as well as an in-house western blot. The strip immunoassay was carried out according to the manufacturers manual, but the secondary human antibody was replaced by horseradish peroxidase (HRP) conjugated goat anti-dog IgG (Dianova GmbH, Hamburg, Germany). Positive samples exhibit significant HEV specific signal especially for C-terminal part of ORF-2 capsid protein (Supplementary Figure S2). Western blotting was performed with recombinant ORF-2 protein encompassing 239 amino acids of the HEV genotype 3 capsid protein. In short, after SDS gel electrophoresis in a 16% acrylamide gel, protein was transferred to a PVDF membrane by semi-dry electroblotting. After blocking 30 min at room temperature with 5% skim milk (Difco) in PBS/0.1% Tween 20, the membranes were incubated for 1 h at RT with corresponding fox transudates in 1:100 dilution. The membranes were then washed three times for 10 min in PBS/0.1% Tween 20, and a secondary HRP-conjugated goat anti-dog antibody (Dianova GmbH, Hamburg, Germany) diluted 1:1,000 was incubated for 1 h. After a second washing step, the proteins were visualized by chemiluminescence detection with ECL substrate (ThermoFisher) and VersaDoc Imaging system (Supplementary Figure S3).
RNA Isolation and Molecular Analysis
RNA was extracted by QIAmp Viral Mini Kit (Qiagen GmbH, Hilden, Germany) as instructed by the manufacturer and HEV-specific genome copies amplified by a nested real-time RT-PCR protocol using primers targeting the RNA-dependent RNA polymerase (RdRp) region (Vina-Rodriguez et al., 2015; Hammerschmidt et al., 2017). In brief, reverse transcription was carried out with Superscript® III Reverse Transcriptase (Thermo Fisher Scientific Inc., USA) and primers HEV.RdRp_F1 (5′-TCGCGCATCACMTTYTTCCARAA-3′) and HEV.RdRp_R1 (5′-GCCATGTTCCAGACDGTRTT CCA-3′) according to the manufacturers’ protocol, followed by 40 cycles of 20 s denaturation at 95°C, 30 s annealing at 50°C, and 1 min elongation at 72°C, finishing with 7 min at 72°C. Subsequently, a nested PCR followed using Maxima SYBR Green/Fluorescein qPCR Master Mix Kit (Thermo Fisher Scientific Inc., USA) and primers HEV.RdRp_F2b (5′-GTGCTCTGTTTGGCCCNTGG TTYMG-3′) and HEV.RdRp_R2 (5′-CCAGGCTCACCR GARTGYTTCTTCCA-3′) according to an established protocol (denaturation for 10 min at 95°C and 40 cycles of 15 s denaturation at 95°C, 30 s annealing at 50°C, 30 s elongation at 95°C, 50°C and 30 s elongation at 95°C). Finally, a melting curve analysis was performed starting with a temperature gradient from 68 to 94°C in increments of 0.2°C. Positive samples were determined by melting peaks and amplicons were excised and subsequently sequenced (Eurofins Genomics, Germany). Standard precautions were taken to prevent PCR contamination including a closed system for PCR amplification and detection. In addition, preparation of PCR mastermix and primers, RNA-extraction, and final addition of RNA were carried out in separate laboratories.
Phylogenetic Analysis
Phylogenetic analysis was carried out with Geneious Tree Builder using Neighbor-Joining analysis. Genetic distances were calculated using the Tamura-Nei Method. Bootstrap values >70 are displayed at nodes. Phylogenetic analysis was carried out with a 280-nt fragment of the RNA-dependent RNA polymerase gene. Sequence of avian Hepatitis E virus was used as outgroup to root the tree.
Statistical Methods
A 95% confidence intervals (CI) were calculated using R (R Development Core Team, 2008) in R studio. Differences in prevalences were compared by the Fisher exact test.
Results
Eight hundred and eighty fox transudates collected from 1993 to 2012 in the federal state of Brandenburg, Germany, were analyzed using in Axiom ELISA and displayed an overall high seroprevalence of about 81% on average, which varied between 48.9 and 100% over the years (Figure 1). The exact number is depicted in Supplementary Table S1. In order to confirm the immunoreactivity against HEV independently, selected samples were tested by a strip immunoassay (Supplementary Figure S2) and an in-house western blot (Supplementary Figure S3). The spatial distribution of positive and negative samples throughout the district are displayed in an overview map (Figure 2A) and in annual maps (Figure 2B). Samples were collected from all 12 districts within the federal state of Brandenburg, but the majority of the 516 samples originated from the two districts Ostprignitz-Ruppin and Prignitz, which are located in the North-West of Brandenburg (Figure 2A, selected section).
