- 1College of Life and Environmental Sciences, Hangzhou Normal University, Hangzhou, China
- 2Key Laboratory of Molecular Biology of Crop Pathogens and Insects, Ministry of Agriculture, Institute of Biotechnology, Zhejiang University, Hangzhou, China
- 3Hangzhou Academy of Agricultural Sciences, Hangzhou, China
- 4Fujian Institute of Tropical Crops, Zhangzhou, China
Phyllosticta capitalensis, Phyllosticta citricarpa, and Phyllosticta citriasiana are three very important Phyllosticta species associated with citrus. P. capitalensis is an endophyte fungus of citrus while P. citricarpa can cause black spot of citrus (e.g., oranges and mandarins). P. citriasiana was identified recently which is the causal agent of the pomelo tan spot. Here, we present the ∼34 Mb genome of P. citriasiana. The genome is organized in 92 contigs, encompassing 9202 predicted genes. Comparative genomic analyses with two other Phyllosticta species (P. citricarpa and P. capitalensis) associated with citrus was conducted to understand their evolutionary conservation and diversification. Pair-wise genome alignments revealed that these species are highly syntenic. All species encode similar numbers of CAZymes and secreted proteins. However, the molecular functions of the secretome showed that each species contains some enzymes with distinct activities. The three Phyllosticta species investigated shared a core set of 7261 protein families. P. capitalensis had the largest set of orphan genes (1991), in complete contrast to that of P. citriasiana (364) and P. citricarpa (262). Most of the orphan genes are functionally unknown, but they contain a certain number of species-specific secreted proteins. A total of 23 secondary metabolites biosynthesis clusters were identified in the three Phyllosticta species, 21 of them being highly conserved among these species while the remaining two showed whole cluster gain and loss polymorphisms or gene content polymorphisms. Taken together, our study reveals insights into the genetic mechanisms of host adaptation of three species of Phyllosticta associated with citrus and paves the way to identify effectors that function in infection of citrus plants.
Introduction
Citrus Black Spot (CBS), caused by Phyllosticta citricarpa, is an important disease of citrus, it can affect almost all grown citrus cultivars, including sweet orange (Citrus sinensis), mandarin (C. reticulata and C. unshiu), lime (C. aurantium) and lemon (C. limon) (Wang et al., 2012; Guarnaccia et al., 2019). This disease mainly causes black lesions in the fruits, making the fruits unsuitable for the fresh market. When the disease is severe, yield losses are significant due to premature fruit drop (Kotzé, 1981). CBS mainly occurs in humid subtropical regions, including Asia, Africa, South America, Australia, and most recently, Florida (Kotzé, 1981; Wang et al., 2012; Wang N.Y. et al., 2016; Miles et al., 2013; Carstens et al., 2017). As this disease was previously thought to be absent in Mediterranean countries like Spain, Italy, Israel, and Turkey, P. citricarpa was listed as an A1 quarantine pest by European Union (Paul et al., 2005; EFSA, 2014). However, a recent survey reported the presence of P. citricarpa in Italy, Malta and Portugal (Guarnaccia et al., 2017).
Besides P. citricarpa, other species of Phyllosticta have been reported to be associated with citrus. P. citriasiana, first identified to be a harmful pathogen of pomelos in 2009, was able to cause necrotic spots (tan spots) on fruit similar to those caused by P. citricarpa (Wulandari et al., 2009). By performing multi-locus phylogenetic analyses on a large number of Phyllosticta species collected in China, Wang et al. (2012) found that P. citriasiana was isolated only from pomelos, and P. citricarpa was isolated from lemons, mandarins, and oranges, but never from pomelos, indicating that the citrus-associated pathogenic Phyllosticta may have a host-related differentiation (Wang et al., 2012). In addition to P. citriasiana, many other Phyllosticta fungi have also been reported from citrus, such as P. capitalensis, P. citribraziliensis, P. citrichinaensis, P. paracapitalensis, and P. paracitricarpa (Glienke et al., 2011; Wang et al., 2012; Guarnaccia et al., 2017, 2019). Of them, P. capitalensis is the most frequently isolated species. This species has a very wide distribution and it has been isolated as endophytes from dozens of plants as well as pathogens of several plant species (Aa, 1973; Wikee et al., 2013).
As the important causal agent of CBS, P. citricarpa has been extensively studied and much information is now available on this pathogen’s population structure, reproduction mode and introduction pathways (Spósito et al., 2011; Wang N.Y. et al., 2016; Carstens et al., 2017). However, little is known about the newly identified pathogen of pomelo tan spot, P. citriasiana. In this study, we sequenced the genome of P. citriasiana, generating a high-quality reference genome assembly and provide an overview of the genome structure of this important pathogen; we also compared its genome with other two closely related Phyllosticta species associated with citrus, i.e., P. citricarpa and P. capitalensis to provide insight into their evolutionary conservation and diversification.
Materials and Methods
Fungal Strain
The Phyllosticta citriasiana strain ZJUCC200914 (deposited at China General Microbiological Culture Collection Center with the accession number CGMCC3.14344) was isolated from tan spot infected pomelos collected from Fujian Province, China (Wang et al., 2012). Cultures of strains were maintained on regular solid PDA (potato dextrose agar) or in liquid potato dextrose broth (PDB) at 25°C.
Genome Assembly and Annotation
The genomic DNA and RNA of P. citriasiana were extracted from mycelia grown in PDB as described previously (Wang et al., 2015). The genome was first surveyed through Illumina HiSeq 2500 platform using TruSeq libraries (150 bp paired-end reads, insert size of 350 bp) and then sequenced using the long reads PacBio technology. A total of 1.9 Gb PacBio data, 6.7 Gb pair-end data were generated in the sequencing process, which corresponds to ∼250 fold of sequence depth. To obtain high-quality gene calls, RNA-Seq was conducted with the same sample and 6.0 Gb Illumina paired-end reads were obtained.
To obtain the P. citriasiana genome, the PacBio reads were initially assembled using Canu 1.7 (Koren et al., 2017) and error correction was conducted using Pilon version 1.22 with the Illumina reads (Walker et al., 2014). Genome quality assessment was performed through the presence of conserved single-copy fungal genes using BUSCO version 3 (−l Pezizomycotina_odb9) (Simao et al., 2015). RNA-seq data were aligned to the genome using TopHat 2.0.9 with default parameters (Kim et al., 2013). Genome annotation was performed using the BRAKER version 1.0 pipeline combining the RNA-seq-based gene prediction and ab initio gene prediction (–fungus, –bam = accepted_hits.bam) (Hoff et al., 2015).
