- 1School of Municipal and Environmental Engineering, Shandong Jianzhu University, Jinan, China
- 2Shandong Medicinal Biotechnology Center, Shandong Academy of Medical Sciences, Shandong First Medical University, Jinan, China
- 3Key Laboratory for Biotech-Drugs of National Health Commission, Jinan, China
- 4State Key Laboratory of Microbial Technology, School of Life Science, Shandong University, Qingdao, China
Microlunatus phosphovorus NM-1 is a polyphosphate (poly-P)-accumulating bacterium that accumulates poly-P under aerobic conditions and degrades poly-P under anaerobic conditions. In this study, the two-component system (TCS) PolS-PolR was identified in NM-1, and the response regulator PolR was found to directly bind to the promoters of genes related to phosphate transport (MLP_RS00235, MLP_RS23035, and MLP_RS24590); poly-P catabolism (MLP_RS12905) and poly-P synthesis (MLP_RS23025). RT-qPCR assays showed that ppgk (MLP_RS12905), ppk (MLP_RS23025), pstS (MLP_RS23035), and pit (MLP_RS24590) were down-regulated during the aerobic-anaerobic shift. The sequence GTTCACnnnnnGTTCaC was identified as a recognition sequence for PolR by MEME analysis and DNase I footprinting. EMSAs and ChIP-qPCR assays indicated that PolR binds to the promoters of pit (MLP_RS00235), ppgk (MLP_RS12905), ppk (MLP_RS23025), pstS (MLP_RS23035) and pit (MLP_RS24590), and ChIP-qPCR further suggested that the binding affinity of PolR was lower under anaerobic conditions than under aerobic conditions in vivo. These findings indicate that the PolS-PolR TCS in M. phosphovorus may be involved in the regulation of poly-P metabolism in response to levels of dissolved oxygen in the environment, and our results provide insights into new approaches for understanding the mechanisms of phosphorus accumulation and release.
Introduction
High concentrations of phosphorus are a leading factor in surface water eutrophication, and eutrophication may occur when the total phosphorus amount achieves 200–500 mg/m2/year in the water (Strokal et al., 2014). However, the residual phosphorus in waste-water can be efficiently removed by an activated sludge process, known as the enhanced biological phosphorus removal (EBPR) system, which contains cyclic anaerobic and aerobic stages (Winkler et al., 2011; Wang et al., 2015; Zhang et al., 2015). The EBPR process produces an effluent with a very low phosphorus content and a sludge enriched in phosphorus, from which microorganisms can “extravagantly” take up more inorganic phosphate than needed metabolically and convert the soluble phosphate to the insoluble phase in the form of intracellular polyphosphate (poly-P) (Tarayre et al., 2016).
The microorganisms that contributed to enriching poly-P in the EBPR system were called polyphosphate-accumulating organisms (PAOs) (Mino, 2000). Acinetobacter spp. were first investigated as PAOs, and “Candidatus Accumulibacter,” Lampropedia sp., Tetrasphaera elongate, and Microlunatus phosphovorus were also considered potentially responsible for phosphorus-removal in activated sludge (Crocetti et al., 2000; He and McMahon, 2011; Tarayre et al., 2016; Coats et al., 2017). M. phosphovorus NM-1 (Actinobacteria, Propionibacteriaceae), which was isolated and identified in 1995, can accumulate large amounts of poly-P (Nakamura et al., 1995), and this species accounts for 9.0% of PAOs in the EBPR system (Mino, 2000; Coats et al., 2017).