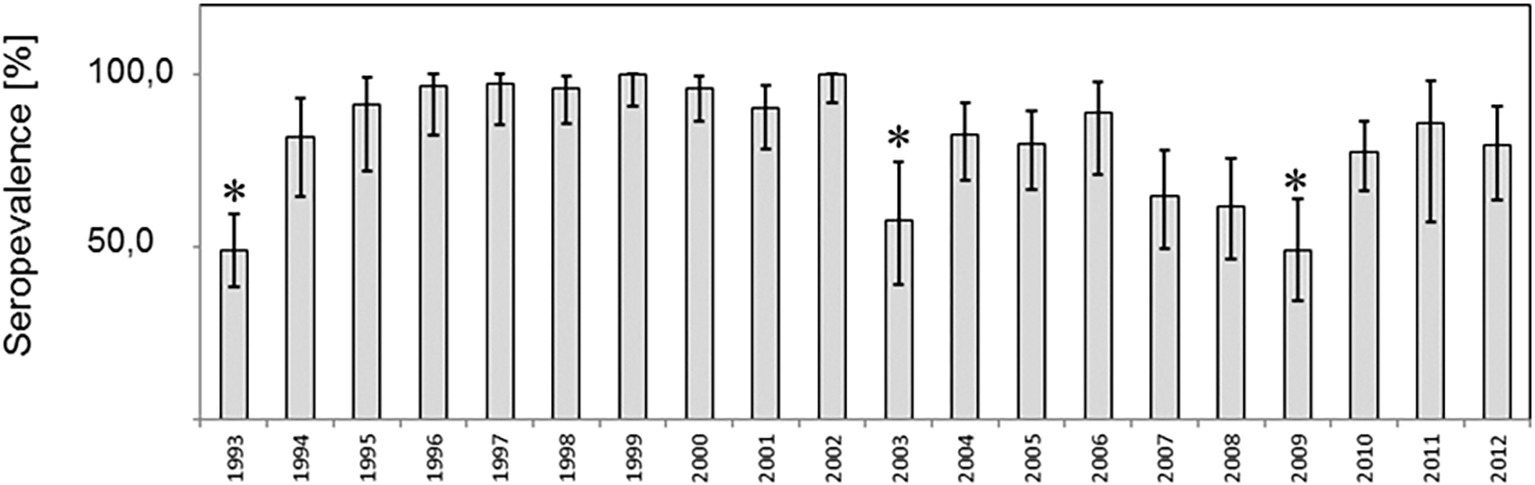
Figure 1. Prevalence of antibodies to Hepatitis E virus among red foxes in the federal state of Brandenburg, Germany. (A) Prevalence estimates per year and the respective two-sided 95% confidence intervals are shown. Differences in prevalences were compared by the Fisher exact test und significant differences (p < 0.05) indicated by asterics.
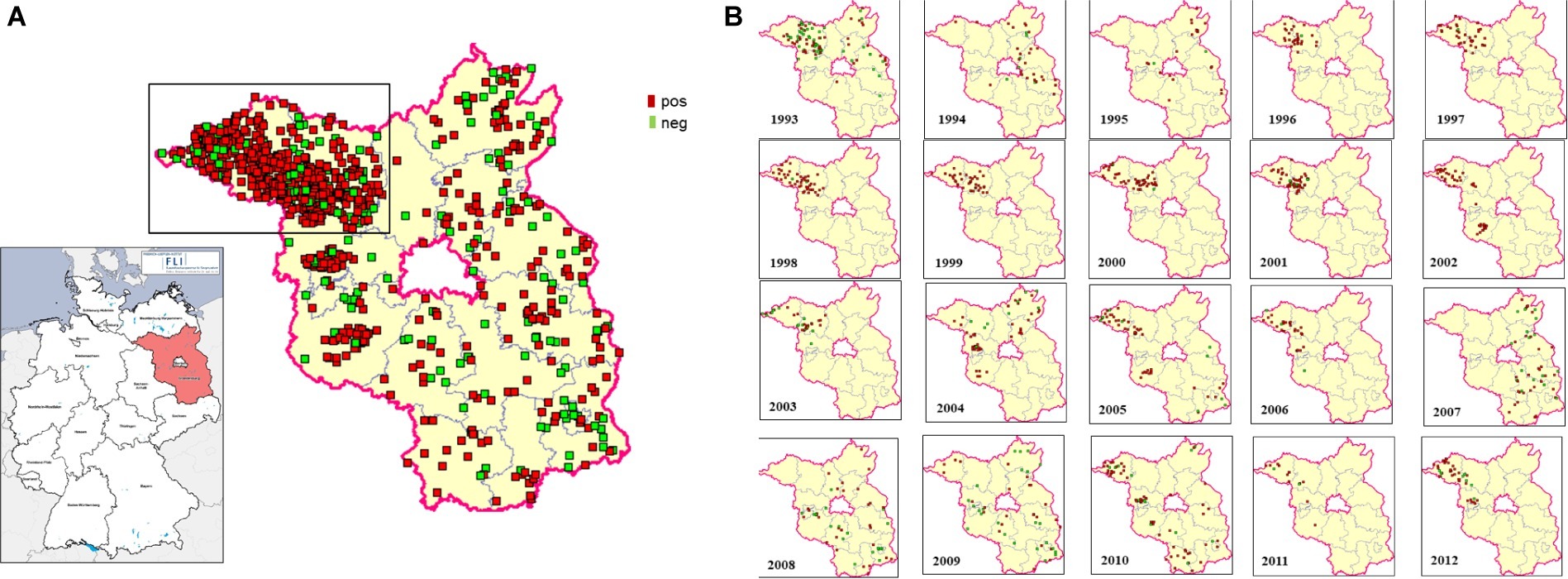
Figure 2. Spatial distribution of HEV-tested fox samples in the federal state of Brandenburg, Germany. The geographic origin of each examined fox sample is plotted on the map. The districts of Ostprignitz-Ruppin and Prignitz, from where the majority of samples were obtained, are marked. Positive samples are represented by red dots, negative samples by green dots. Overview comprising 20 years (A) and annual distributions (B).
About 52.0% of the samples were from male individuals and 32.8% from female individuals. The majority of the samples (74%) derived from adult animals (born in a previous year) and 9.2% from juvenile foxes, i.e., animals born in the hunting year (1 April to 31 March) when they were sampled (Table 1). No statistically significant age- or sex-associated differences in the seroprevalence were found (Table 1).
