- 1Department of Endodontics, Faculty of Dentistry, Adnan Menderes University, Aydin, Turkey
- 2Department of Biology, Faculty of Arts and Sciences, Adnan Menderes University, Aydin, Turkey
- 3Molekulare Biotechnologie, Fachbereich Biowissenschaften, Buchmann Institute for Molecular Life Sciences (BMLS), Goethe Universität Frankfurt Biozentrum, Frankfurt am Main, Germany
- 4Department of Microbiology, Faculty of Medicine, Adnan Menderes University, Aydin, Turkey
Xenorhabdus and/or Photorhabdus bacteria produce antibacterial metabolites to protect insect cadavers against food competitors allowing them to survive in nature with their nematode host. The effects of culture supernatant produced by Xenorhabdus and Photorhabdus spp. were investigated against the multidrug-resistant dental root canal pathogen Enterococcus faecalis. The efficacy of seven different cell-free supernatants of Xenorhabdus and Photorhabdus species against E. faecalis was assessed with overlay bioassay and serial dilution techniques. Additionally, time-dependent inactivation of supernatant was evaluated. Among the seven different bacterial species, X. cabanillasii produced the strongest antibacterial effects. Loss of bioactivity in a phosphopantetheinyl transferase-deficient mutant of X. cabanillasii indicated that this activity is likely based on non-ribosomal peptide synthetases (NRPSs) or polyketide synthases (PKSs). Subsequent in silico analysis revealed multiple possible biosynthetic gene clusters (BGCs) in the genome of X. cabanillasii including a BGC homologous to that of zeamine/fabclavine biosynthesis. Fabclavines are NRPS-derived hexapeptides, which are connected by PKS-derived malonate units to an unusual polyamine, also PKS-derived. Due to the known broad-spectrum bioactivity of the fabclavines, we generated a promoter exchange mutant in front of the fabclavine-like BGC. This leads to over-expression by induction or a knock-out by non-induction which resulted in a bioactive and non-bioactive mutant. Furthermore, MS and MS2 experiments confirmed that X. cabanillasii produces the same derivatives as X. budapestensis. The medicament potential of 10-fold concentrated supernatant of induced fcl promoter exchanged X. cabanillasii was also assessed in dental root canals. Calcium hydroxide paste, or chlorhexidine gel, or fabclavine-rich supernatant was applied to root canals. Fabclavine-rich supernatant exhibited the highest inactivation efficacy of ≥3 log10 steps CFU reduction, followed by calcium hydroxide paste (≤2 log10 step). The mean percentage of E. faecalis-free dental root canals after treatment was 63.6, 45.5, and 18.2% for fabclavine, calcium hydroxide, and chlorhexidine, respectively. Fabclavine in liquid form or preferably as a paste or gel formulation is a promising alternative intracanal medicament.
Introduction
Enterococcus faecalis is a species of the Enterococci that is associated with humans as part of the microbiota of the gastrointestinal system. However, the bacterium sometimes is an opportunistic human pathogen (Karchmer, 2000). E. faecalis is the most common etiological agent of human enterococcal infections (Kayaoglu and Orstavik, 2004). Moreover, this bacterium can exist as a nosocomial infection and result in mortalities surpassing 50% in some immunocompromised and cancer patients (Schmidt-Hieber et al., 2007; Arias and Murray, 2012).
In dentistry, E. faecalis is linked to persistent periradicular lesions with major endodontic infections and persistent infections of the root canal (Sanchez-Sanhueza et al., 2015). Eradication of E. faecalis is challenging because it creates a biofilm, utilizes diverse compounds as energy sources, and survives extreme environmental conditions (Gilmore et al., 2002; Tendolkar et al., 2003). These characteristics contribute to bacterial tenacity and virulence in tooth infections (Stojicic et al., 2010).
The number of bacterial cells can be reduced by shaping of the root canal with mechanical instrumentation and irrigation with antimicrobial agents. However, these techniques are inept in adequately eliminating E. faecalis due to the complex anatomy of the root canal system (Stuart et al., 2006; Vianna and Gomes, 2009; Asnaashari et al., 2017). Accordingly, intracanal treatment is recommended for lowering the number of bacteria before filling the root canal (Bystrom et al., 1985). Calcium hydroxide (Ca(OH)2) pastes and chlorhexidine (CHX) gels are commonly used as intracanal medicaments (Siqueira and de Uzeda, 1997; Tervit et al., 2009).
Even though CHX and Ca(OH)2 are regular intracanal medicaments in endodontic therapy, previous studies have revealed that E. faecalis can still persist (Orstavik and Haapasalo, 1990; Heling et al., 1992; Evans et al., 2002; Delgado et al., 2010). Moreover, an effective antibiotic to decolonize patients with antibiotic-resistant E. faecalis is unknown or unavailable. The health care concern posed by E. faecalis stresses the pressing urgency for new approaches for decolonization and therapeutic treatment. The discovery of novel antibiotics or antibacterial agents can serve as alternatives for E. faecalis suppression. The most important antibacterial sources in nature are viruses (Suttle, 1994; Fuhrman, 1999), fungi (Brian and Hemming, 1947; Zhang et al., 2012), bacteria (Kirkup, 2006; Gillor and Ghazaryan, 2007; Newman and Cragg, 2016), and plants (Cowan, 1999). Various fungi and bacteria produce antimicrobial compounds as secondary metabolites to compete with other organisms. Among bacteria, research over the past three decades has shown that the genera Photorhabdus and Xenorhabdus produce antimicrobial compounds that may have potential use against an array of bacterial pathogens (Boemare and Akhurst, 2006; Shi and Bode, 2018).
Xenorhabdus and Photorhabdus species are insect pathogenic bacteria that are symbiotically associated with nematodes in the families Steinernematidae and Heterorhabditidae, respectively (Hazir et al., 2003). These entomopathogenic nematodes (EPNs) with their bacteria are obligate, lethal parasites of soil insects. The symbiotic organisms have many positive attributes such as safety to humans and nontarget organisms and ease of mass production (Fodor et al., 2017).
The nematode-killed insect is protected from secondary invasion by contaminating organisms that allow the nematodes to develop in the cadaver. The protection is provided by Photorhabdus and Xenorhabdus by the production of a variety of small antibiotic molecules. For example, Xenorhabdus spp. synthesize a variety of secondary metabolites including antimicrobials made of linear and cyclic peptides (Bode, 2009; Shi and Bode, 2018). To date, the compounds examined from X. bovienii, X. nematophila, and X. cabanillasii, are indole, xenorhabdin, xenocoumacin, PAX peptides, and cabanillasin with antibacterial, antifungal, or both activities (Gu et al., 2009; Hazir et al., 2016). It was stated that Photorhabdus species also generate antimicrobial compounds including isopropylstilbenes and the β-lactam carbapenem (Webster et al., 2002). Some of these compounds especially from P. temperata and P. luminescens subsp. luminescens are known to have antibiotic properties. The trans-stilbenes and anthraquinone pigments were detected as antibacterial (Boemare and Akhurst, 2006). These findings of antimicrobial compounds have attracted considerable interest for pharmaceutical and agronomic purposes (Webster et al., 2002; Hazir et al., 2016). It is known that different species/strains of Xenorhabdus and Photorhabdus produce various antimicrobial compounds. Hence, we hypothesized that some species of Xenorhabdus and/or Photorhabdus spp. produce active compound(s) in their secondary metabolites that will inhibit the growth of antibiotic-resistant E. faecalis. Accordingly, we tested this hypothesis with seven different supernatants of Xenorhabdus and Photorhabdus species against antibiotic-resistant E. faecalis with in vitro antibacterial tests. Subsequently, the medicament potential of the antibacterial compound obtained from the most effective species was compared with CHX and Ca(OH)2 against E. faecalis in root canals.
Materials and Methods
Source of Bacteria and Supernatant Preparation
Antibiotic activity of seven bacterial isolates of Xenorhabdus and Photorhabdus was tested against E. faecalis. The nematode species and strains from which each tested bacterial species or subspecies was isolated is presented in Supplementary Table S1. Henceforth, we will refer to all isolates as bacterial species even though we recognize that P. luminescens includes two subspecies (luminescens and laumondii).
Bacterial isolates were recovered from nematode-infected Galleria mellonella (Lepidoptera: Pyralidae) larva (Kaya and Stock, 1997). Xenorhabdus and Photorhabdus bacteria have phase-changing capabilities when cultured in vitro. Phase-I is associated with nematodes and produces toxins, enzymes, antibiotics, etc. that provide better support for nematode growth in insect cadavers, whereas phase-II occurs spontaneously under unfavorable conditions or long incubation periods (Leclerc and Boemare, 1991; Boemare, 2002). Thus, we used only phase-I of each of the bacterial isolates as observed by their distinctive colony and cell morphology on NBTA (nutrient agar 31 g/L, bromothymol blue 25 mg/L, and 2,3,5-triphenyl tetrazolium chloride 40 mg/L) plates and by a catalase test. After isolating the phase-I bacterium, each isolate was stored at −80°C until further use (Boemare and Akhurst, 2006).