The genomes of P. citricarpa (accession number LOEO00000000.1) and P. capitalensis (accession number LOEN00000000.1) were downloaded from the NCBI genome database (Wang N.Y. et al., 2016). Gene model predictions of these two genomes were generated with AUGUSTUS 3.1 using the training annotation file of P. citriasiana (–genemodel = complete) (Stanke et al., 2008). Repetitive elements were annotated in all assemblies using RepeatMasker version open-4.0.71. For pairwise syntenic analysis of genome structures, the contigs of the paired genomes of Phyllosticta species were aligned with the MUMmer 3.23 package (–num, -L 1000) (Delcher et al., 2003). The average nucleotide identity was estimated using the ANI calculator (Rodriguez-R and Konstantinidis, 2016). The statistical reports for genomes were calculated by using in-house Perl scripts.
Functional Annotation of Genes
To functionally annotate gene models, we assigned protein sequence motifs to protein families (Pfam) and gene ontology (GO) terms using the Pfam and eggNOG databases (Huerta-Cepas et al., 2017; El-Gebali et al., 2018). The GO enrichment in molecular functions was produced with the dcGO database (Fang and Gough, 2013). Protein orthogroups were clustered using the orthoMCL algorithm in combination with an all-versus-all protein BLAST search (e-value < 1e-10, identity >50%) (Chen et al., 2006). The carbohydrate-active enzymes were annotated by the web-based dbCAN2 meta server (supported by > = 2 tools) (Zhang et al., 2018). To identify secreted proteins, we use SingalP 4.1 to predict the transmembrane domains (PredHel <2) and we excluded non-extracellular and GPI-anchored proteins by using targetP 1.1 (Emanuelsson et al., 2000) and GPI-SOM (Fankhauser and Mäser, 2005). Fungal secondary metabolite pathways were predicted using the online tool antiSMASH 4.0 (Blin et al., 2017). To estimate the dN/dS ratio, the orthologous proteins between P. citriasiana and the other two Phyllosticta species were first aligned. After removing the gap in the alignment, the amino acid sequences were replaced by the corresponding coding DNA sequences. The resulting codon alignment was then entered into PAML to calculate the Ka and Ks value with the Yang and Nielsen method (Yang and Nielsen, 2000; Yang, 2007).
Results and Discussion
Genome Assembly and General Features
We assembled the genome of P. citriasiana using a combination of Illumina and PacBio reads with ∼250 fold of sequence depth. The de novo assembly resulted in a genome size of 34.2 Mb assembled in 92 contigs with an N50 of ∼1 Mb. The genomes of P. citricarpa and P. capitalensis previously sequenced were utilized and annotated in this study (Wang N.Y. et al., 2016). The completeness of these three genome assemblies was estimated by BUSCO (Simao et al., 2015). We found 3095 out of 3156 (98.1%) BUSCO groups were identified in the P. citriasiana genome, indicating a high degree of completeness. Although the assembly of P. citricarpa and P. capitalensis possess a large number of contigs, the BUSCO results showed that they are around 95% completeness, suggesting that these genomes are reliable for the downstream analyses (Table 1).
The overall G + C content of P. citriasiana (51.4%) is apparently lower than that of P. citricarpa (53.1%) and P. capitalensis (54.6%). The percentage of repetitive sequences of P. citriasiana is 2.19%, around 2-fold of that of P. citricarpa (0.97%) and 7-fold of P. capitalensis (0.29%). The actual percentage of repetitive sequences on the genomes of P. citricarpa and P. capitalensis is expected to be larger as repetitive sequences are the major factor contributing to the fragmented assembly of the genomes. P. citriasiana has the lowest gene density but the longest gene length among the three species. The genome of P. citriasiana was predicted to have 9202 proteins, which is comparable with that of P. citricarpa (9083), but much lower than that (9983) of P. capitalensis genome (Table 1).
During preparing this manuscript, we noticed that a paper describing the genomic sequencing of P. citricarpa and P. capitalensis was published (Rodrigues et al., 2019). However, the general features of their genome sequences are of great difference from ours. For example, the authors predicted ∼15000 proteins for both species while our data only predicted ∼9500 ones. In that study, we found that the P. citricarpa genomic assembly consisted of 19,143 contigs with the N50 of 3049 bp and the P. capitalensis genomic assembly contains 11,080 contigs with the N50 of 4925 bp (Rodrigues et al., 2019). This means that their genome sequence was very fragmented and the incompleteness of the genome was also confirmed by their BUSCO analysis (Rodrigues et al., 2019). Thus, comparing with their genomic data, we believe that the data in this study is much better and more reliable. In the manuscript review process, a review paper focusing on identification, distribution, genomics, epidemiology and disease management of Phyllosticta species was published and provided the genomic data of six species without detailed comparison (Guarnaccia et al., 2019). The genomic data will be of great value for future studies, however, there exist some differences between their genomic features and ours in the respects of assembly length and gene numbers. Taking P. citriasiana for example, their genome was 32.7 Mb in length with 11368 predicted genes while ours was 34.2 Mb with only 9202 genes (Guarnaccia et al., 2019). This should be related to the different methods used in the genome assembly and gene prediction. Braker (the software used in this study) tends to predicted less genes than other softwares, such as Maker, but with a reliable accuracy (Hoff et al., 2015; Min et al., 2017).
Genomic Similarity
The pairwise comparison analysis based on oriented contigs reveals a high degree of genome-wide macrosynteny between P. citriasiana and the other two species (Figure 1A). However, the average nucleotide identity (95.98%) between P. citriasiana and P. citricarpa is much larger than that (81.19%) between P. citriasiana and P. capitalensis (Figure 1B), indicating that P. citriasiana is much closer to P. citricarpa and relatively far from P. capitalensis. This result coincides with the fact that P. capitalensis is an endophyte of citrus while P. citriasiana and P. citricarpa are host-specific pathogens (Wang et al., 2012; Guarnaccia et al., 2019).