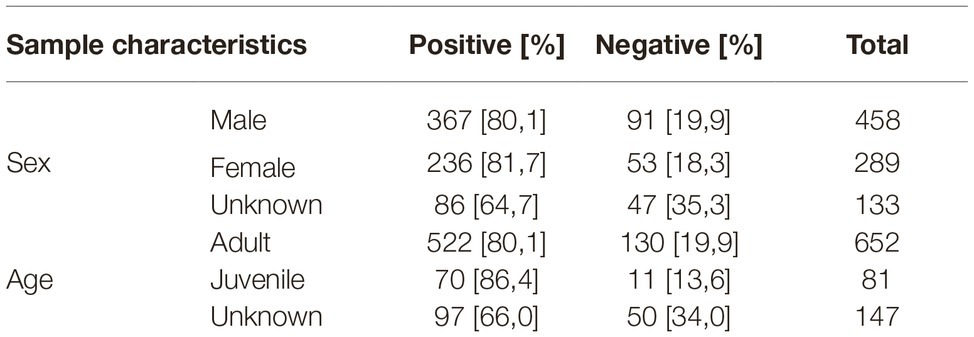
Table 1. Overview of analyzed fox samples. The age of each fox was determined as adult (born in a previous year) or juvenile (born in the year of sampling).
From 1993 to 1994, a significant increase from 48.9 to 81.1% was observed and after 10 years, a significant decline from 100 to 57.6%, followed by a significant rise from 49 to 77% 6 years later 2009. A similar finding in the seroprevalence was observed for the samples collected in 1993 and 2003 when looking only to the districts Ostprignitz-Ruppin and Prignitz (Supplementary Figure S4).
All samples were subjected to a broad-range nested RT-PCR targeting the RNA-dependent RNA-polymerase (RdRp) gene. From one sample, a partial sequence of 280 nucleotides could be recovered (accession number: MN563782), which clustered to the Orthohepevirus C group. Phylogenetic analysis revealed high identity of 82–83% to a HEV isolate from a fox from the Netherlands and to a sequence of kestrel in Hungary (Figure 3). Pairwise comparison of the corresponding 90 amino acid fragment showed sequence identity of 90 and 89%, respectively, corresponding to 9 and 12 amino acid changes (Supplementary Figure S5).
