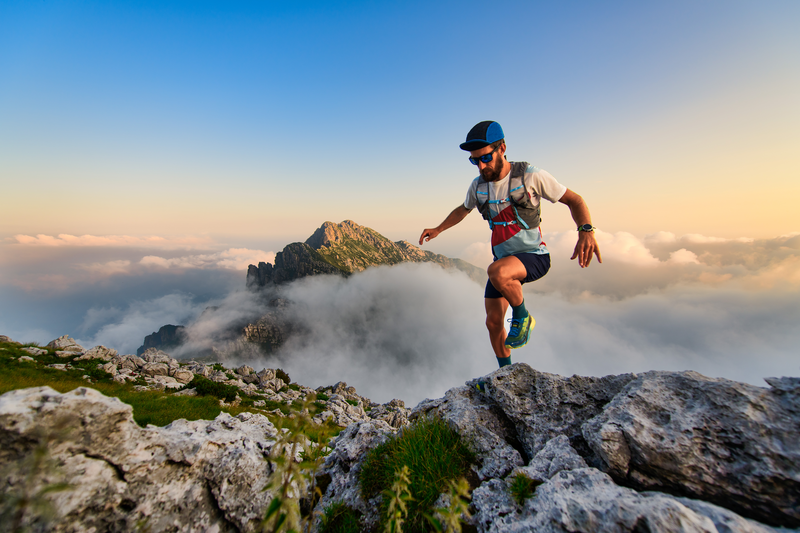
94% of researchers rate our articles as excellent or good
Learn more about the work of our research integrity team to safeguard the quality of each article we publish.
Find out more
ORIGINAL RESEARCH article
Front. Microbiol. , 24 October 2019
Sec. Extreme Microbiology
Volume 10 - 2019 | https://doi.org/10.3389/fmicb.2019.02432
Phosphate metabolism was studied to determine whether polyphosphate (polyP) pools play a role in the enhanced resistance against Cd2+ and metal-removal capacity of Cd2+-preadapted (CdPA) Methanosarcina acetivorans. Polyphosphate kinase (PPK), exopolyphosphatase (PPX) and phosphate transporter transcript levels and their activities increased in CdPA cells compared to control (Cnt) cells. K+ inhibited recombinant Ma-PPK and activated Ma-PPX, whereas divalent cations activated both enzymes. Metal-binding polyP and thiol-containing molecule contents, Cd2+-removal, and biofilm synthesis were significantly higher in CdPA cells >Cnt cells plus a single addition of Cd2+>Cnt cells. Also, CdPA cells showed a higher number of cadmium, sulfur, and phosphorus enriched-acidocalcisomes than control cells. Biochemical and physiological phenotype exhibited by CdPA cells returned to that of Cnt cells when cultured without Cd2+. Furthermore, no differences in the sequenced genomes upstream and downstream of the genes involved in Cd2+ resistance were found between CdPA and Cnt cells, suggesting phenotype loss rather than genome mutations induced by chronic Cd2+-exposure. Instead, a metabolic adaptation induced by Cd2+ stress was apparent. The dynamic ability of M. acetivorans to change its metabolism, depending on the environmental conditions, may be advantageous to remove cadmium in nature and biodigesters.
Inappropriate treatment of water polluted with heavy metals has become a public health problem around the world. Cadmium is found in soils, oceans, lakes, rivers, and other water bodies as well as in sewage sludge (Ahring and Westermann, 1985; Zheng-Bo et al., 2007; Altaş, 2009). Some plants and microorganisms have demonstrated a remarkable ability to remove Cd2+ from soils and water, and hence they may be considered realistic models for bioremediation (Leung et al., 2000; García-García et al., 2016; Moreno-Sánchez et al., 2017).
Organisms from the Archaea, known to inhabit extreme environments, have also been detected in environments with high heavy metal concentrations. The presence of methanogenic archaea in marine sediments polluted by Cd2+, Cu2+, Pb2+, and Hg2+ (Almeida et al., 2009) has led to the hypothesis that archaea have developed mechanisms to cope with heavy metal stress derived from geological events. These self-protective mechanisms now should enable them to counter the elevated anthropogenic-derived pollution. Transcriptomic and proteomic studies have described these mechanisms in archaea. However, biochemical characterization has yet to be elucidated (Mangold et al., 2013; Almárcegui et al., 2014; McCarthy et al., 2014).
Resistance mechanisms against heavy metals (Cu2+ and Cd2+) in archaea are similar to those found in eubacteria and eukaryotes. Thus far, they are extrusion by cation diffusion facilitators or heavy metal-specific ATP-dependent pumps; external binding by extracellular polymeric substances; biotransformation/inactivation by chemical reduction; and intracellular inactivation by chelating agents (Spada et al., 2002; Alvarez and Jerez, 2004; Baker-Austin et al., 2005; Harris et al., 2005; Remonsellez et al., 2006; Focardi et al., 2012; Mangold et al., 2013; Das et al., 2014; Singh et al., 2015).
Methanosarcina acetivorans C2A is a marine archaeon with the ability to accumulate Cd2+ by coupling it with sulfur-containing molecules (Lira-Silva et al., 2012). Cd2+ stress induces an increase of Cys, S2– and the methyl carrier coenzyme M intracellular contents (CoM-SH). However, thiol-containing molecules were not enough to bind and inactivate all Cd2+ (Lira-Silva et al., 2013). Moreover, M. acetivorans strain (CdPA) tolerates up to 2 mM total external Cd2+ (0.8 mM free Cd2+) after chronic pre-exposure to low (50 μM) Cd2+(Lira-Silva et al., 2013). Other CdPA cell features might contribute to enhanced heavy metal resistance/accumulation. Higher capacity of biofilm formation may trap a high percentage of Cd2+ outside the cells. Their increased levels of total orthophosphate (Pi), PPi and polyphosphate (polyP) may trap more Cd2+ inside the cells (Jasso-Chávez et al., 2015). Indeed, it has been described that polyP increases under stress conditions in Escherichia coli and M. acetivorans (Keasling and Hupf, 1996; Jasso-Chávez et al., 2015). Methanogens have also shown ability to contend against other heavy metals like uranium (Holmes et al., 2018) and mercury (Gilmour et al., 2018). Therefore, methanogens emerge as a suitable model that may help to understand the interactions between archaea and the increasing presence of heavy metal in their ecological niches.
