- 1Tianjin Key Laboratory of Aqua-Ecology and Aquaculture, Fisheries College, Tianjin Agricultural University, Tianjin, China
- 2Institute of Tropical Bioscience and Biotechnology, Chinese Academy of Tropical Agricultural Sciences, Haikou, China
Aeromonas species often cause disease in farmed fish. In the present study, dominant bacteria were isolated from diseased crucian carp (Carassius auratus gibelio). Based on this, a bacterial isolate was tentatively named CFJY-623. This isolate was identified as Aeromonas veronii based on analysis of its morphological, physiological, and biochemical features, as well as 16S rRNA and gyrB gene sequences. Six virulence genes related to pathogenicity including aerolysin, cytotonic enterotoxins, elastase, glycerophospholipid: cholesterol acyltransferase, lipase, and serine protease were identified in this A. veronii isolate. The median lethal dosage (LD50) of the CFJY-623 isolate for crucian carp was determined as 1.31 × 107 CFU/mL. Artificial experimental infection showed that the CFJY-623 isolate caused considerable histological lesions in the fish, including tissue cell degeneration, necrosis, and inflammatory cell infiltrating. Drug sensitivity testing showed that the isolate was susceptible to aminoglycosides, carbapenemes, and nitrofurans. Exploring its growing features showed that this isolate exhibited a high level of environmental adaptability. These results provided a scientific basis for the identification of A. veronii and treatment for fish infected by this pathogen.
Introduction
Aeromonas spp. are important conditional pathogens, which often cause infection after host injury or stress response (Jeney and Jeney, 1995). These pathogens cause various infections in human beings, such as endocarditis, gastroenteritis, peritonitis, and septicemia (Janda and Abbott, 2010). They are also primary pathogens in farmed fish (Hossain et al., 2019). In recent years, more and more fish diseases are being caused by Aeromonas spp., such as A. caviae (Thomas et al., 2013), A. veronii (Zhu et al., 2016), A. salmonicida (Long et al., 2016), A. hydrophila (Chandrarathna et al., 2018), A. sobria (Yao et al., 2010) and A. bestiarum (Esteve and Alcaide, 2009). Among them, A. hydrophila had been considered to be the most harmful for aquatic animals, frequently causing haemorrhagic disease in farmed fish (Wang et al., 2017; Marinho-Neto et al., 2019). However, more recently, A. veroni have increasingly been infecting fish, with many similar symptoms and histological lesions, compared to A. hydrophila.
A. veronii, a Gram-negative, rod-shaped bacterium, was originally described by Hickman-Brenner et al. (1987). It is commonly isolated from environmental, clinical, and food samples (Ghenghesh et al., 2008). A. veronii strains have increasing been isolated from diseased fish (Hoai et al., 2019; Raj et al., 2019). The clinical symptoms of infected fish commonly comprised ulcer, fin rot/tail rot, abdominal distention, exophthalmia and hemorrhage. However, symptoms are not presented homogeneously across infected fish; different pathogenicity is observed depending on the particular bacterial isolates or strains. Thus far, there is a dearth of research exploring their characteristics such as virulence, growth features, and histological lesions.
Virulence factors are criteria for judging the virulence and toxicity of bacterial pathogens (Gao et al., 2018). Virulence factors and their synergistic effect are key contributors to the pathogenicity of A. veronii (Sun et al., 2016). These virulence factors include cytotonic enterotoxins (act, alt, ast), aerolysin (aer), polar flagella (fla), serine protease (ser), elastase (ahyB), lipase (lip), DNases (exu), glycerophospholipid: cholesterol acyltransferase (gcaT), and type III secretion system (ascV). Surveying the presence of virulence-related factors in clinical A. veronii isolates is essential to understand the pathogenesis and epidemiology (Sen and Rodgers, 2004). However, the data and information available in this respect from diseased fish is sparse.
At the same time, fish diseases caused by Aeromonas often occur when water quality changes dramatically, which thus might reflect their growth requirements (Xia et al., 2012). It is important to study the growth characteristics of the bacterial isolate in various condition such as across different pH values and temperatures to better understand what constitutes a suitable growth environment for this pathogen.
The crucian carp Carassius auratus gibelio, a subspecies of C. auratus (Rylková et al., 2010), is a typical freshwater fish in China with commercial value (Zhou et al., 2001). Recently, diseases with characteristic symptoms of hemorrhaging have occurred in cultured C. auratus gibelio in a fish farm in Tianjin, China. After isolation of the dominant bacteria from diseased crucian carp, the typical bacterial isolate was identified as A. veronii. In the present study, the results of isolation, identification, pathogenicity, virulent genes, and growing characteristics of the A. veronii isolate are described.
Materials and Methods
Moribund Fish and Bacterial Isolation
Diseased C. auratus gibelio were sampled from a freshwater fish farm in Tianjin, China. Moribund fish were taken from the pond to a laboratory at Tianjin Agricultural University. The diseased fish presented obvious clinical signs (Figure 1).
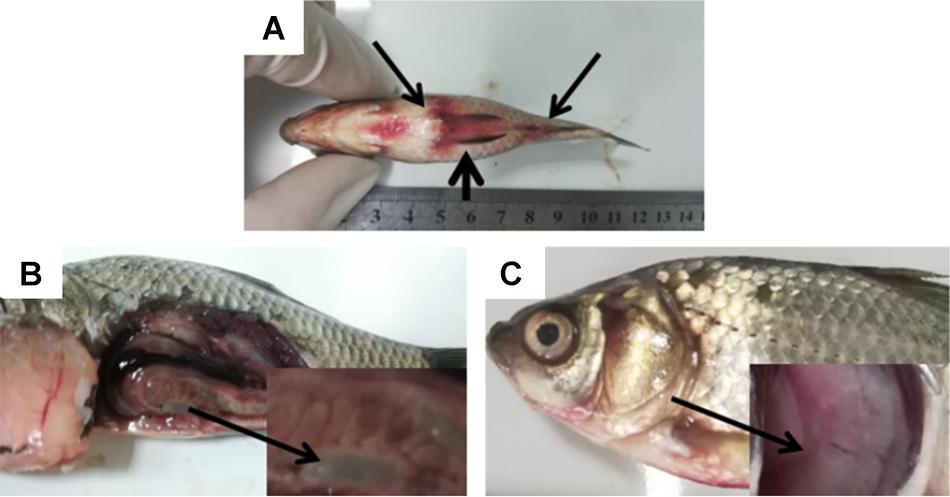
Figure 1. Clinical symptoms in Carassius auratus gibelio. (A) Abdominal distension (short arrow) and congestion of fin base (long arrow). (B) Substantial fluid in the abdominal cavity and intestine (arrow). (C) Branchial ischemia (arrow).