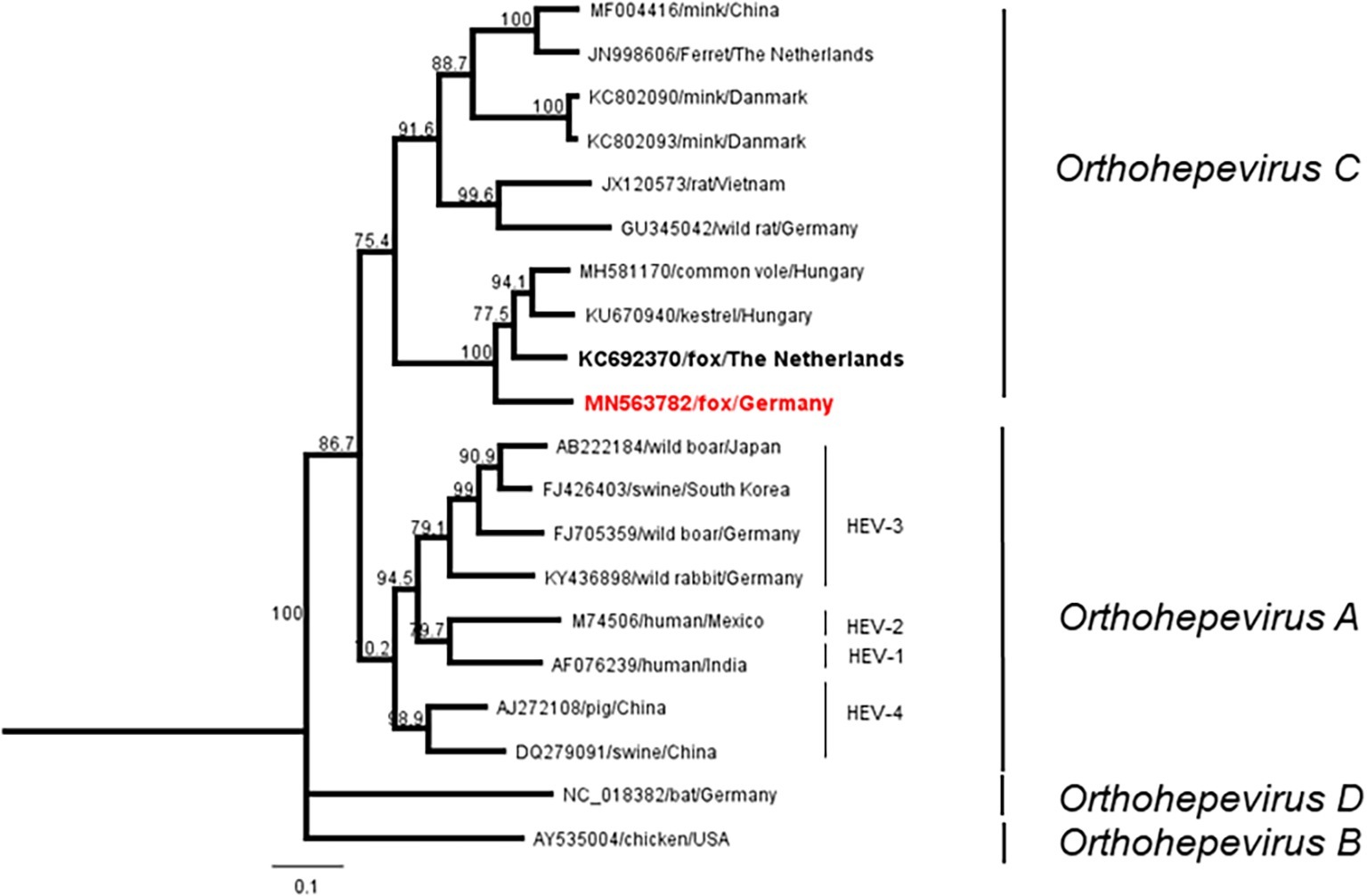
Figure 3. Neighbor-joining phylogenetic tree based on partial RdRp sequences. Red boldface indicates fox sequence from Germany (this study). Boldface displays fox sequence from the Netherlands. Scale bar indicates mean number of substitutions per site.