The genome sequence of M. phosphovorus NM-1 was reported in 2012 (Kawakoshi et al., 2012). Dozens of genes were predicted to be involved in the metabolic processing of poly-P, including: ppk2 (MLP_RS02760, MLP_RS24205) and ppk (MLP_RS23025) which encode polyphosphate kinases (PPK) that catalyze the conversion of ATP into poly-P; ppx (MLP_RS21635), which encodes the exopolyphosphatase (PPX) that subsequently hydrolyzes the terminal residues of poly-P into inorganic phosphate (Pi); ppgk (MLP_RS02595, MLP_RS12905) encoding the polyphosphate glucokinase (PPGK) that phosphorylates glucose using poly-P; pap (MLP_RS11265), encoding a polyphosphate:AMP phosphotransferase (PAP) that synthesizes ADP using poly-P and AMP; and ppnk (MLP_RS08475), which encodes a polyphosphate/ATP-dependent NAD kinase (PPNK) that synthesizes NADP+ using poly-P and ATP. In addition, pstSCAB (MLP_RS23035, MLP_RS23040, MLP_RS23045, and MLP_RS23050) encode the high-affinity Pi-specific transport (Pst) system PstSCAB, which can transport phosphate into cells under low phosphorus concentrations. pit (MLP_RS00235, MLP_RS14415, and MLP_RS24590) encode three low-affinity inorganic Pi transport (Pit) systems, which can transport inorganic phosphate into or out of the cell under high phosphorus concentrations (Tanaka et al., 2003; He and McMahon, 2011; Kawakoshi et al., 2012; Tu and Schuler, 2013). However, the mechanisms regulating poly-P biosynthesis have not been fully delineated, and there are no reports about the global regulation of poly-P metabolism in M. phosphovorus.
Previous studies revealed that PAOs, including M. phosphovorus, can synthesize poly-P under aerobic conditions and degrade poly-P under anaerobic conditions (Tsai and Liu, 2002; Coats et al., 2017), although the regulatory mechanism remained unclear. Two-component systems (TCSs), containing a response regulator (RR) and histidine kinase (HK), normally respond to changes in environmental factors and influence development and metabolism in bacteria (Zschiedrich et al., 2016). In this study, we identified the TCS PolS-PolR, which is annotated as MLP_RS10490—MLP_RS10500 in M. phosphovorus NM-1 and which may be involved in the regulation of poly-P metabolism in response to the level of dissolved oxygen. Target genes of PolS-PolR that are related to poly-P metabolism were also detected in M. phosphovorus NM-1, and our results provide insights into new approaches for investigating mechanisms of phosphorus accumulation and release.
Materials and Methods
Strains and Culture Conditions
Microlunatus phosphovorus NM-1 was obtained from the American Type Culture Collection (Accession number ATCC700054) and grown in a Sequencing Batch Reactor (SBR) containing synthetic waste-water as described previously (Zhong et al., 2018a). Escherichia coli strains DH5α and BL21(DE3)pLysS were used, respectively, for general cloning and for the expression of PolR. All E. coli strains were cultured in Luria-Bertani (LB) medium or on LB-agar medium, and antibiotics were added as needed.
Heterologous Expression and Purification of His-Tagged PolR
The polR gene of M. phosphovorus NM-1 was amplified using primers PolR-28aFor and PolR-28aRev (Table S1) and then inserted into the pMD18-T vector. After confirmation by DNA sequencing, polR gene was cloned into pET-28a resulting in the pET-PolR recombinant vector, which was then introduced into E. coli BL21(DE3)pLysS for protein expression. His-tagged PolR protein was induced by isopropyl β-D-1-thiogalactopyranoside and purified using Ni-NTA. The polyclonal antibody to PolR in rabbits was produced by GenScript Biotech Corporation (Nanjing, China).
Western Blotting Assay
To evaluate the expression of PolR in NM-1, cells from culture stages T3 and T4 in the SBR (Figure 1A) were collected by centrifugation at 10,000 g for 10 min, and then pellets were washed and resuspended in PBS buffer followed by disruption with the sample preparation system (FastPrep, MP). Protein samples in the supernatants were obtained by centrifugation and then separated using a NuPAGE 4–12% gradient polyacrylamide gel (Invitrogen). The protein samples were transferred onto Hybond ECL membrane (GE Life Sciences) by a Trans-blot device (Bio-Rad). The polyclonal antibody to PolR and the Western ECL detection system (Amersham) were used in the immunoblotting assay.