To conduct the experiments, each bacterial isolate was streaked onto a NBTA plate and after 24 h, a loopful of bacterial cells was transferred to 100 ml of Tryptic Soy Broth (TSB) (Difco, Detroit, MI) in an Erlenmeyer flask. Because the optimum time for antibiotic production is 120–144 h (Furgani et al., 2008), cultures were incubated at 28°C and 150 rpm for 144 h (Hazir et al., 2016). Later on, supernatants were obtained from the centrifuged bacterial culture at 4°C and 20,000 g for 15 min. To eliminate all bacterial cells, the supernatants were filtered through a 0.22-μm Millipore filter (Thermo scientific, NY). Each cell-free supernatant was stored at 4°C in sterile falcon tubes (Corning, NY) and used within 2 weeks.
Pathogen Cultures
Multidrug-resistant Enterococcus faecalis V583 (ATCC 700802) was used for the experiments. The strain was grown overnight in Trypticase Soy broth (TSB) (Merck) (Awori et al., 2016) and the bacterial stock suspension was kept at −80°C (Boemare and Akhurst, 2006).
Antibacterial Activity of Different Photorhabdus and Xenorhabdus Spp. Against Enterococcus faecalis
Overlay Bioassay
Cell-to-cell competition of test bacteria with the antibiotic producer colony on a solid media (slightly modified method of Furgani et al., 2008) was used for the antibacterial test. The overlay bioassay permitted us to assess the efficacy of different species of antibiotic producing Xenorhabdus and Photorhabdus spp. against E. faecalis by measuring the inactivation zones. Xenorhabdus and Photorhabdus cultures were prepared by inoculating a loopful of bacteria from NBTA plate into 50 ml of TSB and incubating them at 150 rpm and 28°C in an incubator overnight. A 5-μl bacterial sample from the overnight culture was transferred onto the center of Mueller Hinton Agar (MHA) (Merck) plates. The bacteria were incubated for 5 days at 28°C (Furgani et al., 2008). For preparation of overnight E. faecalis culture, E. faecalis was inoculated in 50 ml of TSB medium and incubated at 37°C and 150 rpm. A volume of 100 μl of the pathogen culture (44 × 108 CFU/ml) was added to 3.5 ml of soft agar (0.6% w/v) at 45–50°C, which was then poured into the test plates where the Xenorhabdus or Photorhabdus colony had been growing. To prevent bacterial expansion on agar media, the propagated Xenorhabdus or Photorhabdus colony was left under UV light for 5 min before layering the mixture of E. faecalis and soft agar over the plate. After the solidification of soft agar, the petri dishes were incubated for 48 h at 37°C. The zone diameter around the colony of antibiotic-producing cells was measured in two directions perpendicular to each other and the average was taken (Mattigatti et al., 2012). Each bacterial species had 10 replicates and the experiment was conducted three times.
Antibacterial Activity of Cell-Free Xenorhabdus or Photorhabdus Supernatants
The cell-free supernatants of all species of Xenorhabdus and Photorhabdus listed in Supplementary Table S1 were tested against E. faecalis. Different proportions (1, 5, 10, 20, 30, 40, 50, and 100%) of supernatants containing bacterial metabolites were tested for inactivation of growth of E. faecalis. For each proportion (1, 5, 10, 20, 30, 40, 50, and 100%), filtrated supernatants were incorporated on a v/v basis into test tubes with 2 ml of sterile Mueller Hinton Broth (MHB) (Merck) (Gualtieri et al., 2012). According to supernatant proportions, the same amount of MHB was discarded before adding bacterial supernatant (for example, for 5%, 0.1 ml supernatant was incorporated into 1.9 ml of MHB). A volume of 10 μl of a culture of E. faecalis (44 × 108 CFU/ml) incubated overnight was pipetted into the test tubes containing MHB and cell-free supernatants. There were positive and negative control groups. Positive control included MHB and E. faecalis, whereas the negative control was only cell-free supernatant. The tubes were incubated in the shaker incubator at 150 rpm and 37°C for 48 h. Following the incubation period, bacterial growth was evaluated visually to determine maximum inhibiting dilutions (MIDs; according to Furgani et al., 2008, we used the term “dilution” not concentration). The MID is the maximum supernatant dilution that yields no visible growth. In each series of tubes, the last tube with clear supernatant was considered to be without any growth and was assumed to give the MID value. Turbidity in the tubes indicated growth of E. faecalis. Visual evaluations were made independently by three examiners and a consensus opinion was agreed upon (Furgani et al., 2008; Aarati et al., 2011). An aliquot of 100 μl was taken from each tube where no bacterial growth had been observed visually and was transferred to the blood-agar medium (5% sheep blood) to determine maximum bactericidal dilution (MBD). After streaking the subsamples on the blood-agar medium, the petri dishes were incubated at 37°C for 48 h to verify total inactivation. The smaller the MID or MBD values, the stronger the antibiotic production obtained (Furgani et al., 2008). National Committee for Clinical Laboratory Standart Institute (CLSI) recommended procedures were used for MID and MBD determination.
Three replicates were used for each supernatant proportion and the experiment was conducted three times.
Time-Dependent Inactivation of Cell-Free Supernatant
Depending on the results of the experiment on overlay bioassay and the MID and MBD values, bacterial supernatant that produced the maximum antibacterial activity (X. cabanillasii supernatant) was used to determine the time to inactivation of E. faecalis. This was done by using 25 ml of sterile TSB mixed with 25 ml of cell-free supernatant of X. cabanillasii in a 100-ml flask. A 0.5-ml aliquot of E. faecalis from an overnight culture (44 × 108 CFU/ml) was transferred to the 50% supernatant, and afterward the flask was incubated at 37°C at 150 rpm. On a 2-h basis from 0 to 16 h, a 10-μl subsample was pipetted from the flask and spread on a blood agar. Plates were incubated for 48 h at 37°C. Three replicates were used and the experiment was conducted three times.
Identification of Bioactive Antibacterial Compound
To determine the bioactive compound, promoter exchanged mutants of X. cabanillasii were generated and matrix assisted laser desorption/ionization-mass spectrometry (MALDI-MS) and MALDI-MS2 experiments were performed.
Generation of Deletion and Promoter Exchange Mutants in Xenorhabdus cabanillasii
Due to the phosphopantetheinyl transferase (PPTase)-dependence of NRPS- and PKS-derived secondary metabolites, we deleted the responsible gene in X. cabanillasii. This should lead to a loss of production and bioactivity in the mutant if the compound is NRPS- or PKS-derived.
Deletion of the phosphopantetheinyl transferase (Xcab_04003) in X. cabanillasii was performed by double homologous recombination. About 1,000 bps were amplified by PCR with the primers SW305_Xcab_LF_fw (5′-CGATCCTCTAGAGTCGACCTGCAGTGTATAGGTCATAGCGCATTTTCC-3′) and SW306_Xcab_LF_rv (5′-TTTCATCTCTTATTTTGTTGTTCTTGGGTATTGTTCG-3′) for the upstream and SW307_Xcab_RF_fw (5′-TACCCAAGAACAACAAAATAAGAGATGAAAACCCCGG-3′) and SW308_Xcab_RF_rv (5′-GAGAGCTCAGATCTACGCGTTTCATATGGGTTTTAGCCCAATCTTATGCC-3′) for the downstream regions. Both were integrated by Hot Fusion assembly into the PstI/NdeI digested deletion vector pDS132 and transformed into E. coli ST18 (Fu et al., 2014). The plasmid pDS132 containing the sacB gene and a kanamycin resistance cassette. X. cabanillasii was conjugated with E. coli ST18 and insertion mutants were selected on kanamycin-supplemented LB agar plates. The second homologous recombination was then enforced by cultivation on sucrose-supplemented LB agar plates, which is toxic due to the SacB conversion.
Promoter exchange in front of the fcl-homologous gene cluster was performed upstream of the fclC-like gene (Xcab_02060) in X. cabanillasii. The first 1,000 bps of Xcab_02060 were amplified by the primers SW128_Xcab_fw (5′-TTTGGGCTAACAGGAGGCTAGCATATGACCAAGACGTATTTTTTGCATG-3′) and SW129_Xcab_rv (5′-TCTGCAGAGCTCGAGCATGCACATTTTACCTGCCCTTCCAGACG-3′) and cloned into the PCR-amplified vector pCEP_kan by Hot Fusion assembly (Fu et al., 2014; Bode et al., 2015). After transformation, positive E. coli S17 clones were confirmed by restriction, conjugated with X. cabanillasii, and insertion mutants selected on kanamycin-supplemented LB agar plates (Supplementary Table S2). Successfully generated deletion and promoter exchange mutants were verified by colony PCR and analyzed by MALDI-MS.