Discussion
In this study, we investigated nearly 900 transudate samples from foxes collected in the federal state of Brandenburg collected during a period of 20 years and found a high seroprevalence for HEV in this population. This first report on HEV antibodies in foxes indicates therefore frequent HEV infections in this wild carnivore species. Neither age nor sex effects were observed which speaks for a general infection via the oral-fecal route probably by exposure to infectious feces.
Time kinetics showed an oscillation between 40 and 100% per year and a declining prevalence in 1993, 2003, and 2009. The underlying reasons are unknown, but it seems possible that the changes may have been triggered by variation of environmental factors or changes in the social organization pattern. In general, adult foxes are solitary hunters with low contact rates, but individuals have short- and long-term relationships and a seasonal community structure, e.g., during to cooperative raising of cubs (Dorning and Harris, 2019).
Seroprevalence studies from other members of the superfamily Canidae are limited. Raccoons and raccoon dogs had a prevalence of about 53.8 and 34.3%, respectively (Dähnert et al., 2018). A disparate picture is seen in dogs where seroprevalences ranged from 0.8% in the UK to 56.6% in Germany up to 88.5% in China (Liu et al., 2009; McElroy et al., 2015; Dähnert et al., 2018).
Only a single HEV genome sequence was extracted, which can be attributed to the age of the samples and a probably short and transient viraemic phase in foxes. In general, transudate fluids and blood/serum samples contain significantly smaller amounts of viral RNA compared to feces or tissue material. The obtained sequence showed a high identity to a fox feces derived HEV sequence isolated in The Netherlands (Bodewes et al., 2013), and both constitute a potential subclade together with other species including voles and a kestrel. Both sequences cluster with sequences from a kestrel and from vole-associated HEV strains (Reuter et al., 2016; Kurucz et al., 2019) and constitute one distinct clade within the Orthohepevirus C species. This includes also sequences isolated most recently from voles in Germany (Ryll et al., 2019).
The results demonstrate endemic HEV infections in a fox population over at least 20 years. As no HEV associated clinical signs in foxes are known to date, this species my perhaps even constitute a reservoir species (Haydon et al., 2002). However, direct information about virus shedding and subsequent infection is lacking. In addition, no information about the virulence of fox HEV and any possible impact on morbidity and mortality is available so far. At least no influence on yearly population density as displayed by means of the hunting index of population density (HIPD) could be observed (Supplementary Figure S6).
Questions regarding zoonotic character of fox-derived HEV remain open due to the lack of further sequence information and life virus. In general, foxes are the most widespread predators throughout the world and have been recently recognized as potential reservoirs of zoonotic pathogens including trematodes, cestodes, and nematodes (Mackenstedt et al., 2015) as well as Babesia spp. and Theileria spp. (Najm et al., 2014). In addition, the marked tendency of foxes to establish populations in suburban and urban areas should be kept in mind, as urbanization is a driving force for the emergence of zoonotic diseases across species and a major risk factor for the transmission of such agents to humans (Hassell et al., 2017). A significant example for dispersal of a fox derived zoonosis is alveolar echinococcosis caused by Echinococcus multilocularis, which displays transmission routes similar to HEV including fecal shedding and subsequent ingestion of the pathogen (Vuitton et al., 2015).
In principle, members of the species Orthohepevirus C may have zoonotic potential as illustrated by a rat HEV isolate that induced a persistent infection in a human patient (Sridhar et al., 2018). The reservoir for rat associated HEV are invasive Rattus species like R. norvegicus and R. rattus (Ryll et al., 2018) that – analogous to red foxes – globally expand to new (sub-) urban areas and thereby provide the appropriate environment for transmission of wild life associated HEV strains to the human population.
More studies are needed to isolate fox HEV from infected animals, to provide further sequence information about fox-associated HEV and to reveal exposure and infection routes between individuals. This will help to gain a deeper understanding of HEV infection patterns and emergence scenarios at the wildlife-livestock-humans interface.
Data Availability Statement
The datasets generated for this study can be found in the GenBank BankIt submission, accession number: MN563782.
Ethics Statement
Ethical review and approval were not required for the animal study because samples were collected from hunted animals during an Echinococcus multilocularis surveillance program conducted in the German federal state of Brandenburg.
Author Contributions
ME and MG designed the research. ME, LD, and SS performed the experiments. RS, AV-R, and FC analyzed the data. FC provided the animal samples. ME and MG wrote the paper. FC revised the manuscript.