To elaborate, clinical observation on the diseased fish showed abdominal distension and congestion at each fin base (Figure 1A). The abdominal cavities and intestines of the morbid fish were filled with a substantial amount of ascitic fluid (Figure 1B), and the fish gills were pink or gray due to ischemia (Figure 1C). No parasites were found in the diseased fish after examination using inverted microscope. Next, the fish liver, spleen, and kidney were used as samples for virus examination. The liver cell line of the fish was used for virus isolation. The cells were cultured at 20°C in DMEM (Beijing Solarbio Science & Technology Co., Ltd., China) containing 10% fetal bovine serum. No cytopathic effect was observed in the cells, thus excluding viral infection.
Further, three typically diseased fish were used for bacterial collection and further isolation. The fish were dissected after their skin was cleaned with 75% ethyl alcohol. Their liver, spleen and kidney were used for bacterial examination. A Leria-Bertani (LB) medium (10 g/L tryptone, 5 g/L yeast extract, 10 g/L NaCl, 15 g/L agar; pH 7) was employed for bacteria isolation at 30°C for 24 h and the dominant uniform bacterial colonies developed. Some isolates were purified by streaking onto the LB plates three times. A single bacterial colony was selected and inoculated in LB broth at 30°C for 18 h, then preserved at −80°C in the LB medium containing 20% (v/v) sterile glycerol. A dominant strain was tentatively named CFJY-623.
Physiological and Biochemical Characterization
Morphological observation of the CFJY-623 isolate was carried out by Gram staining. A commercial microtest systems (Hangzhou Binhe Microorganism Reagent Co., Ltd., China) was used for measurement of physiological and biochemical characteristics, including maltose, glucose, sucrose, lactose, fructose, raffinose, xylose, melezitose, sorbose, L-rhamnose, sorbitolum, inositol, dulcitol, adonitol, urea, esculin, Voges-Proskauer test, tartrate, malonate, citrate, starch, salicin, H2S, indole, lysine decarboxylase, ornithine decarboxylase, arginine dihydrolase, and motility. Results were observed after incubation according to the manufacturer’s instructions.
16S rRNA and gyrB Gene Sequences Analysis
The genomic DNA of the CFJY-623 isolate extracted using an UNIQ-10 column DNA extraction kit (Sangon, China) was used as templates for PCR amplification. A pair of universal primer 27F: 5′-AGAGTTTGATCCTGGC TCAG-3′ and 1492R: 5′-GGCTACCTTGTTACGACTT-3′ (Jensen et al., 2002) was used for amplification of the 16S rRNA gene. A pair of primer gyrB-F: 5′-GAAGTCATCATGACCGTTCTGCA(TC)GC(TCAG)GG(TCA G)GG(TCAG)AA(AG)TT(TC)GA-3′ and gyrB-R: 5′-AGCAGGGTACGGATGTGCGAGCC(AG)TC(TCAG)AC(AG) TC(TCAG)GC(AG)TC(TCAG)GTCAT-3′ (Yamamoto and Harayama, 1995) was used for amplification of the gyrB gene. PCR amplification was performed using a Biometra TProfessional Thermocycler (TaKaRa, Japan) according to a previous report (Albarral et al., 2016). The amplified products were observed and then sequenced at Sangon (Shanghai, China). A BLAST search for sequences was carried out via the NCBI website. Phylogenetic trees were established using the neighbor-joining method in the MEGA 5.1 software package following Han et al. (2017).
Experimental Infections
Sixty healthy C. auratus gibelio, with average length 10 ± 1 cm, were kept at 25 ± 1°C with an acclimation period of 14 days. Then, these fish were randomly divided into six groups with each containing ten fish. Following Han et al. (2017), five bacterial concentrations of the CFJY-623 isolate were prepared: 1 × 105, 1 × 106, 1 × 107, 1 × 108 and 1 × 109 CFU/mL. Fish in five groups were subjected to intraperitoneal injection with the five bacterial concentrations at a dose of 200 μL/fish, respectively. The remaining control group was injected with 0.9% physiological saline. All fish were raised for 7 days at 25°C for the purpose of observing and recording pathologic symptoms. The occurrence of disease symptoms and morbidity were also recorded. Bacteria from the liver, spleen and ascites of experimentally infected fish were re-isolated. All interventions were carried out in strict accordance with the guiding principles of the Animal Experiment Ethics Committee at Tianjin Agricultural University.
Histopathological Observations
Intestine, spleen, liver, kidney and gill from the moribund fish after infection were fixed in Bouin’s fixative, dehydrated in ethanol, embedded in paraffin wax blocks, sectioned at 4 μm, and stained with hematoxylin and eosin (H&E) for histopathological observation.
Drug Sensitivity Tests
Drug sensitivity tests were performed using drug impregnated disks (Hangzhou Binhe Microorganism Reagent Co., Ltd., China), which contained 34 drugs (Table 1). Four to five disks were placed on LB plates and then inoculated with the CFJY-623 isolate at 30°C for 24 h. A reference A. veronii strain ATCC 35624 was also included.
Screening of Virulence Genes
Eleven virulence genes (act, alt, ast, aer, fla, ser, ahyB, lip, exu, gcaT, and ascV) were screened by conventional PCR. The primers used for amplification of these genes are shown in Table 2. Each PCR reaction contained 10 μL of 2 × PCR Master Mix (Tiangen Biotech, Beijing Co., Ltd., China), 1 μL of each paired primer, 2 μL of template DNA, and 11 μL of H2O. Each PCR reaction commenced with denaturation at 94°C for 2 min, then 30 cycles of amplification, and finally extension at 72°C for 10 min. Each cycle included 94°C denaturation for 30 s, annealing for 50 s and 72°C extension for 30 s. The PCR were conducted using a Biometra TProfessional Thermocycler (TaKaRa, Japan) and the results were recorded after electrophoresis on 1% agarose gel stained with ethidium bromide.
Growing Characteristics
Growing characteristics were explored according to a previous study (Han et al., 2017). The impact of pH on growth was investigated within a 3–11 value range in LB broth at 37°C; the effect of temperature was assessed at 27°C, 32°C, 37°C and 42°C in LB broth at pH 7. The CFJY-623 isolate was cultured in LB broth until the OD600 of the bacterial suspension reached the value of 1.2. This suspension (0.1 mL) was used as inoculation for further determination. The isolate was cultured at 150 rpm and growth was observed for 52 h at 600 nm every 4 h by calculating the optical density.
Results
Morphological and Biochemical Characteristics
The CFJY-623 isolate was a typically Gram-negative bacterium. It was rod-shaped, with a length of 1.5 ± 0.3 μm, and grew at 4°C and at 0–4% NaCl concentration (w/v), but not at 5% NaCl. The biochemical characteristics are listed in Table 3. The isolate was motile and urea was hydrolyzed. Further, it registered positive for malonate, the Voges-Proskauer test, and acid production from maltose, arabitol, mannose, glucose, sucrose, lactose, and fructose, but negative for raffinose, xylose, melezitose, sorbose, L-rhamnose, sorbitolum, inositol, dulcitol, adonitol, and production from indole, lysine decarboxylase, and ornithine decarboxylase.