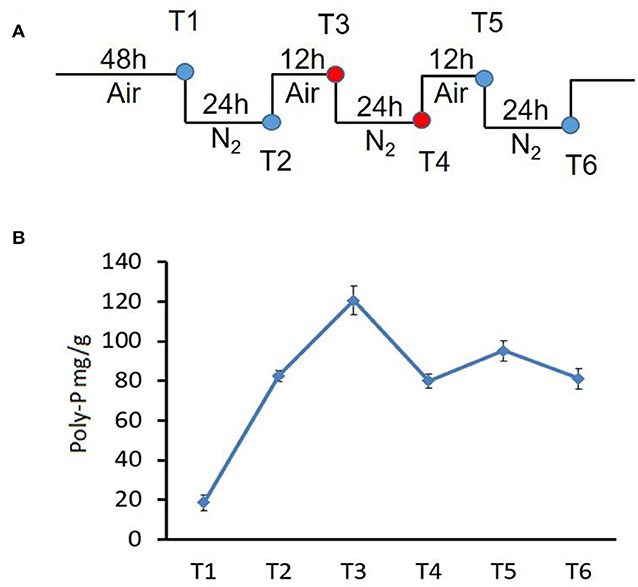
Figure 1. Changes in poly-P content during growth of strain NM-1 in the SBR system. (A) Diagram of the aerobic-anaerobic shifts during the SBR process. T1–T6 refer to growth stages. (B) Cell pellets were collected from the SBR at different times, and the poly-P content was determined. The values were obtained by three independent experiments and are shown as means ± standard deviations.
Poly-P Content Analysis
To investigate the metabolism of poly-P under aerobic and anaerobic conditions, bacterial cells were collected at stages T1-T6 from the SBR (Figure 1A), and the amounts of poly-P were determined using described methods (Zhong et al., 2018a).
Electrophoretic Mobility Shift Assays (EMSAs)
The fragments of promoters of genes involved in poly-P metabolism were amplified by PCR using primers listed in Table S1. The PCR products were labeled by biotin at the 3′-end using the Biotin 3′ End DNA Labeling kit (Thermo Scientific). Purified His-tagged PolR was used in the assays, with described procedures (Zhang et al., 2014). For competition assays, excess of unlabelled specific probes and non-specific probes were added into the reaction. The mixtures were analyzed on non-denaturing page (8% polyacrylamide), then the DNA probes were transferred to nylon membrane and developed by ECL Western blotting Analysis System (GE Healthcare).
RNA Extraction and Real-Time Quantitative PCR (RT-qPCR)
For total RNA extraction, bacterial cells were collected by centrifugation at stages T3–T4 from the SBR, and then the cells were disrupted using the FastPrep sample preparation system. TRIzol (Takara) was used for the extraction of total RNA, and Turbo DNA-free DNase reagents (Thermofisher) were used to remove DNA contamination. RNA samples were then reverse transcribed into cDNA using an M-MLV reverse transcriptase kit (Takara). RT-qPCR was performed by using SYBR Premix Ex Taq kit (TaKaRa) on Roche LightCycler480 thermal cycler. According to the recommended conditions of kit, the relative amounts of cDNA were normalized for the quantity of 16s rRNA. The primers used for RT-qPCR are listed in Table S1.
DNase I Footprinting Assay
DNase I footprinting was performed as described (Wang et al., 2012). Briefly, the promoter fragment of pit (MLP_RS00235) was amplified and ligated into the pMD18-T vector, and then the DNA fragment was amplified from the resulting plasmid template using primers M13F-47 (FAM-labeled) and M13R-48, and purified as a probe. A certain amount of probes were incubated with 0 μg PolR and 3.0 μg PolR for 30 min at room temperature, followed by incubated with DNase I for 1 min at 25°C. And then the reactions were stopped by DNase I stop solution and extracted by phenol/chloroform. DNA samples were precipitated by ethanol and dissolved in ultrapure water.
Chromatin Immunoprecipitation and Real-Time Quantitative PCR Assay (ChIP-qPCR Assay)
The chromatin immunoprecipitation assay was performed as previously described (Fu et al., 2019). Bacterial cells were collected at stages T3 and T4 from the SBR, and the polyclonal antibody to PolR and protein G-Agarose (Sigma-Aldrich) were used to recover target DNA. A qPCR assay was performed to determine the enrichment of promoter fragments of genes related to poly-P metabolism (primers are listed in Table S1). For the negative control, the input DNA (non-immunoprecipitated) was used as template.
Identification of a PolR DNA Binding Motif
The sequences of promoters bound by PolR were used as input for the MEME software tool (Bailey et al., 2015) and RegPredict (Novichkov et al., 2010) for the motif search.