Conflict of Interest
The authors declare that the research was conducted in the absence of any commercial or financial relationships that could be construed as a potential conflict of interest.
Acknowledgments
We thank Birke Boettcher and Tobias Winterfeld for their excellent technical support, Jana Schulz for help in statistics, Patrick Wysocki for providing the map of Germany, and Dr. Kirsten Tackmann for the organization and participation of fox sampling.
Supplementary Material
The Supplementary Material for this article can be found online at: https://www.frontiersin.org/articles/10.3389/fmicb.2020.00115/full#supplementary-material
SUPPLEMENTARY TABLE S1 | Prevalence of antibodies to Hepatitis E virus among red foxes in the federal state of Brandenburg, Germany. Table compiling total number of tested fox samples. Prevalence estimates per year and the respective two-sided 95% confidence intervals are shown.
SUPPLEMENTARY FIGURE S1 | Number of hunted foxes in the federal state of Brandenburg. Source: hunting report Ministry of Rural Development, Environment and Agriculture (https://mlul.brandenburg.de/mlul/de/), German hunting organization (www.jagdverband.de).
SUPPLEMENTARY FIGURE S2 | Strip immunoassay recomLine HEV IgG/IgM. ELISA negative fox (1), positive fox (2), positive fox (3), samples. O2N N-terminal part of ORF-2 protein (HEV capsid protein, genotype 1 and 3) O2M central part of ORF-2 protein (HEV capsid protein, genotype 1). O2C C-terminal part of ORF-2 protein (HEV capsid protein, genotypes 1 and 3).
SUPPLEMENTARY FIGURE S3 | Western blot analysis of ELISA positive (lanes 1–4) and ELISA negative (lanes 5–9) fox samples.
SUPPLEMENTARY FIGURE S4 | Prevalence of antibodies to HEV in fox samples from the districts of Ostprignitz-Ruppin and Prignitz. Prevalence estimates and the respective two-sided 95% confidence intervals are shown. Differences in prevalences were compared by the Fisher exact test und significant differences (p < 0.05) indicated by asterics. No samples were received for this region 1995, 2007, and 2008.
SUPPLEMENTARY FIGURE S5 | Amino acid alignment of Fox HEV sequences. Fox HEV Germany (#MN563782), fox HEV, the Netherlands (#KC692370), common vole, Hungary (#MH581170), kestrel Hungary (#KU670940), and common vole Germany (#MK192413).
SUPPLEMENTARY FIGURE S6 | Development of the hunting index of population density (HIPD) of Brandenburg for the period 1993–2012.
References
Anheyer-Behmenburg, H. E., Szabo, K., Schotte, U., Binder, A., Klein, G., and Johne, R. (2017). Hepatitis E virus in wild boars and spillover infection in red and roe deer, Germany, 2013–2015. Emerg. Infect. Dis. 23, 130–133. doi: 10.3201/eid2301.161169
Baechlein, C., Seehusen, F., Nathues, H., Beilage, E. G., Baumgärtner, W., and Grummer, B. (2013). Molecular detection of hepatitis E virus in German domestic pigs. Berl. Munch. Tierarztl. Wochenschr. 126, 25–31. doi: 10.2376/0005-9366-126-25
Bodewes, R., van der Giessen, J., Haagmans, B. L., Osterhaus, A. D., and Smits, S. L. (2013). Identification of multiple novel viruses, including a parvovirus and a hepevirus, in feces of red foxes. J. Virol. 87, 7758–7764. doi: 10.1128/JVI.00568-13
Bögel, K., Arata, A. A., Moegle, H., and Knorpp, F. (1974). Recovery of reduced fox population in rabies control. Zentralbl. Veterinarmed. B 21, 401–412.
