- 1Key Laboratory of Biotechnology and Bioresources Utilization, Ministry of Education, Dalian Minzu University, Dalian, China
- 2College of Food Science and Technology, Bohai University, National and Local Joint Engineering Research Center of Storage, Processing and Safety Control Technology for Fresh Agricultural and Aquatic Products, Jinzhou, China
- 3School of Food Science and Technology, Jiangnan University, Wuxi, China
- 4Beijing Key Laboratory of Flavor Chemistry, Beijing Technology and Business University, Beijing, China
Some bacteria can modulate their spoilage potential by responding to environmental signaling molecules via the quorum sensing (QS) system. However, the ability of Pseudomonas fluorescens, the specific spoilage organism (SSO) of turbot, to response to environmental signaling molecules remains unclear. This study investigated the effects of six synthetic N-acyl homoserine lactones (AHLs) on typical behaviors mediated by QS in P. fluorescens, such as biofilm formation and extracellular protease activity. Total volatile basic nitrogen (TVB-N) was used as a spoilage indicator to evaluate quality changes in AHL-treated turbot filets during storage. The results confirm the enhancing effect of environmental AHLs on QS-dependent factors of P. fluorescens and quality deterioration of turbot filets, with C4-HSL and C14-HSL being the most effective. Moreover, the content decrease of exogenous AHLs was also validated by gas chromatography–mass spectrometry analysis. Further, changes in rhlR transcription levels in P. fluorescens suggest that this bacterium can sense environmental AHLs. Finally, molecular docking analysis demonstrates the potential interactions of RhlR protein with various exogenous AHLs. These findings strongly implicate environmental AHLs in turbot spoilage caused by P. fluorescens, suggesting preservation of turbot should not exclusively consider the elimination of SSO-secreted AHLs.
Introduction
Bacterial growth and metabolism results in aquatic food spoilage through protein degradation and formation of unacceptable volatile constituents, such as amines, alcohols, aldehydes, and sulfides (Gram and Dalgaard, 2002). Specific spoilage organisms (SSOs) play a crucial role in this process. Recent studies have indicated that bacterial spoilage may be regulated by a cell density-dependent communication mechanism called quorum sensing (QS), which is based on the self-secretion and detection of QS signaling molecules (Fuqua et al., 1994; Gui et al., 2017; Zhao et al., 2017). Bacteria trigger the expression of factors required for food spoilage (such as extracellular proteases, lipases, pectases, and cellulases) or pathogenesis (such as biofilm formation, motility alteration, hemolysin production, and pyocyanin generation) when the concentration of these signaling molecules reaches a certain threshold level (Bai and Rai, 2011). Interestingly, different microorganisms rely on different types of signaling molecules to regulate their QS systems (Papenfort and Bassler, 2016). Pseudomonas aeruginosa typically regulates collective behaviors via a wide range of signaling molecules such as 3-oxo-C12-HSL, C4-HSL, 2-heptyl-3-hydroxy-4-quinolone (PQS), 2-heptyl-4-hydroxyquinoline (HHQ), and 2-(2-hydroxyphenyl)-thiazole-4-carbaldehyde (IQS). Alternatively, Vibrio harveyi typically employs secreted harveyi autoinducer-1 (HAI-1), autoinducer-2 (AI-2), and cholerae autoinducer-1 (CAI-1) as signaling molecules (Galloway et al., 2011; Defoirdt, 2017). Recent studies have found that the above signaling molecules are often detected in contaminated aquatic products (Li et al., 2016; Zhao et al., 2017). Accordingly, additional work is still needed to explore the potential relationship between signaling molecule-based QS system and food spoilage.
Pseudomonas fluorescens is a gram-negative bacterium widely distributed in soil, plants, and the marine environment. Moreover, P. fluorescens possesses the characteristics of psychrotrophs, which are often associated with the spoilage of food stored at low temperatures, such as aquatic products and milk (Bandlitz and Berke, 2013; Stuknytė et al., 2016). Like most gram-negative bacteria, N-acyl-homoserine lactones (AHLs) have been detected in many foods spoiled by P. fluorescens (Liu et al., 2008; Bai and Rai, 2011). This suggests that the AHL-mediated QS system may be an important QS system in P. fluorescens, although it cannot be detected in all P. fluorescens because of the diversity of it (Pinto et al., 2007; Oliveira et al., 2015). Commonly, AHLs are synthesized by the bacterial LuxI-type enzymes and are bound to LuxR-type receptors to form receptor–AHL complexes, which associate with short DNA sequences termed “lux boxes” upstream of target genes to regulate diverse biological functions (Schuster et al., 2004). However, an increasing number of studies indicate that bacteria that do not produce, or produce fewer, QS signaling molecules can benefit from monitoring the density of environmental molecules, rather than investing effort into the production of “public goods” such as their own QS signaling molecules (Diggle et al., 2007). This phenomenon of using QS signaling molecules synthesized by other microorganisms to enhance their own collective behavior is known as “eavesdropping” in bacterial populations (Case et al., 2008). For example, Smith et al. (2011) demonstrated eavesdropping of AHLs by Salmonella promoted growth and survival in food environments. Similarly, Almeida et al. (2017) found that C12-HSL induced biofilm formation of Salmonella enterica, indicating these bacteria can respond to AHLs synthesized by other bacterial species. However, scarce information is available on this phenomenon in foodborne spoilage bacteria, including P. fluorescens, and its relationship with food spoilage.
Turbot (Scophthalmus maximus) is one of the most economically important aquaculture fish species in China. Turbot is vulnerable to microbial spoilage due to its soft texture and high moisture and protein content. Our previous study demonstrated that P. fluorescens is a SSO of turbot stored at 4°C and its spoilage potential has been demonstrated to be regulated by the QS system (Yang et al., 2017). Interestingly, AHL production of P. fluorescens used in this study is strikingly weaker, regardless of AHL type, than other microorganisms simultaneously isolated from spoiled turbot, such as Aeromonas sobria (Li et al., 2016). However, P. fluorescens is capable of becoming the SSO of turbot and demonstrates strong spoilage potential in later storage periods. Generally, microorganisms with strong QS tend to have better environmental stress tolerance and competitiveness due to up-regulated expression of related genes (Yuan et al., 2005; Bronesky et al., 2016). Therefore, the phenomenon of signaling molecule eavesdropping may also exist in P. fluorescens to improve its spoilage potential, especially in the condition that a variety of AHLs had been detected in spoiled turbots (Zhang et al., 2016a). Hence, the effects of various non-secreted AHLs on QS phenotypes (i.e., biofilm formation and ECP activity) and spoilage potential of P. fluorescens were evaluated in this study. Furthermore, changes in QS-related gene expression and the binding ability of exogenous AHLs with receptor proteins in P. fluorescens were analyzed.
Materials and Methods
Materials and Bacterial Strains
Live commercial-size (900 ± 5 g) turbots were purchased from a local Aquatic Market (Jinzhou, China) and transported to the Food Safety Key Lab of Liaoning Province for further experiments. All six AHL standards, N-butanoyl-L-homoserine lactone (C4-HSL), N-hexanoyl-L-homoserine lactone (C6-HSL), N-octanoyl-L-homoserine lactone (C8-HSL), N-decanoyl-L-homoserine lactone (C10-HSL), N-dodecanoyl-L-homoserine lactone (C12-HSL), and N-tetradecanoyl-L-homoserine lactone (C14-HSL) were purchased from Sigma-Aldrich (St. Louis, MO, United States), and dissolved in methanol as stock solutions with the concentration of 2 mg/mL.
In the subsequent experiments, the stock solution was diluted at 1:1000 ratio in the medium to the final concentration of 2 μg/mL (C4-HSL: 11.68 μM, C6-HSL: 10.04 μM, C8-HSL: 8.8 μM, C10-HSL: 7.83 μM, C12-HSL: 7.06 μM, C14-HSL: 6.42 μM). Other chemical agents were all of analytical grade and commercially available.
Pseudomonas fluorescens (GenBank accession number: CP032618) used in the present study was previously isolated from refrigerated turbots following the method of Yi et al. (2011) and was cultured overnight in Luria–Bertani (LB) broth (1% peptone, 1% NaCl, 0.5% yeast extract, pH 7.0 ± 0.2) at 160 rpm and 28°C, until an OD600 of 1 was reached.
Assay of Bacterial Growth
The effect of different AHLs on the bacterial growth was evaluated according to the method of Jie et al. (2018). Overnight culture of P. fluorescens (OD600 = 1) was diluted at 1:100 ratio in fresh LB broth containing 2 μg/mL of different AHLs (C4-HSL, C6-HSL, C8-HSL, C10-HSL, C12-HSL, and C14-HSL), respectively. Equivalent methanol was added to P. fluorescens culture as the control group. Then, all samples were incubated at 28°C for 48 h. Bacterial density was estimated by measuring the OD600 in 3 h intervals.
Assay of Biofilm Formation
Biofilm formation was assessed following the method described by Rode et al. (2007). First, 100 μL of P. fluorescens overnight culture was inoculated in the sterile LB broth containing 2 μg/mL of different AHLs (C4-HSL, C6-HSL, C8-HSL, C10-HSL, C12-HSL, and C14-HSL), respectively. Samples that were treated with equivalent methanol were used as control groups. Immediately, 1 mL culture containing AHLs was transferred to germ-free 1.5 mL microcentrifuge tube and further incubated statically at 28°C for 48 h. After discarding the suspension cultures, each microtube was rinsed thrice with sterile water to eliminate planktonic bacteria, and dried for 30 min. Then, 1 mL of 0.1% (w/v) crystal violet was used to stain the surface-adhered cells in the tubes for 15 min. Next, the tubes were washed thoroughly with sterile water and the crystal violet-stained biofilm was re-solubilized in 1 mL of 33% acetic acid, followed by transferring 200 μL of the above solution to a 96-well microtiter plate. Finally, the absorbance in each well at 595 nm was measured. Each sample was independently repeated nine times.