Identification of Antibacterial Compound by MALDI-MS and MALDI-MS2
Cultures for MALDI-MS measurements were prepared with 10 ml of lysogeny broth media supplemented with kanamycin (50 μg/ml) if appropriate, inoculated with 400 μl of a preculture, and incubated at 30°C for 72 h with shaking. Induced promoter exchange mutants were additionally supplemented with 0.2% L-arabinose (Bode et al., 2015). Liquid cultures were spotted on a steel target with a volume of 0.3 μl mixed with 0.25 μl of 1:10 diluted ProteoMass Normal Mass Calibration Mix (Sequazyme™ Peptide Mass Standards Kit) for internal calibration and 0.9 μl of alpha-Cyano-4-hydroxycinnamic acid (CHCA) matrix (3 mg/ml in 75% acetonitrile, 0.1% trifluoroacetic acid). After air-drying, the sample spot was washed with 5% formic acid and mixed again with 0.6 μl of CHCA. Cell MALDI measurements were performed with a MALDI LTQ Orbitrap XL (Thermo Fisher Scientific, Inc., Waltham, MA) instrument with a nitrogen laser at 337 nm in FTMS scan mode with 100 shots per measurement in a mass range of 350–1,500 m/z with high resolution. MALDI-MS2 experiments were performed in CID-mode using ITMS scan mode with the following parameters: Normalized collision energy, 28; Act. Q, 0.250; and Act. Time (ms), 30.0. Data were analyzed using Qual Browser version 2.0.7 (Thermo Fisher Scientific, Inc., Waltham, MA).
Testing the Antibacterial Activity of Xenorhabdus cabanillasii Mutant Strains
Antibacterial activity of 5-day-old cell-free supernatants of wild-type, pptase deletion mutant, induced (with arabinose) and non-induced (without arabinose) fclC promoter exchange mutant of X. cabanillasii was tested with agar-well diffusion bioassay as described before. Each petri dish included four wells and each well was filled with 70 μl of one of the cell-free X. cabanillasii supernatants. After the incubation period for 48 h at 37°C, the inactivation zones (mm) on plates were measured (Mattigatti et al., 2012). Five replicates were used and the experiment was conducted three times.
Medicament Potential of the Antibacterial Compound in Dental Root Canals
One-day-old cell-free supernatant of induced fclC mutant strain of X. cabanillasii was concentrated 10-fold using an evaporator and tested in dental root canals. Recently extracted human mandibular premolars were collected from patients who signed a patients’ consent protocol (2016/1052) approved by Adnan Menderes University, Local Ethical Committee. Soft tissue remnants and calculus were removed from the external root surfaces by using a periodontal scaler. Periapical radiographs were taken from both buccolingual and mesiodistal directions to determine the teeth that have a straight single root canal. Next, a dental operating microscope (Leica M320) was used to select the teeth with no resorption, defect, or cracks. According to these criteria, 44 mandibular premolar teeth with curvature less than 5° and 15–18 mm long were selected (Schneider, 1971; Pladisai et al., 2016). The roots were sectioned using a diamond disk, perpendicular to the long axis into samples 13 mm long from the cementoenamel junction to the apical root end (Pladisai et al., 2016). A single endodontist removed the pulp tissue and checked the canal patency with a #10 stainless steel K-File (Mani Inc. Tochigi, Japan) until it was visible at the apical foramen. Working length was set at 1 mm short of this length. Then root canals were instrumented with Protaper Next rotary system (Dentsply, Ballaigues, Switzerland) up to X3 (0.30 mm tip with 7% taper) by the same endodontist in a crown-down manner, at a rotational speed of 300 rpm and 200 g/cm torque. Root canals were washed with 2 ml of 5% NaOCl using a 27-gauge notched-tip irrigation needle (Ultradent, UT, USA) between each instrument. At the end of the instrumentation, a final flush was applied using a sequence of 5 ml of 17% EDTA and 5 ml of 5% NaOCl to remove the smear layer, followed by 5 ml of 10% sodium thiosulfate and 5 ml of sterile distilled water (Sasanakul et al., 2019). Finally, specimens were dried with paper points. All teeth were sterilized in an autoclave (121°C for 15 min) before using in the experiments (Alves et al., 2013; Zan et al., 2013).
A 10-μl aliquot of E. faecalis from an overnight culture adjusted to 5 × 105 CFU/ml (Ardizzoni et al., 2009) was placed in the root canals of 44 teeth using a sterile micropipette. The teeth were placed individually in 2-ml capacity sterile centrifuge tubes containing 1 ml of BHI broth and incubated for 21 days at 37°C. This is to allow bacteria to penetrate the dentinal tubules and biofilm formation (Pladisai et al., 2016). The media were replaced with sterile BHI every other day (Sasanakul et al., 2019).
After the incubation period, the teeth were embedded in the silicone impression material to create a closed-end channel (Tay et al., 2010). Then, to imitate the final irrigation process before medicament applications in clinical conditions, the specimens were irrigated with 3 ml of 17% EDTA, 3 ml of 5% NaOCl, 3 ml of 10% sodium thiosulfate, and 3 ml of sterile distilled water, respectively. The root canals were subsequently dried with paper points and they were randomly divided into four groups (n = 11 specimens per group).
The root canals of the first and second groups were filled with Ca(OH)2 paste (Ultracal XS, Ultradent Products Inc., USA) and 2% CHX gluconate gel (Gluco-Chex, Cerkamed, Poland) by using a Lentulo spiral (Dentsply-Maillefer), respectively. The root canals in the third group were filled with 10-fold concentrated supernatant of fclC-induced X. cabanillasii with the help of a sterile syringe. The remaining 11 teeth were filled with sterile BHI medium and this served as the control group. Coronal access of the teeth was closed with parafilm. After the treatments, silicon impression material around the specimens was removed and it was placed in 2-ml sterile centrifuge tubes containing BHI medium individually. The tubes were incubated aerobically at 37°C for 7 days. The media were replaced with sterile BHI every other day.
At the end of experimental period, a ProTaper X3 rotary instrument was used for 20 s to remove CHX gel and Ca(OH)2 paste. For the neutralization of Ca(OH)2, 3 ml of 5% citric acid, and for CHX gel, the mixture of 0.3% L-α-Lecithin and 3% Tween 80 were used. Then both medicament groups were irrigated with 3 ml of physiological saline (Pektas et al., 2013). The specimens in supernatant applied and control group were treated with 6 ml of sterile physiological saline.
The remaining bacteria in the root canals and inner dentins were collected by shaving the root canal walls with a No. 4 Peeso reamer (Dentsply-Maillefer). The collected dentin chips were transferred to 0.5 ml of BHI medium in Eppendorf tube and vortexed vigorously. Then, 50-μl sample was streaked on blood-agar plates. The plates were incubated at 37°C for 48 h and the colonies on blood agar were counted and inferred as colony forming units (CFUs).
Statistical Analysis
SPSS 25.0 (IBM Corp., Chicago, IL, USA) package program with a level of significance set at 0.05 was used. Differences in antibacterial effects of the supernatants were compared with one-way ANOVA and the means separated using Tukey’s test. The data of time-dependent CFU reduction and medicament potential of the antibacterial compound in dental root canals were shown as medians, including 25 and 75% quartiles. In these results, horizontal dotted, solid, and dashed lines represent reductions of 2, 3, and 5 log10 steps CFU, respectively. Medians on or below these lines exhibit bacterial killing efficacy of 99% (2 log10), 99.9% (3 log 10), and 99.999 (5 log 10) (Boyce and Pittet, 2002).
Results
Antibacterial Activity of Different Xenorhabdus and Photorhabdus Spp. Against Enterococcus faecalis
Overlay Bioassay
The antibiotic production of Xenorhabdus and Photorhabdus against E. faecalis differed significantly among species (F = 527.73; df = 6, 202; p < 0.001) (Figure 1). Xenorhabdus cabanillasii had the most pronounced inactivation (50.4 mm) and X. bovienii and P. luminescens laumondii the lowest (Figure 1).
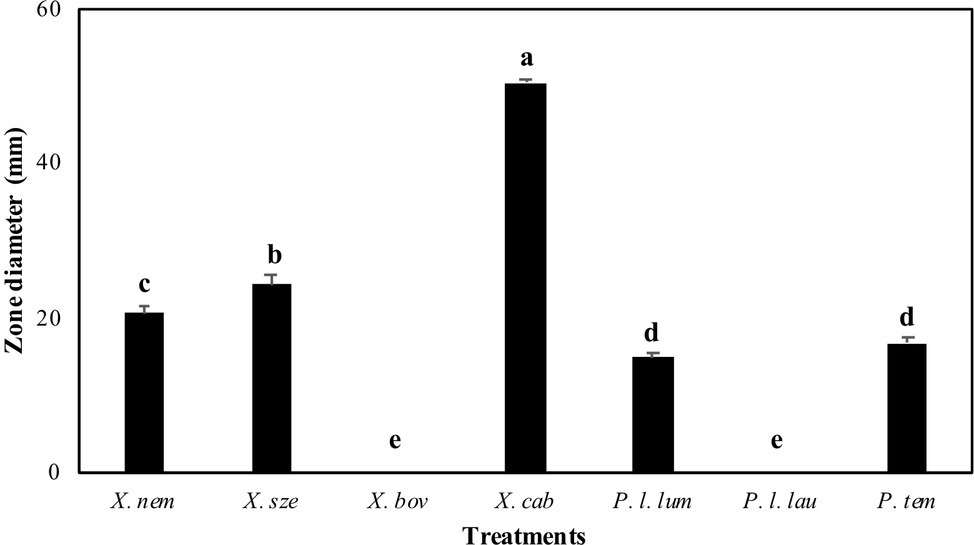
Figure 1. Inactivation zones (mm) resulting from overlay experiments using species of Xenorhabdus and Photorhabdus against Enterococcus faecalis. X. nem, Xenorhabdus nematophilus; X. sze, X. szentirmaii; X. bov, X. bovienii; X. cab, X. cabanillasii; P. l. lum, Photorhabdus luminescens luminescens; P. l. lau, P. luminescens laumondii; P. tem, P. temperata. Means indicated by the different lower-case letters on the bars are significantly different (p < 0.05).