Conraths, F. J., Staubach, C., and Tackmann, K. (2003). Statistics and sample design in epidemiological studies of Echinococcus multilocularis in fox populations. Acta Trop. 85, 183–189. doi: 10.1016/S0001-706X(02)00215-2
Dähnert, L., Conraths, F. J., Reimer, N., Groschup, M. H., and Eiden, M. (2018). Molecular and serological surveillance of hepatitis E virus in wild and domestic carnivores in Brandenburg, Germany. Transbound. Emerg. Dis. 65, 1377–1380. doi: 10.1111/tbed.12877
Dorning, J., and Harris, S. (2019). Understanding the intricacy of canid social systems: structure and temporal stability of red fox (Vulpes vulpes) groups. PLoS One 14:e0220792. doi: 10.1371/journal.pone.0220792
Dremsek, P., Joel, S., Baechlein, C., Pavio, N., Schielke, A., Ziller, M., et al. (2013). Hepatitis E virus seroprevalence of domestic pigs in Germany determined by a novel in-house and two reference ELISAs. J. Virol. Methods 190, 11–16. doi: 10.1016/j.jviromet.2013.03.010
Hammerschmidt, F., Schwaiger, K., Dähnert, L., Vina-Rodriguez, A., Höper, D., Gareis, M., et al. (2017). Hepatitis E virus in wild rabbits and European brown hares in Germany. Zoonoses Public Health 64, 612–622. doi: 10.1111/zph.12355
Hassell, J. M., Begon, M., Ward, M. J., and Fèvre, E. M. (2017). Urbanization and disease emergence: dynamics at the wildlife-livestock-human Interface. Trends Ecol. Evol. 32, 55–67. doi: 10.1016/j.tree.2016.09.012
Haydon, D. T., Cleaveland, S., Taylor, L. H., and Laurenson, M. K. (2002). Identifying reservoirs of infection: a conceptual and practical challenge. Emerg. Infect. Dis. 8, 1468–1473. doi: 10.3201/eid0812.010317
Johne, R., Dremsek, P., Kindler, E., Schielke, A., Plenge-Bönig, A., Gregersen, H., et al. (2012). Rat hepatitis E virus: geographical clustering within Germany and serological detection in wild Norway rats (Rattus norvegicus). Infect. Genet. Evol. 12, 947–956. doi: 10.1016/j.meegid.2012.02.021
Kaci, S., Nöckler, K., and Johne, R. (2008). Detection of hepatitis E virus in archived German wild boar serum samples. Vet. Microbiol. 128, 380–385. doi: 10.1016/j.vetmic.2007.10.030
Krog, J. S., Breum, S. Ø., Jensen, T. H., and Larsen, L. E. (2013). Hepatitis E virus variant in farmed mink, Denmark. Emerg. Infect. Dis. 19, 2028–2030. doi: 10.3201/eid1912.130614
Kurucz, K., Hederics, D., Bali, D., Kemenesi, G., Horváth, G., and Jakab, F. (2019). Hepatitis E virus in common voles (Microtus arvalis) from an urban environment, Hungary: discovery of a cricetidae-specific genotype of Orthohepevirus C. Zoonoses Public Health 66, 259–263. doi: 10.1111/zph.12543
LeDesma, R., Nimgaonkar, I., and Ploss, A. (2019). Hepatitis E virus replication. Viruses 11:719. doi: 10.3390/v11080719
Liu, J., Zhang, W., Shen, Q., Yang, S., Huang, F., Li, P., et al. (2009). Prevalence of antibody to hepatitis E virus among pet dogs in the Jiang-Zhe area of China. Scand. J. Infect. Dis. 41, 291–295. doi: 10.1080/00365540902767031
Mackenstedt, U., Jenkins, D., and Romig, T. (2015). The role of wildlife in the transmission of parasitic zoonoses in peri-urban and urban areas. Int. J. Parasitol. Parasites Wildl. 4, 71–79. doi: 10.1016/j.ijppaw.2015.01.006
McElroy, A., Hiraide, R., Bexfield, N., Jalal, H., Brownlie, J., Goodfellow, I., et al. (2015). Detection of hepatitis E virus antibodies in dogs in the United Kingdom. PLoS One 10:e0128703. doi: 10.1371/journal.pone.0128703
Najm, N. A., Meyer-Kayser, E., Hoffmann, L., Herb, I., Fensterer, V., Pfister, K., et al. (2014). A molecular survey of Babesia spp. and Theileria spp. in red foxes (Vulpes vulpes) and their ticks from Thuringia, Germany. Ticks Tick Borne Dis. 5, 386–391. doi: 10.1016/j.ttbdis.2014.01.005
Neumann, S., Hackl, S. S., Piepenschneider, M., Vina-Rodriguez, A., Dremsek, P., Ulrich, R. G., et al. (2016). Serologic and molecular survey of hepatitis E virus in German deer populations. J. Wildl. Dis. 52, 106–113. doi: 10.7589/2014-12-282
R Development Core Team (2008). R: A language and environment for statistical computing. Vienna, Austria: R Foundation for Statistical Computing. Available at: http://www.R-project.org (Accessed December 12, 2019).