Analysis of Biofilms by SEM
The SEM observation was performed as previously described (Gomes and Mergulhão, 2017), and P. fluorescens was cultured overnight in LB broth with shaking (160 rpm) at 28°C. Then, 100 μL of culture was diluted in 10 mL of sterile LB broth containing 2 μg/mL of different AHLs (C4-HSL, C6-HSL, C8-HSL, C10-HSL, C12-HSL, and C14-HSL). Next, a piece of polished zinc was placed in each dilution culture to facilitate biofilm formation. After incubation at 28°C for 48 h, the zinc pieces were removed from the cultures and rinsed thrice with sterile water. Immediately, the zinc pieces were immersed in 2.5% (v/v) precooled glutaraldehyde for another 4 h, and soaked in 50, 70, 80, and 90% (v/v) ethanol for 10 min, followed by twice incubation in 100% ethanol for 15 min. Finally, the biofilms were visualized by SEM, and samples without signaling molecules were used as control groups.
Extracellular Protease Activity Assay
Milk Plate Assay
Extracellular protease activity in P. fluorescens was measured as previously described (Vijayaraghavan and Vincent, 2013). Briefly, 15% (w/v) skim milk and 1.5% (w/v) agar were prepared, sterilized at 115°C for 30 min, and cooled to 50°C. The milk agar plates were prepared by mixing skim milk and agar at a 1:1 ratio. Then, overnight culture of P. fluorescens (OD600 = 1) was diluted at 1:100 ratio in fresh LB broth containing 2 μg/mL of different AHLs (C4-HSL, C6-HSL, C8-HSL, C10-HSL, C12-HSL, and C14-HSL), respectively. After cultivation (28°C, 48 h), the culture was centrifuged at 8000 × g for 10 min. Immediately, the supernatant containing the extracellular protease was collected. Next, 200 μL of aliquot was added to the milk agar plates after filtering through a 0.22-μm filter and incubated for another 48 h. Finally, the size of the transparent enzymolysis zones was determined and samples without signaling molecules were used as control groups.
Azocasein Assay
Proteolytic activity of P. fluorescens culture was determined according to the method described by Martins et al. (2014) by using azocasein assay. Briefly, overnight cultures of P. fluorescens (OD600 = 1) were diluted at a 1:100 ratio in fresh LB containing 2 μg/mL of different AHLs. After culturing at 28°C for 48 h, different cultures were centrifuged at 8000 × g for 10 min. Thereafter, 150 μL of sterile filtered culture supernatant was added to 250 μL of 2% azocasein (w/v). After incubation (30°C for 12 h), this was mixed with 1.2 mL of 10% (w/v) trichloroacetic acid and the new mixture was further incubated at 25°C for 15 min. After centrifuging for 10 min at 15,000 × g, 600 μL of supernatant was removed and added to 50 μL of 1 M NaOH. Finally, the proteolytic activity of P. fluorescens culture was quantified by determining the OD440 of the mixture. Moreover, 150 μL of LB media was used as blank and the P. fluorescens culture without any AHLs was used as a control group. Each sample was used for three independent replicates.
Detection of AHL Eavesdropping by GC–MS
AHL Extraction
N-Acyl homoserine lactones extraction was based on the procedure introduced by Ravn et al. (2001). First, 1 mL of P. fluorescens overnight culture (OD600 = 1) was inoculated in 100 mL of LB broth containing 2 μg/mL of different AHLs (C4-HSL, C6-HSL, C8-HSL, C10-HSL, C12-HSL, and C14-HSL), respectively, and cultured at 160 rpm and 28°C for only 24 h to avoid the alkaline environment and acylase accumulation caused by long-term culture. Moreover, as blank control, equal amounts (2 μg/mL) of different AHLs were added to sterile fresh LB broth (100 mL) and cultured under the same conditions. After incubation and centrifugation (8000 × g, 10 min), the supernatant was extracted thrice with 100 mL of ethyl acetate, followed by evaporating the organic phase in a rotary evaporator. Finally, the extract was dissolved in 1 mL of methanol and filtered through 0.22-μm filters before storage at −20°C for further analysis. AHLs in pure culture of P. fluorescens (without exogenous AHLs) were also extracted and detected by gas chromatography–mass spectrometry (GC–MS) to identify the composition of AHLs secreted by P. fluorescens.
GC–MS Conditions
The AHLs were further identified and quantified by GC–MS according to Zhu et al. (2016), with slight modification. GC–MS analyses were carried out on the GC–MS-7890N/5975 instrument (Agilent, Palo Alto, CA, United States) with a HP-5 MS capillary column (30 m length × 0.25 mm internal diameter × 0.25 μm film thickness). All sample injections were done in a splitless mode when the inlet temperature was maintained at 200°C, and pure helium (1.0 mL/min) was used as the carrier gas. When measuring AHLs, the GC oven temperature was programmed as follows: 150°C ramped at 10°C/min to 220°C, next ramped at 5°C/min to 250°C, and ramped at 0.5°C/min to 252.5°C. Mass spectra were obtained under the following conditions: electron ionization source was set to 70 eV, emission current 500 μA, MS Quad temperature 150°C, and MS Source temperature 230°C. Data were acquired by scanning the m/z range of 35–800 amu and by selected ion monitoring of m/z 143. AHLs in extracts were identified by comparing the retention times and chromatographic characteristics with each AHL standard.
RNA Isolation, cDNA Synthesis, and Quantitative Real-Time PCR
Four QS-related genes (rhlI, rhlR, flgA, and aprA) were previously found in P. fluorescens by sequencing and whole genome sequence analysis of the test strain. Overnight culture of P. fluorescens (OD600 = 1) was diluted at 1:100 ratio in fresh LB broth containing 2 μg/mL of different AHLs (C4-HSL, C6-HSL, C8-HSL, C10-HSL, C12-HSL, and C14-HSL), respectively. Samples without signaling molecules were used as control groups. After cultivation (adjusted to 1.0 at OD600 nm), the total RNA of test strains was extracted according to the manufacturer’s instructions using TRIzol reagent (Sigma Chemicals), and treated twice with DNase I (Thermo Scientific, #EN0521). After using a Nanodrop to ensure an OD260/OD280 between 1.7 and 2.1, the RNA was reverse transcribed using RevertAid First Strand cDNA Synthesis Kit (Thermo Scientific, #K1621) following the manufacturer’s instructions. Finally, Power SYBR® Green PCR Master Mix (Applied Biosystems® Cat: 4367659) was used for performing quantitative real-time PCR (qPCR) analysis (CFX ConnectTM System, BIO-RAD, United States), under the following conditions: 95°C for 3 min, followed by 40 cycles of denaturation at 95°C for 10 s, annealing at 55°C for 20 s, and extension at 72°C for 20 s. The housekeeping gene 16S rRNA was used as an internal reference. The primer sequences are listed in Table 1. The expression level of different genes was quantified with the standard formula by calculating 2–ΔΔCt.
In silico Analysis
Homology protein models of QS transcriptional activator (RhlR) were generated and assessed with SWISS-MODEL1 (Biasini et al., 2014; Waterhouse et al., 2018) according to the primary structure of RhlR protein. The 3D files of AHLs (C4-HSL, C6-HSL, C8-HSL, C10-HSL, C12-HSL, and C14-HSL) were obtained from the open chemistry database of NCBI2. Before docking the ligands into the active site, the 3D structure of the modeled protein was analyzed and further optimized by the addition of missing hydrogen atoms and removing excess crystallographic water using the SYBYL-X 2.1 program (Ding et al., 2017). Furthermore, spheres within 5 Å of the ligand were selected and defined as docking packets, and the residues not in the active site were deleted. Finally, the docking results were analyzed using the Cscore (Consensus Score) module of SYBYL-X 2.1 program to search for the best ligand–receptor conformations.
Spoilage Potential of P. fluorescens in Turbot Filets
Turbot filets were prepared following the method of Dalgaard (1995), with slight modifications. First, the turbots were killed and fileted by a professional operator in “Lin Xi” aquatic market. Then, the filets were transport to the laboratory under aseptic conditions, followed by washing the filets with sterile water. After skinning the filets, the samples were wiped thoroughly with 75% (v/v) ethanol and exposed under an ultraviolet lamp until the total viable counts were <102 CFU/g. For the preparation of inocula, cells of P. fluorescens cultured in LB broth (OD600 = 1) were collected by centrifugation at 8000 × g for 10 min, and the deposit was resuspended in sterile saline solution to dilute the bacterial concentration to 106 CFU/mL. Finally, the turbot filets were immersed in the prepared bacterial suspension containing 2 μg/mL of different AHLs (C4-HSL, C6-HSL, C8-HSL, C10-HSL, C12-HSL, and C14-HSL), respectively, for 5 s to ensure the filets at a level of 104 CFU/g. All filets were individually packed and divided into five groups (three subsamples in each group) stored at 4 ± 1°C. The total volatile basic nitrogen (TVB-N) (mg N/100 g muscle) contents were evaluated at 3-day intervals (0, 3, 6, 9, and 12 days, corresponding to five groups) to analyze the spoilage potential of P. fluorescens. Furthermore, the TVB-N changes of turbots without inoculating P. fluorescens were also determined as blank control.
For TVB-N analysis, each subsample was ground individually using a handheld blender (WBL25B26, Midea, China). Then, 10 g of ground filets was taken from each subsample, respectively, and added to a distilling tube containing 1 g of MgO and 50 mL of distilled water. The TVB-N contents of each subsample were determined using a Kjeltec 8400 automatic nitrogen determination apparatus (FOSS, Hiller, Denmark) in the Kjeldahl mode. The experimental results of each group were the average of three subsamples.
Statistical Analysis
Each test was performed for three independently repeated experiments and data were presented as the mean ± standard deviation (SD). Statistical analysis was conducted by repeated measures using SPSS 20.0, and differences in mean values were tested for significance using one-way analysis of variance (ANOVA) with Tukey’s test. The level of statistical significance was determined at P < 0.05.