Antibacterial Activity of Cell-Free Xenorhabdus or Photorhabdus Supernatants
All Photorhabdus and X. bovienii supernatants caused inactivation (based on lack of visible growth; MID) when undiluted supernatants were used (Supplementary Table S3). Xenorhabdus nematophila and X. szentirmaii caused inactivation at 20 and 40% proportions of supernatant including cultures, respectively. The greatest inactivation was achieved by X. cabanillasii, which showed inactivation even at a 1% concentration of supernatant. After the transfer of samples where no visually bacterial growth was observed on the blood-agar media, E. faecalis colonies were observed even at undiluted supernatants (100%) of X. nematophila, X. bovienii, and all Photorhabdus species (Supplementary Table S3). Xenorhabdus szentirmaii exhibited complete inactivation (MBD) only at 100% supernatant concentration. Similar to the visual inactivation results, we observed that the 5% supernatant of X. cabanillasii eliminated all cells of E. faecalis (Supplementary Table S3). Enterococcus faecalis proliferated in all positive controls, whereas no bacterial growth was observed in the negative control groups.
Time-Dependent Inactivation of Cell-Free Supernatant
Bacterial culture media that included 50% X. cabanillasii supernatant showed inactivation starting from the time of the first subsample (2 h after inoculation) and the number of colonies of E. faecalis gradually decreased over time. The supernatant killed 99.999% of the bacteria after 2 h. Complete inactivation of E. faecalis occurred 6 h after the inoculation (Figure 2).
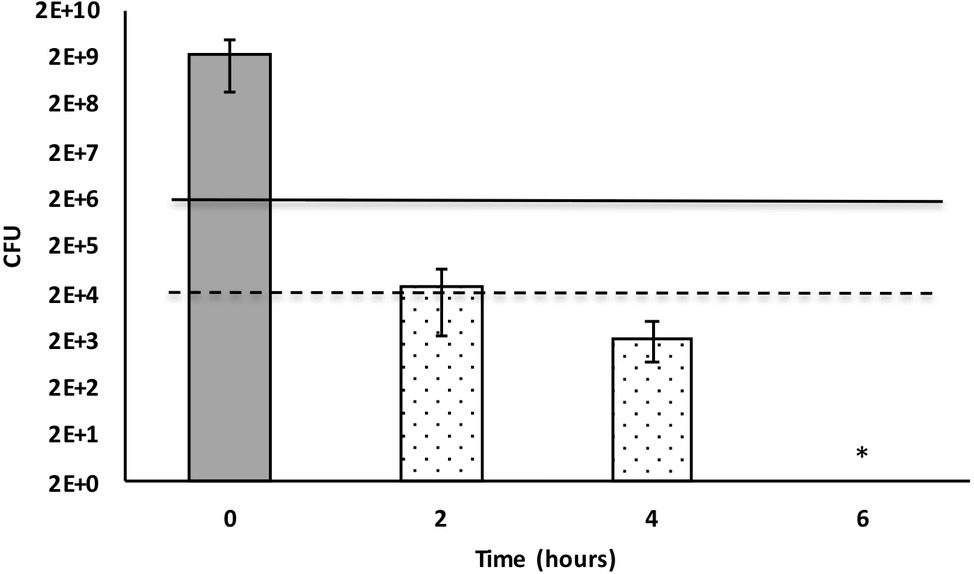
Figure 2. Time-dependent inactivation of Enterococcus faecalis cells incubated in 50% Xenorhabdus cabanillasii supernatant. Data in this figure are shown as CFU medians with 25 and 75% quartiles. Solid and dashed lines represent reductions of ≥3 and ≥ 5 log10 steps CFU, respectively. * indicates reduction below detection limit.
Testing the Antibacterial Activity of Xenorhabdus cabanillasii Mutant Strains
Wild-type and induced fclC promoter exchange mutant of X. cabanillasii supernatants exhibited average of 18 (±1.4) and 17.5 (±0.8) mm zone diameters, respectively. But, there was no inactivation circle around the wells of Δpptase and non-induced fclC mutants (Figure 3).
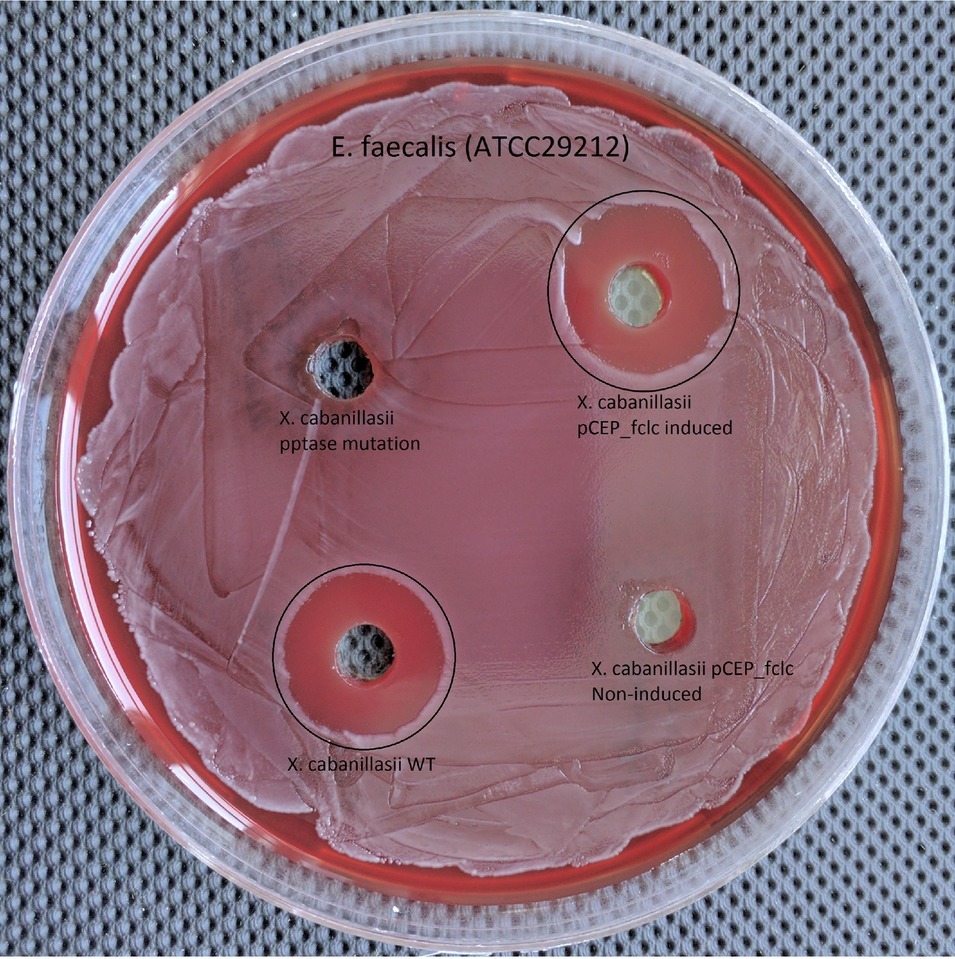
Figure 3. Comparison of wild-type, Δpptase, pCEP-fclC induced, and pCEP-fclC non-induced mutant of X. cabanillasii against Enterococcus faecalis.
Identification of Bioactive Antibacterial Compound Produced by Xenorhabdus cabanillasii
Since the Δpptase mutant showed no more bioactivity (Figure 3), it can be postulated that the responsible compound is generated by a non-ribosomal peptide synthetase (NRPS) or a polyketide synthase (PKS). In silico analysis of the X. cabanillasii genome revealed multiple potential NRPS- and PKS-BGCs. Due to the known broad-spectrum bioactivity of zeamine/fabclavine, we focused on the fcl-homologous BGC (Fuchs et al., 2014; Masschelein et al., 2015a,b). We performed a promoter exchange in front of the first essential biosynthesis gene fclC and observed that the bioactive antibacterial compound is produced by this BGC (Figure 3) (Wenski et al., 2019). High-resolution MALDI-MS comparison revealed that the active compounds are the fabclavines Ia, Ib, IIa, and IIb, which were previously described for X. budapestensis DSM 16342 (Figure 4) as confirmed by the fragmentation pattern of signal 1302.92 (IIb) (Supplementary Table S4 and Supplementary Figure S1).