Results
M. phosphovorus NM-1 Accumulates Poly-P Under Aerobic Conditions and Degrades Poly-P During Anaerobic Conditions
To investigate poly-P metabolism in M. phosphovorus NM-1, the strain was cultured in synthetic waste-water in an SBR. Strain NM-1 was first incubated under aerobic conditions for 48 h (T1), and the air was then replaced by N2 to maintain anaerobic conditions for 24 h (T2), followed by another aerobic phase for 12 h (T3), and then a second anaerobic phase for 24 h (T4) (Figure 1A). A third aerobic phase was then carried out for 12 h (T5), followed by a third anaerobic phase for 24 h (T6) (Figure 1A). The poly-P content of strain NM-1 was analyzed during the SBR stages, reaching 18.61 mg/g cells at T1 and 82.64 mg/g cells at T2. Poly-P increased to 120.58 mg/g in the second aerobic phase (T3) but decreased to 80.02 mg/g in the following anaerobic phase (T4). Poly-P again increased in the third aerobic phase, to 95.21 mg/g at T5, followed by another decrease in the third anaerobic phase, to 81.12 mg/g at T6 (Figure 1B). These changes in poly-P content indicated that strain NM-1 can synthesize poly-P under aerobic conditions and degrade poly-P during anaerobic conditions, which is consistent with previously reported results (Nakamura et al., 1995).
PolS-PolR Is Predicted to Be a TCS in M. phosphovorus NM-1
Although the metabolism of poly-P differed in NM-1 under aerobic and anaerobic conditions, the genes regulating the response to the changing levels of dissolved oxygen remained largely uncharacterized. In Mycobacterium tuberculosis, the DevS-DevR TCS responds to oxygen levels, with the GAF domain of the HK DevS sensing oxygen signals, and following phosphorylation by DevS, the RR DevR regulates the expression of dormancy-related genes (Madrona et al., 2016; Lobao et al., 2019). We speculated that a TCS might mediate the transition response to changing oxygen levels in M. phosphovorus, and bioinformatics analysis indicated that its genome (NC_015635.1) contained three genes encoding proteins with GAF-like domains: MLP_RS09510, MLP_RS09650, and MLP_RS10500 (Figure 2). MLP_RS09510 has low similarity with DevS, and MLP_RS09650 is an orphan histidine kinase. MLP_RS10500 has 38% amino acid identity with DevS, and similar to DevS, it contains two GAF domains at the N terminus and a histidine kinase-like ATPase at the C terminus. The second GAF domain of DevS was demonstrated to sense signals such as oxygen, hypoxia, CO or NO (Madrona et al., 2016). In addition, MLP_RS10490 has 76% identity with DevR, which has a helix-turn-helix (HTH) DNA binding domain. Given their close location on the genome and their similarities to DevS and DevR, we predicted that MLP_RS10500 and MLP_RS10490 formed a TCS involved in the hypoxic signal transduction pathway, and we named this TCS PolS-PolR for the putative regulation of poly-P metabolism.