To unveil whether chronic exposure to Cd2+ may induce permanent or transitory changes in polyP metabolism, in the present work the genome of CdPA and their control cells were sequenced and compared with the previously reported genome (Galagan et al., 2002) which was taken as the reference (NCBI ID: 1072). Also, the transcript contents of the ppk, ppx, and pstA genes, PPK and PPX activities, and thiol- and poly phosphate-molecule levels were analyzed. Kinetic characterization of the PPK and PPX recombinant enzymes was also carried out. Data suggested that the chronic Cd2+ stress did not induce mutations in the genes involved in metal resistance; instead, fast and transitory metabolic shifts were in polyP, thiol-molecule synthesis and the capacity to synthesize biofilm. This metabolic strategy seemed plentiful to manage Cd2+ toxicity.
ATP and phosphoenolpyruvate were from Roche (Germany). L-cysteine, NADH, PK/LDH mix and toluidine blue were purchased from Sigma (United States). Trimethylsilyl polyphosphate was from Sigma-Aldrich (Switzerland). Standard solutions of CdCl2 and monobasic potassium phosphate (KH2PO2) were from Sigma-Aldrich (Germany). HEPES was acquired from Research Organics (United States). Absolute methanol, sodium acetate, Na2S⋅9 H2O, acetic acid and CdCl2⋅2.5 H2O, were of analytical grade.
Methanosarcina acetivorans C2A was cultured in absence (control cells) or presence of 50 μM CdCl2, under anoxic conditions in high salt (HS) medium supplemented with 100 mM methanol or acetate as carbon source (Sowers et al., 1993). Cd2+-preadapted cells (CdPA) were generated by culturing C2A cells in media containing 50 μM CdCl2 and sub-culturing them in fresh 50 μM CdCl2 containing medium every 2 weeks for more than 1 year as previously reported (Lira-Silva et al., 2013). Cultures were carried out in 100 mL anaerobic bottles by adding 0.5–0.7 mg cell protein into 50 mL of growth medium, sealed with rubber septa and aluminum crimps. Cell growth was determined by their methane production and protein content. Optical density was not considered as a reliable cell growth parameter, since Cd2+ induced biofilm formation also brought about significant turbidity. To determine whether chronic Cd2+ stress also conferred resistance toward other heavy metals, Cd2+ was replaced by CuSO4 or ZnCl2 in the culture medium.
Determination of methane, thiol-containing molecules (Cys, CoM-SH) and sulfide were carried out by gas chromatography, HPLC and spectrophotometry, respectively (Lira-Silva et al., 2013). For extracellular orthophosphate (Pi) quantitation, aliquots of cell-free growth medium withdrawn at the indicated times were assayed by molybdenum blue generation at 870 nm (LeBel et al., 1978). Intracellular polyP content was assessed in cell suspensions previously washed and mixed with 3% (v/v) ice-cold perchloric acid (PCA) as described elsewhere (Jasso-Chávez et al., 2015).
PolyP was co-isolated with DNA as reported (Jasso-Chávez et al., 2017). Thereafter, samples were treated with 1 U of DNAse for 2 h at 37°C and then precipitated with ice-cold absolute ethanol for 1 h at –20°C. The pellet (polyP) was resuspended in 50 mM NaOH + 1 mM EDTA. PolyP samples were mixed with toluidine blue solution and the absorbance measured at 530/630 nm as previously described. PolyP was also identified by 14% urea-PAGE and stained with 0.05% toluidine blue in 25% methanol and 5% glycerol (Gomez Garcia, 2014). Cellular protein precipitated with 10% (w/v) trichloroacetic acid (TCA) overnight, was determined by Lowry method.
Methods regarding the analyses of cell ultrastructure, heavy metal contents and biofilm are described in the Supplementary Text S1.
Changes in transcript levels were determined by semiquantitative reverse-transcriptase PCR reaction following real time PCR (see Supplementary Table S4 for primers sequences used). mRNA content changes were determined by the 2–ΔΔCt method in CdPA cells or acetate-grown control cells (without Cd2+; Cnt), or control cells with a single 50 μM Cd2+ exposure (Cnt + Cd), or CdPA cells further cultured without Cd2+ for one pass (3–4 generations; CdPA-Cd cells). gapd but not MA_3998 gene was used as a house-keeping reference gene (Livak and Schmittgen, 2001; Rohlin and Gunsalus, 2010; Santiago-Martínez et al., 2016; also see Supplementary Text S1 and Supplementary Table S5). Detailed methodology regarding the cloning and heterologous overexpression of recombinant PPX and PPK is described in Supplementary Text S1 (Saavedra et al., 2005; Studier, 2005).
Cytosol-enriched fractions were used to measure enzyme activities and were obtained from cells harvested after 7 or 14 days of growth as described elsewhere (Santiago-Martínez et al., 2016). PPK and PPX activities were determined in the absence and presence of K+ using commercial trimethylsilyl polyP. The synthesis or degradation of polyP was determined by mixing the reaction with a dying solution (0.001% toluidine blue in 40 mM acetic acid) and measuring absorbance at 530 and 630 nm (Skorko, 1989; Mullan et al., 2002). The reaction was started by adding the recombinant protein (1.7–4.6 μg protein) or cytosol-enriched fraction (50 μg protein). The reaction mixture containing variable Mg-ATP (PPK) or polyP (PPX) was incubated for 15 or 60 min for PPK or PPX, respectively, at 37°C under 150 rpm orbital agitation. Enzymatic activities were also determined with endogenous polyP-enriched-fractions after incubating for 60 min. See Supplementary Text S1 for details.
Genomic DNA extraction from Cnt- and CdPA-M. acetivorans was performed as reported elsewhere (Jasso-Chávez et al., 2017). These strains were sequenced using the Illumina Genome Analyzer IIx platform at the USSDNA (IBT, UNAM) with paired end libraries and 72 base pair reads. FastQC version 0.10.1 and Trimmomatic version 0.32 were used for adaptor sequence removal and quality trimming (Bolger et al., 2014). De novo assembly was carried out using ABySS version 1.3.7 (Simpson et al., 2009). For k-mer abundance analysis k-mer version 0.4 was used with a k-mer size of 64. Genome annotation was accomplished using Rapid Annotation using Subsystem Technology (RAST) (Aziz et al., 2008). A comparative analysis was carried out with BLASTN version 2.7.1, using all 4567 coding genes from the reference genome and the strain genomic assemblies. From these datasets, 52 genes associated with putative heavy metal resistance mechanisms were selected for further assessment.
To assess how the CdPA phenotype develops, an array of biochemical/physiological parameters were compared with control cells (without Cd2+; Cnt) and control cells with a single 50 μM Cd2+ exposure (Cnt + Cd). To explore phenotype reversibility, CdPA cells were further cultured without Cd2+ for one time (3–4 generations; CdPA-Cd).