Results
The Effect of Exogenous AHLs on the Growth of P. fluorescens
The effects of six synthesized AHLs on the growth of P. fluorescens in LB broth were evaluated (Supplementary Figure S1). The results showed that the growth of P. fluorescens was significantly (P < 0.05) accelerated by shortening the lag phase in the presence of C4-HSL, C6-HSL, C8-HSL, C12-HSL, and C14-HSL. Moreover, the addition of most exogenous AHLs increased the maximum cell density of P. fluorescens during the 48 h, which demonstrated the promotion effect of AHLs on the growth of P. fluorescens. The results also showed that the treatment of C10-HSL had no significant effect on the bacterial growth.
The Effect of Exogenous AHLs on Biofilm Formation in P. fluorescens
The effects of exogenous AHLs on biofilm formation in P. fluorescens were investigated by crystal violet assay (Supplementary Table S1). Addition of exogenous AHL molecules significantly enhanced biofilm formation of P. fluorescens (P < 0.05), except for C10-HSL. A maximum biofilm stimulation rate of 127.49% was obtained with C4-HSL, suggesting that exogenous AHLs can promote biofilm formation in P. fluorescens.
The images obtained by SEM further support these findings (Figure 1). The biofilm of samples treated with C4-HSL, C6-HSL, C12-HSL, and C14-HSL were thicker and more layered than that of the control group, confirming the promotion of biofilm formation in P. fluorescens by utilizing exogenous AHLs. Moreover, no significant effect on biofilm formation in P. fluorescens was observed in the presence of C10-HSL, consistent with the crystal violet assay.
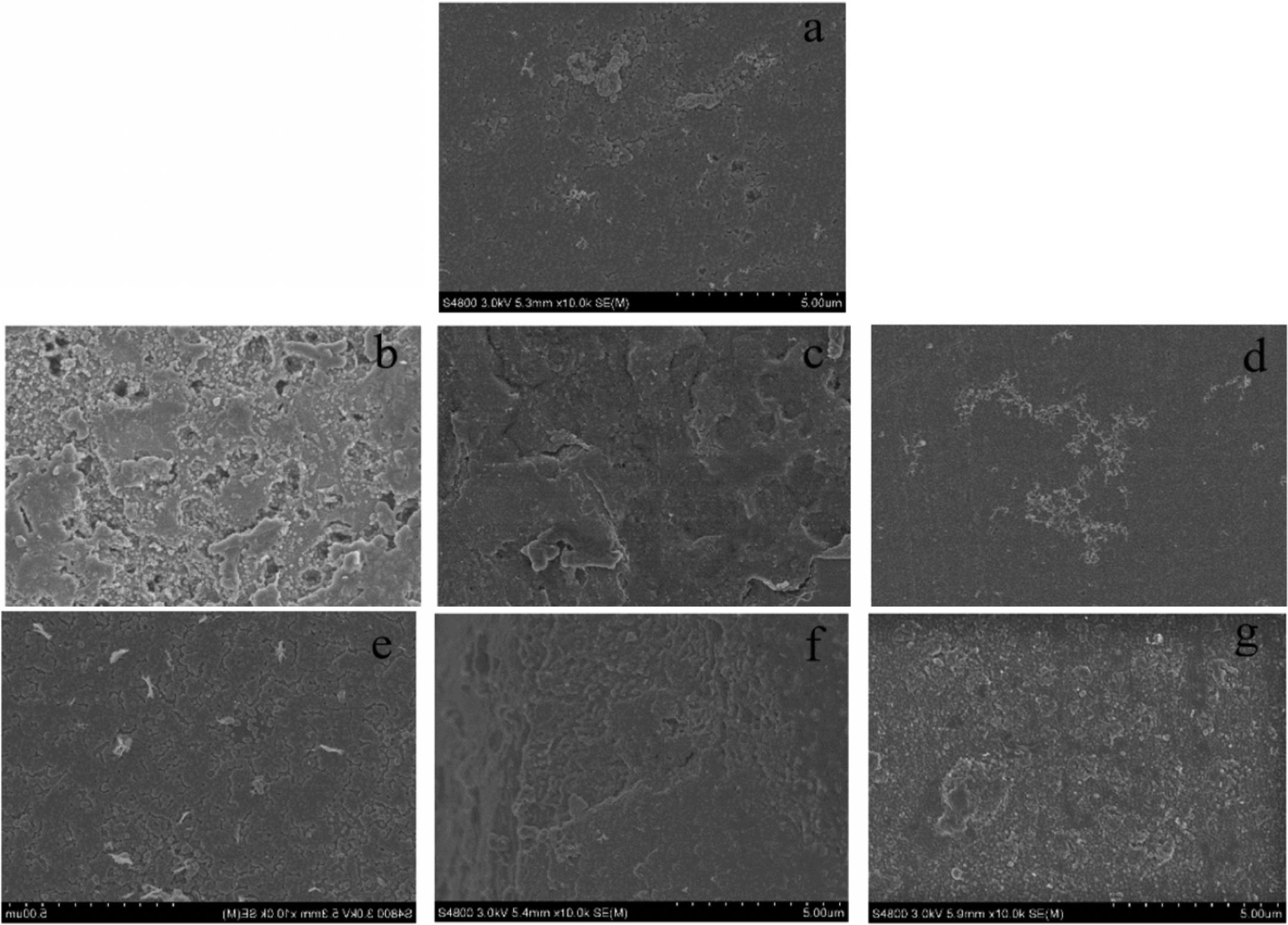
Figure 1. SEM images of biofilm formation in P. fluorescens after incubation with or without exogenous AHLs for 48 h. (a) Control and (b–g) AHLs treatment with C4-HSL, C6-HSL, C8-HSL, C10-HSL, C12-HSL, and C14-HSL at 2 μg/mL.
The Effect of Exogenous AHLs on the Protease Activity of P. fluorescens
As shown in Figure 2A, the effect of exogenous AHLs on extracellular protease secretion by P. fluorescens was studied using milk agar plates. A larger enzymolysis circle was clearly observed in the presence of C4-HSL, C6-HSL, C8-HSL, C12-HSL, and C14-HSL, confirming the effective utilization of AHLs by P. fluorescens. Treatment with C10-HSL had no significant effect (P > 0.05). Among all the exogenous AHLs, C4-HSL and C14-HSL had more significant stimulation rates at 63.40 and 52.42%, respectively (Supplementary Table S2). Similar to the results of milk plate assay, the proteolytic activity in P. fluorescens was also promoted by the addition of AHLs, indicating that this phenotype can be regulated by exogenous AHLs in the test strain (Figure 2B).
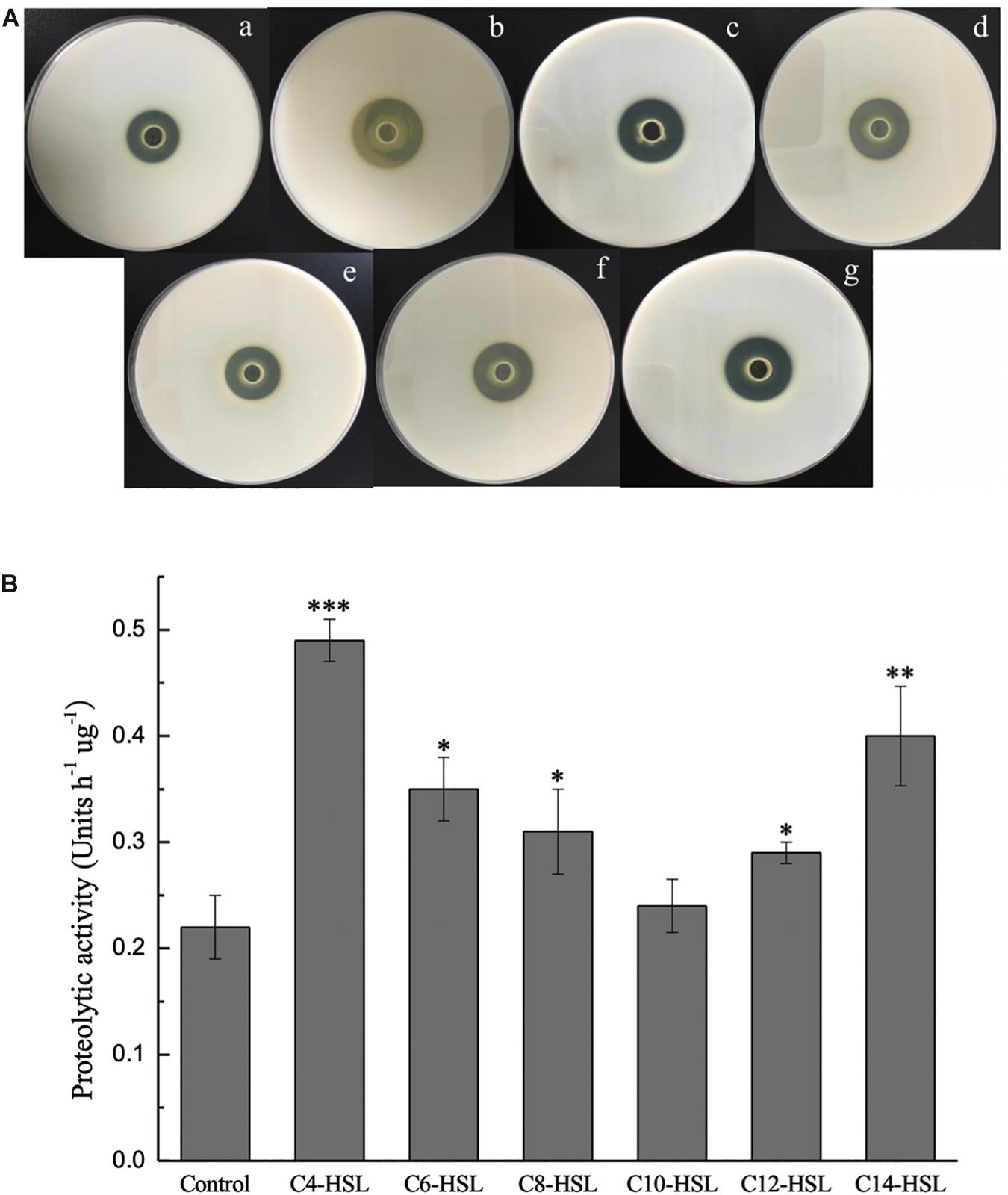
Figure 2. Extracellular proteinase activity of P. fluorescens after incubation with or without exogenous AHLs for 48 h tested by milk agar plates assay (A) and azocasein assay (B). (a) Control and (b–g) AHLs treatment with C4-HSL, C6-HSL, C8-HSL, C10-HSL, C12-HSL, and C14-HSL at 2 μg/mL. Data are presented as means ± SD (n = 3, ∗P < 0.05, ∗∗P < 0.01, ∗∗∗P < 0.001).