Sequence Analysis of the 16S rRNA and gyrB Genes
The 16S rRNA gene sequence was 1,406 bp in length (Figure 2), with accession number MK392048 in GenBank. It was 100% similar to those of A. veronii biovar veronii (X60414.2), A. veronii (MG063196.1) and A. veronii (MG905287.1). The gyrB gene sequence was 1,149 bp in length (Figure 2), with accession number MK415380. It was 98–99% similar to those of A. veronii biovar sobria (MF784486.1), A. veronii (AF417626.1) and A. veronii (KJ747145.1). Phylogenetic trees were used to understand the relationship between the CFJY-623 isolate and representative Aeromonas species (Figure 3). According to phylogenetic trees established on the 16S rRNA sequence (Figure 3A) and gyrB sequence (Figure 3B), the CFJY-623 isolate is clearly grouped with a cluster of known species of A. veronii strains.
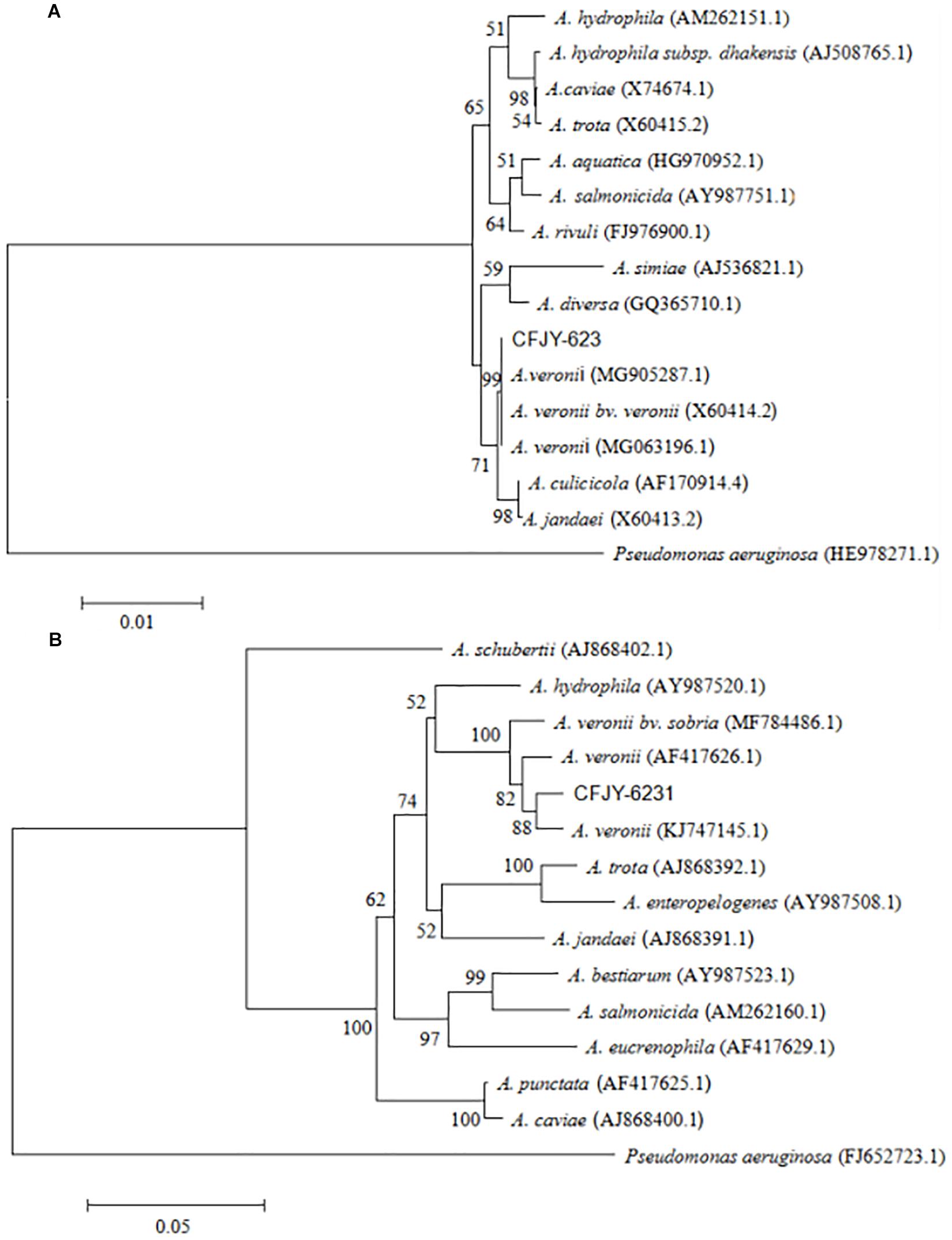
Figure 3. Unrooted neighbor-joining phylogenetic trees based on 16S rRNA gene (A) and gyrB gene (B) sequences of Aeromonas strains. Evolutionary history was inferred using the neighbor-joining method. The percentage of replicate trees in which the associated taxa clustered together based on 1,000 bootstrap replicates are shown adjacent to branches. Sequence accession numbers are in parentheses.
Experimental Infections
The mortality of fish infected with the CFJY-623 isolate are summarized in Table 4. The values in two groups infected with 1 × 109 and 1 × 108 CFU/mL bacterial suspension are both 100% while those in the other groups are all 10%. The LD50 was calculated as 1.31 × 107 CFU/mL using the Bliss method (Finney, 1985). The symptoms of the artificially infected fish were in good agreement with those of natural illness (Figure 1). Three bacterial isolates were re-isolated from the infected fish and they exhibited the same morphological and biochemical features as the CFJY-623 isolate (data not shown).
Histopathological Observations
The experimentally infected fish showed obvious pathological lesions. Multiple organs and tissues displayed extensive hemorrhaging, necrosis, and inflammatory cells infiltrating (Figure 4).