Methanogenesis rates, at the exponential phase of growth (up to day 5), were higher in acetate-grown cultures of CdPA and CdPA-Cd vs. Cnt cells, whereas the protein contents were higher in CdPA and Cnt + Cd cells (Figure 1). ATP contents ranged 3.7–4.7 nmol (mg protein)–1 among the different cell culture conditions. ATP/ADP ratios were 1.2 for Cnt and Cnt + Cd cells and 1.6 for CdPA and CdPA-Cd cells. These data suggested that Cd2+ chronic exposure did not affect energy metabolism. In methanol-grown cells, no differences in methane production or cellular protein content were found among the four different cells used in this study (data not shown). Since no differences in phenotype developed in presence of methanol, and acetate is M. acetivorans “natural” substrate, no further experimentation was carried out in methanol-grown cells.
Figure 1. Growth of Methanosarcina acetivorans cultured with acetate. Methane production (A) and protein synthesis (B) were determined in control cell cultures (□); control cells exposed for first time to Cd2+ (■); cultures with CdPA cells () and CdPA cells cultures where Cd2+ was omitted for first time (
). Rates of methane production determined by considering the linear part of the plot (up to day 5) in mmole x day–1 were: Cnt cells: 0.15 ± 0.03; Cnt + Cd: 0.13 ± 0.02; CdPA: 0.25 ± 0.05 and CdPA-Cd: 0.29 ± 0.06. Values shown are the mean ± SD of 3 independent experiments. a P < 0.05 in methane synthesis at day 5 between CdPA vs. Cnt and Cnt + Cd; as well as in protein content at day 5 and 7 between CdPA vs. Cnt and CdPA-Cd and at day 10 vs. cnt, cnt + Cd and CdPA-Cd.
Chronic Cd2+ exposure in acetate-grown CdPA cells increased both the content of thiol-containing metabolites (1.25 vs. 0.48 μmoles thiols in Cnt + Cd cells; n = 2) and the Cd2+ removal capacity (2.56 ± 0.86 vs. 1.38 ± 0.25 μmoles Cd2+ in Cnt + Cd cells; n = 3; X ± SD; P < 0.005). Thiol/Cd2+ ratios were 0.49 and 0.35 for CdPA and Cnt + Cd, respectively. Acetate-grown CdPA-Cd cells still contained intracellular Cd2+ after one culture in the absence of metal (0.78 ± 0.01 μmoles Cd2+; X ± SD; n = 3). Thiol-metabolites content (0.20 μmoles thiols; n = 2) and thiol/Cd2+ ratio (0.25) were lower in CdPA-Cd cells than in Cnt + Cd cells.
Low thiol/Cd2+ ratios in CdPA cells suggested that other chelating molecules, such as polyP, could be involved in binding the accumulated metal ions. It has been reported that PPX and Pi transporters may be involved in Cu2+ resistance in the archaeon Metallosphaera sedula (Rivero et al., 2018). These observations led us to survey genes coding for proteins involved in polyP metabolism (ppx, ppk and phosphate transporters). Methanogens and other archaea genomes available in the KEGG data base were inspected1.
Polyphosphate kinase or polyP/ATP NAD+ kinase, and PPX or Ppx/GppA phosphatase or NAD+-binding component fused to domain related to PPX genes were found in Methanosarcina sp., other methanogens and other archaea. Some exceptions are Methanohalobium and Methanosalsum (Methanosarcinales) and Thermoplasma (Thermoplasmata). Regarding the phosphate transporter, most of the genomes analyzed showed genes encoding the pst (phosphate specific transport) operon, the ABC type phosphate transporter or both (Supplementary Table S1). The widespread localization of these genes among Archaea suggested an essential role for polyP in cell homeostasis, and potentially as mechanism of resistance by binding and accumulating heavy metals. Therefore, the biochemical study of polyP metabolism appears crucial to understand its physiological role in enhanced heavy metal accumulation phenotypes.
The synthesis of polyP, driven by active Pi uptake, was stimulated by Cd2+ at the exponential growth phase (Figure 2A). Extracellular Pi was in excess, since approximately 1.6 mM Pi remained in the medium at the end of the growth curve (Figure 2B). The high polyP/Cdremoval ratios (>2; Table 1), plus the thiol-molecule content, sufficed to completely inactivate intracellular accumulated Cd2+. Indeed, elemental analysis of the acetate-grown CdPA cells by high-angle annular dark-field imaging scanning transmission electron microscope (HAADFSTEM) showed numerous electrodense granules (acidocalcisomes; Supplementary Figure S1A), in which Cd, P, S, and Ca were all detected (Supplementary Figures S1B,C). On the contrary, acidocalcisomes were scarce in Cnt cells (Supplementary Figure S1D).
Figure 2. Phosphate metabolism in Methanosarcina acetivorans cultured in acetate. PolyP synthesis (A) and phosphate uptake (B) were determined in Cnt (□), Cnt + Cd (■), CdPA () and CdPA-Cd (
) cells. Values shown are the mean ± SD of 3 independent experiments. PolyP synthesis: aP < 0.01 and bP < 0.05 CdPA vs. Cnt and Cnt + Cd; cP < 0.01 CdPA vs. Cnt, Cnt + Cd and CdPA-Cd. Pi uptake: aP < 0.05; bP < 0.01 CdPA vs. Cnt and Cnt + Cd.
When cells were cultured under limiting extracellular 0.05 mM Pi, low polyP and higher content of thiol molecules in all growth conditions were detected (Supplementary Figure S2). The cell Cd2+ removal capacity could not be reliably determined due to an extensive cell agglomeration, although no Cd2+ was detected in the supernatant (data not shown).
To unveil whether the synthesis/degradation of polyP is modified by Cd2+ in M. acetivorans, transcript levels or/and activities of the Pi transporter subunit A (pstA), PPK (ppk) and PPX (ppx) were determined. Transcript levels of the ppk gene did not vary among the different cell types at day 7 of culture but increased at day 14. In turn, ppx gene transcript levels increased in Cnt + Cd, CdPA and CdPA-Cd cells, with respect to Cnt cells, after 7 or 14 days of culture (Figure 3). CdPA and Cnt + Cd transcript contents of the pstA gene increased at both days 7 and 14 but decreased by 50% in the CdPA-Cd cells at day 7 (Figure 3). PPK and PPX activities in CdPA cells, at day 7, increased as compared to Cnt cells (Table 2). At day 14 of growth, PPK activity was negligible in all cell types whereas PPX activities increased, but only significantly in CdPA-Cd cells (Table 2).
Figure 3. Relative transcript contents of the ppx and ppk genes in Methanosarcina acetivorans. Transcript contents of the ppk (white bars), ppx (light gray bars) and pstA (gray bars) genes were determined in Cnt + Cd, CdPA and CdP-Cd cells and compared to control cells (Cnt), after 7 (A) and 14 (B) days of culture. Data shown are the mean ± SD of three independent experiments carried out by triplicate. aP < 0.05, bP < 0.01, cP < 0.001 vs. Cnt cells, dP < 0.01 vs. Cnt + Cd and CdPA, eP < 0.05 vs. CdPA-Cd.