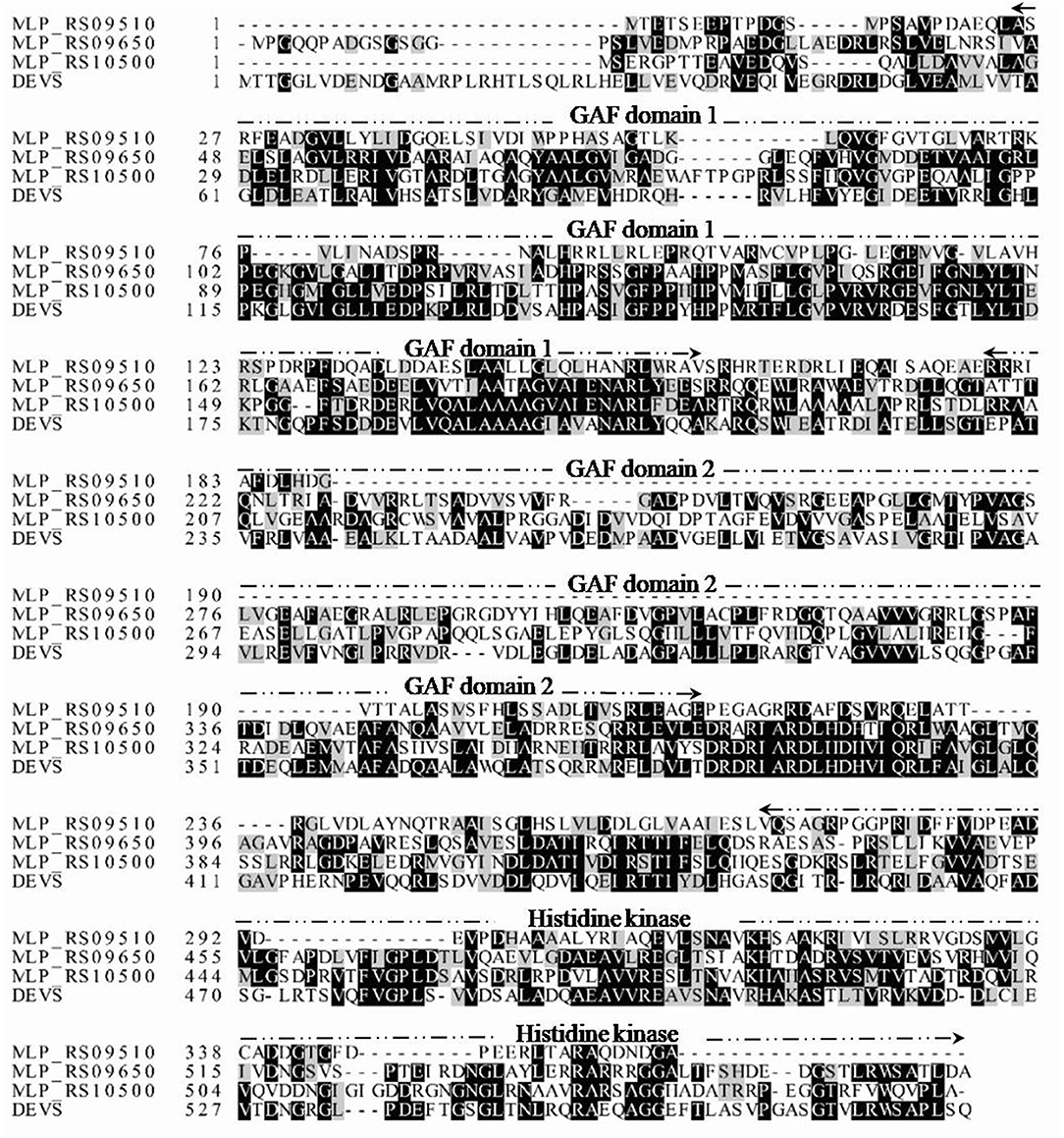
Figure 2. Multiple sequence alignment of M. tuberculosis DevS with putative GAF-containing proteins of strain NM-1. Darkened background indicates conserved amino acids. GAF domain 1, GAF domain 2, and the histidine kinase domain are marked by dotted lines.
PolR Binds to the Promoters of Five Genes Related to Poly-P Metabolism in vitro
In order to investigate if the genes related to poly-P metabolism are targets of PolR (MLP_RS10490), EMSAs were performed using His-tagged PolR protein. The promoters of genes involved in the synthesis and hydrolysis of poly-P were amplified by PCR and labeled by biotin for use as probes. The results indicated that PolR binds to the promoters of pit (MLP_RS00235, MLP_RS24590), ppgk (MLP_RS12905), ppk (MLP_RS23025), and pstS (MLP_RS23035) (Figure 3), which is consistent with a previous report (Zhong et al., 2018b), in which EMSA shows the homologous protein of PolR binds to the promoters of responding genes in M. phosphovorus JN459, suggesting that these genes could be targets of PolR. The results of competitive EMSAs assay (Figure S1) indicated that PolR binds to the promoters specifically. ppgk (MLP_RS12905), which encodes polyphosphate glucokinase, is responsible for the catabolism of poly-P. pstS (MLP_RS23035) encodes a component of the PstSCAB transport system, which is involved in the transport of phosphate into cells at low environmental phosphorus levels, whereas pit (MLP_RS00235) and pit (MLP_RS24590) encode a Pit transport system for transporting phosphate at high phosphate levels. ppk (MLP_RS23025) encodes a polyphosphate kinase that is involved in the synthesis of poly-P. We hypothesized that PolR could regulate poly-P metabolism by directly regulating the transcription of genes related to phosphate uptake and synthesis or catabolism of poly-P. While PolR could not bind to the promoters of ppgk (MLP_RS02595), ppk2 (MLP_RS02760, MLP_RS24205), ppnk (MLP_RS08475), pap (MLP_RS11265), phoB (MLP_RS11885), pit (MLP_RS14415), and phoU (MLP_RS22885) (Figure 3), suggesting that these genes are not directly regulated by PolR.