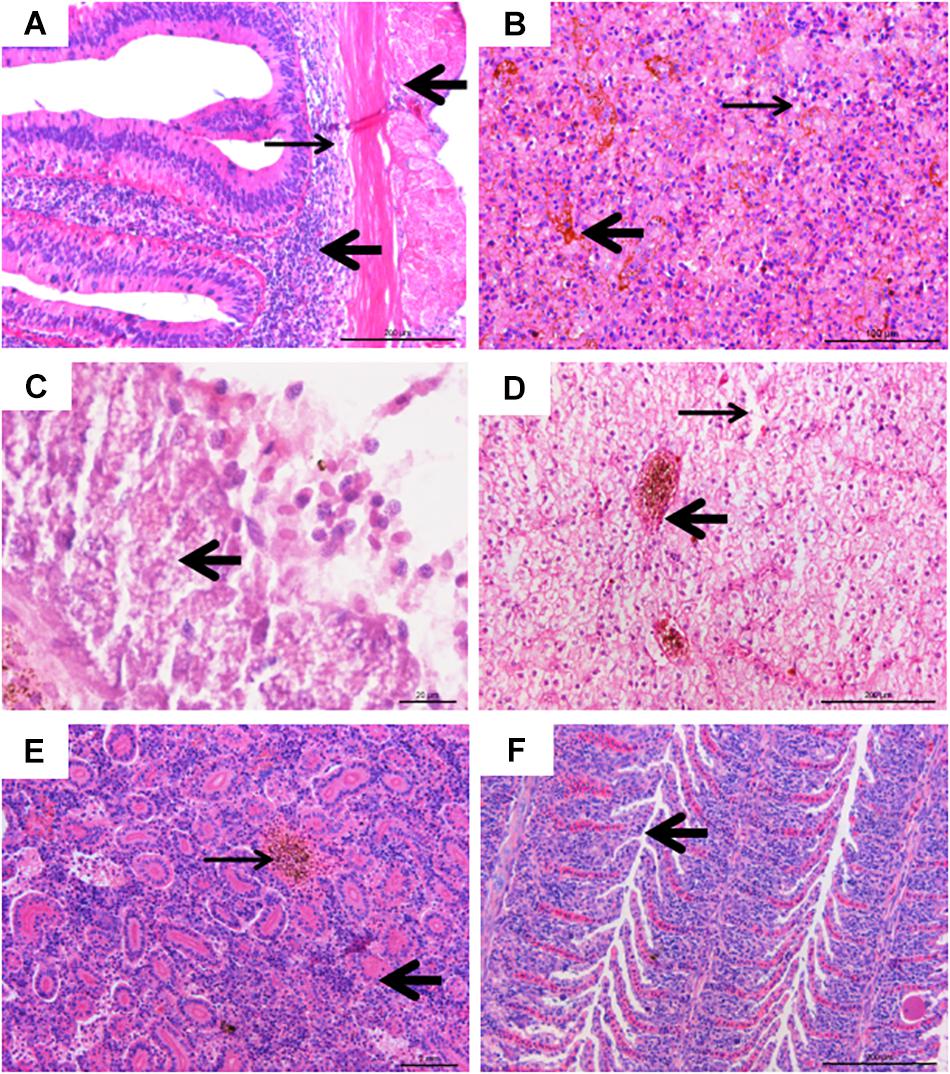
Figure 4. Histological lesions of diseased fish. (A) Inflammatory infiltration (thick arrow) and tissue loosening (thin arrow), bar = 200 μm. (B) Hemosiderin stratification in spleen tissue (thick arrow), with vacuolar degeneration (thin arrow), bar = 100 μm. (C) Islet cell necrosis and vacuolar degeneration (thick arrow), bar = 20 μm. (D) Severe intravascular congestion (thick arrow), and severe swelling of liver cells, karyolysis, cell necrosis (thin arrow), bar = 200 μm. (E) Renal tubular (thick arrow) necrosis, hematopoietic and inflammatory cell infiltration, interstitial congestion (thin arrow), bar = 200 μm. (F) Hyperplasia (arrow) in gill lamellae, with inflammatory cells infiltration, bar = 200 μm.
In the intestine, the connective tissue of submucosa was loose and inflammatory cells infiltrated the muscular layer and serosa (Figure 4A). The splenic tissue presented intensive alterations (Figure 4B), with abnormal structure and hemosiderin deposits. Extensive cell necrosis and punctate vacuolation of islet cells were found in the pancreas (Figure 4C). In the liver, severe cell swelling, karyolysis, cell necrosis, and intravascular congestion were observed (Figure 4D). In the kidney, hematopoietic and inflammatory cell infiltration, partial detachment of the tubular epithelium from the basement membrane, and pink chromatin could be observed in the tubular lumen, and there was also noticeable congestion between tubules (Figure 4E). In the gills, mononuclear inflammatory infiltrating, hyperplasia, and necrosis of epithelia, as well as mild bleeding in gill lamellae were observed (Figure 4F).
Drug Sensitivity
Results of drug sensitivity testing are listed in Table 1. The CFJY-623 isolate was susceptible to cefoperazone, neomycin, kanamycin, amikacin, meropenem, imipenem, nitrofurantoin, furazolidone, tetracycline, azithromycin, polymyxin B. Further, it was moderately susceptible to piperacillin, gentamycin, and minocycline. It was resistant to other tested antibiotics. An A. veronii type strain, ATCC 35624, was sensitive to most tested drugs except for oxacillin, ampicillin, penicillin G, amoxicillin, azithromycin, clindamycin, novobiocin, and vancomycin.
Virulence Genes
The PCR profiles of 11 virulence genes screened in this study showed that six genes (aer, alt, ahyB, gcaT, lip, and ser) was present in the CFJY-623 isolate (Figure 5).
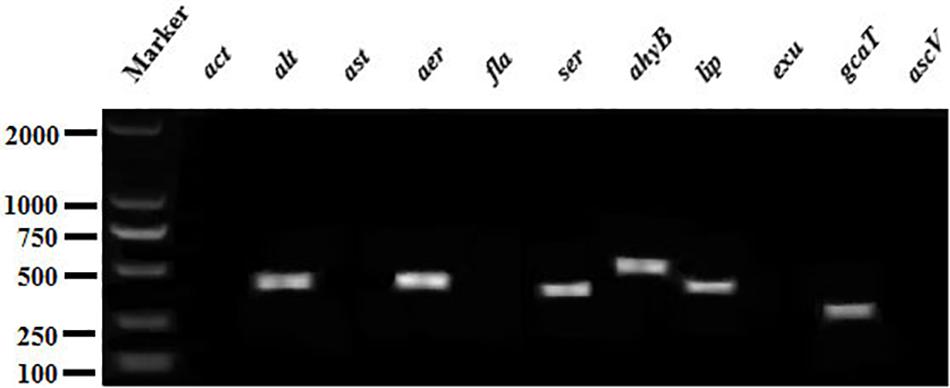
Figure 5. Agarose gel electrophoresis of the amplification products of virulent genes. Six virulent genes (aer, alt, ahyB, gcaT, lip, and ser) were present in CFJY-623.
Growing Characteristics
The growing characteristics of the CFJY-623 isolate were studied (Figure 6). As shown in Figure 6A, the growth and concentration of this isolate were maximized at pH 7. The latent phase of growth was somewhat extended at pH 5, and extended markedly at pH 10. At pH 6, 8 and 9, the growth trends were similar, and considerable final concentrations were obtained. Growth was halted at pH 2, 3, 4, and 11. In terms of temperature, as shown in Figure 6B, growth and concentration were maximized at 37°C. At 42°C growth was clearly inhibited. The growth curve drawn at pH 7 and 37°C (Figure 6C) shows the latent phase occurred between 0–4 h, the logarithmic phase was between 4–20 h, and the stationary phase was between 20–48 h. A secondary growth period was noted during 24–36 h within the stationary phase. After 48 h, the growth curve entered the aging phases.