Heterologous transcription of the ppk and ppx genes resulted in 2187 and 1632 bp products, respectively, which were the expected sizes according to the NCBI-Gene (ID 1471973 for ppk and ID 1471975 for ppx) loci (Supplementary Figure S3A). In turn, cloning, heterologous overexpression and purification resulted in 81 and 61 kDa proteins for the recombinant Ma-PPK and Ma-PPX, respectively (Supplementary Figure S3B). Both enzymes showed activity using commercial trimethylsilyl polyP as polyP source in the presence of 120 mM K+, the physiological intracellular concentration in M. acetivorans (Santiago-Martínez et al., 2016). Both enzymes displayed Michaelis-Menten type kinetics (Supplementary Figures S4A–C); 120 mM K+ decreased the Vmax value of Ma-PPK and increased that of Ma-PPX, whereas their Km values remained unchanged (Supplementary Table S2). Also, K+ induced a change in Ma-PPX kinetics, from hyperbolic (Michaelis-Menten) to sigmoidal (Hill) behavior (Supplementary Figure S4D) with a Hill value ≥2 (Supplementary Figure S4D, insert). To determine the specific regulating effect of K+, other monovalent cations were also tested; 120 mM K+ or Li+, 50 mM Na+ and 10 mM NH4+ or Rb+, indeed inhibited the PPK activity by more than 90% (n = 2). In turn, PPX activity increased by 2.5 times with 120 mM K+, Li+ or Na+ with respect to activity in absence of monovalent cations (n = 2).
Polyphosphate kinase was activated by 10 and 25 μM of Cd2+, Cu2+or Zn2+, whereas 50 μM did not bring about significant activity changes (Figure 4A). In turn, PPX was also 2.5–3 times activated by 10–50 μM of Cd2+, Cu2+ and Zn2+ (Figure 4B). The absence of K+ (a non-physiological condition) decreased its activity (Figures 4C,D). Interestingly, CdPA cells were more resistant to 100 μM Zn2+ or Cu2+ stress than Cnt cells (Figure 5), suggesting that enhanced polyP metabolism also served for other divalent cations inactivation.
Figure 4. Effect of heavy metal on the activity of recombinant Ma-PPK and Ma-PPX Activities of PPK and PPX in presence (A,B) or absence of 120 mM K+ (C,D), respectively, were determined in absence (white bar) or presence of 10 (light gray bar), 25 (dark gray bar) or 50 μM (yellow bar) of the indicated heavy metal. The concentration of substrates was for PPK (A,C) 2.5 mM ATP and for PPX (B,D) 5 mg Trimethylsilyl polyP/mL, respectively. Student t-test for non-paired samples, aP < 0.01; bP < 0.025; cP < 0.05 vs. Cnt without heavy metal; dP < 0.01 vs. 10 and 25 μM Cd2+; eP < 0.05 vs. 25 μM Cu2+; fP < 0.05 vs. 25 μM Zn2+; gP < 0.025 vs. 50 μM Zn2+.
Figure 5. Growth of Methanosarcina acetivorans cultured with Cu2+ or Zn2+. Methane production was determined in control cell cultures in absence (□) or in presence of Cu2+ (◦) or Zn2+ (△) as well as in CdPA cells in absence (■) or in presence of Cu2+ (•) or Zn2+ (▲). Concentration was 100 μM for Cu2+ and Zn2+. It is noted that when CdPA cells were cultured in presence of Cu2+ or Zn2+, the 50 μM Cd2+ was not added for first time. Values shown are the mean ± SD of 3 independent experiments. aP < 0.01 CdPA vs. control; bP < 0.01 CdPA vs. control (in presence of Cu2+).
Recombinant enzyme activities were also determined with an enriched-fraction of native polyP isolated from M. acetivorans. Ma-PPK showed detectable activity using 30 μg native polyP/mL as substrate, with a Vmax of 0.06 ± 0.02 μmol polyPsynthesized (min × mg prot)–1, (X ± SD; n = 5), whereas Ma-PPX required 0.3–1.2 mg native polyP/mL, showing a Vmax of 0.3 ± 0.2 μmol polyPhydrolyzed (min × mg prot)–1 (n = 4).
Acetate-grown Cnt cells were able to synthesize biofilm, but a single exposure to >250 μM Cd2+ was toxic and diminished by 75% their biofilm synthesis capacity. In contrast, the CdPA cells synthesized eight times more biofilm with respect to Cnt cells after 14 days (Supplementary Figure S5).
The genomes of M. acetivorans Cnt and CdPA cells were successfully assembled into high-quality drafts. The raw data comprised a total of 51,501,938 and 50,029,628 reads that were assembled into 382 and 348 contigs with N50 of 28,555 and 32,300 for Cnt and CdPA cells, respectively. This complete genome project has been deposited in GenBank and the data are available through BioProject accession number PRJNA477340 and biosample accession numbers are SAMN09467189 and SAMN09467190. Quality of the genome assembly was assessed with QUAST (Gurevich et al., 2013). Total length of the contigs were ordered and compared with M. acetivorans C2A (NC_003552.1, May 2017) reference genome (Galagan et al., 2002) using Mauve version 2.0 (Rissman et al., 2009); they were very similar, with no gaps or inversions observed. Genome sequence and annotation results showed that both genomes were identical, with respect to the number of the genes. Further genome analysis was carried out to identify genes participating in thiol-metabolites or polyP synthesis, antioxidant defense and gluconeogenic metabolism. This was an initial attempt to find the source of phenotypic differences between CdPA and Cnt strains. From the 51 analyzed genes, and in agreement with the whole sequence genome, no differences were found in CdPA cells compared to the reference genome. An unexpected finding was that 11 genes in the CdPA genome, encoding proteins with no putative roles assigned yet, showed changes in identity, length and E-value with respect to the M. acetivorans genome used as a reference (Supplementary Table S3).
In a wide variety of organisms such as the protist Euglena gracilis (Avilés et al., 2003), algae, plants (Küpper et al., 2002; Ranjard et al., 2008; Meyer et al., 2016) and M. acetivorans (Jasso-Chávez et al., 2015), the pre-adaptation to low doses of stressors such as heavy metals or O2 triggers the development of resistance mechanisms toward higher stressor concentrations. In the present work, it was shown that under chronic Cd2+ exposure increased rates of methane synthesis were attained, in agreement with previous reports where acetate-grown cells but not methanol-grown cells increase their methane production rates in the presence of Cd2+ (Lira-Silva et al., 2012, 2013). In addition, the protein content was higher in CdPA and Cnt + Cd cells with respect to Cnt cells. This suggested that indeed Cd2+ may activate transcription factors that promotes cell duplication.