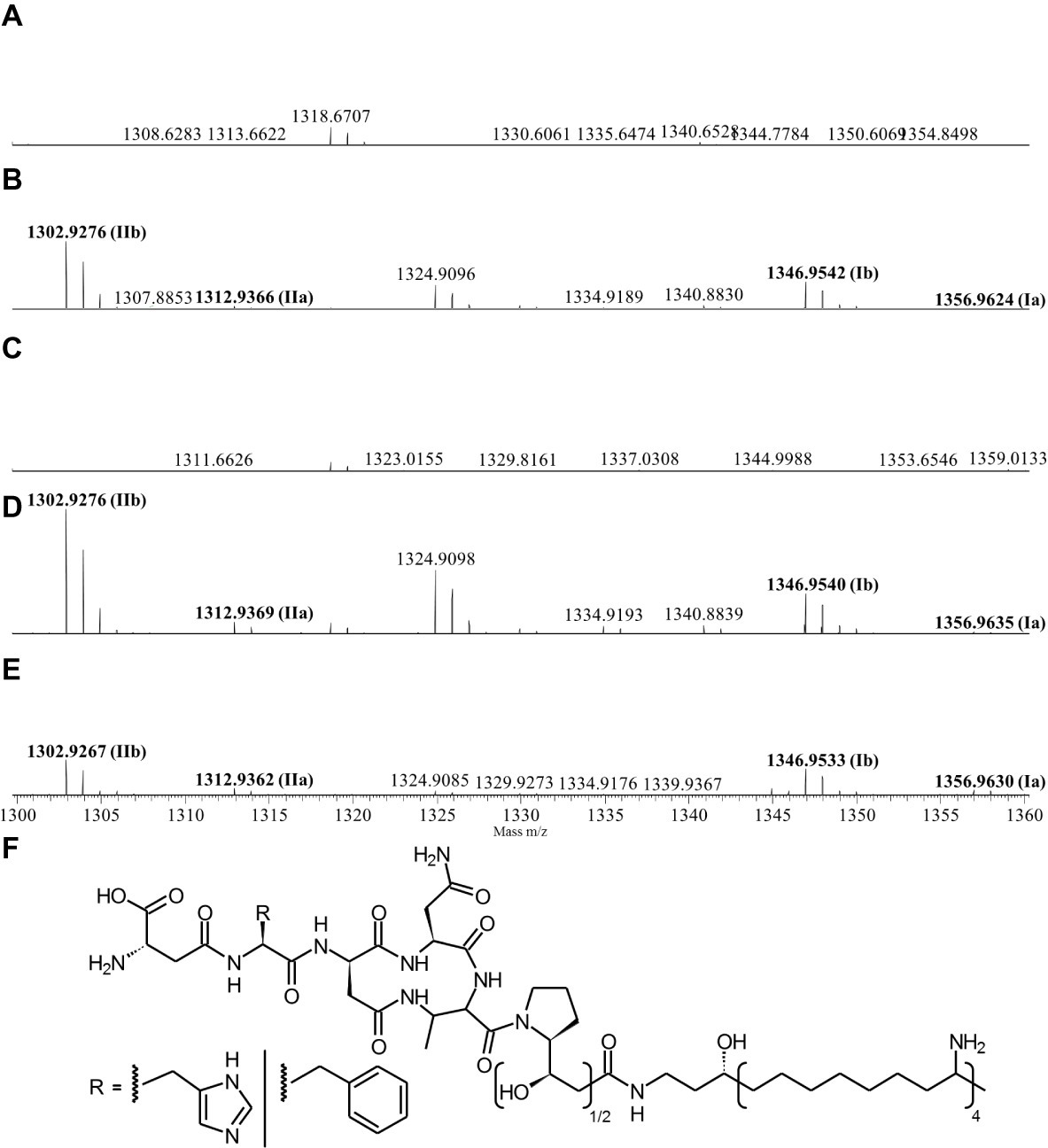
Figure 4. High-resolution MALDI-MS data of liquid cultures of X. cabanillasii ∆ppt (A), X. cabanillasii JM26 WT (B), X. cabanillasii PBad-fclC (non-induced) (C), X. cabanillasii PBad-fclC (induced) (D), X. budapestensis DSM 16342 (E), and the corresponding fabclavine derivatives (F). Highlighted in bold are signals that correspond with identified fabclavines in X. budapestensis (Fuchs et al., 2014). Cultures were grown for 72 at 30°C. MALDI-MS measurement was internally calibrated.
Medicament Potential of the Antibacterial Compound in Dental Root Canals
Fabclavine-rich supernatant exhibited the highest inactivation efficacy of ≥3 log10 steps CFU reduction, followed by calcium hydroxide paste (≤2 log10 step) (Figure 5).
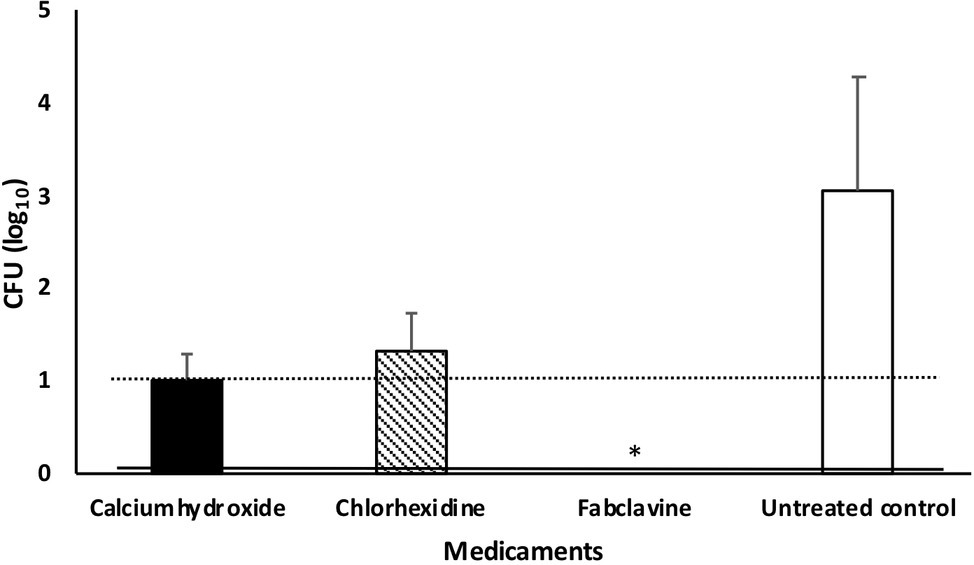
Figure 5. Median number of Enterococcus faecalis colony after treating dental root canals with different medicaments. Data were shown as CFU medians. Dotted and solid lines represent reductions of ≥2 and ≥ 3 log10 steps CFU, respectively. * indicates reduction below detection limit.
If we considered no tolerance to any bacterial growth in root canals (even one cell), the fabclavine-rich supernatant completely eradicated E. faecalis in 63.6% of the treated teeth. The mean percentage of E. faecalis-free dental root canals after treatment was 45.5% for Ca(OH)2 and 18.2% for CHX. The median number of colonies were 0, 10, and 20 for fabclavine-rich supernatant, Ca(OH)2, and CHX, respectively. However, number of colonies in the control group was 1,120 CFU (Figure 5).
Discussion
Our data showed that there were significant variations among the seven bacterial species in the production of effective antibacterial compounds against multidrug-resistant E. faecalis. Overall, the supernatant of X. cabanillasii exhibited the greatest inhibitory and bactericidal effect followed by supernatants of X. nematophila and X. szentirmaii. On the other hand, supernatants of X. bovienii and P. luminescens laumondii did not display any antibacterial efficacy against E. faecalis. Prior studies have reported variations among Xenorhabdus or Photorhabdus species (or strains) in the production of antimicrobial metabolites, and the efficacy of metabolites differed depending on the target organisms (Furgani et al., 2008; Fodor et al., 2010; Hazir et al., 2016). Furgani et al. (2008) reported that X. cabanillasii and X. szentirmaii produced larger diameter inhibitory zones than X. nematophila and X. bovienii against the primary mastitis pathogens Staphylococcus aureus, Klebsiella pneumoniae, and Escherichia coli when a cell-to-cell competition bioassay was conducted. In another study, X. szentirmaii produced a larger inhibitory zone (73.7 mm) than X. cabanillasii (60.7 mm) against Gram positive S. aureus (Fodor et al., 2010). However, in our overlay bioassay, cell-free supernatant of X. cabanillasii resulted in an inactivation zone of 50.4 mm, whereas X. szentirmaii exhibited an inactivation zone of 24.4 mm with E. faecalis. This discrepancy probably can be attributed to differences in the target organisms, or possibly strain differences in X. szentirmaii and X. cabanillasii used in the two different studies.
Previous studies demonstrated that Xenorhabdus and Photorhabdus secrete antimicrobial compounds (Furgani et al., 2008; Fodor et al., 2010; Hazir et al., 2016). Furgani et al. (2008) and Gualtieri et al. (2012) observed antibiotic activity when used not only as the purified antimicrobial compound, but also the cell-free supernatants of Xenorhabdus spp. Similarly, we observed antibacterial activity and determined MID and MBD values of Xenorhabdus and Photorhabdus in cell-free supernatants. The best MID and MBD values against E. faecalis were obtained from X. cabanillasii supernatant. Concentrations of 1 and 5% of X. cabanillasii supernatant were sufficient to inhibit bacterial growth (MID) and completely eliminate E. faecalis (MBD), respectively. Gualtieri et al. (2012) determined MBC values as the lowest concentration of antibiotic that resulted in 0.1% survival in the subculture. But we aimed for the complete inactivation of E. faecalis to prevent its re-colonization in the dental root canal. Therefore, our MBD values were designated according to full eradication. Our data clearly demonstrated that X. cabanillasii produce bactericidal molecule(s) rather than one with bacteriostatic effects, whereas other Xenorhabdus and Photorhabdus spp. tested in our study showed only a bacteriostatic effect.