Detection of AHL Eavesdropping by GC–MS
GC–MS analysis was performed to identify the AHLs secreted by P. fluorescens, by comparing the chromatographic characteristics of different synthetic AHL standards. As shown in Supplementary Figure S2, C4-HSL and C10-HSL were verified from the supernatant of P. fluorescens, with C4-HSL was the predominant AHL type. Meanwhile, the AHLs contents in sterile LB broth and P. fluorescens culture were also determined by GC–MS after incubation in the same condition. As shown in Table 2, the AHLs contents in sterile LB broth did not change significantly before and after the cultivation, which indicated that exogenous AHLs could be stable in the process of cultivation without apparent degradation. In contrast, a reduction in AHL contents was observed in P. fluorescens cultures, suggesting that P. fluorescens could enhance the QS behavior by eavesdropping exogenous AHLs. Furthermore, the utilization of C14-HSL by P. fluorescens was the most significant, followed by C8-HSL and C6-HSL (Table 2).
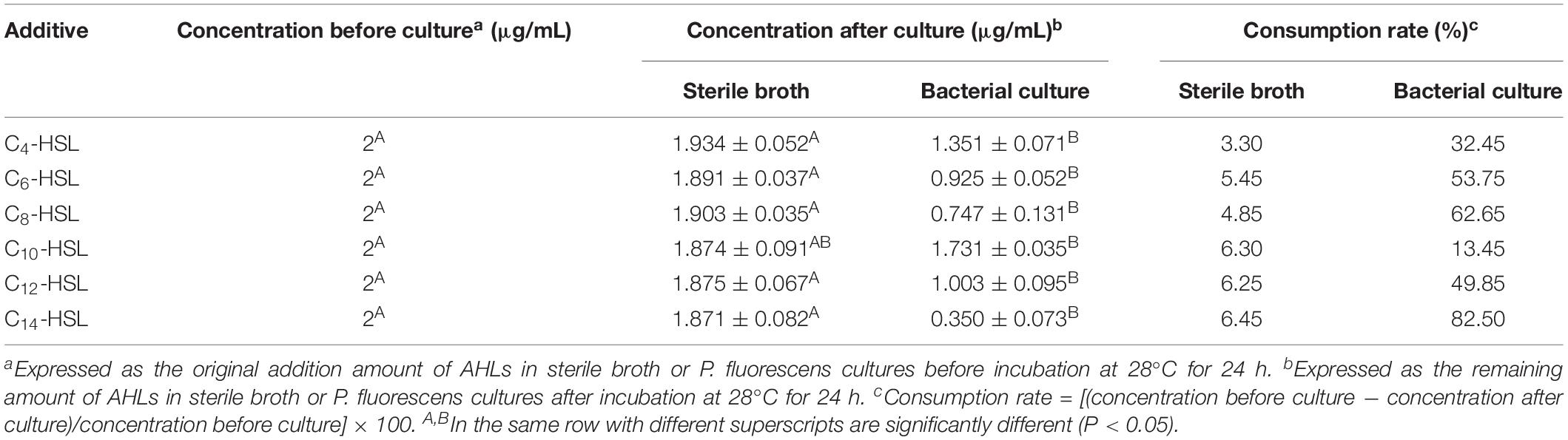
Table 2. The concentration changes of exogenous AHLs in different cultures during incubation (mean ± SD).
The Effect of Exogenous AHLs on QS-Related Gene Expression of P. fluorescens
The relative expression of rhlR was examined to explore whether P. fluorescens could sense AHLs in the environment. The result of qPCR analysis suggests that the AHLs used in this study can upregulate the expression level of rhlR in P. fluorescens, except for C10-HSL (Figure 3). As shown in Figure 3, C4-HSL and C14-HSL were the most effective AHLs in upregulating rhlR expression, 6.11-fold and 2.18-fold, respectively. Furthermore, the expression of rhlI, flgA, and aprA was also analyzed. Consistent with the results of the rhlR, the addition of most AHLs stimulated expression of these genes, but C10-HSL had no significant effect.
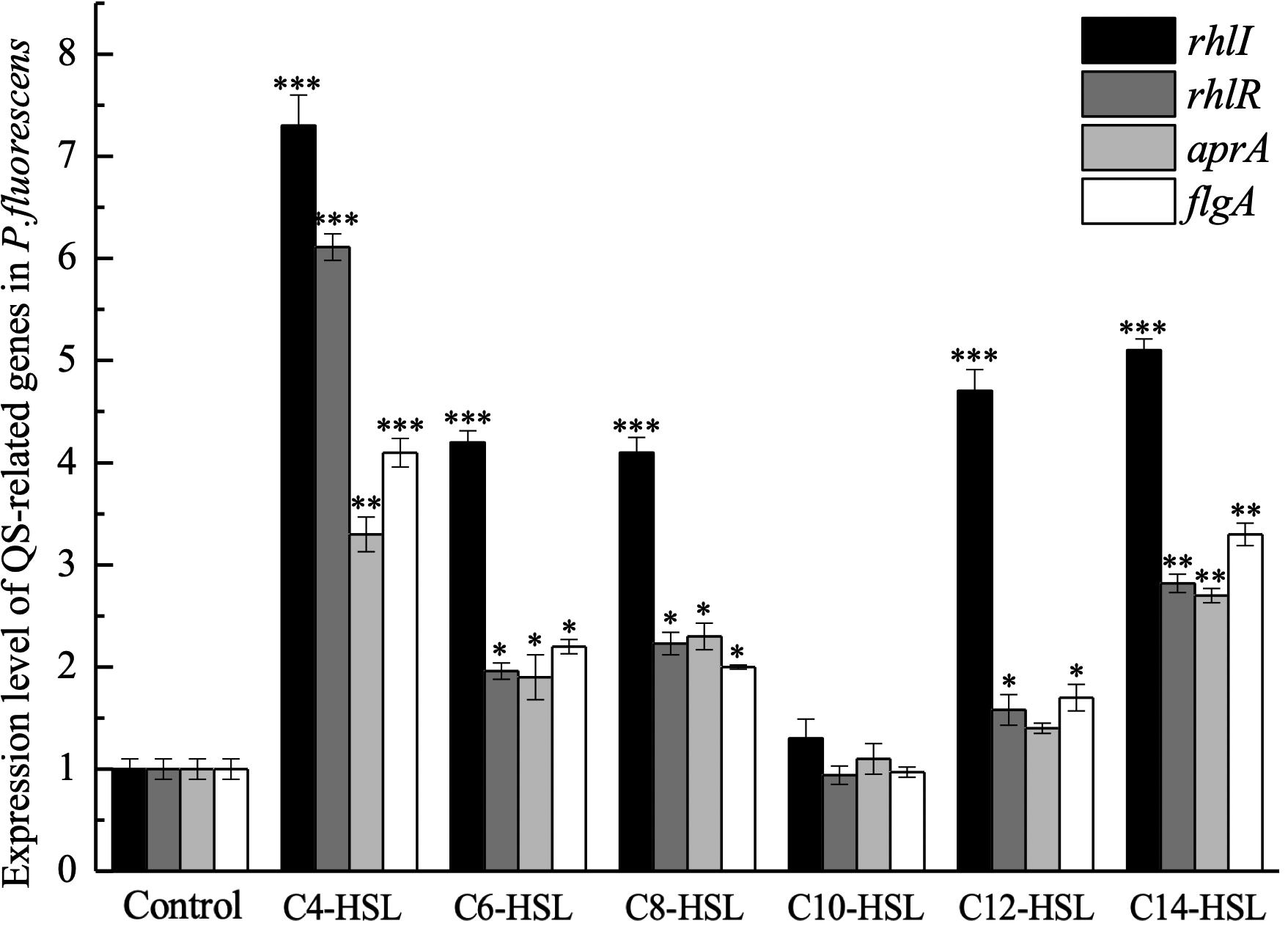
Figure 3. Expression level of QS-related genes in P. fluorescens after incubation with 2 μg/mL exogenous AHLs until an optical density at 600 nm (OD600) of 1 was reached. Data are presented as means ± SD (n = 3, ∗P < 0.05, ∗∗P < 0.01, ∗∗∗P < 0.001).
Homology Modeling and Model Quality Estimation
The RhlI/R system is known as one of the typical QS systems in Pseudomonas sp. and was the only QS system annotated in the genome of the test strain (GenBank accession number: CP032618). Meanwhile, RhlR protein, as the specific receptor of C4-HSL in this system, can regulate several biological functions of Pseudomonas sp. (Yan et al., 2007). In this study, 3D models of RhlR protein in P. fluorescens were generated using SWISS-MODEL in the first approach mode, and the obtained models were estimated by using the Protein Structure & Model Assessment Tools in SWISS-MODEL. Sequence alignment results showed that the two models of 4y13.1.A and 3qp5.1.A had high similarity (>35%) with the RhlR protein sequence of P. fluorescens in all 50 template sequences matched by the Template Library of SWISS-MODEL, based on sequence similarities and the Global Model Quality Estimation (GMQE) score (Supplementary Figure S3).