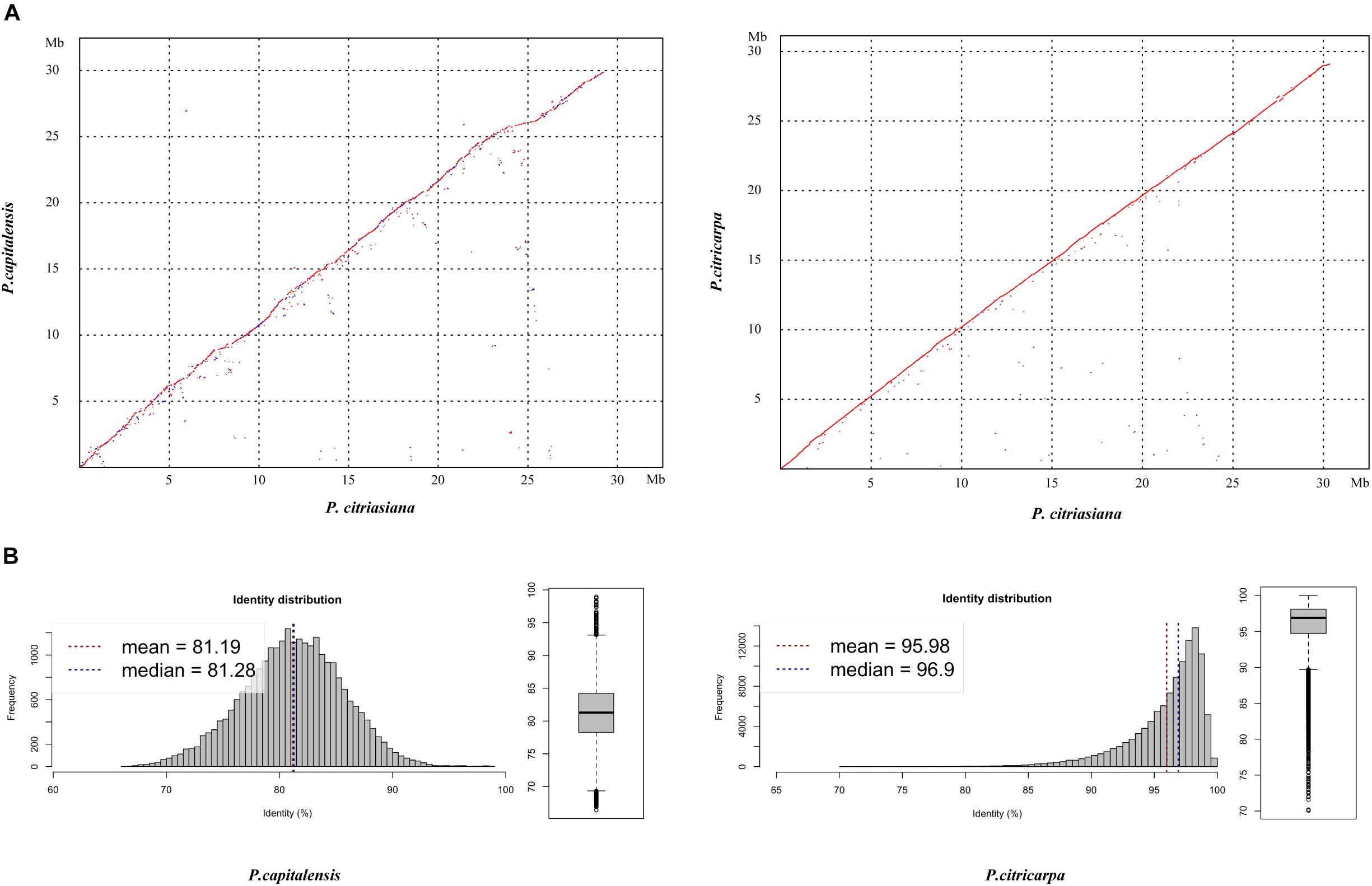
Figure 1. Genomic similarity between Phyllosticta citriasiana and the other two Phyllosticta species (P. citricarpa and P. capitalensis) associated with citrus. (A) Dotplos showing genome nucleotide alignments of P. citriasiana with P. citricarpa and P. capitalensis. Red diagonals represent alignments in the same direction, whereas blue ones suggest a reverse orientation. (B) Distribution of nucleotide identities between P. citriasiana and the other two Phyllosticta species.
Carbohydrate Active Enzymes and Secretomes
The cell wall in plant forms a complex network of different polysaccharides that includes cellulose, hemicellulose, pectin, and lignin. Carbohydrate-active enzymes (CAZymes) play important roles in the breakdown of complex carbohydrates and are responsible for the acquisition of nutrients from the plant for plant-associated fungi (Kubicek et al., 2014). A total of 267 putative CAZyme genes were identified in P. citriasiana, which includes 140 Glycoside Hydrolases (GHs), 62 Glycosyl Transferases (GTs), 48 Auxiliary Activities (AAs), 10 Carbohydrate Esterases (CEs), 6 Polysaccharide Lyases (PLs), and 1 Carbohydrate-Binding Modules (CBMs) (Supplementary Tables S1–S4). The types and numbers of CAZymes among different species of Phyllosticta are very similar (Supplementary Tables S1–S4). When compared with other species in the Dothideomycetes, P. citriasiana appears to contain a less extensive set of CAZymes, for example, Alternaria alternata has 373 CAZymes while Zymoseptoria tritici contains 324 ones (Goodwin et al., 2011; Ohm et al., 2012; Wang M. et al., 2016). It has been reported that Phyllosticta species have a relatively long time to infect citrus fruits and the scab expanded slowly in the fruit peels (Wang et al., 2012; Goulin et al., 2016), however, it is still unclear whether this phenomenon is related to the smaller number of CAZymes in these species.
Pathogens can secrete a series of proteins that are deployed to the host-pathogen interface during infection, and secretome proteins play an important role in pathogenicity (Presti et al., 2015). Approximately 5% of the total proteins (470) of P. citriasiana are predicted to be secreted (Supplementary Tables S5–S8). “Hydrolase activity” was the most abundant molecular function of the secretome, other GO terms over-represented among the secreted proteins include “hydrolase activity, acting on glycosyl bonds”, “carboxylic ester hydrolase activity”, “lipase activity”, “exopeptidase activity”, “serine hydrolase activity”, and “hydrolase activity, acting on carbon-nitrogen bonds” (Table 2). The other two Phyllosticta species contain a similar number of SPs with P. citriasiana, i.e., 465 in P. citricarpa and 491 in P. capitalensis (Supplementary Tables S5–S8). However, their GO categories showed some differences from that of P. citriasiana. The SPs of P. citricarpa were not enriched in “exopeptidase activity”, “serine hydrolase activity”, and “hydrolase activity, acting on carbon-nitrogen bonds” but was in “transferase activity, transferring hexosyl groups” (Table 2). The SPs of P. capitalensis lacks “exopeptidase activity” and “hydrolase activity, acting on carbon-nitrogen bonds” but contains “phosphatase activity”, “transferase activity, transferring hexosyl groups”, and “UDP-glycosyltransferase activity” (Table 2). These results suggested that the constitution of different Phyllosticta secretomes has changed, each species have some preferred enzymes with distinct activities.