To restrict the quantity of potential biosynthesis gene clusters responsible for the bactericidal compound, we compared the WT and Δpptase mutant of X. cabanillasii. The loss of bioactivity of the Δpptase mutant suggested the antibacterial compound against E. faecalis to be a natural product dependent on a PPTase as it is the case for typical NRPS- or PKS-derived natural products. Different biosynthetic gene clusters are known for X. cabanillasii, which could produce potential bioactive compounds like PAX-peptides, rhabdopeptides, or fabclavines (Tobias et al., 2017). The bioactivity of the mutant strain with a promoter exchange in front of the fabclavine-homologous gene cluster indeed confirmed that the bioactive compounds are fabclavines (Fuchs et al., 2014; Fodor et al., 2017). Biochemically, they are derived from a NRPS that produces a hexapeptide, which is elongated with one or two malonate units by a PKS and connected with an unusual polyamine (Fuchs et al., 2014; Wenski et al., 2019). Furthermore, our MALDI-MS and MS2 experiments confirmed that the fabclavines produced by X. cabanillasii are identical to the already described derivatives of X. budapestensis (Fuchs et al., 2014). It was reported that fabclavines Ia and Ib had a broad-spectrum bioactivity against different organisms such as Micrococcus luteus, Escherichia coli, Bacillus subtilis, Saccharomyces cerevisiae, Trypanosoma cruzi, T. brucei, and Plasmodium falciparum (Fuchs et al., 2014). Tobias et al. (2017) stated that except for Photorhabdus asymbiotica that might produce a shortened fabclavine derivative, other Photorhabdus species do not produce fabclavines, which were more widespread in Xenorhabdus strains. These data can explain why none of our tested Photorhabdus showed antibacterial efficacy against E. faecalis.
The success of endodontic treatment depends mainly on the complete inactivation of the infecting microorganisms from the root canal and prevention of reinfection. However, it is known that conventional root canal irrigants have limited action inside dentinal tubules beyond which viable bacteria are present (Gu et al., 2009). Our control data also showed that the irrigation of root canal with conventional irrigants (EDTA and NaOCl) was not enough to eradicate E. faecalis from dentinal tubules. Therefore, the use of intracanal medicaments between appointments is suggested for complete inactivation of bacteria before filling root canals (Bystrom et al., 1985; Pektas et al., 2013). Over the years, a number of synthetic antimicrobial agents have been employed as endodontic irrigants and medicaments against E. faecalis. Because of toxic and harmful side effects of common antibacterial agents and the increased antibiotic resistance to antimicrobial agents, a search for alternative agents that are non-toxic, affordable, and effective is needed.
Calcium hydroxide and CHX gel are known as the most effective intracanal medicaments and commonly used in dental practices (Haapasalo and Orstavik, 1987; Evans et al., 2003; Lakhani et al., 2017). However, they are not sufficient for the complete inactivation of E. faecalis from root canals in all cases (Siqueira, 2001; Evans et al., 2003; Mozayeni et al., 2014). The results of our study showed that 9 and 5 of 11 teeth treated with CHX and Ca(OH)2 were still contaminated with E. faecalis, respectively. Fabclavine-rich supernatant of X. cabanillasii exhibited more antimicrobial efficacy than CHX and Ca(OH)2 in root canals with complete inactivation from 7 of 11 teeth. Furthermore, Ca(OH)2 and CHX are formulated as paste and gluconate gel, respectively, to provide longer and better contact with microorganisms in root canals, whereas bacterial supernatant was in liquid form. This could be a possible reason why we did not obtain complete eradication in the root canals of all teeth (even though X. cabanillasii supernatant exhibited very strong bactericidal activity). We observed that some of the supernatant filled in root canal run off from the apex. Thus, it might be that the antibacterial compound does not reach E. faecalis hidden deep in the dentinal tubules. Accordingly, it will be useful to test the efficacy of formulated fabclavine-rich supernatant in future studies.
Fabclavines show very strong antimicrobial effects against both prokaryotic and eukaryotic pathogens and therefore they might also display adverse effects to host cells (Fuchs et al., 2014; Fodor et al., 2017; Tobias et al., 2017).
Our data clearly revealed that the fabclavine in the supernatant of X. cabanillasii has strong antibacterial activity against E. faecalis. Here we used fabclavine-rich supernatant as intracanal medicament for simplicity and it was highly effective against multidrug-resistant E. faecalis. Fabclavines might not display equivalent efficiencies against all bacterial species especially in primary endodontic infections which are polymicrobial. In this type of situation, they can be combined with other antibiotics which would lead to synergistic effects on pathogens while simultaneously reducing the potential adverse side effects (Fuchs et al., 2014).
We also compared the efficacy of cell-free supernatant of X. cabanillasii against multidrug-resistant V583 (ATCC 700802), antibiotic susceptible (ATCC 29212) and a clinic isolate (obtained from root canal of a patient) of E. faecalis using overlay bioassay method and there was no difference among the zone diameters (data were not shown in the manuscript). This indicates that the antibacterial mechanism of fabclavine derivatives has a different mode of action than commonly used traditional antibiotics. Based on the structural similarities to the (pre-)zeamines, we assume a similar mode of action (Masschelein et al., 2015a). Zeamines also show a broad-spectrum bioactivity against a wide variety of organisms, which is probably caused by a membrane disruptive mode of action (Masschelein et al., 2015b).
In conclusion, the data of the induced and non-induced fabclavine promoter exchange mutants clearly show that fabclavine derivatives are bioactive compounds responsible for the bactericidal effect. Although commonly used synthetic intracanal medicaments CHX gel and Ca(OH)2 paste do not eradicate infected root canals in all cases, they are commonly used as medicaments in root canals. Instead, purified and formulated fabclavine derivatives have a great potential to be used as intracanal medicament against dental root canal infections. Further studies with fabclavine-rich derivatives at different concentrations, different formulations and combine applications with other medicaments are needed to test against potential root canal pathogens in in vitro and in vivo bioassays. Of course one must determine the toxicity of fabclavines against eukaryotic cells at the applied concentration, since they also show some bioactivity against cell lines (Fuchs et al., 2014). However, the large number of fabclavine derivatives found in other Xenorhabdus strains might enable the identification of more specific derivatives with less toxicity.
Though Enterococcus faecalis is a commensal organism of humans, it is the third most common pathogen isolated from human bloodstream infections (Karchmer, 2000). It can also cause endocarditis; meningitis; and nosocomial, urinary tract, and wound infections (Kau et al., 2005). The efficacy of fabclavine derivatives obtained from X. cabanillasii can also be evaluated against these infections in the future.
Data Availability Statement
All datasets generated for this study are included in the article/Supplementary Material.
Ethics Statement
The studies involving human participants were reviewed and approved by Adnan Menderes University, Local Ethical Committee. The patients/participants provided their written informed consent to participate in this study.
Author Contributions
SH and HD designed the research. HD, HC, DU, and MT carried out the research, collected the data, and contributed to data analyses. HB and SW generated promoter exchanged mutant strains. NA and SY assisted with the experiments. SH and HB wrote the paper. All authors discussed the results and commented on the manuscript.
Funding
This work was financially supported by Aydin Adnan Menderes University (project number DHF-19009) and work in the Bode lab was supported by an ERC advanced grant under grant agreement no. 835108.
Conflict of Interest
The authors declare that the research was conducted in the absence of any commercial or financial relationships that could be construed as a potential conflict of interest.
Acknowledgments
We appreciate Dr. Harry K. Kaya from the University of California, Davis-USA, and Dr. David Shapiro-Ilan from USDA-ARS, SEA SE Fruit and Tree Nut Research Unit, Byron-USA, for editing the manuscript.
Supplementary Material
The Supplementary Material for this article can be found online at: https://www.frontiersin.org/articles/10.3389/fmicb.2019.02672/full#supplementary-material
References
Aarati, N., Ranganath, N. N., Soumya, G. B., Kishore, B., and Mithun, K. (2011). Evaluation of antibacterial and anticandidal efficacy of aqueous and alcoholic extract of neem (Azadirachta indica) an in vitro study. Int. J. Res. Ayurveda Pharm. 2, 230–235.
Alves, F. R., Neves, M. A., Silva, M. G., Roças, I. N., and Siqueira, J. F. Jr. (2013). Antibiofilm and antibacterial activities of farnesol and xylitol as potential endodontic irrigants. Braz. Dent. J. 24, 224–229. doi: 10.1590/0103-6440201302187
Ardizzoni, A., Blasi, E., Rimoldi, C., Giardino, L., Ambu, E., Righi, E., et al. (2009). An in vitro and ex vivo study on two antibiotic-based endodontic irrigants: a challenge to sodium hypochlorite. New Microbiol. 32, 57–66.