Genes encoding proteins involved in polyP metabolism are widespread in Archaea (Supplementary Table S1). It has been suggested that under nutritional stress, polyP provides Pi for ATP production and metabolism, thus preserving cell viability (Brown and Kornberg, 2008). Furthermore, it has also been proposed that in the archaea Pyrococcus horikoshii, M. acetivorans, Archaeoglobus sp. and Metallosphaera sedula as well as in the proteobacteria Acidithiobacillus ferrooxidans, enhanced polyP and thiol-metabolite syntheses are two of the main mechanisms used to deal with the toxicity of Cu2+ and Cd2+ (Alvarez and Jerez, 2004; Lira-Silva et al., 2013; Toso et al., 2016; Rivero et al., 2018). In the present work, it was determined that CdPA cells cultured once without Cd2+ still contained intracellular Cd2+, probably because the metal ion was tightly bound by thiol-containing molecules (Lira-Silva et al., 2012) and polyP.
It was previously determined that M. acetivorans cells contained polyP granules (acidocalcisomes) even when cells were grown without Cd2+ and phosphate (Lira-Silva et al., 2012). The present study demonstrated that polyP granules increased under persistent Cd2+ exposure. These data suggested that polyP is not only synthesized for Pi storage, but also as a protective mechanism to bind and neutralize divalent cations at toxic concentrations.
Noteworthy, the polyP contents were much higher than those of the intracellular thiol-containing molecules at 5 mM external Pi. On the contrary, when phosphate was limiting (0.05 mM), thiol-containing molecules prevailed over polyP synthesis. These data suggested that the mechanisms raised by M. acetivorans against Cd2+ depend on external sources of sulfur and phosphorus.
The roughly constant pstA transcript level, except for CdPA-Cd cells at day 7 of growth, correlated with an active external Pi uptake. In addition, an increased Pi transport into cells also correlated with an active polyP synthesis in the presence of Cd2+. In M. acetivorans the ppk and ppx genes are in the same operon, as in E. coli (Akiyama et al., 1993). Cd2+ activated the transcription of both, ppk and ppx genes in Cnt + Cd and CdPA cells. However, no correlation was found between transcript contents and enzymatic activities, i.e., PPX activity at day 7 and PPX activity at day 14 were low or negligible, whereas their transcript levels were significant. These observations suggested that, besides transcriptional regulation, PPK and PPX may be also modulated at the post-translational (covalent regulation) and metabolic levels (inhibition by products) in M acetivorans. In turn, the higher PPX transcript level and enzyme activity in CdPA-Cd cells, with respect to control cells, was probably due to the presence of remaining internal Cd2+
It is well known that many enzymes are regulated by metal ion binding into the catalytic site, and they become efficient and selective promoters of catalysis and/or stability (Gohara and Di Cera, 2016). In the present work, Zn2+, Cd2+, and Cu2+, as well as K+ displayed a regulatory role of Ma-PPK and Ma-PPX activities. It has been suggested that K+ may serve to position and activate the phosphoryl transfer process (Jurica et al., 1998). Similarly, PPX from Chlorobium tepidum and E. coli are activated by 20 and 175 mM K+, respectively (Proudfoot et al., 2004; Albi and Serrano, 2014). In turn, PPK from Sulfolobus acidocaldarius is activated by 2 mM K+ or Mg2+ (Skórko et al., 1989); no inhibitory effect of K+ on PPK has been reported.
Heavy metal cations stimulated both PPK and PPX activities in M. acetivorans. Similarly, PPX from S. cerevisiae and Corynebacterium glutamicum are activated by 0.05 Co2+ and 2 mM Zn2+, respectively (Andreeva et al., 2004; Lindner et al., 2007). When comparing PPX with the superfamily of metal-dependent phosphohydrolases (Aravind and Koonin, 1998), BLASTp analysis identified a “HD” (His-Asp) motif in PPX at amino acids 345–468 (Aravind and Koonin, 1998). Therefore, this region may be involved in the divalent cation activation of Ma-PPX. In contrast, some heavy metals are inhibitors of E. coli and C. glutamicum PPK (Ahn and Kornberg, 1990; Lindner et al., 2009). These differences in the effect of divalent cations are consistent with a low amino acid identity among different species. Alignment of the M. acetivorans PPK (gene ID 1471973) with protein sequences of other microorganisms revealed 35–44% identity with high query cover of 94% (Supplementary Figure S6A). In turn, alignment of the M. acetivorans PPX (gene ID 1471975) showed 26–33% identity and wide range 54–94% query cover (Supplementary Figure S6B).
Physiological (K+, Na+, and NH4+) and non- physiological (Li+ and Rb+) monovalent cations affected the activity of both Ma-PPK and Ma-PPX, suggesting a specific binding site for K+/Na+. Whether the binding sites of divalent cations are different from those for K+, or Cd2+ modifies the intracellular concentration of K+ to further regulate PPK and or/PPK remains to be determined.
Ma-PPK showed a KmATP = 1.4 mM whereas the intracellular ATP concentration ranged 5–6.5 mM (3.5–5 times the Km value), suggesting that in M. acetivorans this enzyme does not display its maximal catalytic potential; in contrast, the high intracellular polyP levels (Figure 2A) and KmpolyP = 1.2 mg/mL indicated that the Ma-PPX substrate was saturating. Moreover, Ma-PPK showed a lower catalytic efficiency with respect to Ma-PPX (i.e., Vmax/Km of 0.7 vs. 3, respectively; see Supplementary Table S2). Thus, these data suggested that Ma-PPK may have a higher controlling role on polyP homeostasis because it is slower and less efficient than Ma-PPX.
The CdPA phenotype was reverted once Cd2+ was omitted from the culture media. As cell biomass (see Figure 1) and levels of Cd2+-binding molecules (Figures 2A and Supplementary Figure S3) were lower in CdPA-Cd cells than in CdPA cells, it is suggested that these cell functions and in general the CdPA phenotype are regulated by the presence of the metal and not determined by point mutations, at least in genes coding for proteins involved in the metabolism of carbohydrates, sulfur or phosphate. Further cultivation of CdPA cells with 100 μM Cu2+ or Zn2+ produced more biomass than that determined for Cnt cells; this observation deserves further analysis.
Persistent exposure to Cd2+ activated polyP metabolism. The mechanisms here described may provide insights on how phosphate metabolism in M. acetivorans and other archaea is regulated and therefore, the present study proposes the use of these organisms as a potential tool for the removal of metals and phosphate in polluted waters with the associated benefit of high biogas yields.
The datasets generated for this study can be found in the complete genome project has been deposited in GenBank, and the data are available through BioProject accession number PRJNA477340 and biosamples accession numbers are SAMN09467189 and SAMN09467190.