The primary structure of a protein is the basis of its 3D structure, and so, two sequences with homologous relationships usually have similar spatial structures. Therefore, homology models generated based on the two template sequences were shown in Supplementary Table S3 and Qualitative Model Energy Analysis (QMEAN), a comprehensive scoring function for model quality assessment was used to assess the entire model (Benkert and Tosatto, 2008). The nearest 0 score conforms a high quality for the model, whereas −4.0 or lower scores are indicated as low quality. The result showed that the model of 4y13.1.A was selected as the optimal model of RhlR for further analysis, due to the higher GMQE score (0.75) and QMEAN score (−2.31) (Supplementary Table S3). Furthermore, 4y13.1.A was also closer to the central dot, representing the target protein RhlR, in the visual map of the model comprehensive evaluation, which indicates that model 4y13.1.A can predict the 3D structure of RhlR protein well (Supplementary Figure S4).
Docking Analysis
The interaction of AHLs–RhlR was analyzed using computer-aided molecular docking. The protein model selected above (4y13.1.A) was prepared and docked with AHL molecules using the SYBYL-X 2.1 program. As shown in Figure 4A, all different AHL molecules bound to the active sites of the RhlR-type protein by hydrogen bonding, indicating the binding potential of signaling molecules to the RhlR protein in P. fluorescens. Furthermore, the Cscores of all six ligands were not less than 4, which realistically reflected the space conformation of the AHL–RhlR complex (Supplementary Table S4).
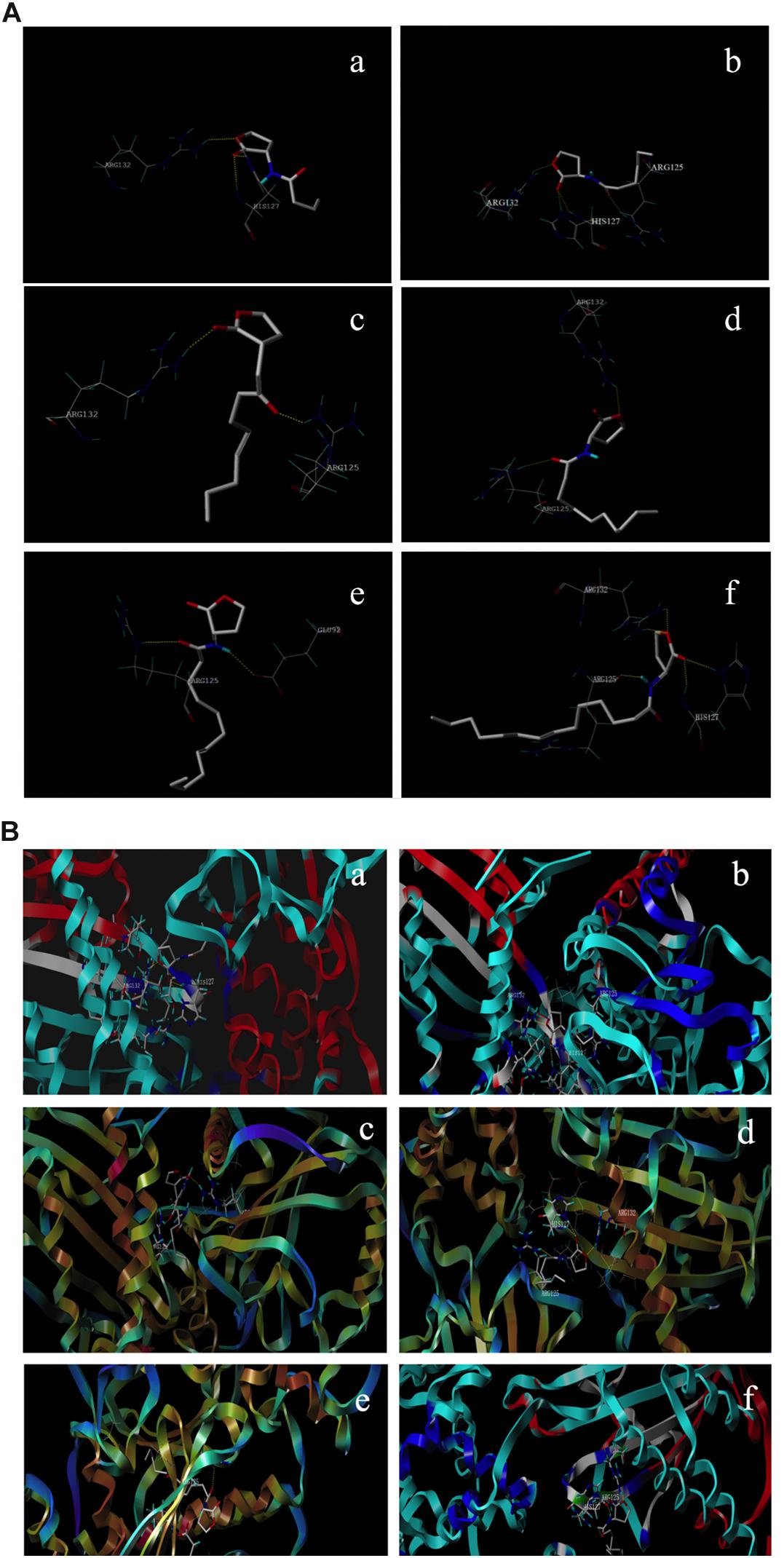
Figure 4. Interaction of different AHLs with the active site of RhlR type proteins in P. fluorescens (A) and the predicted conformation of AHLs in the binding packet (B). (a–f) C4-HSL, C6-HSL, C8-HSL, C10-HSL, C12-HSL, and C14-HSL.
Effects of Exogenous AHLs on the Spoilage Process of Refrigerated Turbot Filets
The production of TVB-N by P. fluorescens in turbot filets was monitored to evaluate the potential involvement of exogenous AHLs in the spoilage process. The results showed an increase in TVB-N values during storage, where the increased levels were significantly influenced by the presence of exogenous AHLs (Figure 5). TVB-N value increased from 5.21 to 40.17 mg N/100 g in the control group. Comparing all the AHLs added, exogenous C4-HSL and C14-HSL exhibited the greatest impact on the promotion of TVB-N values at the end of storage, reaching final values of 60.57 and 61.73 mg N/100 g, respectively.
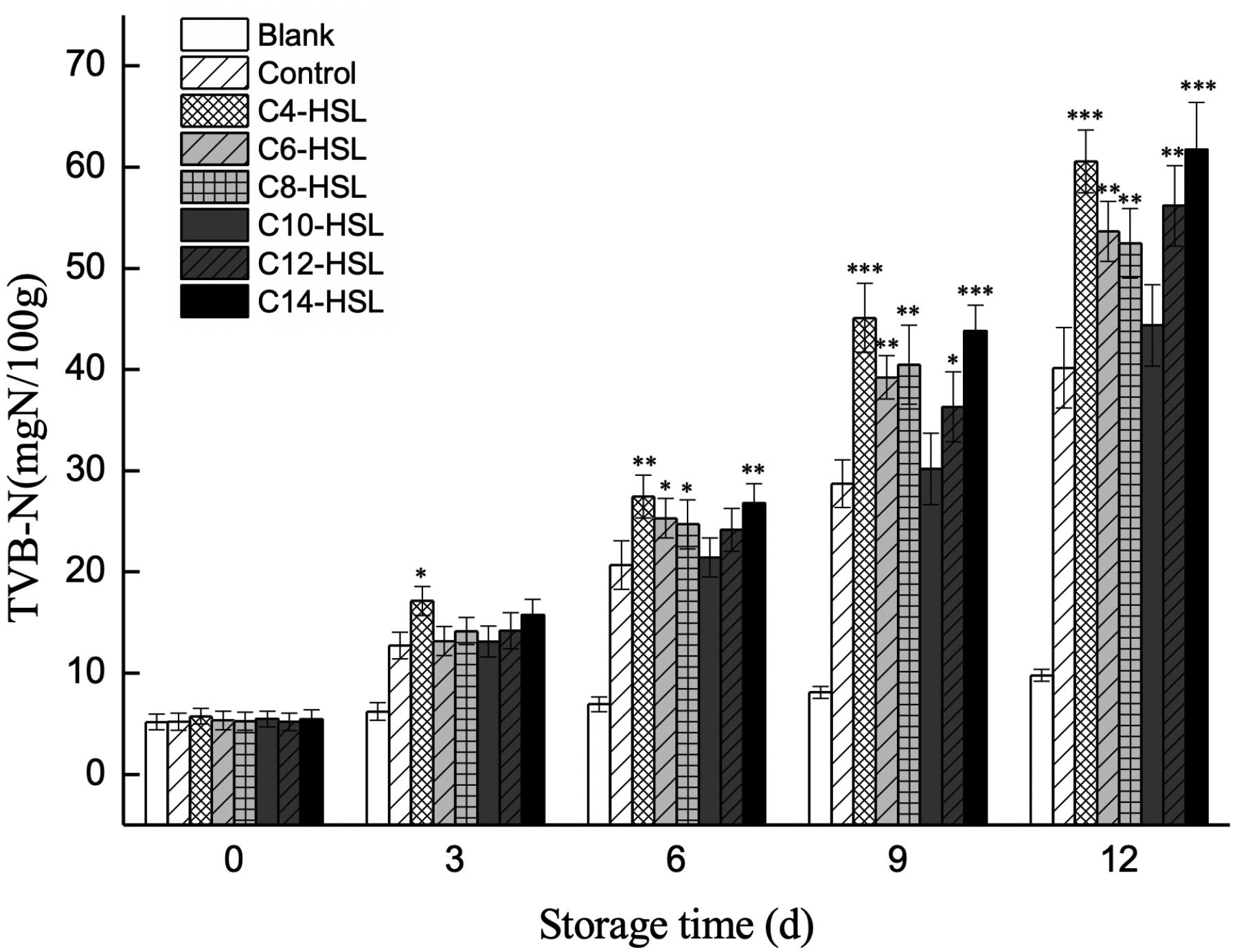
Figure 5. Changes in the TVB-N value of turbot filets contaminated with different AHLs during refrigerated storage. Data are presented as means ± SD (n = 3, ∗P < 0.05, ∗∗P < 0.01, ∗∗∗P < 0.001).