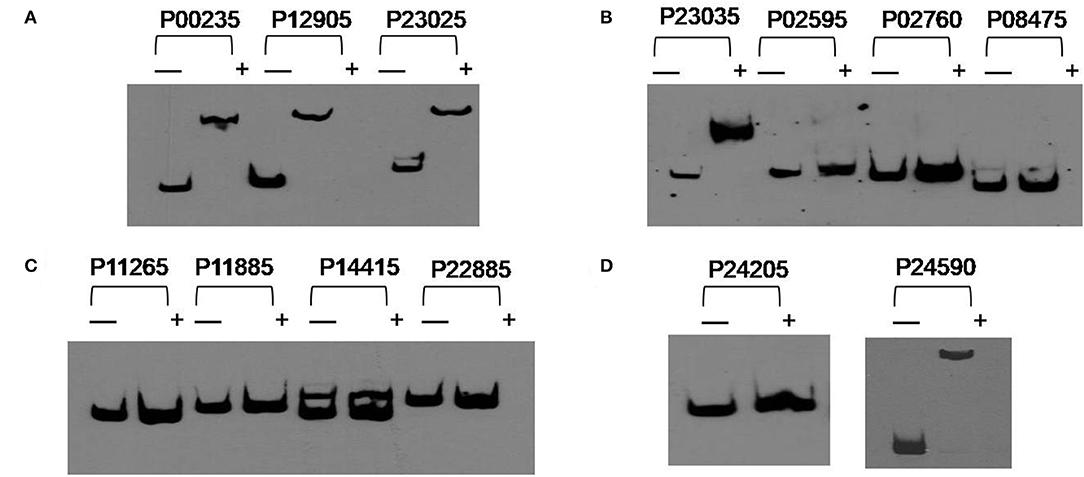
Figure 3. PolR binding to the promoter regions of predicted target genes. (A–D) EMSAs of the promoter regions of predicted target genes with purified His-tagged PolR. Probes for different promoters were incubated either without protein (–) or with 3.0 μg PolR (+). Poly(dI-dC) (1.0 μg) was added to all samples as competitor.
Expression of Several PolR Target Genes Changed Significantly During the Aerobic-Anaerobic Shift
To find out whether the transcription of target genes of PolR was changed during the aerobic-anaerobic shift, the transcription levels of pit (MLP_RS00235, MLP_RS24590), ppgk (MLP_RS12905), ppk (MLP_RS23025), and pstS (MLP_RS23035) in M. phosphovorus NM-1 at T3 (aerobic) and T4 (anaerobic) were analyzed by RT-qPCR. Expression of ppgk (MLP_RS12905), ppk (MLP_RS23025), pstS (MLP_RS23035), and pit (MLP_RS24590) was significantly down-regulated under anaerobic conditions compared to aerobic conditions (Figure 4A). The transcription levels of ppgk (MLP_RS12905), ppk (MLP_RS23025), pstS (MLP_RS23035), and pit (MLP_RS24590) were 3.7-, 4.1-, 4.2-. and 2.8-fold higher at T3 than at T4, respectively. However, the transcription of pit (MLP_RS00235) showed no significant change (Figure 4A).