Raj, V. S., Smits, S. L., Pas, S. D., Provacia, L. B., Moorman-Roest, H., Osterhaus, A. D., et al. (2012). Novel hepatitis E virus in ferrets, the Netherlands. Emerg. Infect. Dis. 18, 1369–1370. doi: 10.3201/eid1808.111659
Reuter, G., Boros, Á., Mátics, R., Kapusinszky, B., Delwart, E., and Pankovics, P. (2016). Divergent hepatitis E virus in birds of prey, common kestrel (Falco tinnunculus) and red-footed falcon (F. vespertinus), Hungary. Infect. Genet. Evol. 43, 343–346. doi: 10.1016/j.meegid.2016.06.013
Ryll, R., Bernstein, S., Heuser, E., Schlegel, M., Dremsek, P., Zumpe, M., et al. (2017). Detection of rat hepatitis E virus in wild Norway rats (Rattus norvegicus) and black rats (Rattus rattus) from 11 European countries. Vet. Microbiol. 208, 58–68. doi: 10.1016/j.vetmic.2017.07.001
Ryll, R., Eiden, M., Heuser, E., Weinhardt, M., Ziege, M., Höper, H., et al. (2018). Hepatitis E virus in feral rabbits along a rural-urban transect in Central Germany. Infect. Genet. Evol. 61, 155–159. doi: 10.1016/j.meegid.2018.03.019
Ryll, R., Heckel, G., Corman, V. M., Drexler, J. F., and Ulrich, R. G. (2019). Genomic and spatial variability of a European common vole hepevirus. Arch. Virol. 164, 2671–2682. doi: 10.1007/s00705-019-04347-1
Schielke, A., Sachs, K., Lierz, M., Appel, B., Jansen, A., and Johne, R. (2009). Detection of hepatitis E virus in wild boars of rural and urban regions in Germany and whole genome characterization of an endemic strain. Virol. J. 6:58. doi: 10.1186/1743-422X-6-58
Spahr, C., Knauf-Witzens, T., Vahlenkamp, T., Ulrich, R. G., and Johne, R. (2018). Hepatitis E virus and related viruses in wild, domestic and zoo animals: a review. Zoonoses Public Health 65, 11–29. doi: 10.1111/zph.12405
Sridhar, S., Yip, C. C. Y., Wu, S., Cai, J., Zhang, A. J., Leung, K. H., et al. (2018). Rat hepatitis E virus as cause of persistent hepatitis after liver transplant. Emerg. Infect. Dis. 24, 2241–2250. doi: 10.3201/eid2412.180937
Van der Poel, W. H. (2014). Food and environmental routes of hepatitis E virus transmission. Curr. Opin. Virol. 4, 91–96. doi: 10.1016/j.coviro.2014.01.006
Vina-Rodriguez, A., Schlosser, J., Becher, D., Kaden, V., Groschup, M. H., and Eiden, M. (2015). Hepatitis E virus genotype 3 diversity: phylogenetic analysis and presence of subtype 3b in wild boar in Europe. Viruses 22, 2704–2726. doi: 10.3390/v7052704
Keywords: hepatitis E virus, foxes, infection, transudates, Orthohepevirus C
Citation: Eiden M, Dähnert L, Spoerel S, Vina-Rodriguez A, Schröder R, Conraths FJ and Groschup MH (2020) Spatial-Temporal Dynamics of Hepatitis E Virus Infection in Foxes (Vulpes vulpes) in Federal State of Brandenburg, Germany, 1993–2012. Front. Microbiol. 11:115. doi: 10.3389/fmicb.2020.00115
Edited by:
Maria Teresa Pérez-Gracia, Universidad CEU Cardenal Herrera, SpainReviewed by:
Marta Canuti, Memorial University of Newfoundland, CanadaAndrei Daniel Mihalca, University of Agricultural Sciences and Veterinary Medicine of Cluj-Napoca, Romania
Copyright © 2020 Eiden, Dähnert, Spoerel, Vina-Rodriguez, Schröder, Conraths and Groschup. This is an open-access article distributed under the terms of the Creative Commons Attribution License (CC BY). The use, distribution or reproduction in other forums is permitted, provided the original author(s) and the copyright owner(s) are credited and that the original publication in this journal is cited, in accordance with accepted academic practice. No use, distribution or reproduction is permitted which does not comply with these terms.
*Correspondence: Martin Eiden, martin.eiden@fli.de