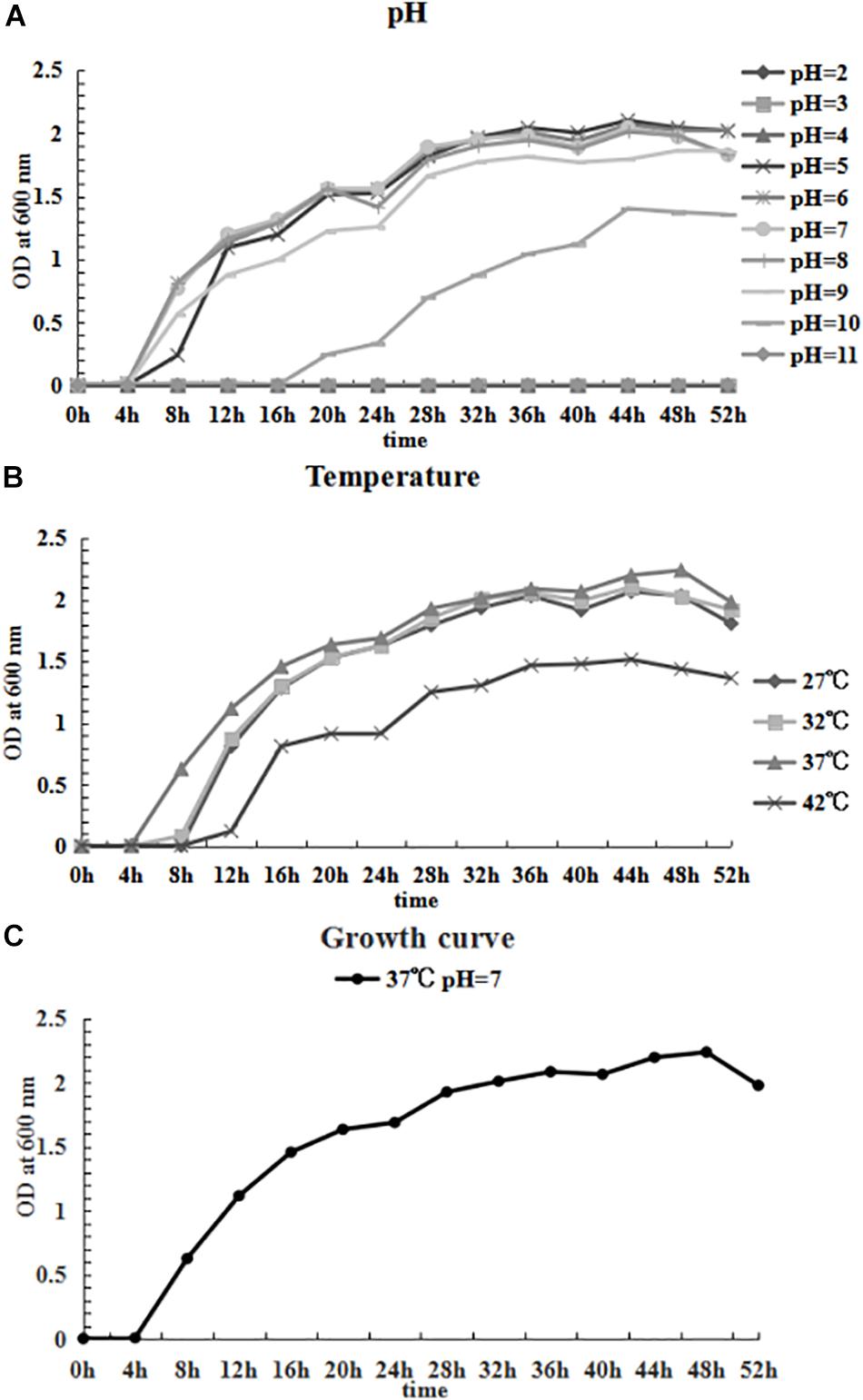
Figure 6. Growing characteristics of CFJY-623. Growth at pH 2-11 (A) and temperature 27–42°C (B). The growth curve of CFJY-623 at pH 7.0 and 37°C exhibited four distinct phases of bacterial growth (C).
Discussion
The genus Aeromonas contains a number of opportunistic pathogens causing diseases of aquatic and terrestrial animals, including human beings (Van der Marel et al., 2008). Of which, A. veronii has been described as an important pathogen of human beings as well as aquatic animals (Cui et al., 2007). Herein, an A. veronii bacterium was isolated from diseased C. auratus gibelio.
The biochemical features of the CFJY-623 isolate were basically consistent with those of the A. veronii type strain ATCC 25604 (Hickman-Brenner et al., 1987), with positive reactions to lysine and ornithine decarboxylase, negative reactions to arginine dihydrolase, and utilization of sugars including maltose, mannose, glucose, and sucrose. It is worthwhile identifying phenotypic features of bacteria; however, this becomes implausible when only classic phenotypic features are applied (Zhang et al., 2011). Therefore, molecular identification in terms of the 16S rRNA and gyrB genes was pursued.
16S rRNA sequencing is one of the most powerful and commonly used methods for identifying bacteria (Busse et al., 1996). However, Aeromonas spp. exhibit relatively low discrimination (Zhang et al., 2011) and as such their 16S rRNA gene sequences are not ideal for species identification. The gyrB gene is variable and conservative due to its inherent genetic codon usage that allows the DNA sequence to undergo more substitutions without changing the amino acid sequence (Kasai et al., 1998). This gene is thus advantageous for species identification within Aeromonas spp. Blast alignments showed that both 16S rRNA and gyrB gene sequences of the CFJY-623 isolate shared the highest identities with those of the known A. veronii strains. As expected, the phylogenetic trees built based on the sequences of the two genes showed the CFJY-623 isolate clustered with the A. veronii strains.
According to the criteria put forward by Mittal et al. (1980), a bacterial strain can be considered as moderately virulent if LD50 values are within the range of 106 to 107 CFU/g fish body weight. In our study, the LD50 of the CFJY-623 isolate was calculated as 1.31 × 107 CFU/mL which thus indicates that it is moderately virulent to C. auratus gibelio. Deaths occurring at 12 h after challenge indicated that the infection caused by this pathogen had a short incubation period. Similar phenomena in tongue soles infected with a pathogenic Shewanella algae were reported by Han et al. (2017), who noted that the short incubation period resulted in rapid disease progression.
The diseased fish infected with Aeromonas species tended to exhibit various clinical symptoms including cutaneous hemorrhage, gill congestion, hyphema, hemorrhages in fins and operculum, abdominal congestion, abdominal swelling, hepatic portal redness, and intestinal swelling. Different Aeromonas strains sometimes caused similar clinical symptoms, however sometimes dissimilarities were observed. Even the same Aeromonas species could cause divergent clinical symptoms in different fish. In this study, gray gill and intestinal edema occurred in crucian carp infected with the CFJY-623 isolate. However, these symptoms were not manifested following infection with an A. veronii isolate in a study reported by Zhang et al. (2017), but were manifested following infection with an A. militaris isolate reported by Li et al. (2017). Histopathological observation of the intestine, gill, and viscera of infected fish showed that the A. veronii isolate caused obvious histological changes, which were similar to those associated with A. hydrophila (Sun et al., 1991; Marinho-Neto et al., 2019) and A. sobrinus (Sun et al., 1991). Fish diseases caused by different Aeromonas species are difficult to accurately diagnose according to the agent responsible for clinical symptoms and histological lesions, probably because the different disease processes and pathologicities of the pathogens are complex, subsequently causing multifarious levels of infection. The results of the infection experiment also supported the viewpoint that A. veronii could probably be considered as an opportunistic pathogen in extensive circumstances (Xia et al., 2012). Further, this pathogen became more pathogenic to fish under stress or in an unfavorable culture condition.