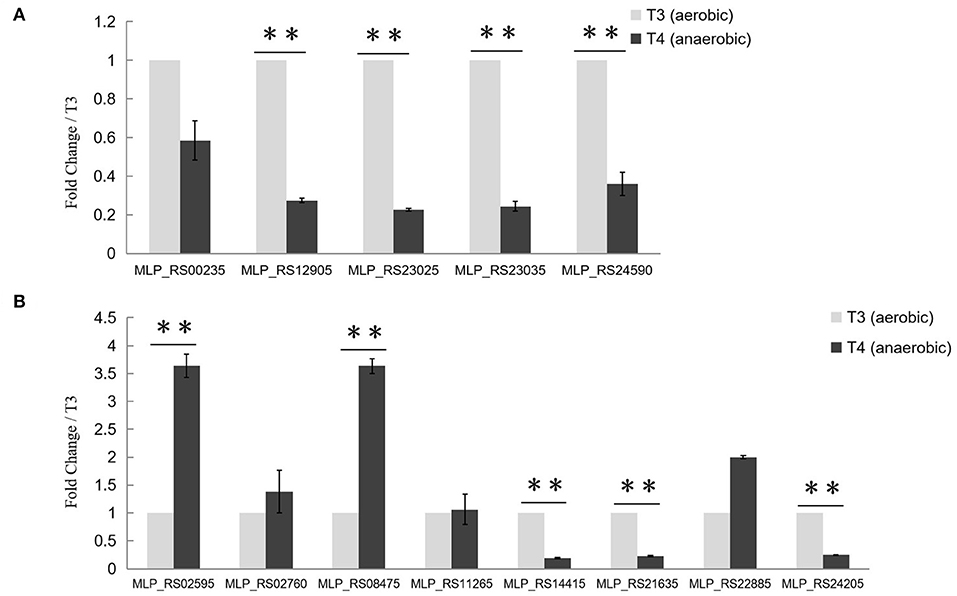
Figure 4. Transcriptional analysis of Poly-P metabolism related genes. (A) Transcriptional analysis of putative target genes of PolR. The expression of pit (MLP_RS00235, MLP_RS24590), ppgk (MLP_RS12905), ppk (MLP_RS23025), and pstS (MLP_RS23035) at stage T3 (aerobic condition, light gray) and stage T4 (anaerobic condition, dark gray) was determined by RT-qPCR. (B) Transcriptional analysis of other Poly-P metabolism related genes. The transcription levels of genes at T3 were set to one, and the expression of the 16S rRNA gene was used as the control. The values were obtained by three independent experiments and are shown as means ± standard deviations. **, compared with the expression data at stage T3, P < 0.01.
The transcription levels of other genes related to poly-P metabolism were also analyzed in this study (Figure 4B). ppgk (MLP_RS02595), ppnk (MLP_RS08475), ppx (MLP_RS21635), and pit (MLP_RS14415) which are related to the catabolism of poly-P, exhibited lower expression levels at stage T3 than at stage T4, whereas ppk2 (MLP_RS24205), the gene responsible for poly-P synthesis, was up-regulated at T3. While the expression levels of ppk2 (MLP_RS02760), pap (MLP_RS11265), and phoU (MLP_RS22885) at T3 showed no obvious difference with T4. A similar transcription pattern was found in M. phosphovorus JN459, as determined by RNA-seq assay (Table S2). Strain JN459, with a little higher concentration of Poly-P than that of NM-1, was derived from strain NM-1 through spontaneous mutation in EBPR system, while the mechanism of high ability to accumulate Poly-P remains unclear (Zhong et al., 2018a).
A Consensus Sequence for PolR Binding in Target Promoters
Based on the promoter targets of PolR, we deduced a conserved DNA binding sequence for PolR by MEME analysis (Figure 5A). The 17-bp consensus sequence GTTCACnnnnnGTTCaC, containing 6-bp direct repeats, was found in all five promoters bound by PolR in vitro (Figure 5B), whereas this sequence was not found in the promoters of genes that were not targets of PolR in the EMSAs. In order to validate the sequence as a target site for PolR binding, a DNase I footprinting assay was performed. A 32-bp region from the pit (MLP_RS00235) promoter was protected by PolR, and this region included the deduced consensus sequence (Figure 5C), providing further evidence that GTTCACnnnnnGTTCaC is the recognition sequence for PolR binding.