RJ-C, EL-S, and RM-S: conceptualization and methodology. RJ-C and RM-S: resources. VL-S, DM-M, and FP-V: data curation. EL-S, KG-S, AV-S, and AZ-R: investigation. R-JC, EM, and RM-S: formal analysis. RJ-C: original draft. RJ-C, MT-M, and RM-S: writing-review and editing.
This work was supported by grants # 156969 (RJ-C), and 239930 and 281428 (RM-S) from the CONACyT, Mexico.
VL-S and DM-M were employed by company Winter Genomics.
The remaining authors declare that the research was conducted in the absence of any commercial or financial relationships that could be construed as a potential conflict of interest.
We thank Dr. Ricardo Grande, from USSDNA (IBT, UNAM), for genome sequencing; Patricia Bizarro-Nevares and Francisco Pasos-Nájera (Laboratory of Electron Microscopy, Faculty of Medicine, UNAM) for sample preparation for the ultrastructure analysis; and physicist Roberto Hernandez (Institute of Physics, UNAM) for the HAADF-STEM ultrastructure analysis.
The Supplementary Material for this article can be found online at: https://www.frontiersin.org/articles/10.3389/fmicb.2019.02432/full#supplementary-material
Ahn, K., and Kornberg, A. (1990). Polyphosphate kinase from Escherichia coli. Purification and demonstration of a phosphoenzyme intermediate. J. Biol. Chem. 265, 11734–11739.
Ahring, B. K., and Westermann, P. (1985). Sensitivity of thermophilic methanogenic bacteria to heavy metals. Curr. Microbiol. 12, 273–276. doi: 10.1007/BF01567977
Akiyama, M., Crooke, E., and Kornberg, A. (1993). An exopolyphosphatase of Escherichia coli. The enzyme and its ppx gene in a polyphosphate operon. J. Biol. Chem. 268, 633–639.
Albi, T., and Serrano, A. (2014). Two exopolyphosphatases with distinct molecular architectures and substrate specificities from the thermophilic green-sulfur bacterium Chlorobium tepidum TLS. Microbiology 160, 2067–2078. doi: 10.1099/mic.0.080952-80950
Almárcegui, R. J., Navarro, C. A., Paradela, A., Albar, J. P., Von Bernath, D., and Jerez, C. A. (2014). New copper resistance determinants in the extremophile Acidithiobacillus ferrooxidans: a quantitative proteomic analysis. J. Proteome Res. 13, 946–960. doi: 10.1021/pr4009833
Almeida, W. I., Vieira, R. P., Cardoso, A. M., Silveira, C. B., Costa, R. G., González, A. M., et al. (2009). Archaeal and bacterial communities of heavy metal contaminated acidic waters from zinc mine residues in Sepetiba Bay. Extremophiles 13, 263–271. doi: 10.1007/s00792-008-021-212
Altaş, L. (2009). Inhibitory effect of heavy metals on methane-producing anaerobic granular sludge. J. Hazard. Mater. 162, 1551–1556. doi: 10.1016/j.jhazmat.2008.06.048
Alvarez, S., and Jerez, C. A. (2004). Copper ions stimulate polyphosphate degradation and phosphate efflux in Acidithiobacillus ferrooxidans. Appl. Environ. Microbiol. 70, 5177–5182. doi: 10.1128/aem.70.9.5177-5182.2004
Andreeva, N. A., Kulakovskaya, T. V., and Kulaev, I. S. (2004). Purification and properties of exopolyphosphatase from the cytosol of Saccharomyces cerevisiae not encoded by the PPX1 gene. Biochemistry 69, 387–393. doi: 10.1023/b:biry.0000026193.44046.29
Aravind, L., and Koonin, E. V. (1998). The HD domain defines a new superfamily of metal-dependent phosphohydrolases. Trends Biochem. Sci. 23, 469–472. doi: 10.1016/s0968-0004(98)01293-6
Avilés, C., Loza-Tavera, H., Terry, N., and Moreno-Sánchez, R. (2003). Mercury pretreatment selects an enhanced cadmium-accumulating phenotype in Euglena gracilis. Arch. Microbiol. 180, 1–10. doi: 10.1007/s00203-003-0547-2
Aziz, R. K., Bartels, D., Best, A. A., DeJongh, M., Disz, T., Edwards, R. A., et al. (2008). The RAST Server: rapid annotations using subsystems technology. BMC Genomics 9:75. doi: 10.1186/1471-2164-9-75
Baker-Austin, C., Dopson, M., Wexler, M., Sawers, R. G., and Bond, P. L. (2005). Molecular insight into extreme copper resistance in the extremophilic archaeon Ferroplasma acidarmanus Fer1. Microbiology 151, 2637–2646. doi: 10.1099/mic.0.28076-28070
Bolger, A. M., Lohse, M., and Usadel, B. (2014). Trimmomatic: a flexible trimmer for Illumina sequence data. Bioinformatics 30, 2114–2120. doi: 10.1093/bioinformatics/btu170
Brown, M. R., and Kornberg, A. (2008). The long and short of it polyphosphate, PPK and bacterial survival. Trends Biochem. Sci. 33, 284–290. doi: 10.1016/j.tibs.2008.04.005
Das, D., Salgaonkar, B. B., Mani, K., and Braganca, J. M. (2014). Cadmium resistance in extremely halophilic archaeon Haloferax strain BBK2. Chemosphere 112, 385–392. doi: 10.1016/j.chemosphere.2014.04.058
Focardi, S., Pepi, M., Landi, G., Gasperini, S., Ruta, M., and Di Biasio, P. (2012). Hexavalent chromium reduction by whole cells and cell free extract of the moderate halophilic bacterial strain Halomonas sp TA-04. Int. Biodeter. Biodegr. 66, 63–70. doi: 10.1016/j.ibiod.2011.11.003
Galagan, J. E., Nusbaum, C., Roy, A., Endrizzi, M. G., Macdonald, P., FitzHugh, W., et al. (2002). The genome of M. acetivorans reveals extensive metabolic and physiological diversity. Genome Res. 12, 532–542. doi: 10.1101/gr.223902
García-García, J. D., Sánchez-Thomas, R., and Moreno-Sánchez, R. (2016). Bio-recovery of non-essential heavy metals by intra-and extracellular mechanisms in free-living microorganisms. Biotechnol. Adv. 34, 859–873. doi: 10.1016/j.biotechadv.2016.05.003
Gilmour, C. C., Bullock, A. L., McBurney, A., Podar, M., and Elias, D. A. (2018). Robust mercury methylation across diverse methanogenic Archaea. MBio 9, e2403–e2417. doi: 10.1128/mBio.02403-2417