Arias, C. A., and Murray, B. E. (2012). The rise of the enterococcus: beyond vancomycin resistance. Nat. Rev. Microbiol. 10, 266–278. doi: 10.1038/nrmicro2761
Asnaashari, M., Ashraf, H., Rahmati, A., and Amini, N. (2017). A comparison between effect of photo-dynamic therapy by LED and calcium hydroxide therapy for root canal disinfection against Enterococcus faecalis: a randomized controlled trial. Photodiagn. Photodyn. Ther. 17, 226–232. doi: 10.1016/j.pdpdt.2016.12.009
Awori, R. M., Nganga, P. N., Nyongesa, L. N., Amugune, N. O., and Masiga, D. (2016). Mursamacin: a novel class of antibiotics from soil-dwelling roundworms of Central Kenya that inhibits methicillin-resistant Staphylococcus aureus. F1000Res 5, 2431–2439. doi: 10.12688/f1000research.9652.1
Bode, H. B. (2009). Entomopathogenic bacteria as a source of secondary metabolites. Curr. Opin. Chem. Biol. 13, 224–230. doi: 10.1016/j.cbpa.2009.02.037
Bode, E., Brachmann, A. O., Kegler, C., Simsek, R., Dauth, C., Zhou, Q., et al. (2015). Simple "on-demand" production of bioactive natural products. ChemBioChem 16, 1115–1119. doi: 10.1002/cbic.201500094
Boemare, N. (2002). “Biology, taxonomy and systematics of Photorhabdus and Xenorhabdus” in Entomopathogenic nematology. ed. R. Gaugler (UK: CABI Publishing), 35–56.
Boemare, N. E., and Akhurst, R. J. (2006). “The genera Photorhabdus and Xenorhabdus” in The prokaryotes. eds. M. Dworkin, S. Falkow, E. Rosenberg, K. H. Schleifer, and E. Stackebrandt (New York: Springer Science + Business Media Inc.), 451–494.
Boyce, J. M., and Pittet, D. (2002). Guideline for hand hygiene in health-care settings: recommendations of the healthcare infection control practices advisory committee and the HICPAC/SHEA/APIC/IDSA hand hygiene task force. Infect. Control Hosp. Epidemiol. 23, 3–40.
Brian, P. W., and Hemming, H. G. (1947). Production of antifungal and antibacterial substances by fungi; preliminary examination of 166 strains of fungi imperfecti. J. Gen. Microbiol. 1, 158–167. doi: 10.1099/00221287-1-2-158
Bystrom, A., Claesson, R., and Sundqvist, G. (1985). The antibacterial effect of camphorated paramonochlcorophenol, camphorated phenol and calcium hydroxide in the treatment of infected root canals. Endod. Dent. Traumatol. 1, 170–175. doi: 10.1111/j.1600-9657.1985.tb00652.x
Cowan, M. M. (1999). Plant products as antimicrobial agents. Clin. Microbiol. Rev. 12, 564–582. doi: 10.1128/CMR.12.4.564
Delgado, R. J., Gasparoto, T. H., Sipert, C. R., Pinheiro, C. R., Moraes, I. G., Garcia, R. B., et al. (2010). Antimicrobial effects of calcium hydroxide and chlorhexidine on Enterococcus faecalis. J. Endod. 36, 1389–1393. doi: 10.1016/j.joen.2010.04.013
Evans, M. D., Baumgartner, J. C., Khemaleelakul, S. U., and Xia, T. (2003). Efficacy of calcium hydroxide: chlorhexidine paste as an intracanal medication in bovine dentin. J. Endod. 29, 338–339. doi: 10.1097/00004770-200305000-00005
Evans, M., Davies, J. K., Sundqvist, G., and Figdor, D. (2002). Mechanisms involved in the resistance of Enterococcus faecalis to calcium hydroxide. Int. Endod. J. 35, 221–228. doi: 10.1046/j.1365-2591.2002.00504.x
Fodor, A., Fodor, A. M., Forst, S., Hogan, J. S., Klein, M. G., Lengley, K., et al. (2010). Comparative analysis of antibacterial activities of Xenorhabdus species on related and non-related bacteria in vivo. J. Microbiol. Antimicrob. 2, 36–46.
Fodor, A., Makrai, L., Fodor, L., Venekei, I., Husveth, F., Pal, L., et al. (2017). Anti-coccidiosis potential of autoclaveable antimicrobial peptides from Xenorhabdus budapestensis resistant to proteolytic (pepsin, trypsin) digestion based on in vitro studies. Microbiol. Res. J. Int. 22, 1–17. doi: 10.9734/MRJI/2017/38516
Fu, C., Donovan, W. P., Shikapwashya-Hasser, O., Ye, X., and Cole, R. H. (2014). Hot fusion: an efficient method to clone multiple DNA fragments as well as inverted repeats without ligase. PLoS One 9:e115318. doi: 10.1371/journal.pone.0115318
Fuchs, S. W., Grundmann, F., Kurz, M., Kaiser, M., and Bode, H. B. (2014). Fabclavines: bioactive peptide-polyketide-polyamino hybrids from Xenorhabdus. ChemBioChem 15, 512–516. doi: 10.1002/cbic.201300802
Fuhrman, J. A. (1999). Marine viruses and their biogeochemical and ecological effects. Nature 399, 541–548. doi: 10.1038/21119
Furgani, G., Böszörmenyi, E., Fodor, A., Mathe-Fodor, A., Forst, S., Hogan, J. s., et al. (2008). Xenorhabdus antibiotics: a comparative analysis and potential utility for controlling mastitis caused by bacteria. J. Appl. Microbiol. 104, 745–758. doi: 10.1111/j.1365-2672.2007.03613.x
Gillor, O., and Ghazaryan, L. (2007). Recent advances in bacteriocin application as antimicrobials. Recent Pat. Antiinfect. Drug Discov. 2, 115–122. doi: 10.2174/157489107780832613
Gilmore, M. S., Clewell, D. B., Courvalin, P., Dunny, G. M., Murray, B. E., and Rice, L. B. (2002). The enterococci: Pathogenesis, molecular biology, and antibiotic resistance. Washington DC: ASM Press, 439.
Gu, L. S., Kim, J. R., Ling, J., Choi, K. K., Pashley, D. H., and Tay, F. R. (2009). Review of contemporary irrigant agitation techniques and devices. J. Endod. 35, 791–804. doi: 10.1016/j.joen.2009.03.010
Gualtieri, M., Villain-Guillot, P., Givaudan, A., and Pages, S. (2012). Nemaucin, an antibiotic produced by entomopathogenic Xenorhabdus cabanillasii. Google Patents.
Haapasalo, M., and Orstavik, D. (1987). In vitro infection and disinfection of dentinal tubules. J. Dent. Res. 66, 1375–1379.
Hazir, S., Kaya, H. K., Stock, S. P., and Keskin, N. (2003). Entomopathogenic nematodes (Steinernematidae and Heterorhabditidae) for biocontrol of soil pests. Turk. J. Biol. 27, 181–202.
Hazir, S., Shapiro-Ilan, D. I., Bock, C. H., Hazir, C., Leite, L. G., and Hotchkiss, M. W. (2016). Relative potency of culture supernatants of Xenorhabdus and Photorhabdus spp. on growth of some fungal phytopathogens. Eur. J. Plant Pathol. 146, 369–381. doi: 10.1007/s10658-016-0923-9
Heling, M., Steinberg, D., Kenig, S., Gavrilovich, I., Sela, M. N., and Friedman, M. (1992). Efficacy of a sustained-release device containing chlorhexidine and Ca(OH)2 in preventing secondary infection of dentinal tubules. Int. Endod. J. 25, 20–24. doi: 10.1111/j.1365-2591.1992.tb00944.x
Karchmer, A. W. (2000). Nosocomial bloodstream infections: organisms, risk factors, and implications. Clin. Infect. Dis. 31, 139–143. doi: 10.1086/314078
Kau, A. L., Martin, S. M., Lyon, W., Hayes, E., Caparon, M. G., and Hultgren, S. J. (2005). Enterococcus faecalis tropism for the kidneys in the urinary tract of C57BL/6J mice. Infect. Immun. 73, 2461–2468. doi: 10.1128/IAI.73.4.2461-2468.2005
Kaya, H. K., and Stock, S. P. (1997). “Techniques in nematology” in Manual of techniques in insect pathology. ed. L. A. Lacey (San Diego: Academic Press), 281–324.
Kayaoglu, G., and Orstavik, D. (2004). Virulence factors of Enterococcus faecalis: relationship to endodontic disease. Crit. Rev. Oral Biol. Med. 15, 308–320. doi: 10.1177/154411130401500506
Kirkup, B. C. Jr. (2006). Bacteriocins as oral and gastrointestinal antibiotics: theoretical considerations, applied research, and practical applications. Curr. Med. Chem. 13, 3335–3350. doi: 10.2174/092986706778773068
Lakhani, A. A., Sekhar, K. S., Gupta, P., Tejolatha, B., Gupta, A., Kashyap, S., et al. (2017). Efficacy of triple antibiotic paste, moxifloxacin, calcium hydroxide and 2% chlorhexidine gel in elimination of E. faecalis: an in vitro study. J. Clin. Diagn. Res. 11, 6–9. doi: 10.7860/JCDR/2017/22394.9132
Leclerc, M. C., and Boemare, N. (1991). Plasmids and phase variations in Xenorhabdus spp. Appl. Environ. Microbiol. 57, 2597–2601.