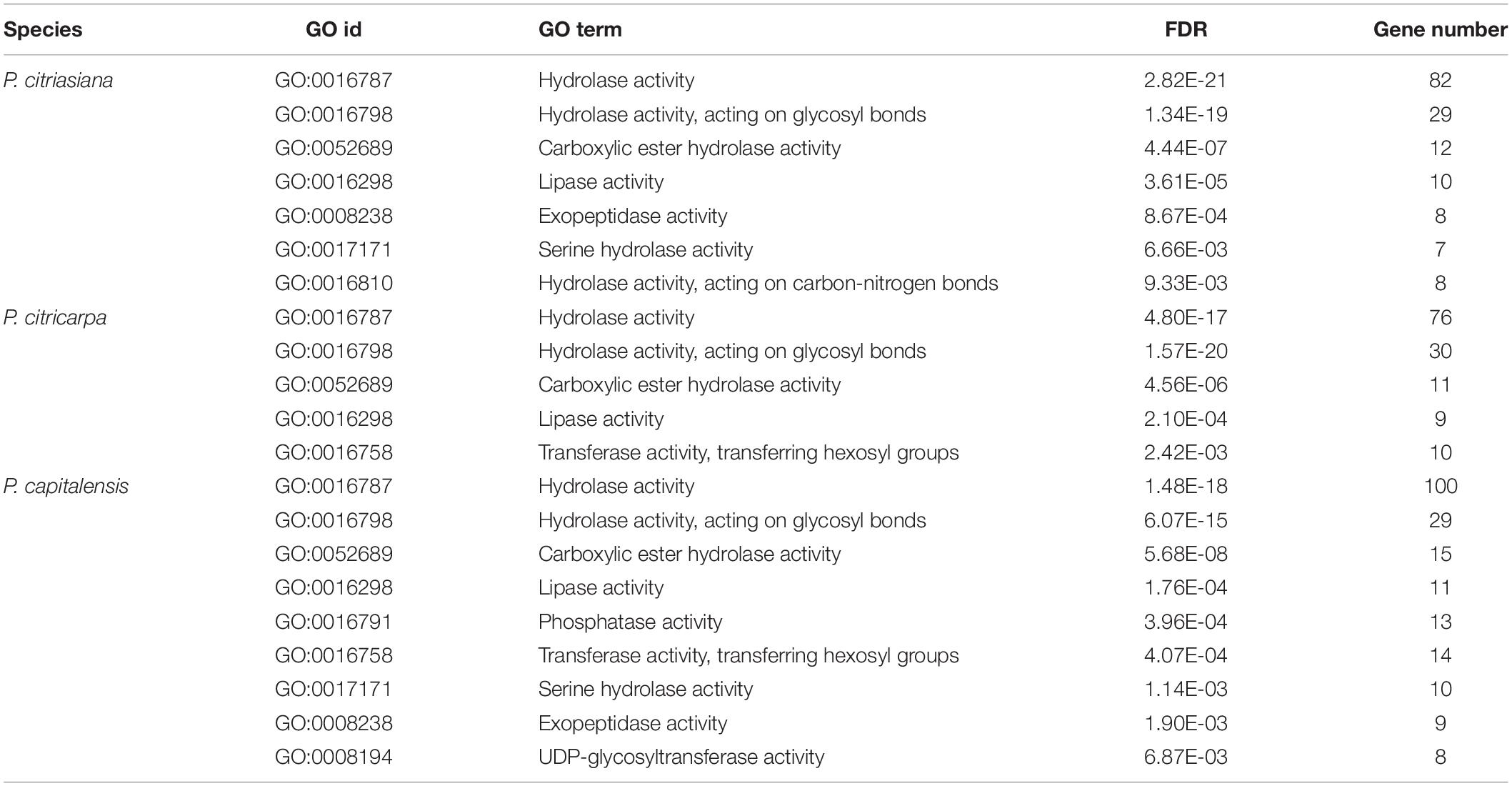
Table 2. Enriched molecular functional categories for secreted proteins of Phyllosticta species associated with citrus.
Orthologs Groups and Orphan Genes
We then searched the conservation and diversification of proteins among different Phyllosticta species from the genome-scale. The protein orthology analysis identified 7261 orthologous groups that existed in all the three Phyllosticta species, constituting the core gene set of Phyllosticta (Figure 2). To find if any protein might be under positive selection, the dN/dS ratios for predicted proteins in a pairwise comparison between P. citriasiana and the other two Phyllosticta species were calculated. However, all genes show signs of purifying selection (dN/dS < 1). 1991 genes in P. capitalensis have no orthologs in the other two species (Figure 2), suggesting that these genes might play roles in constructing the endophytic or pathogenic relationship of P. capitalensis with its hosts. P. citriasiana and P. citricarpa encoded 364 and 262 species-specific proteins, respectively (Figure 2), suggesting that these genes might be related to the host-specific pathogenicity. To know the functions of the genes in those three gene sets, we annotated them using the eggNOG database. However, the results showed that the majority of genes in each group encoded proteins without well-characterized domains and very few sequences can be assigned to the GO terms (Supplementary Tables S9–S12).
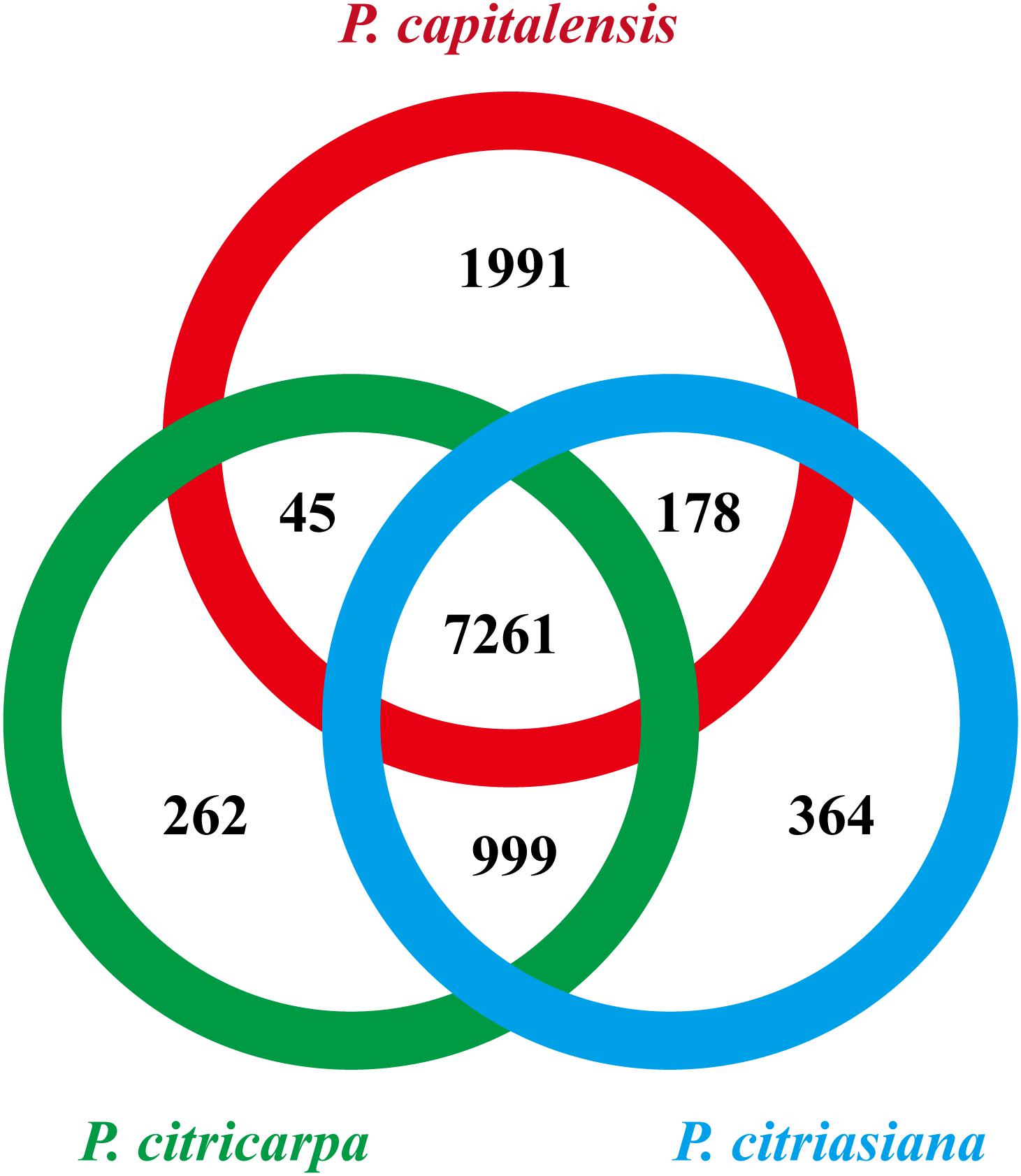
Figure 2. Numbers of orthologous groups that are unique to each isolate, specific to two isolates, and common to all three Phyllosticta isolates.