Gohara, D. W., and Di Cera, E. (2016). Molecular mechanisms of enzyme activation by monovalent cations. J. Biol. Chem. 29, 20840–20848. doi: 10.1074/jbc.R116.737833
Gomez Garcia, M. R. (2014). Extraction and quantification of polyP, polyP analysis by Urea-PAGE. Bio Prot. 4:e1113. doi: 10.21769/BioProtoc.1113
Gurevich, A., Saveliev, V., Vyahhi, N., and Tesler, G. (2013). QUAST: quality assessment tool for genome assemblies. Bioinformatics 29, 1072–1075. doi: 10.1093/bioinformatics/btt086
Harris, D. R., Ward, D. E., Feasel, J. M., Lancaster, K. M., Murphy, R. D., Mallet, T. C., et al. (2005). Discovery and characterization of a coenzyme A disulfide reductase from Pyrococcus horikoshii. Implications for this disulfide metabolism of anaerobic hyper-thermophiles. FEBS J. 272, 1189–1200. doi: 10.1111/j.1742-4658.2005.04555.x
Holmes, D. E., Orelana, R., Giloteaux, L., Wang, L. Y., Shrestha, P. M., Williams, K., et al. (2018). Potential for Methanosarcina to contribute to uranium reduction during acetate-promoted groundwater bioremediation. Microb. Ecol. 76, 660–667. doi: 10.1007/s00248-018-1165-1165
Jasso-Chávez, R., Diaz-Perez, C., Rodríguez-Zavala, J. S., and Ferry, J. G. (2017). Functional role of MrpA in the MrpABCDEFG Na+/H+ antiporter complex from the archaeon Methanosarcina acetivorans. J. Bacteriol. 199:e00662-16. doi: 10.1128/JB.00662-16
Jasso-Chávez, R., Santiago-Martínez, M. G., Lira-Silva, E., Pineda, E., Zepeda-Rodríguez, A., Belmont-Díaz, J., et al. (2015). Air-Adapted Methanosarcina acetivorans shows high methane production and develops resistance against oxygen stress. PLoS One 10:e0117331. doi: 10.1371/journal.pone.0117331
Jurica, M. S., Mesecar, A., Heath, P. J., Shi, W., Nowak, T., and Stoddard, B. L. (1998). The allosteric regulation of pyruvate kinase by fructose-1,6-bisphosphate. Structure 6, 195–210. doi: 10.1016/S0969-2126(98)00021-5
Keasling, J. D., and Hupf, G. A. (1996). Genetic manipulation of polyphosphate metabolism affects cadmium tolerance in Escherichia coli. Appl. Environ. Microbiol. 62, 743–746.
Küpper, H., Šetlík, I., Spiller, M., Küpper, F., and Prášil, O. (2002). Heavy metal-induced inhibition of photosynthesis: targets of in vivo heavy metal chlorophyll formation. J. Phycol. 38, 429–441. doi: 10.1046/j.1529-8817.2002.01148.x
LeBel, D., Poirier, G. G., and Beaudoin, A. R. (1978). A convenient method for the ATPase assay. Anal. Biochem. 85, 86–89. doi: 10.1016/0003-2697(78)90277-4
Leung, W. C., Wong, M. F., Chua, H., Lo, W., Yu, P. H. F., and Leung, C. K. (2000). Removal and recovery of heavy metals by bacteria isolated from activated sludge treating industrial effluents and municipal wastewater. Water. Sci. Technol. 41, 233–240. doi: 10.2166/wst.2000.0277
Lindner, S. N., Knebel, S., Wesseling, H., Schoberth, S. M., and Wendisch, V. F. (2009). Exopolyphosphatases PPX1 and PPX2 from Corynebacterium glutamicum. Appl. Environ. Microbiol. 75, 3161–3170. doi: 10.1128/AEM.02705-2708
Lindner, S. N., Vidaurre, D., Willbold, S., Schoberth, S. M., and Wendisch, V. F. (2007). NCgl2620 encodes a class II polyphosphate kinase in Corynebacterium glutamicum. Appl. Environ. Microbiol. 73, 5026–5033. doi: 10.1128/AEM.00600-07
Lira-Silva, E., Santiago-Martínez, M. G., García-Contreras, R., Zepeda-Rodríguez, A., Marín-Hernández, A., Moreno-Sánchez, R., et al. (2013). Cd2+ resistance mechanisms in Methanosarcina acetivorans involve the increase in the coenzyme M content and induction of biofilm synthesis. Environ. Microbiol. Rep. 5, 799–808. doi: 10.1111/1758-2229.12080
Lira-Silva, E., Santiago-Martínez, M. G., Hernández-Juárez, V., García-Contreras, R., Moreno-Sánchez, R., and Jasso-Chávez, R. (2012). Activation of methanogenesis by cadmium in the marine archaeon Methanosarcina acetivorans. PloS One 7:e48779. doi: 10.1371/journal.pone.0048779
Livak, K. J., and Schmittgen, T. D. (2001). Analysis of relative gene expression data using real-time quantitative PCR and the 2- ΔΔCT method. Methods 25, 402–408. doi: 10.1006/meth.2001.1262
Mangold, S., Potrykus, J., Björn, E., Lövgren, L., and Dopson, M. (2013). Extreme zinc tolerance in acidophilic microorganisms from the bacterial and archaeal domains. Extremophiles 17, 75–85. doi: 10.1007/s00792-012-0495-3
McCarthy, S., Ai, C., Wheaton, G., Tevatia, R., Eckrich, V., Kelly, R., et al. (2014). Role of an archaeal PitA transporter in the copper and arsenic resistance of Metallosphaera sedula an extreme thermoacidophile. J. Bacteriol. 196, 3562–3570. doi: 10.1128/JB.017071714
Meyer, C. L., Pauwels, M., Briset, L., Godé, C., Salis, P., Bourceaux, A., et al. (2016). Potential preadaptation to anthropogenic pollution: evidence from a common quantitative trait locus for zinc and cadmium tolerance in metallicolous and non metallicolous accessions of Arabidopsis halleri. New Phytol. 212, 934–943. doi: 10.1111/nph.14093
Moreno-Sánchez, R., Rodríguez-Enríquez, S., Jasso-Chávez, R., Saavedra, E., and García-García, J. D. (2017). Biochemistry and physiology of heavy metal resistance and accumulation in Euglena. Adv. Exp. Med. Biol. 979, 91–121. doi: 10.1007/978-3-319-54910-1_6
Mullan, A., Quinn, J. P., and McGrath, J. W. (2002). A nonradioactive method for the assay of polyphosphate kinase activity and its application in the study of polyphosphate metabolism in Burkholderia cepacia. Anal. Biochem. 308, 294–299. doi: 10.1016/S0003-2697(02)00249-X
Proudfoot, M., Kuznetsova, E., Brown, G., Rao, N. N., Kitagawa, M., Mori, H., et al. (2004). General enzymatic screens identify three new nucleotidases in Escherichia coli biochemical characterization of SurE. J. Biol. Chem. 279, 54687–54694. doi: 10.1074/jbc.M411023200