Masschelein, J., Clauwers, C., Awodi, U. R., Stalmans, K., Vermaelen, W., Lescrinier, E., et al. (2015a). A combination of polyunsaturated fatty acid, nonribosomal peptide and polyketide biosynthetic machinery is used to assemble the zeamine antibiotics. Chem. Sci. 6, 923–929. doi: 10.1039/c4sc01927j
Masschelein, J., Clauwers, C., Stalmans, K., Nuyts, K., De Borggraeve, W., Briers, Y., et al. (2015b). The zeamine antibiotics affect the integrity of bacterial membranes. Appl. Environ. Microbiol. 81, 1139–1146. doi: 10.1128/AEM.03146-14
Mattigatti, S., Ratnakar, P., Moturi, S., varma, S., and Rairam, S. (2012). Antimicrobial effect of conventional root canal medicaments vs. propolis against Enterococcus faecalis, Staphylococcus aureus and Candida albicans. J. Contemp. Dent. Pract. 13, 305–309. doi: 10.5005/jp-journals-10024-1142
Mozayeni, M. A., Haeri, A., Dianat, O., and Jafari, A. R. (2014). Antimicrobial effects of four intracanal medicaments on Enterococcus faecalis: an in vitro study. Iran. Endod. J. 9, 195–198.
Newman, D. J., and Cragg, G. M. (2016). Natural products as sources of new drugs from 1981 to 2014. J. Nat. Prod. 79, 629–661. doi: 10.1021/acs.jnatprod.5b01055
Orstavik, D., and Haapasalo, M. (1990). Disinfection by endodontic irrigants and dressings of experimentally infected dentinal tubules. Endod. Dent. Traumatol. 6, 142–149. doi: 10.1111/j.1600-9657.1990.tb00409.x
Pektas, B. A., Yurdakul, P., Gülmez, D., and Görduysus, Ö. (2013). Antimicrobial effects of root canal medicaments against Enterococcus faecalis and Streptococcus mutans. Int. Endod. J. 46, 413–418. doi: 10.1111/iej.12004
Pladisai, P., Ampornaramveth, R. S., and Chivatxaranukul, P. (2016). Effectiveness of different disinfection protocols on the reduction of bacteria in Enterococcus faecalis biofilm in teeth with large root canals. J. Endod. 42, 460–464. doi: 10.1016/j.joen.2015.12.016
Sanchez-Sanhueza, G., González-Rocha, G., Dominguez, M., and Bello-Toledo, H. (2015). Enterococcus spp. isolated from root canals with persistent chronic apical periodontitis in a Chilean population. Braz. J. Oral. Sci. 14, 240–245. doi: 10.1590/1677-3225v14n3a13
Sasanakul, P., Ampornaramveth, R. S., and Chivatxaranukul, P. (2019). Influence of adjuncts to irrigation in the disinfection of large root canals. J. Endod. 45, 332–337. doi: 10.1016/j.joen.2018.11.015
Schmidt-Hieber, M., Blau, I. W., Schwartz, S., Uharek, L., Weist, K., Eckmanns, T., et al. (2007). Intensified strategies to control vancomycin-resistant enterococci in immunocompromised patients. Int. J. Hematol. 86, 158–162. doi: 10.1532/IJH97.E0632
Schneider, S. W. (1971). A comparison of canal preparations in straight and curved root canals. Oral Surg. Oral Med. Oral Pathol. 32, 271–275. doi: 10.1016/0030-4220(71)90230-1
Shi, Y. M., and Bode, H. B. (2018). Chemical language and warfare of bacterial natural products in bacteria–nematode–insect interactions. Nat. Prod. Rep. 35, 309–335. doi: 10.1039/C7NP00054E
Siqueira, J. F. Jr. (2001). Aetiology of root canal treatment failure: why well-treated teeth can fail. Int. Endod. J. 34, 1–10. doi: 10.1046/j.1365-2591.2001.00396.x
Siqueira, J. F. Jr., and de Uzeda, M. (1997). Intracanal medicaments: evaluation of the antibacterial effects of chlorhexidine, metronidazole, and calcium hydroxide associated with three vehicles. J. Endod. 23, 167–169. doi: 10.1016/S0099-2399(97)80268-3
Stojicic, S., Zivkovic, S., Qian, W., Zhang, H., and Haapasalo, M. (2010). Tissuse dissolution by sodium hypochlorite: effect of concentration, temperature, agitation and surfactant. J. Endod. 36, 1558–1562. doi: 10.1016/j.joen.2010.06.021
Stuart, C. H., Schwartz, S. A., Beeson, T. J., and Owatz, C. B. (2006). Enterococcus faecalis: its role in root canal treatment failure and current concepts in retreatment. J. Endod. 32, 93–98. doi: 10.1016/j.joen.2005.10.049
Suttle, C. A. (1994). The significance of viruses to mortality in aquatic microbial communities. Microb. Ecol. 28, 237–243. doi: 10.1007/BF00166813
Tay, F. R., Gu, L. S., Schoeffel, G. J., Wimmer, C., Susin, L., Zhang, K., et al. (2010). Effect of vapor lock on root canal debridement by using a side-vented needle for positive-pressure irrigant delivery. J. Endod. 36, 745–750. doi: 10.1016/j.joen.2009.11.022
Tendolkar, P. M., Baghdayan, A. S., and Shankar, N. (2003). Pathogenic Enterococci: new developments in the 21st century. Cell. Mol. Life Sci. 60, 2622–2636. doi: 10.1007/s00018-003-3138-0
Tervit, C., Paquette, L., Torneck, C. D., Basrani, B., and Friedman, S. (2009). Proportion of healed teeth with apical periodontitis medicated with two percent chlorhexidine gluconate liquid: a case-series study. J. Endod. 35, 1182–1185. doi: 10.1016/j.joen.2009.05.010
Tobias, N. J., Heinrich, A. K., Eresmann, H., Wright, P. R., Neubacher, N., and Backofen, R. (2017). Photorhabdus-nematode symbiosis is dependent on hfq-mediated regulation of secondary metabolites. Environ. Microbiol. 19, 119–129. doi: 10.1111/1462-2920.13502
Vianna, M. E., and Gomes, B. P. (2009). Efficacy of sodium hypochlorite combined with chlorhexidine against Enterococcus faecalis in vitro. Oral Surg. Oral Med. Oral Pathol. Oral Radiol Endod. 107, 585–589. doi: 10.1016/j.tripleo.2008.10.023
Webster, J. M., Chen, G., Hu, K., and Li, J. (2002). “Bacterial metabolites” in Entomopathogenic nematology. ed. R. Gaugler (London: CABI International), 9–114.
Wenski, S. L., Kolbert, D., Grammbitter, G. L. C., and Bode, H. B. (2019). Fabclavine biosynthesis in X. szentirmaii: shortened derivatives and characterization of the thioester reductase FclG and the condensation domain-like protein FclL. J. Ind. Microbiol. Biotechnol. 46, 565–572. doi: 10.1007/s10295-018-02124-8
Zan, R., Hubbezoglu, I., Ozdemir, A. K., Tunc, T., Sumer, Z., and Alici, O. (2013). Antibacterial effect of different concentration of boric acid against Enterococcus faecalis biofilms in root canal. Marmara Dent. J. 2, 76–80.
Keywords: endodontic infections, Enterococcus faecalis, fabclavine, Photorhabdus, Xenorhabdus
Citation: Donmez Ozkan H, Cimen H, Ulug D, Wenski S, Yigit Ozer S, Telli M, Aydin N, Bode HB and Hazir S (2019) Nematode-Associated Bacteria: Production of Antimicrobial Agent as a Presumptive Nominee for Curing Endodontic Infections Caused by Enterococcus faecalis. Front. Microbiol. 10:2672. doi: 10.3389/fmicb.2019.02672
Edited by:
Fabian Cieplik, University Medical Center Regensburg, GermanyReviewed by:
Daniel Manoil, Karolinska University Hospital, SwedenPrasanna Neelakantan, The University of Hong Kong, Hong Kong
Copyright © 2019 Donmez Ozkan, Cimen, Ulug, Wenski, Yigit Ozer, Telli, Aydin, Bode and Hazir. This is an open-access article distributed under the terms of the Creative Commons Attribution License (CC BY). The use, distribution or reproduction in other forums is permitted, provided the original author(s) and the copyright owner(s) are credited and that the original publication in this journal is cited, in accordance with accepted academic practice. No use, distribution or reproduction is permitted which does not comply with these terms.
*Correspondence: Hicran Donmez Ozkan, aGljcmFuLmRvbm1lekBhZHUuZWR1LnRy; Selcuk Hazir, c2VsY3VrLmhhemlyQGdtYWlsLmNvbQ==