Discussion
The QS system, mediated by AHL signaling molecules, is prevalent among diverse bacterial species, particularly in gram-negative bacteria. The RhlI/R system is a common AHL-mediated QS system discovered in P. aeruginosa. As a transcriptional regulator, RhlR can specificity bind C4-HSL (synthesized by RhlI) to promote the expression of downstream genes, regulating biofilm formation, rhamnolipid biosynthesis, and other behaviors (Maier and Soberón-Chávez, 2000). However, the RhlI/R system in P. fluorescens and its regulatory effect are rarely reported. Furthermore, the QS system of microorganisms based on interactions between exogenous signaling molecules and receptor proteins has attracted attention (Cheng et al., 2018; Lv et al., 2018). Therefore, further research is still needed to evaluate the involvement of exogenous signaling molecules in the spoilage potential of bacteria, though the effects of the AHL-related QS system on aquatic product spoilage have been confirmed (Fu et al., 2018). The present study aimed to explore the effect of exogenous signaling molecules on QS-mediated spoilage of turbot by P. fluorescens via the rhl-system.
It has been reported that the QS system may play a positive role in the growth of some bacteria, such as Shewanella putrefaciens, Shewanella baltica, and P. aeruginosa, in order to improve their competitiveness in response to the environmental change (Zhang et al., 2014; Zhu et al., 2015). This may be due to the beneficial effects of some metabolites regulated by QS on cell division. Interestingly, it has been shown that some QS-deficient mutant bacteria which lack QS-controlled factors display growth advantages relative to wild strains under certain conditions (Sandoz et al., 2007). Likely, this phenomenon is due to “cheaters,” which can directly utilize, rather than produce, “public goods” such as AHLs. This reduces the metabolic burden for these species under conditions that ensure normal metabolism. Because of the importance of QS on microbial growth, different exogenous AHLs were added to the cultures of P. fluorescens to analyze their effect on bacterial growth. As shown in Supplementary Figure S1, exogenous AHLs successfully elevated population density levels of P. fluorescens and accelerated bacterial growth. This effect has been previously reported by many researchers (Nychas et al., 2009; Dourou et al., 2011). Our previous study also found that exogenous C4-HSL and C8-HSL accelerated growth and increased cell density of A. sobria AS7 in turbot (Li et al., 2016). The results obtained here confirmed a similar effect of AHLs on P. fluorescens. As previously discussed, this may be because exogenous AHLs stimulated the QS system in P. fluorescens, thus enhancing the production of growth-beneficial factors. It may also be that exogenous AHLs reduced the metabolic burden on P. fluorescens. Notably, some researchers also believe that phenomenon is observed because AHLs are used by bacteria as a carbon source. While it is difficult to fully define the causes of this phenomenon, the results obtained here suggested that P. fluorescens have the ability of utilizing non-secreted AHLs to regulate their growth. These findings agreed with those of Zhu et al. (2018), who showed that exogenous AHLs can be utilized by S. baltica through the LuxR receptor to promote growth.
The selective pressure of antimicrobials or preservatives forces microorganisms to evolve a series of resistance mechanisms to ensure the species survival; among these, biofilm formation is one of the most effective strategies and is regulated by the QS system. Furthermore, in the food industry, microbial biofilms usually pose potential safety risks to food processing due to their adhesiveness ability to the contact surfaces of food processing equipment. Therefore, the ability of biofilm formation of spoilage bacteria can lead to the potential risk of biofilm-food cross-contamination in food processing. Previously, Davies et al. (1998) demonstrated the necessity of self-secreted signaling molecules in the development of P. aeruginosa biofilms by constructing lasI or rhlI mutants. In this study, the positive regulation of non-secreted AHLs on biofilm formation in P. fluorescens was further verified via the crystal violet assay (Supplementary Table S1), which showed that the addition of C4-HSL, C6-HSL, C8-HSL, C12-HSL, and C14-HSL promoted biofilm production in P. fluorescens. Moreover, the thicker biofilm images (Figures 1b,c,g) were also observed by SEM, indicating the treatment of exogenous AHLs stimulated the production of extracellular matrix in P. fluorescens. GC–MS results showed that the P. fluorescens used in this study could not produce C6-HSL and C14-HSL (Supplementary Figure S2), which confirmed the ability of P. fluorescens to promote biofilm formation by using exogenous signaling molecules in the environment. Similarly, Almeida et al. (2017) also confirmed the obvious effect of exogenous AHLs on biofilm production via the LuxR homolog regulator – SdiA. This is also consistent with the findings of Kusada et al. (2014), who found that the biofilm formation in Acidovorax sp. strain MR-S7 was induced by different kinds of AHL, in which 3-oxo-C8-HSL and C10-HSL had a better promotion rate.
As one of the major QS-mediated metabolites of bacteria, extracellular protease is considered to be largely responsible for the spoilage of aquatic products. Accordingly, the protease activity of P. fluorescens after treatment with various AHLs was evaluated to further explain the relationship between the eavesdropping phenomenon and the spoilage potential of bacteria. As shown in Figure 2A, P. fluorescens secreted extracellular proteases and produced clear protein lysis circles in milk agar plates during culture. Upon addition of exogenous signaling molecules, the protease activity of P. fluorescens increased relative to the control group. The proteolytic activity in P. fluorescens was also determined by an azocasein assay, with the unit of enzyme activity per hour per gram of protein defined as one unit of proteolytic activity. The results demonstrated that proteolytic activity in P. fluorescens treated with C4-HSL and C14-HSL increased significantly (P < 0.05) compared to the control group, with the proteolytic activity reaching 0.42 and 0.40 h–1μg–1, respectively. Moreover, the proteolytic activity of the other samples also increased, except for C10-HSL group (Figure 2B). These results are in agreement with the observations of Zhu et al. (2015), who found that C14-HSL, 3-oxo-C6-HSL, 3-oxo-C8-HSL, and cyclo-(L-Pro-L-Leu) increased the extracellular proteolytic activity of S. baltica SA02, whereas a contrary effect was found with the addition of C6-HSL, C8-HSL, C10-HSL, and C12-HSL. Interestingly, Martins et al. (2014) showed that AHLs have no effect on the proteolytic activity in P. fluorescens 07A and 041, and even these two strains do not produce AHLs. These authors attributed this to the diversity of P. fluorescens, which the results of this paper undoubtedly confirmed this again.
As previously mentioned, AHLs secreted by bacteria can specifically bind to receptors, resulting in expression of downstream target genes and changes to their own composition. Accordingly, GC–MS was used to monitor the content changes of added exogenous AHLs in P. fluorescens cultures to determine whether P. fluorescens was directly capable of AHLs eavesdropping. Culture time was strictly controlled at 24 h to avoid the negative effects of the alkaline environment and the accumulation of acylase on the AHLs that occur with extended culturing (>30 h) (data not shown). GC–MS verified the eavesdropping of P. fluorescens on exogenous AHLs, with an 82.5% reduction of C14-HSL after culturing with P. fluorescens for 24 h (Table 2). Cultures without P. fluorescens showed no significant AHLs depletion. Similarly, the determination of β-galactosidase by Zhu et al. (2018) revealed that S. baltica could eavesdrop on the ambient AHLs produced by Acinetobacter, and AHL activity of pure Acinetobacter culture was significantly higher than that of the co-culture of S. baltica and Acinetobacter with an inoculation ratio of 1:1. Notably, the content of C4-HSL in P. fluorescens cultures did not change dramatically after incubation compared to that of C14-HSL, although C4-HSL plays the most important role in biofilm and protease experiments. This finding is likely due to the continuous supplementation of C4-HSL into the media via production by P. fluorescens (Supplementary Figure S2). Moreover, the content of C10-HSL in P. fluorescens did not change significantly before and after incubation, with the concentration maintained at 1.731 μg/mL, which was the highest among the six AHLs. This suggested that C10-HSL was not used by P. fluorescens, which was consistent with the results of the biofilm and protease assays.
Previously, the whole genome of the test strain was sequenced and analyzed by Novogene Bioinformatics Technology Co., Ltd. (Beijing, China) to identify the QS-related genes in the P. fluorescens used in this study. The gene functions were predicted (E-value <1e−5, minimal alignment length percentage >40%) based on Gene Ontology (GO), Non-redundant Protein Database (NR), and Kyoto Encyclopedia of Genes and Genomes (KEGG) databases. Further, the predictive function of selected genes was verified by searching in the GenBank sequence database3. Finally, the AHLs synthetase gene (rhlI), QS signaling molecules receptor protein gene (rhlR), spoilage-related alkaline proteinase gene (aprA), and biofilm-related flagellar gene (flgA) were found in P. fluorescens.
In this study, the effect of synthesized AHLs on the transcription of rhlI, rhlR, aprA, and flgA was further measured using qPCR to evaluate whether the promotion of biofilm formation and protease activity by exogenous AHLs was related to the rhl-mediated QS system in P. fluorescens. The results verified that C4-HSL, C6-HSL, C8-HSL, C12-HSL, and C14-HSL promoted the transcription level of these genes, which indicated that P. fluorescens could sense the exogenous AHLs in the environment. Further, upregulation of QS genes caused by exogenous AHLs could explain the promotion of biofilm formation and protease activity in P. fluorescens. However, the rhlR transcription was slightly downregulated in response to C10-HSL, suggesting a specific selectivity of AHLs in regulating QS system in P. fluorescens. Similarly, Zhu et al. (2016) indicated that cyclo-(L-Pro-L-Leu) as exogenous autoinducers promoted the transcription levels of luxR and torA in both S. baltica 02 and S. baltica 08, while no significant changes were observed in the presence of cyclo-(L-Pro-L-Phe) or 4,5-dihydroxy-2,3-pentanedione.