Virulent genes are good indicators for assessing the pathogenicity of a certain microorganism (Senderovich et al., 2012). Of the 11 virulent genes related to Aeromonas detected in this study, six genes (aer, alt, ahyB, gcaT, lip and ser) were present in the CFJY-623 isolate. These genes, respectively, code the aerolysin, cytotonic enterotoxin, elastase, acyltransferase, lipase, and serine protease. These six virulent genes have been extensively utilized to investigate the potential pathogenicity of Aeromonas species (Aguilera-Arreola et al., 2007). The aer gene is found in most A. veronii isolates from cultured catfish (Nawaz et al., 2010). The coding product (aerolysin) is found in A. hydrophila and plays a key role in the pathogenesis of its infection (Abrami et al., 2003). Aerolysin and cytotoxic enterotoxin are necessary factors for the motile Aeromonads, which are considered potential foodborne pathogens (Chopra and Houston, 1999). These two virulence factors play significant roles in the occurrence of infections (Sha et al., 2002; Soler et al., 2002). It has been reported that the gcaT, lip and ser virulence factors play a coherent, integrated role in affecting the overall pathogenicity of Aeromonad infections (Lee and Ellis, 1990; Pemberton et al., 2006). The lip gene, which codes an extracellular lipase, increases the severity of Aeromonad infection by participating in alteration of the host plasma membrane (Pemberton et al., 2006). The enzyme elastase coded by the ahyB gene supports infection and colonization by damaging tissue and degrading immune proteins (Song et al., 2018). Several virulent genes present in the CFJY-623 isolate indicate that synergistic effects conferred by combinations of these genes contribute to its pathogenicity. This accords with the observation that combinations of more virulent genes commonly harbored in particularly virulent strains of Aeromonas (Li et al., 2011).
In this study, the optima for growth of the CFJY-623 isolate was similar to other strains of A. veronii (Hu et al., 2012; Shan et al., 2015). The short latent phase displayed on the growth curve of the CFJY-623 isolate might be related to a short incubation period of fish disease caused by it. Findings with respect to its growth and proliferation occurring under unsuited conditions indicate this A. veronii isolate is well adapted to the environment. After its preferred nutrients are exhausted, the bacterium probably use other nutrients to maintain growth (Hu et al., 2012). The secondary growth period during 24–36 h after entering the stationary phase indicated the isolate could use a broad-spectrum of carbon and energy sources as nutrients. The results concerning the optimal growth conditions for the A. veronii isolate provide the basis for further studies into this pathogen.
Drug sensitivity testing on the CFJY-623 isolate in this study provide a reference for when antimicrobials may be a potential treatment for A. veronii infection. This isolate has developed drug resistance to many tested drugs, especially quinolones and cephalosporins, compared with the type A. veronii strain ATCC 35624 and the A. veronii strain reported by Vila et al. (2002). In addition, although some drugs such as chloramphenicol, norfloxacin, and ofloxacin are officially banned in aquaculture, the results of the antimicrobial spectrum still contribute to the classification of this microorganism.
In sum, A. veronii CFJY-623 was isolated from diseased C. auratus gibelio. Its characteristics indicated it had considerable virulence and resulted in acute infection in fish. Since the infection caused by A. veronii have commonly occurred in a wide range of hosts in recent years, their pathogenicity and epidemiological characteristics warrant attention by the academic community.
Data Availability Statement
All data generated for this study are included in the article/supplementary material.
Ethics Statement
The animal study was reviewed and approved by the Animal Experiment Ethics Committee of Tianjin Agricultural University.
Author Contributions
FC and ZH designed the laboratory experiments. FC and XY conducted the laboratory experiments, data analyses, and drafted the manuscript. J-AX and AL assisted with the laboratory experiments, data analyses, and contributed to drafting the manuscript. JS, XH, and HS supervised the execution of laboratory experiments and data analyses, and provided a critical review of the final version of the manuscript. All authors read and approved the manuscript prior to submission.
Funding
Financial support for this study was received from the National Natural Science Foundation of China (31972840), the Scientific Program of Tianjin City (19JCZDJC34600, 18JCTPJC64700, and 18JCYBJC299000), and the Innovation Team of Tianjin Fisheries Research System (ITTFRS2017009).
Conflict of Interest
The authors declare that the research was conducted in the absence of any commercial or financial relationships that could be construed as a potential conflict of interest.
References
Abrami, L., Fivaz, M., Glauser, P. E., Sugimoto, N., Zurzolo, C., and van der Goot, F. G. (2003). Sensitivity of polarized epithelial cells to the pore-forming toxin aerolysin. Infect. Immun. 71, 739–746. doi: 10.1128/IAI.71.2.739-746.2003
Aguilera-Arreola, M. G., Hernández-Rodríguez, C., Zúñiga, G., Figueras, M. J., Garduño, R. A., and Castro-Escarpulli, G. (2007). Virulence potential and genetic diversity of Aeromonas caviae, Aeromonas veronii, and Aeromonas hydrophila clinical isolates from Mexico and Spain: a comparative study. Can. J. Microbiol. 53, 877–887. doi: 10.1139/W07-051
Albarral, V., Sanglas, A., Palau, M., Miñana-Galbis, D., and Fusté, M. C. (2016). Potential pathogenicity of Aeromonas hydrophila complex strains isolated from clinical, food, and environmental sources. Can. J. Microbiol. 62, 296–306. doi: 10.1139/cjm-2015-0466
Busse, H. J., Denner, E. B. M., and Lubitz, W. (1996). Classification and identification of bacteria: current approaches to an old problem. Overview of methods used in bacterial systematics. J. Biotechnol. 47, 3–38. doi: 10.1016/0168-1656(96)01379-X
Chandrarathna, H. P. S. U., Nikapitiya, C., Dananjaya, S. H. S., Wijerathne, C. U. B., Wimalasena, S. H. M. P., Kwun, H. J., et al. (2018). Outcome of co-infection with opportunistic and multidrug resistant, Aeromonas hydrophila and A. veronii, in zebrafish: identification, characterization, pathogenicity and immune responses. Fish Shellfish Immunol. 80, 573–581. doi: 10.1016/j.fsi.2018.06.049
Chopra, A. K., and Houston, C. W. (1999). Enterotoxins in Aeromonas-associated gastroenteritis. Microbes Infect. 1, 1129–1137. doi: 10.1016/s1286-4579(99)00202-6
Cui, H., Hao, S., and Arous, E. (2007). A distinct cause of necrotizing fasciitis: Aeromonas veronii biovar sobria. Surg. Infect. 8, 523–528. doi: 10.1089/sur.2006.046
Esteve, C., and Alcaide, E. (2009). Influence of diseases on the wild eel stock: the case of Albufera Lake. Aquaculture 289, 143–149. doi: 10.1016/j.aquaculture.2008.12.015
Finney, D. J. (1985). The median lethal dose and its estimation. Arch. Toxicol. 56, 215–218. doi: 10.1007/BF00295156
Gao, C. X., Ren, Y., Wang, Q., Zeng, W. W., Li, Y. Y., Wang, Y. Y., et al. (2018). Isolation, identification and antimicrobial susceptibility of pathogenic Aeromonas veronii isolated from grass carp. J. Anhui Agr. Univ. 45, 409–415. doi: 10.13610/j.cnki.1672-352x.20180620.019
Ghenghesh, K. S., Ahmed, S. F., El-Khalek, R. A., Al-Gendy, A., and Klena, J. (2008). Aeromonas-associated infections in developing countries. J. Infect. Dev. Ctries. 2, 81–98. doi: 10.3855/jidc.277
Han, Z. R., Sun, J. F., Lv, A. J., Sung, Y., Shi, H. Y., Hu, X. C., et al. (2017). Isolation, identification and characterization of Shewanella algae from reared tongue sole, Cynoglossus semilaevis Günther. Aquaculture 468, 356–362. doi: 10.1016/j.aquaculture.2016.10.038
Hickman-Brenner, F. W., Macdonald, K. L., Steigerwalt, A. G., Fanning, G. R., Brenner, D. J., and Farmer, J. J. (1987). Aeromonas veronii, a new ornithine decarboxylase-positive species that may cause diarrhea. J. Clin. Microbiol. 25, 900–906.