We are then curious about if the distribution of CAZymes and secreted proteins might differ among different gene sets. We found that the P. capitalensis orphan genes contain only 3 CAZyme genes which encode an AA3 family of glucose oxidase, a GT90 family of xylosyltransferase, and a CE5 family of cutinase while the other two species’ orphan genes contain no CAZyme gene (Supplementary Table S13). However, the case for the secreted proteins is much different. P. capitalensis, P. citricarpa, and P. citriasiana contain 75, 8, and 17 species-specific secreted proteins, respectively (Supplementary Table S13). These results indicate that Phyllosticta species have formed lineage-specific sets of orphan genes which might have a potential role in species diversification. Although functions of most orphans are unknown, the secreted proteins (potential effectors) are likely the essential factors of host specialization and they might be good candidates for future functional characterization in distinct Phyllosticta species.
Secondary Metabolite Gene Clusters
We identified 23 secondary metabolites (SM) biosynthesis clusters in the three Phyllosticta species (Supplementary Table S14). These clusters are comprised of 3 NRPS clusters, 5 PKS clusters, 4 terpene clusters, 1 terpene-NRPS cluster, 1 PKS-NRPS cluster, and 9 clusters do not fit into any category (Supplementary Table S14). Of them, cluster C9 contains all Alternaria solani genes involved in alternapyrone synthesis, suggesting that these Phyllosticta species have the potential to synthesize alternapyrone or its derivatives (Fujii et al., 2005). Most SM clusters (21) are well conserved among the three Phyllosticta species while 2 SM clusters of them showed whole cluster gain and loss polymorphisms or gene content polymorphisms. Cluster C7 was present in P. citricarpa and P. citriasiana but absent from P. capitalensis, indicating that this cluster might be lost in P. capitalensis or gained in the common ancestor of P. citricarpa and P. citriasiana. Meanwhile, Cluster C7 in P. citricarpa possesses another 3 genes while P. citriasiana contains a ∼11 Kb region encoding no proteins, showing gene content polymorphisms (Figure 3). SM cluster C23 showed two gene content polymorphisms. One is that the P. capitalensis has an additional four genes between orthologous gene OG0649 and OG0648. The other is P. citriasiana lost two genes, of which gene OG7537 encodes the backbone of this cluster (Figure 3). A following tBLASTn analysis against the P. citriasiana genome confirmed the loss of these two genes. This gene content polymorphism was most likely generated through a genomic deletion event, rendering the SM gene cluster non-functional.
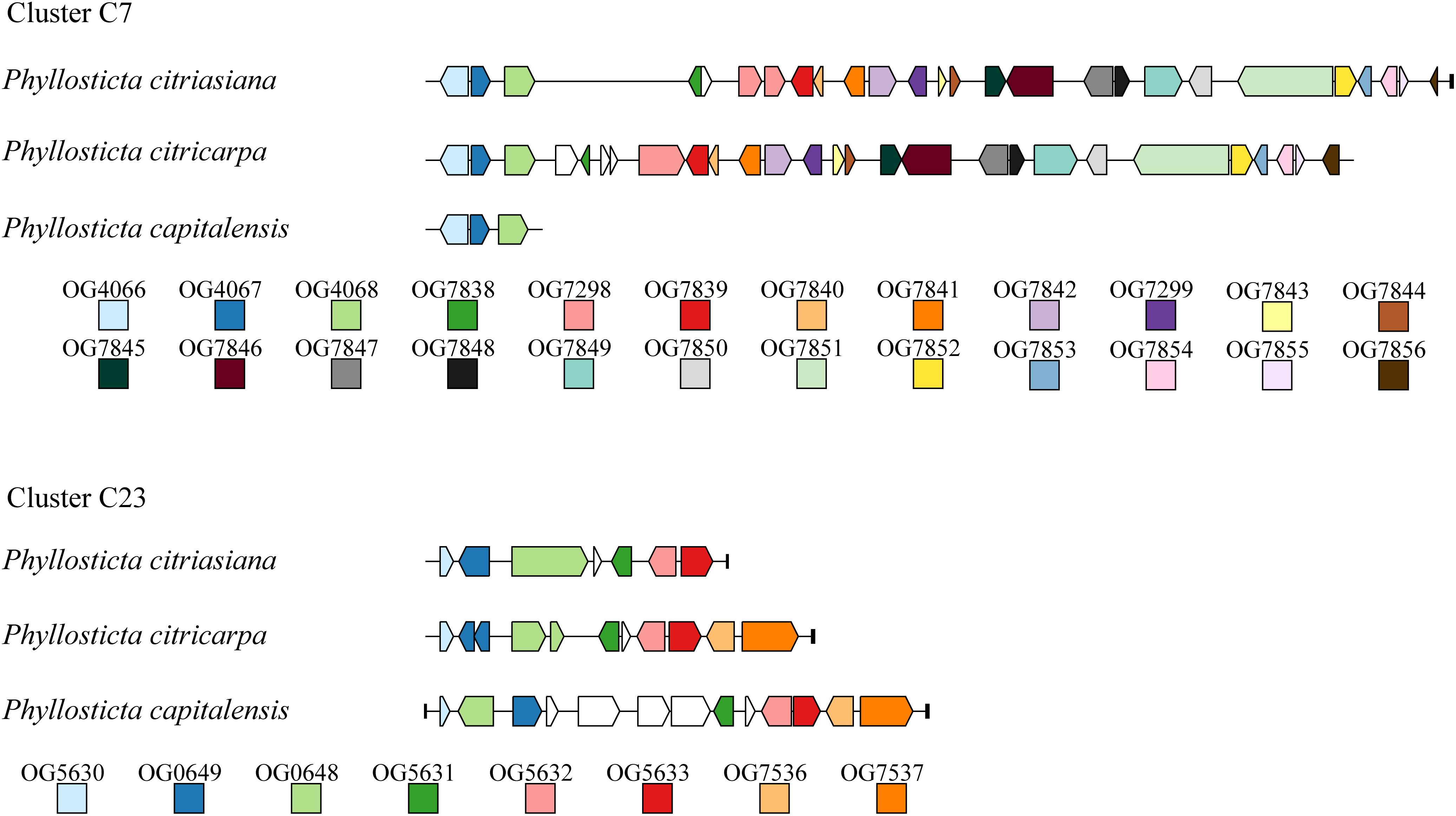
Figure 3. Structural variations of secondary metabolic (SM) gene cluster C7 and C23 among Phyllosticta species associated with citrus. For each SM cluster, ortholog among different species are marked with the same color. Genes marked by white lack orthologs in other species. The short black vertical line indicates the end of the contig.