The results of qPCR in this study verified the expression change of rhlR caused by exogenous AHLs. Therefore, the computer-aided molecular docking technique which has been widely applied to the prediction of binding-conformation of small molecule ligands to their receptors was used to explore the recognition and interaction of RhlR-type protein with AHLs in P. fluorescens. The docking results confirmed that all exogenous AHLs, like the native ligand C4-HSL, rigidly docked into the RhlR binding pocket (Figure 4B). The results suggested that the RhlR protein in P. fluorescens could combine with environmental signaling molecules. Interestingly, the docking scores of C4-HSL and C14-HSL were lower than those of others. This indicated that the binding ability of C4-HSL and C14-HSL to RhlR protein is not better than other AHLs, whereas C10-HSL exhibited the strongest binding affinity with RhlR protein (Supplementary Table S4).
Levels of TVB-N mainly reflect the accumulation of biogenic amines and other compounds derived from protein degradation by bacteria and are thus regarded as an essential quality parameter for assessing aquatic products spoilage, especially during cold storage. In this study, the regulation of exogenous AHLs on spoilage potential was measured by monitoring the production of TVB-N by P. fluorescens in turbot filets. As shown in Figure 5, the initial TVB-N values were similar in all the samples. However, the production of TVB-N was significantly stimulated in the presence of exogenous C4-HSL, C6-HSL, C8-HSL, C12-HSL, and C14-HSL, while no significant effect of exogenous C10-HSL was observed. On day 9, the TVB-N value of C4-HSL treated groups reached 45.11 mg N/100 g, which exceeded the acceptable limit of 30 mg N/100 g for marine fish products (Ding et al., 2014). Furthermore, the results also showed that the TVB-N value of blank control always kept at a low level, indicating that other bacteria contributed less to the spoilage of turbots. The result indicated the crucial role of environmental AHLs in the spoilage process of turbot caused by P. fluorescens. These results also fall in line with those of Zhang et al. (2016b), who found that C4-HSL and C6-HSL significantly increased the production of TVB-N in vacuum-packaged farmed turbot, while the addition of exogenous 3-oxo-C6-HSL had no significant effect. Similarly, Zhao et al. (2017) indicated that the addition of exogenous AHLs promoted the production of TVB-N by Aeromonas veronii in surimi juice.
Conclusion
In conclusion, this study validated the involvement of environmental AHLs on turbot spoilage via the promotion of QS phenotypes in P. fluorescens, which are required for fish spoilage. Furthermore, an interesting potential utilization ability of environmental AHLs by P. fluorescens was found based on the results of qPCR and molecular docking analysis. Although this utilization should be further elucidated by constructing mutant strains deficient in the RhlR receptor, the results obtained here still provide insight into the role of AHLs eavesdropping in QS-based spoilage regulation of P. fluorescens. Moreover, the study also suggested that some QS inhibitors, such as some AHLs-quenching enzymes, should not focus solely on the degradation of AHLs secreted by SSO and the results could help formulate new strategies for QS control to further reduce the post-harvest loss of aquatic products.
Data Availability Statement
The datasets generated for this study can be found in the GenBank accession number: CP032618.
Author Contributions
TL conceived and designed the experiments, analyzed the data, contributed reagents, materials, and analysis tools, authored or reviewed drafts of the manuscript, and approved the final draft. DW and LR conceived and designed the experiments, performed the experiments, analyzed the data, prepared the figures, and/or tables, and authored or reviewed drafts of the manuscript. HC performed the GC–MS analysis. YM prepared the figures and/or tables. TD performed the docking molecular docking analysis. QL authored or reviewed drafts of the manuscript. JL conceived and designed the experiments, approved the final draft, and had overall responsibility for this project.
Funding
This study was supported by the National Natural Science Foundation of China (No. 31471639), the National Key Research and Development Program of China (Nos. 2018YFD0400601 and 2017YFD0400106), the Opening Foundation of University-Enterprise Alliance of Food Industry in Liaoning Province (2018LNSPLM0104), and the Open Project Program of Beijing Key Laboratory of Flavor Chemistry (SPFW2019YB08).
Conflict of Interest
The authors declare that the research was conducted in the absence of any commercial or financial relationships that could be construed as a potential conflict of interest.
Acknowledgments
We thank the Novogene Bioinformatics Technology Co., Ltd. for the work in genome sequencing and analysis. We also thank the executive editor at Editage Inc. for English editing.
Supplementary Material
The Supplementary Material for this article can be found online at: https://www.frontiersin.org/articles/10.3389/fmicb.2019.02716/full#supplementary-material
Footnotes
References
Almeida, F. A., Pimentel, N. D. J., Pinto, U. M., Mantovani, H. C., Oliveira, L. L., and Vanetti, M. C. D. (2017). Acyl homoserine lactone-based quorum sensing stimulates biofilm formation by Salmonella Enteritidis in anaerobic conditions. Arch. Microbiol. 199, 475–486. doi: 10.1007/s00203-016-1313-6
Bai, A. J., and Rai, V. R. (2011). Bacterial quorum sensing and food industry. Compr. Rev. Food Sci. 10, 184–194.
Bandlitz, S., and Berke, A. (2013). Use of desirability approach to predict the inhibition of Pseudomonas fluorescens, Shewanella putrefaciens and Photobacterium phosphoreum in fish fillets through natural antimicrobials and modified atmosphere packaging. Food Bioprocess. Tech. 6, 2319–2330. doi: 10.1007/s11947-012-0889-3
Benkert, P., and Tosatto, S. D. (2008). QMEAN: a comprehensive scoring function for model quality assessment. Proteins 71, 261–277. doi: 10.1002/prot.21715
Biasini, M., Bienert, S., Waterhouse, A., Arnold, K., STUDER, G., Schmidt, T., et al. (2014). SWISS-MODEL: modelling protein tertiary and quaternary structure using evolutionary information. Nucleic Acids Res. 42, W252–W258. doi: 10.1093/nar/gku340
Bronesky, D., Wu, Z., and Marzi, S. (2016). Staphylococcus aureus RNAIII and its regulon link quorum sensing, stress responses, metabolic adaptation, and regulation of virulence gene expression. Annu. Rev. Microbiol. 70:299. doi: 10.1146/annurev-micro-102215-095708
Case, R. J., Labbate, M., and Kjelleberg, S. (2008). AHL-driven quorum-sensing circuits: their frequency and function among the Proteobacteria. ISME J. 2, 345–349. doi: 10.1038/ismej.2008.13
Cheng, F., Ma, A., Zhuang, G., and Fray, R. G. (2018). Exogenous N-acyl-homoserine lactones enhance the expression of flagella of Pseudomonas syringae and activate defence responses in plants. Mol. Plant Pathol. 19, 104–115. doi: 10.1111/mpp.12502
Dalgaard, P. (1995). Qualitative and quantitative characterization of spoilage bacteria from packed fish. Int. J. Food Microbiol. 26, 319–333. doi: 10.1016/0168-1605(94)00137-u
Davies, D. G., Parsek, M. R., Pearson, J. P., Iglewski, B. H., Costerton, J. W., and Greenberg, E. P. (1998). The Involvement of cell-to-cell signals in the development of a bacterial biofilm. Science 280, 295–298. doi: 10.1126/science.280.5361.295
Defoirdt, T. (2017). Quorum-sensing systems as targets for antivirulence therapy. Trends Microbiol. 26, 313–328. doi: 10.1016/j.tim.2017.10.005
Diggle, S. P., Griffin, A. S., Campbell, G. S., and West, S. A. (2007). Cooperation and conflict in quorum-sensing bacterial populations. Nature 450, 411–414. doi: 10.1038/nature06279
Ding, T., Li, T. T., and Li, J. R. (2014). Comprehensive evaluation on freshness of salmon slices at 0°C storage. J. Chin. Inst. Food Sci. Tech. 14, 252–259.