Hoai, T. D., Trang, T. T., Van Tuyen, N., Giang, N. T. H., and Van Van, K. (2019). Aeromonas veronii caused disease and mortality in channel catfish in Vietnam. Aquaculture 513:734425. doi: 10.1016/j.aquaculture.2019.734425
Hossain, S., Dahanayake, P. S., De Silva, B. C. J., Wickramanayake, M. V. K. S., Wimalasena, S. H. M. P., and Heo, G. J. (2019). Multi-drug resistant Aeromonas spp. isolated from zebrafish (Danio rerio): antibiogram, antimicrobial resistance genes and class 1 integron gene cassettes. Lett. Appl. Microbiol. 68, 370–377. doi: 10.1111/lam.13138
Hu, T. Y., Wu, T. L., Meng, Q. F., Bian, Y., Shan, X. F., Kang, Y. H., et al. (2012). Biological characteristics of Aeromonas veronii isolated from Cyprinus carpio. Chin. J. Vet. Med. 48, 18–21.
Janda, J. M., and Abbott, S. L. (2010). The genus Aeromonas: taxonomy, pathogenicity, and infection. Clin. Microbiol. Rev. 23, 35–73. doi: 10.1128/cmr.00039-09
Jeney, Z., and Jeney, G. (1995). Recent achievements in studies on diseases of common carp (Cyprinus carpio L.). Aquaculture 129, 397–420. doi: 10.1016/0044-8486(94)00283-T
Jensen, S., Bergh, Ø., Enger, Ø., and Hjeltnes, B. (2002). Use of PCR-RFLP for genotyping 16S rRNA and characterizing bacteria cultured from halibut fry. Can. J. Microbiol. 48, 379–386. doi: 10.1139/w02-033
Kasai, H., Watanabe, K., Gasteiger, E., Bairoch, A., Isono, K., Yamamoto, S., et al. (1998). Construction of the gyrB database for the identification and classification of bacteria. Genome. Inform. Ser. Workshop. Genome. Inform. 9, 13–21. doi: 10.11234/gi1990.9.13
Lee, K. K., and Ellis, A. E. (1990). Glycerophospholipid: cholesterol acyltransferase complexed with lipopolysaccharide (LPS) is a major lethal exotoxin and cytolysin of Aeromonas salmonicida: LPS stabilizes and enhances toxicity of the enzyme. J. Bacteriol. 172, 5382–5393. doi: 10.1128/jb.172.9.5382-5393.1990
Li, J., Ni, X. D., Liu, Y. J., and Lu, C. P. (2011). Detection of three virulence genes alt, ahp and aerA in Aeromonas hydrophila and their relationship with actual virulence to zebrafish. J. Appl. Microbiol. 110, 823–830. doi: 10.1111/j.1365-2672.2011.04944.x
Li, S. F., Chen, X., and Chen, Y. (2017). Distribution characteristics and virulence gene analysis of intestinal and extraintestinal Aeromonas. Chin. J. Clin. Lab. Sci. 35, 503–506. doi: 10.13602/j.cnki.jcls.2017.07.06
Long, M., Nielsen, T. K., Leisner, J. J., Hansen, L., Shen, Z. X., Zhang, Q. Q., et al. (2016). Aeromonas salmonicida subsp. salmonicida strains isolated from Chinese freshwater fish contain a novel genomic island and possible regional-specific mobile genetic elements profiles. FEMS Microbiol. Lett. 363:fnw190. doi: 10.1093/femsle/fnw190
Marinho-Neto, F. A., Claudianol, G. S., Yunis-Aguinaga, J., Cueva-Quiroz, V. A., Kobashigawa, K. K., Cruzl, N. R. N., et al. (2019). Morphological, microbiological and ultrastructural aspects of sepsis by Aeromonas hydrophila in Piaractus mesopotamicus. PLoS One 14:e0222626. doi: 10.1371/journal.pone.0222626
Mittal, K. R., Lalonde, G., Leblanc, D., Olivier, G., and Lallier, R. (1980). Aeromonas hydrophila in rainbow trout: relation between virulence and surface characteristics. Can. J. Microbiol. 26, 1501–1503. doi: 10.1139/m80-248
Nawaz, M., Khan, S. A., Khan, A. A., Sung, K., Tran, Q., Kerdahi, K., et al. (2010). Detection and characterization of virulence genes and integrons in Aeromonas veronii isolated from catfish. Food Microbiol. 27, 327–331. doi: 10.1016/j.fm.2009.11.007
Pemberton, J. M., Kidd, S. P., and Schmidt, R. (2006). Secreted enzymes of Aeromonas. FEMS Microbiol. Lett. 152, 1–10. doi: 10.1111/j.1574-6968.1997.tb10401.x
Raj, N. S., Swaminathan, T. R., Dharmaratnam, D. A., Raja, S. A., Ramraj, D., and Lal, K. K. (2019). Aeromonas veronii caused bilateral exophthalmia and mass mortality in cultured Nile tilapia, Oreochromis niloticus (L.) in India. Aquaculture 512:734278. doi: 10.1016/j.aquaculture.2019.734278
Rylková, K., Kalous, L., Šlechtová, V., and Bohlen, J. (2010). Many branches, one root: first evidence for a monophyly of the morphologically highly diverse goldfish (Carassius auratus). Aquaculture 302, 36–41. doi: 10.1016/j.aquaculture.2010.02.003
Sen, K., and Rodgers, M. (2004). Distribution of six virulence factors in Aeromonas species isolated from US drinking water utilities: a PCR identification. J. Appl. Microbiol. 97, 1077–1086. doi: 10.1111/j.1365-2672.2004.02398.x
Senderovich, Y., Ken-Dror, S., Vainblat, I., Blau, D., Izhaki, I., and Halpern, M. (2012). A molecular study on the prevalence and virulence potential of Aeromonas spp. recovered from patients suffering from diarrhea in Israel. PLoS One 7:e30070. doi: 10.1371/journal.pone.0030070
Sha, J., Kozlova, E. V., and Chopra, A. K. (2002). Role of various enterotoxins in Aeromonas hydrophila-induced gastroenteritis: generation of enterotoxin gene-deficient mutants and evaluation of their enterotoxic activity. Infect. Immun. 70, 1924–1935. doi: 10.1128/IAI.70.4.1924-1935.2002