Secondary metabolites, especial fungal toxins, are believed to be involved in the pathogenicity of many plant pathogenic fungal species and can be described as potential virulence factors. Previously, a handful of secondary metabolites from the citrus pathogen P. citricarpa were identified and characterized. Of them, a new dioxolanone, phenguignardic acid butyl ester, showed low phytotoxic activity in citrus leaves and fruits (at a dose of 100 μg) (Savi et al., 2019). However, the involvement of this compound in the formation of citrus black spot disease needs to be further addressed. In this study, we observed the major structural variation of two SM clusters among different Phyllosticta species, therefore, distinct corresponding metabolites are expected. However, if they are involved in the host specialization are not known. So, future investigations and elucidations of secondary metabolic mechanisms in Phyllosticta species and their functions involved in plant-fungal interactions will be of great significance.
Conclusion
Phyllosticta capitalensis, P. citricarpa, and P. citriasiana are three very important Phyllosticta species associated with citrus (Guarnaccia et al., 2019). P. capitalensis is an endophyte fungus of citrus while P. citricarpa can cause black spot of citrus (e.g., oranges and mandarins) (Kotzé, 1981; Wang et al., 2012). P. citriasiana was identified recently which is the causal agent of the pomelo tan spot (Wulandari et al., 2009; Wang et al., 2012). So far, a systematically comparative genomics analysis among these species is absent. Our results showed that large-scale genome synteny exists and more than 65% of orthologs groups were shared among these species. The similarity between P. citricarpa and P. citriasiana is more striking with the mean nucleotide identity of ∼96%. Such large scale genome conservation was expected since macrosynteny is often observed among taxa that are phylogenetically closely related (Ohm et al., 2012; Wang M. et al., 2016). Carbohydrate-active enzymes (CAZymes) is thought to be of importance for the breakdown of plant cell wall and are responsible for the acquisition of nutrients from the plant for plant-associated fungi (Kubicek et al., 2014). However, the types and numbers of CAZymes among different species of Phyllosticta are very similar. The content of CAZymes seems to be unrelated with host specialization. Although the number of secreted proteins of different Phyllosticta species is also very similar, the molecular function of different Phyllosticta secretomes has changed, and each species has some preferred enzymes with distinct activities. Effector genes usually evolve rapidly in response to selection pressure exerted by the host, causing the functional diversification in closely related species (Presti et al., 2015). Moreover, sets of orphan genes were detected for each species. The majority of the orphan genes encoded proteins without well-characterized domains, but they contain a certain number of species-specific secreted proteins. Hence, effector proteins should remain the focus of future research. Finally, we observed that 2 SM clusters of Phyllosticta showed whole cluster gain and loss polymorphisms or gene content polymorphisms. These types of variation in SM gene clusters have been recorded to be important driving forces for the fungal SM gene cluster divergence (Lind et al., 2017). Therefore, distinct corresponding metabolites are expected.
Deciphering the genetic basis of the transition between closely related pathogenic and endophytic fungi is crucial for a better understanding of the evolutionary history of fungal lifestyles. A previous report identifies genomic signatures indicative of an evolutionary transition from pathogenic Colletotrichum incanum to endophytic C. tofieldiae, including a narrowed repertoire of secreted effector proteins and expanded secondary metabolism-related proteins (Hacquard et al., 2016). However, these signatures are not observed in our comparative analyses of the three Phyllosticta species. An enormous number of orphan genes, a set of preferred secreted proteins and variations in some SM gene clusters are the main features for the endophytic P. capitalensis to be distinct from the pathogenetic P. citricarpa and P. citriasiana. It is commonly known that P. capitalensis is an endophyte in a wide range of hosts as well as a weak pathogen of several plant species (Aa, 1973; Wikee et al., 2013), thus, the P. capitalensis genome is speculated to include different forms of interaction. Future investigation of the population genomes of P. capitalensis might provide more insights into the underlying mechanism.
Taken together, we sequenced the genome of P. citriasiana, the causal agent of the pomelo tan spot, generating a high-quality reference genome assembly and provide an overview of the genome structure of this important pathogen. We performed comparative genomics analysis to reveal overall high similarities in sequence identity and gene content among P. citriasiana, P. citricarpa, and P. capitalensis. Our data also highlighted several striking differences in the constitution of secretomes, species-specific genes, and secondary metabolite gene clusters. However, it is yet to be determined how a Phyllosticta species emerged as a pathogen of alternate hosts. These data would be valuable in the future investigation of the driving forces of fungal host switch, in population genomic studies for identification of haplotypes and alleles, and in identifying effectors that may function in infection of citrus plants.
Data Availability Statement
The assembled Phyllosticta citriasiana genome has been deposited in GenBank under the accession number QOCM00000000. All the annotation data generated in this study have been deposited on the figshare repository at https://doi.org/10.6084/m9.figshare.9178061.
Author Contributions
MW and HL conceived the study. All authors analyzed the data and reviewed the manuscript. MW wrote the manuscript.
Funding
This study was supported by the National Key Research and Development Program of China (2017YFD0202000), the China Agriculture Research System (CARS-26), and basic special funds for public welfare research institutes in Fujian Province (2019R1010-4).
Conflict of Interest
The authors declare that the research was conducted in the absence of any commercial or financial relationships that could be construed as a potential conflict of interest.