Ding, T., Li, T. T., Wang, Z., and Li, J. R. (2017). Curcumin liposomes interfere with quorum sensing system of Aeromonas sobria and in silico analysis. Sci. Rep. 7:8612. doi: 10.1038/s41598-017-08986-9
Dourou, D., Ammor, M. S., Skandamis, P. N., and Nychas, G. J. (2011). Growth of Salmonella enteritidis and Salmonella typhimurium in the presence of quorum sensing signalling compounds produced by spoilage and pathogenic bacteria. Food Microbiol. 28:10111018. doi: 10.1016/j.fm.2011.02.004
Fu, L., Wang, C., Liu, N., Ma, A. J., and Wang, Y. B. (2018). Quorum sensing, system-regulated genes affect the spoilage potential of Shewanella baltica. Food Res. Int. 107, 1–9. doi: 10.1016/j.foodres.2018.01.067
Fuqua, W. C., Winans, S. C., and Greenberg, E. P. (1994). Quorum sensing in bacteria: the LuxR-LuxI family of cell density-responsive transcriptional regulators. J. Bacteriol. 176, 269–275. doi: 10.1128/jb.176.2.269-275.1994
Galloway, W. R., Hodgkinson, J. T., Bowden, S. D., Welch, M., and Spring, D. R. (2011). Quorum sensing in gram-negative bacteria: small-molecule modulation of AHL and AI-2 quorum sensing pathways. Chem. Rev. 111, 28–67. doi: 10.1021/cr100109t
Gomes, L. C., and Mergulhão, F. J. (2017). SEM analysis of surface impact on biofilm antibiotic treatment. Scanning 2017, 1–7. doi: 10.1155/2017/2960194
Gram, L., and Dalgaard, P. (2002). Fish spoilage bacteria-problems and solutions. Curr. Opin. Biotechnol. 13, 262–266. doi: 10.1016/s0958-1669(02)00309-9
Gui, M., Wu, R., Liu, L., Sun, W., and Li, P. L. (2017). Effects of quorum quenching by AHL lactonase on AHLs, protease, motility and proteome patterns in Aeromonas veronii LP-11. Int. J. Food Microbiol. 252:61. doi: 10.1016/j.ijfoodmicro.2017.04.005
Jie, J., Yu, H., Han, Y., Liu, Z., and Zeng, M. (2018). Acyl-homoserine-lactones receptor LuxR of Shewanella baltica involved in the development of microbiota and spoilage of refrigerated shrimp. J. Food Sci. Technol. 55, 2795–2800. doi: 10.1007/s13197-018-3172-4
Kusada, H., Hanada, S., Kamagata, Y., and Kimura, N. (2014). The effects of N-acylhomoserine lactones, β-lactam antibiotics and adenosine on biofilm formation in the multi-β-lactam antibiotic-resistant bacterium Acidovorax sp. strain MR-S7. J. Biosci. Bioeng. 118, 14–19. doi: 10.1016/j.jbiosc.2013.12.012
Li, T. T., Cui, F. C., Bai, F. L., and Li, J. R. (2016). Involvement of acylated homoserine lactones (AHLs) of Aeromonas sobria in spoilage of refrigerated turbot (Scophthalmus maximus L.). Sensors 16:1083. doi: 10.3390/s16071083
Liu, M., Wang, H., and Griffiths, M. W. (2008). Regulation of alkaline metalloprotease (aprX) promoter by N-acyl homoserine lactone quorum sensing in Pseudomonas fluorescens. J. Appl. Microbiol. 103, 2174–2184. doi: 10.1111/j.1365-2672.2007.03488.x
Lv, L., Li, W., Zheng, Z., Li, D., and Zhang, N. (2018). Exogenous acyl-homoserine lactones adjust community structures of bacteria and methanogens to ameliorate the performance of anaerobic granular sludge. J. Hazard. Mater. 354, 72–80. doi: 10.1016/j.jhazmat.2018.04.075
Maier, R. M., and Soberón-Chávez, G. (2000). Pseudomonas aeruginosa arhamnolipids: biosynthesis and potential applications. Appl. Microbiol. Biotechnol. 54, 625–633. doi: 10.1007/s002530000443
Martins, M. L., Uelinton, M. P., Riedel, K., Vanetti, M. C. D., Mantovani, H. C., and Araújo, E. F. (2014). Lack of AHL-based quorum sensing in Pseudomonas fluorescens isolated from milk. Braz. J. Microbiol. 45, 1039–1046. doi: 10.1590/s1517-83822014000300037
Nychas, G. J., Dourou, D., Skandamis, P., Koutsoumanis, K., Baranyi, J., and Sofos, J. (2009). Effect of microbial cell-free meat extract on the growth of spoilage bacteria. J. Appl. Microbiol. 107, 1819–1829. doi: 10.1111/j.1365-2672.2009.04377.x
Oliveira, G. B., Favarin, L., Luchese, R. H., and McIntosh, D. (2015). Psychrotrophic bacteria in milk: how much do we really know? Braz. J. Microbiol. 46, 313–321. doi: 10.1590/S1517-838246220130963
Papenfort, K., and Bassler, B. L. (2016). Quorum sensing signal-response systems in Gram-negative bacteria. Nat. Rev. Microbiol. 14, 576–588. doi: 10.1038/nrmicro.2016.89
Pinto, U. M., De Souza, V. E., Martins, M. L., and Vanetti, M. C. D. (2007). Detection of acylated homoserine lactones in gram-negative proteolytic psychrotrophic bacteria isolated from cooled raw milk. Food Control 18, 1322–1327. doi: 10.1016/j.foodcont.2006.09.005
Ravn, L., Christensen, A. B., Molin, S., Givskov, M., and Gram, L. (2001). Methods for detecting acylated homoserine lactones produced by Gram-negative bacteria and their application in studies of AHL-production kinetics. J. Microbiol. Methods 44, 239–251. doi: 10.1016/s0167-7012(01)00217-2
Rode, T. M., Langsrud, S., and Holck, A. (2007). Different patterns of biofilm formation in Staphylococcus aureus under food-related stress conditions. Int. J. Food Microbiol. 116, 372–383. doi: 10.1016/j.ijfoodmicro.2007.02.017
Sandoz, K. M., Mitzimberg, M. M., and Schuster, M. (2007). Social cheating in Pseudomonas aeruginosa quorum sensing. Proc.Natl.Acad. Sci. U.S.A. 104, 15876–15881. doi: 10.1073/pnas.0705653104
Schuster, M., Urbanowski, M. L., and Greenberg, E. P. (2004). Promoter specificity in Pseudomonas aeruginosa quorum sensing revealed by DNA binding of purified LasR. Proc. Natl. Acad. Sci. U.S.A. 101, 15833–15839. doi: 10.1073/pnas.0407229101
Smith, J. L., Fratamico, P. M., and Yan, X. (2011). Eavesdropping by bacteria: the role of SdiA in Escherichia coli and Salmonella enterica, serovar typhimurium quorum sensing. Foodborne Pathog. Dis. 8, 169–178. doi: 10.1089/fpd.2010.0651
Stuknytė, M., Decimo, M., Colzani, M., Silvetti, T., Brasca, M., Cattaneo, S., et al. (2016). Extracellular thermostable proteolytic activity of the milk spoilage bacterium Pseudomonas fluorescens PS19 on bovine caseins. J. Dairy Sci. 99, 4188–4195. doi: 10.3168/jds.2016-10894
Vijayaraghavan, P., and Vincent, S. G. P. (2013). Simple method for the detection of protease activity on agar plates using bromocresolgreen dye. J. Biochem. Tech. 4, 628–630.
Waterhouse, A., Bertoni, M., Bienert, S., Studer, G., Tauriello, G., Gumienny, R., et al. (2018). Swiss-model: homology modelling of protein structures and complexes. Nucleic Acids Res. 46, W296–W303. doi: 10.1093/nar/gky427
Yan, A. C., Huang, X. Q., Liu, H. M., Dong, D. X., Zhang, D. B., Zhang, X. H., et al. (2007). An rhl-like quorum-sensing system negatively regulates pyoluteorin production in Pseudomonas sp M18. Microbiology 153(Pt 1), 16–28. doi: 10.1099/mic.0.29211-0
Yang, B., Li, T. T., and Li, J. R. (2017). Isolation, identification and quorum sensing of dominant spoilage bacteria from Scophthalmus maximus. J. Chin. Inst. Food Sci. Tech. 17, 139–145.
Yi, S. M., Li, J. R., Zhu, J. L., Lin, Y., Fu, L. L., Chen, W., et al. (2011). Effect of tea polyphenols on microbiological and biochemical quality of Collichthys fish ball. J. Sci. Food Agric. 91, 1591–1597. doi: 10.1002/jsfa.4352
Yuan, L., Hillman, J. D., and Progulske, F. A. (2005). Microarray analysis of quorum-sensing-regulated genes in Porphyromonas gingivalis. Infect. Immun. 73, 4146–4154. doi: 10.1128/iai.73.7.4146-4154.2005
Zhang, C., Zhu, S., Jatt, A. N., and Zeng, M. Y. (2016a). Characterization of N-acyl homoserine lactones (AHLs) producing bacteria isolated from vacuum-packaged refrigerated turbot (Scophthalmus maximus) and possible influence of exogenous AHLs on bacterial phenotype. J. Gen Appl Microbiol. 62, 60–67. doi: 10.2323/jgam.62.60
Zhang, C., Zhu, S., Wu, H., Jatt, A. N., Pan, Y. R., and Zeng, M. Y. (2016b). Quorum sensing involved in the spoilage process of the skin and flesh of vacuum-packaged farmed turbot (Scophthalmus maximus) stored at 4°C. J. Food Sci. 81, M2776–M2784.
Zhang, Q., Ye, K., Wang, H., Xiao, H., Xu, X., and Zhou, G. (2014). Inhibition of biofilm formation of Pseudomonas aeruginosa by an acylated homoserine lactones-containing culture extract. LWT Food Sci. Technol. 57, 230–235. doi: 10.1016/j.lwt.2013.12.022
Zhao, D., Fei, L., Liu, S., Zhang, J. Y., Ding, Y. T., Chen, W. X., et al. (2017). Involvement of bacterial quorum sensing signals in spoilage potential of Aeromonas veronii bv. veronii isolated from fermented surimi. J. Food Biochem. 42:e12487. doi: 10.1111/jfbc.12487
Zhu, J., Zhao, A., Feng, L., and Gao, H. (2016). Quorum sensing signals affect spoilage of refrigerated large yellow croaker (Pseudosciaena crocea) by Shewanella baltica. Int. J. Food Microbiol. 217, 146–155. doi: 10.1016/j.ijfoodmicro.2015.10.020
Zhu, S., Wu, H., Zeng, M., Liu, Z., and Wang, Y. (2015). The involvement of bacterial quorum sensing in the spoilage of refrigerated Litopenaeus vannamei. Int. J. Food Microbiol. 192, 26–33. doi: 10.1016/j.ijfoodmicro.2014.09.029
Keywords: quorum sensing, Pseudomonas fluorescens, spoilage potential, eavesdropping, molecular docking
Citation: Li T, Wang D, Ren L, Mei Y, Ding T, Li Q, Chen H and Li J (2019) Involvement of Exogenous N-Acyl-Homoserine Lactones in Spoilage Potential of Pseudomonas fluorescens Isolated From Refrigerated Turbot. Front. Microbiol. 10:2716. doi: 10.3389/fmicb.2019.02716
Received: 04 July 2019; Accepted: 08 November 2019;
Published: 29 November 2019.
Edited by:
Lin Lin, Jiangsu University, ChinaReviewed by:
Uelinton Manoel Pinto, University of São Paulo, BrazilMaria Cristina D. Vanetti, Universidade Federal de Viçosa, Brazil
Copyright © 2019 Li, Wang, Ren, Mei, Ding, Li, Chen and Li. This is an open-access article distributed under the terms of the Creative Commons Attribution License (CC BY). The use, distribution or reproduction in other forums is permitted, provided the original author(s) and the copyright owner(s) are credited and that the original publication in this journal is cited, in accordance with accepted academic practice. No use, distribution or reproduction is permitted which does not comply with these terms.
*Correspondence: Jianrong Li, lijr6491@163.com