Shan, X. F., Kang, Y. H., Xia, J. J., Long, J. B., Chen, L., Wang, H., et al. (2015). Comparative studies on biological characteristics of Aeromonas veronii from different hosts. Chin. J. Vet. Med. 51, 82–85. doi: 10.3969/j.issn.0529-6005.2015.03.030
Soler, L., Figueras, M. J., Chacón, M. R., Vila, J., Marco, F., Martinez-Murcia, A. J., et al. (2002). Potential virulence and antimicrobial susceptibility of Aeromonas popoffii recovered from freshwater and seawater. FEMS Immunol. Med. Microbiol. 32, 243–247. doi: 10.1016/s0928-8244(01)00303-0
Song, M. F., Zhang, D. X., Zhang, H. P., Chen, L., Kang, Y. H., Zhang, L., et al. (2018). Research advances of virulence factors in Aeromonas veronii. Chin. Vet. Sci. 48, 1038–1042. doi: 10.16656/j.issn.1673-4696.2018.0152
Sun, J. J., Zhang, X. J., Gao, X. J., Jiang, Q., Wen, Y., and Lin, L. (2016). Characterization of virulence properties of Aeromonas veronii isolated from diseased Gibel carp (Carassius gibelio). Int. J. Mol. Sci. 17:496. doi: 10.3390/ijms17040496
Sun, Q. H., Sun, P. F., Jin, L. H., and Wu, J. N. (1991). On the pathogenic bacteria of the hemolytic ascitesosis of Allogynoenetic crucian carp. J. Fish. Chin. 15, 130–139. doi: 10.1007/s00253-019-10096-7
Thomas, J., Madan, N., Nambi, K. S. N., Abdul Majeed, S., Nazeer Basha, A., and Sahul Hameed, A. S. (2013). Studies on ulcerative disease caused by Aeromonas caviae-like bacterium in Indian catfish, Clarias batrachus (linn). Aquaculture 37, 146–150. doi: 10.1016/j.aquaculture.2012.11.015
Van der Marel, M., Schroers, V., Neuhaus, H., and Steinhagen, D. (2008). Chemotaxis towards, adhesion to, and growth in carp gut mucus of two Aeromonas hydrophila strains with different pathogenicity for common carp, Cyprinus carpio L. J. Fish Dis. 31, 321–330. doi: 10.1111/j.1365-2761.2008.00902.x
Vila, J., Marco, F., Soler, L., Chacon, M., and Figueras, M. J. (2002). In vitro antimicrobial susceptibility of clinical isolates of Aeromonas caviae, Aeromonas hydrophila and Aeromonas veronii biotype sobria. J. Antimicrob. Chemother. 49, 701–702. doi: 10.1093/jac/49.4.701
Wang, S. T., Meng, X. Z., Li, L. S., Dang, Y. F., Fang, Y., Shen, Y., et al. (2017). Biological parameters, immune enzymes, and histological alterations in the livers of grass carp infected with Aeromonas hydrophila. Fish Shellfish Immunol. 70, 121–128. doi: 10.1016/j.fsi.2017.08.039
Xia, F., Liang, L. G., and Xie, J. (2012). Isolation, identification and susceptibility test of the pathogenic Aeromonas veronii from Carassius auratus gibelio. Freshw. Fish. 42, 22–26. doi: 10.3969/j.issn.1000-6907.2012.05.005
Yamamoto, S., and Harayama, S. (1995). PCR amplification and direct sequencing of gyrB genes with universal primers and their application to the detection and taxonomic analysis of Pseudomonas putida strains. Appl. Environ. Microbiol. 61, 1104–1109.
Yao, D. R., Bing, X. W., Zhu, M., Bi, K. R., Chen, L., and Zhang, X. J. (2010). Molecular identification and drug resistance of pathogenic Aeromonas sobria isolated from Misgurnus anguillicaudatus. Oceanol. Limnol. Sinica 41, 756–762. doi: 10.11693/hyhz201005014014
Zhang, D. X., Kang, Y. H., Tian, J. X., Jü, A. Q., Fei, Y. D., Jia, J. P., et al. (2017). Isolation, identification and drug susceptibility of the pathogenic Aeromonas veronii from Carassius aumtus. Chin. Vet. Sci. 47, 366–374.
Zhang, X. J., Qin, G. M., Bing, X. W., Yan, B. L., and Bi, K. R. (2011). Phenotypic and molecular characterization of Photobacterium damselae, a pathogen of the cultured tongue sole Cynoglossus semilaevis in China. New Zeal. J. Mar. Fresh. 45, 1–13. doi: 10.1080/00288330.2010.531745
Zhou, L., Wang, Y., and Gui, J. F. (2001). Molecular analysis of silver crucian carp (Carassius auratus gibelio Bloch) clones by SCAR markers. Aquaculture 201, 219–228. doi: 10.1016/S0044-8486(01)00603-2
Keywords: Carassius auratus gibelio, Aeromonas veronii, 16S rRNA gene, gyrB gene, virulence genes, growing characteristics
Citation: Chen F, Sun J, Han Z, Yang X, Xian J-a, Lv A, Hu X and Shi H (2019) Isolation, Identification and Characteristics of Aeromonas veronii From Diseased Crucian Carp (Carassius auratus gibelio). Front. Microbiol. 10:2742. doi: 10.3389/fmicb.2019.02742
Received: 03 September 2019; Accepted: 11 November 2019;
Published: 26 November 2019.
Edited by:
Jonathan Shaw, The University of Sheffield, United KingdomReviewed by:
Graciela Castro Escarpulli, National Polytechnic Institute, MexicoVijai Singh, Indrashil University, India
Copyright © 2019 Chen, Sun, Han, Yang, Xian, Lv, Hu and Shi. This is an open-access article distributed under the terms of the Creative Commons Attribution License (CC BY). The use, distribution or reproduction in other forums is permitted, provided the original author(s) and the copyright owner(s) are credited and that the original publication in this journal is cited, in accordance with accepted academic practice. No use, distribution or reproduction is permitted which does not comply with these terms.
*Correspondence: Jingfeng Sun, sun_jf@163.com