Supplementary Material
The Supplementary Material for this article can be found online at: https://www.frontiersin.org/articles/10.3389/fmicb.2019.02979/full#supplementary-material
Footnotes
References
Blin, K., Wolf, T., Chevrette, M. G., Lu, X., Schwalen, C. J., Kautsar, S. A., et al. (2017). antiSMASH 4.0-improvements in chemistry prediction and gene cluster boundary identification. Nucleic Acids Res. 45, W36–W41. doi: 10.1093/nar/gkx319
Carstens, E., Linde, C., Slabbert, R., Miles, A., Donovan, N., Li, H., et al. (2017). A global perspective on the population structure and reproductive system of Phyllosticta citricarpa. Phytopathology 107, 758–768. doi: 10.1094/PHYTO-08-16-0292-R
Chen, F., Mackey, A. J., Stoeckert, C. J. Jr., and Roos, D. S. (2006). OrthoMCL-DB: querying a comprehensive multi-species collection of ortholog groups. Nucleic Acids Res. 34, D363–D368.
Delcher, A. L., Salzberg, S. L., and Phillippy, A. M. (2003). Using MUMmer to identify similar regions in large sequence sets. Curr. Protoc. Bioinformatics 10:Unit10.3. doi: 10.1002/0471250953.bi1003s00
EFSA (2014). Scientific opinion on the risk of Phyllosticta citricarpa (Guignardia citricarpa) for the EU territory with identification and evaluation of risk reduction options. EFSA J. 12:3557. doi: 10.2903/j.efsa.2014.3557
El-Gebali, S., Mistry, J., Bateman, A., Eddy, S. R., Luciani, A., Potter, S. C., et al. (2018). The Pfam protein families database in 2019. Nucleic Acids Res. 47, D427–D432. doi: 10.1093/nar/gky995
Emanuelsson, O., Nielsen, H., Brunak, S., and Von Heijne, G. (2000). Predicting subcellular localization of proteins based on their N-terminal amino acid sequence. J. Mol. Biol. 300, 1005–1016. doi: 10.1006/jmbi.2000.3903
Fang, H., and Gough, J. (2013). DcGO: database of domain-centric ontologies on functions, phenotypes, diseases and more. Nucleic Acids Res. 41, D536–D544. doi: 10.1093/nar/gks1080
Fankhauser, N., and Mäser, P. (2005). Identification of GPI anchor attachment signals by a Kohonen self-organizing map. Bioinformatics 21, 1846–1852. doi: 10.1093/bioinformatics/bti299
Fujii, I., Yoshida, N., Shimomaki, S., Oikawa, H., and Ebizuka, Y. (2005). An iterative type I polyketide synthase PKSN catalyzes synthesis of the decaketide alternapyrone with regio-specific octa-methylation. Chem. Biol. 12, 1301–1309. doi: 10.1016/j.chembiol.2005.09.015
Glienke, C., Pereira, O. L., Stringari, D., Fabris, J., Kava-Cordeiro, V., Galli-Terasawa, L., et al. (2011). Endophytic and pathogenic Phyllosticta species, with reference to those associated with Citrus Black Spot. Persoonia 26, 47–56. doi: 10.3767/003158511X569169
Goodwin, S. B., M’barek, S. B., Dhillon, B., Wittenberg, A. H., Crane, C. F., Hane, J. K., et al. (2011). Finished genome of the fungal wheat pathogen Mycosphaerella graminicola reveals dispensome structure, chromosome plasticity, and stealth pathogenesis. PLoS Genet. 7:e1002070. doi: 10.1371/journal.pgen.1002070
Goulin, E. H., Savi, D. C., Petters, D. A. L., Kava, V., Galli-Terasawa, L., Silva, G. J., et al. (2016). Identification of genes associated with asexual reproduction in Phyllosticta citricarpa mutants obtained through Agrobacterium tumefaciens transformation. Microbiol. Res. 192, 142–147. doi: 10.1016/j.micres.2016.06.010
Guarnaccia, V., Gehrmann, T., Silva-Junior, G. J., Fourie, P. H., Haridas, S., Vu, D., et al. (2019). Phyllosticta citricarpa and sister species of global importance to Citrus. Mol. Plant Pathol 20, 1619–1635. doi: 10.1111/mpp.12861
Guarnaccia, V., Groenewald, J. Z., Li, H., Glienke, C., Carstens, E., Hattingh, V., et al. (2017). First report of Phyllosticta citricarpa and description of two new species, P. paracapitalensis and P. paracitricarpa, from citrus in Europe. Stud. Mycol. 87, 161–185. doi: 10.1016/j.simyco.2017.05.003
Hacquard, S., Kracher, B., Hiruma, K., Münch, P. C., Garrido-Oter, R., Thon, M. R., et al. (2016). Survival trade-offs in plant roots during colonization by closely related beneficial and pathogenic fungi. Nat. Commun. 7:11362. doi: 10.1038/ncomms11362
Hoff, K. J., Lange, S., Lomsadze, A., Borodovsky, M., and Stanke, M. (2015). BRAKER1: unsupervised RNA-Seq-based genome annotation with GeneMark-ET and AUGUSTUS. Bioinformatics 32, 767–769. doi: 10.1093/bioinformatics/btv661
Huerta-Cepas, J., Forslund, K., Coelho, L. P., Szklarczyk, D., Jensen, L. J., von Mering, C., et al. (2017). Fast genome-wide functional annotation through orthology assignment by eggNOG-mapper. Mol. Biol. Evol. 34, 2115–2122. doi: 10.1093/molbev/msx148
Kim, D., Pertea, G., Trapnell, C., Pimentel, H., Kelley, R., and Salzberg, S. L. (2013). TopHat2: accurate alignment of transcriptomes in the presence of insertions, deletions and gene fusions. Genome Biol. 14, 2013–2014. doi: 10.1186/gb-2013-14-4-r36
Koren, S., Walenz, B. P., Berlin, K., Miller, J. R., Bergman, N. H., and Phillippy, A. M. (2017). Canu: scalable and accurate long-read assembly via adaptive k-mer weighting and repeat separation. Genome Res. 27, 722–736. doi: 10.1101/gr.215087.116
Kotzé, J. M. (1981). Epidemiology and control of citrus black spot in south-Africa. Plant Dis. 65, 945–950.