Ranjard, L., Nowak, V., Echairi, A., Faloy, V., and Chaussod, R. (2008). The dynamics of soil bacterial community structure in response to yearly repeated agricultural copper treatments. Res. Microbiol. 159, 251–254. doi: 10.1016/j.resmic.2008.02.004
Remonsellez, F., Orell, A., and Jerez, C. A. (2006). Copper tolerance of the thermoacidophilic archaeon Sulfolobus metallicus: possible role of polyphosphate metabolism. Microbiology 152, 59–66. doi: 10.1099/mic.0.28241-0
Rissman, A. I., Mau, B., Biehl, B. S., Darling, A. E., Glasner, J. D., and Perna, N. T. (2009). Reordering contigs of draft genomes using the Mauve aligner. Bioinformatics 25, 2071–2073. doi: 10.1093/bioinformatics/btp356
Rivero, M., Torres-Paris, C., Muñoz, R., Cabrera, R., Navarro, C. A., and Jerez, C. A. (2018). Inorganic polyphosphate, exopolyphosphatase and Pho84-like transporters may be involved in copper resistance in Metallosphaera sedula DSM5348T. Archaea. 2018:5251061. doi: 10.1155/2018/5251061
Rohlin, L., and Gunsalus, R. P. (2010). Carbon-dependent control of electron transfer and central carbon pathway genes for methane biosynthesis in the Archaean, Methanosarcina acetivorans strain C2A. BMC Microbiol. 10:62. doi: 10.1186/1471-2180-10-62
Saavedra, E., Encalada, R., Pineda, E., Jasso-Chávez, R., and Moreno-Sánchez, R. (2005). Glycolysis in Entamoeba histolytica. FEBS J. 272, 1767–1783. doi: 10.1111/j.1742-4658.2005.04610.x
Santiago-Martínez, M. G., Encalada, R., Lira-Silva, E., Pineda, E., Gallardo-Pérez, J. C., Reyes-García, M. A., et al. (2016). The nutritional status of Methanosarcina acetivorans regulates glycogen metabolism and gluconeogenesis and glycolysis fluxes. FEBS J. 283, 1979–1999. doi: 10.1111/febs.13717
Simpson, J. T., Wong, K., Jackman, S. D., Schein, J. E., Jones, S. J. M., and Birol, I. (2009). ABySS: a parallel assembler for short read sequence data. Genome Res. 19, 1117–1123. doi: 10.1101/gr.089532.108
Singh, R., Dong, H., Liu, D., Zhao, L., Marts, A. R., Farquhar, E., et al. (2015). Reduction of hexavalent chromium by the thermophilic methanogen Methanothermobacter thermautotrophicus. Geochim. Cosmochim. Acta 148, 442–456. doi: 10.1016/j.gca.2014.10.012
Skorko, R. (1989). Polyphosphate as a source of phosphoryl group in protein modification in the archaebacterium Sulfolobus acidocaldarius. Biochimie 71, 1089–1093. doi: 10.1016/0300-9084(89)90115-6
Skórko, R., Osipiuk, J., and Stetter, K. O. (1989). Glycogen-bound polyphosphate kinase from the archaebacterium Sulfolobus acidocaldarius. J. Bacteriol. 171, 5162–5164. doi: 10.1128/jb.171.9.5162-5164.1989
Sowers, K. R., Boone, J. E., and Gunsalus, R. P. (1993). Disaggregation of Methanosarcina spp. and growth as single cells at elevated osmolarity. Appl. Environ. Microbiol. 59, 3832–3839.
Spada, S., Pembroke, T. J., and Wall, G. J. (2002). Isolation of a novel Thermus thermophilus metal efflux protein that improves Escherichia coli growth under stress conditions. Extremophiles 6, 301–308. doi: 10.1007/s00792-001-0257-0
Studier, F. W. (2005). Protein production by auto-induction in high-density shaking cultures. Prot. Expr. Purif. 41, 207–234. doi: 10.1016/j.pep.2005.01.016
Toso, D. B., Javed, M. M., Czornyj, E., Gunsalus, R. P., and Zhou, Z. H. (2016). Discovery and characterization of iron sulfide and polyphosphate bodies coexisting in Archaeoglobus fulgidus cells. Archaea 2016:4706532. doi: 10.1155/2016/4706532
Keywords: archaeal metabolism, heavy metal-binding molecules, polyphosphate kinase, exopolyphosphatase, biofilm induction, methanogenesis
Citation: Jasso-Chávez R, Lira-Silva E, González-Sánchez K, Larios-Serrato V, Mendoza-Monzoy DL, Pérez-Villatoro F, Morett E, Vega-Segura A, Torres-Márquez ME, Zepeda-Rodríguez A and Moreno-Sánchez R (2019) Marine Archaeon Methanosarcina acetivorans Enhances Polyphosphate Metabolism Under Persistent Cadmium Stress. Front. Microbiol. 10:2432. doi: 10.3389/fmicb.2019.02432
Received: 27 May 2019; Accepted: 09 October 2019;
Published: 24 October 2019.
Edited by:
Cassandre Sara Lazar, Université du Québec à Montréal, CanadaReviewed by:
Emma Jayne Gagen, The University of Queensland, AustraliaCopyright © 2019 Jasso-Chávez, Lira-Silva, González-Sánchez, Larios-Serrato, Mendoza-Monzoy, Pérez-Villatoro, Morett, Vega-Segura, Torres-Márquez, Zepeda-Rodríguez and Moreno-Sánchez. This is an open-access article distributed under the terms of the Creative Commons Attribution License (CC BY). The use, distribution or reproduction in other forums is permitted, provided the original author(s) and the copyright owner(s) are credited and that the original publication in this journal is cited, in accordance with accepted academic practice. No use, distribution or reproduction is permitted which does not comply with these terms.
*Correspondence: Ricardo Jasso-Chávez, cmphc3NfY2FyZGlvbEB5YWhvby5jb20ubXg=; cmphc3NvY2hAZ21haWwuY29t
Disclaimer: All claims expressed in this article are solely those of the authors and do not necessarily represent those of their affiliated organizations, or those of the publisher, the editors and the reviewers. Any product that may be evaluated in this article or claim that may be made by its manufacturer is not guaranteed or endorsed by the publisher.
Research integrity at Frontiers
Learn more about the work of our research integrity team to safeguard the quality of each article we publish.