Kubicek, C. P., Starr, T. L., and Glass, N. L. (2014). Plant cell wall–degrading enzymes and their secretion in plant-pathogenic fungi. Annu. Rev. Phytopathol. 52, 427–451. doi: 10.1146/annurev-phyto-102313-045831
Lind, A. L., Wisecaver, J. H., Lameiras, C., Wiemann, P., Palmer, J. M., Keller, N. P., et al. (2017). Drivers of genetic diversity in secondary metabolic gene clusters within a fungal species. PLoS Biol. 15:e2003583. doi: 10.1371/journal.pbio.2003583
Miles, A. K., Tan, Y. P., Tan, M. K., Donovan, N. J., and Ghalayini, A. (2013). Phyllosticta spp. on cultivated Citrus in Australia. Aust. Plant Pathol. 42, 461–467. doi: 10.1007/s13313-013-0208-200
Min, B., Grigoriev, I. V., and Choi, I.-G. (2017). FunGAP: fungal genome annotation pipeline using evidence-based gene model evaluation. Bioinformatics 33, 2936–2937. doi: 10.1093/bioinformatics/btx353
Ohm, R. A., Feau, N., Henrissat, B., Schoch, C. L., Horwitz, B. A., Barry, K. W., et al. (2012). Diverse lifestyles and strategies of plant pathogenesis encoded in the genomes of eighteen dothideomycetes fungi. PLoS Pathog. 8:e1003037. doi: 10.1371/journal.ppat.1003037
Paul, I., van Jaarsveld, A. S., Korsten, L., and Hattingh, V. (2005). The potential global geographical distribution of Citrus Black Spot caused by Guignardia citricarpa (Kiely): likelihood of disease establishment in the European Union. Crop Prot. 24, 297–308. doi: 10.1016/j.cropro.2004.08.003
Presti, L. L., Lanver, D., Schweizer, G., Tanaka, S., Liang, L., Tollot, M., et al. (2015). Fungal effectors and plant susceptibility. Annu. Rev. Plant Biol. 66, 513–545. doi: 10.1146/annurev-arplant-043014-14623
Rodrigues, C. M., Takita, M. A., Silva, N. V., Ribeiro-Alves, M., and Machado, M. A. (2019). Comparative genome analysis of Phyllosticta citricarpa and Phyllosticta capitalensis, two fungi species that share the same host. BMC Genomics 20:554. doi: 10.1186/s12864-019-5911-y
Rodriguez-R, L. M., and Konstantinidis, K. T. (2016). The enveomics collection: a toolbox for specialized analyses of microbial genomes and metagenomes. PeerJ 4:e1900v1. doi: 10.7287/peerj.preprints.1900v1
Savi, D. C., Shaaban, K. A., Mitra, P., Ponomareva, L. V., Thorson, J. S., Glienke, C., et al. (2019). Secondary metabolites produced by the citrus phytopathogen Phyllosticta citricarpa. J. Antibiot. 72:306. doi: 10.1038/s41429-019-0154-3
Simao, F. A., Waterhouse, R. M., Ioannidis, P., Kriventseva, E. V., and Zdobnov, E. M. (2015). BUSCO: assessing genome assembly and annotation completeness with single-copy orthologs. Bioinformatics 31, 3210–3212. doi: 10.1093/bioinformatics/btv351
Spósito, M. B., Amorim, L., Bassanezi, R. B., Yamamoto, P. T., Felippe, M. R., and Czermainski, A. B. C. (2011). Relative importance of inoculum sources of Guignardia citricarpa on the citrus black spot epidemic in Brazil. Crop Prot. 30, 1546–1552. doi: 10.1016/j.cropro.2011.08.007
Stanke, M., Diekhans, M., Baertsch, R., and Haussler, D. (2008). Using native and syntenically mapped cDNA alignments to improve de novo gene finding. Bioinformatics 24, 637–644. doi: 10.1093/bioinformatics/btn013
Walker, B. J., Abeel, T., Shea, T., Priest, M., Abouelliel, A., Sakthikumar, S., et al. (2014). Pilon: an integrated tool for comprehensive microbial variant detection and genome assembly improvement. PLoS One 9:e112963. doi: 10.1371/journal.pone.0112963
Wang, M., Sun, X., Yu, D., Xu, J., Chung, K., and Li, H. (2016). Genomic and transcriptomic analyses of the tangerine pathotype of Alternaria alternata in response to oxidative stress. Sci. Rep. 6:32437. doi: 10.1038/srep32437
Wang, N. Y., Zhang, K., Huguet-Tapia, J. C., Rollins, J. A., and Dewdney, M. M. (2016). Mating type and simple sequence repeat markers indicate a clonal population of Phyllosticta citricarpa in Florida. Phytopathology 106, 1300–1310. doi: 10.1094/phyto-12-15-0316-r
Wang, M., Sun, X., Zhu, C., Xu, Q., Ruan, R., Yu, D., et al. (2015). PdbrlA, PdabaA and PdwetA control distinct stages of conidiogenesis in Penicillium digitatum. Res. Microbiol. 166, 56–65. doi: 10.1016/j.resmic.2014.12.003
Wang, X., Chen, G., Huang, F., Zhang, J., and Hyde, K. D. (2012). Phyllosticta species associated with citrus diseases in China. Fungal Divers. 52, 209–224. doi: 10.1007/s13225-011-0140-y
Wikee, S., Lombard, L., Crous, P. W., Nakashima, C., Motohashi, K., Chukeatirote, E., et al. (2013). Phyllosticta capitalensis, a widespread endophyte of plants. Fungal Divers. 60, 91–105. doi: 10.1007/s13225-013-0235-238
Wulandari, N., To-Anun, C., Hyde, K., Duong, L., De Gruyter, J., Meffert, J., et al. (2009). Phyllosticta citriasiana sp. nov., the cause of Citrus tan spot of Citrus maxima in Asia. Fungal Divers. 34, 23–39.
Yang, Z. (2007). PAML 4: phylogenetic analysis by maximum likelihood. Mol. Biol. Evol. 24, 1586–1591. doi: 10.1093/molbev/msm088
Yang, Z., and Nielsen, R. (2000). Estimating synonymous and nonsynonymous substitution rates under realistic evolutionary models. Mol. Biol. Evol. 17, 32–43. doi: 10.1093/oxfordjournals.molbev.a026236
Keywords: Phyllosticta citriasiana, pomelo tan spot, comparative genomics, secreted proteins, secondary metabolism
Citation: Wang M, Liu B, Ruan R, Zeng Y, Luo J and Li H (2020) Genomic Sequencing of Phyllosticta citriasiana Provides Insight Into Its Conservation and Diversification With Two Closely Related Phyllosticta Species Associated With Citrus. Front. Microbiol. 10:2979. doi: 10.3389/fmicb.2019.02979
Received: 10 August 2019; Accepted: 10 December 2019;
Published: 10 January 2020
Edited by:
Gustavo Henrique Goldman, University of São Paulo, BrazilReviewed by:
Yunzeng Zhang, Yangzhou University, ChinaChirlei Glienke, Federal University of Paraná, Brazil
Marcelo Falsarella Carazzolle, State University of Campinas, Brazil
Copyright © 2020 Wang, Liu, Ruan, Zeng, Luo and Li. This is an open-access article distributed under the terms of the Creative Commons Attribution License (CC BY). The use, distribution or reproduction in other forums is permitted, provided the original author(s) and the copyright owner(s) are credited and that the original publication in this journal is cited, in accordance with accepted academic practice. No use, distribution or reproduction is permitted which does not comply with these terms.
*Correspondence: Mingshuang Wang, mswang@hznu.edu.cn; wangmingshuang0916@126.com; Hongye Li, hyli@zju.edu.cn