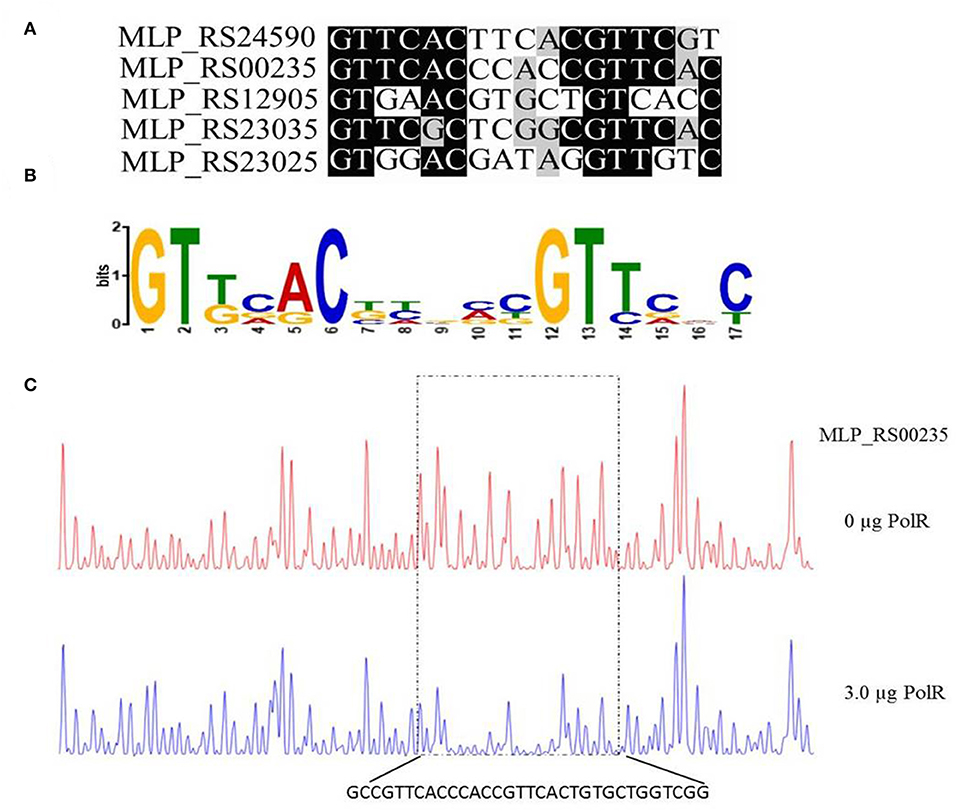
Figure 5. Determination of the PolR DNA binding sequence. (A) Alignment of promoters of target genes of PolR. The conserved nucleotides are marked by a dark background. (B) The consensus DNA binding site of PolR predicted by RegPredict. (C) PolR-protected region upstream of pit (MLP_RS00235) determined by DNaseI footprinting. The control reaction (no protein) is shown in red. The reaction including 3.0 μg PolR is shown in blue. The dotted box indicates the protected region. The nucleotides protected by PolR are shown beneath the chromatograms.
PolR Binding to Target Promoters in vivo Differs Under Aerobic and Anaerobic Conditions
Western blotting analysis with anti-PolR revealed bands corresponding to PolR protein in lysates of strain NM-1 and indicated that PolR is expressed under both aerobic and anaerobic conditions (Figure 6A). Two additional bands responding to the antibody of PolR were detected at T2–T6 stage, and we speculate that these bands might be the different modification of PolR. To confirm the target genes of PolR in vivo, a ChIP assay was performed with anti-PolR and strain NM-1 growing at stages T3 and T4, and qPCR was then performed to detect recovery of promoter fragments of genes associated with poly-P metabolism. The results showed that a region upstream of pit (MLP_RS00235) was enriched at T3 (17.13 ± 2.21) and T4 (17.97 ± 3.88) (Figure 6B), suggesting that PolR binds to the promoter of pit (MLP_RS00235) under both aerobic and anaerobic conditions. The promoter of ppgk (MLP_RS12905) was enriched at T3 (5.50 ± 0.98), but no enrichment was detected at T4 (Figure 6B). The promoter fragment of ppk (MLP_RS23025) was more highly enriched at T3 (7.23 ± 0.44) than at T4 (3.56 ± 0.11) (Figure 6B), suggesting that the binding affinity of PolR for the promoter of ppk (MLP_RS23025) under aerobic conditions is stronger than under anaerobic conditions. Similarly, the enrichment of the pstS (MLP_RS23035) promoter at T3 (4.32 ± 0.08) was about 2-fold higher than at T4 (2.16 ± 0.07), and the pit (MLP_RS24590) promoter was also more highly enriched at T3 (5.83 ± 1.53) than at T4 (1.17 ± 0.12) (Figure 6B). The ChIP-qPCR assay also showed that the promoters of the other genes associated with poly-P metabolism were not significantly enriched in the ChIP sample compared to the input sample, which is consistent with the EMSAs (Figure 6C).
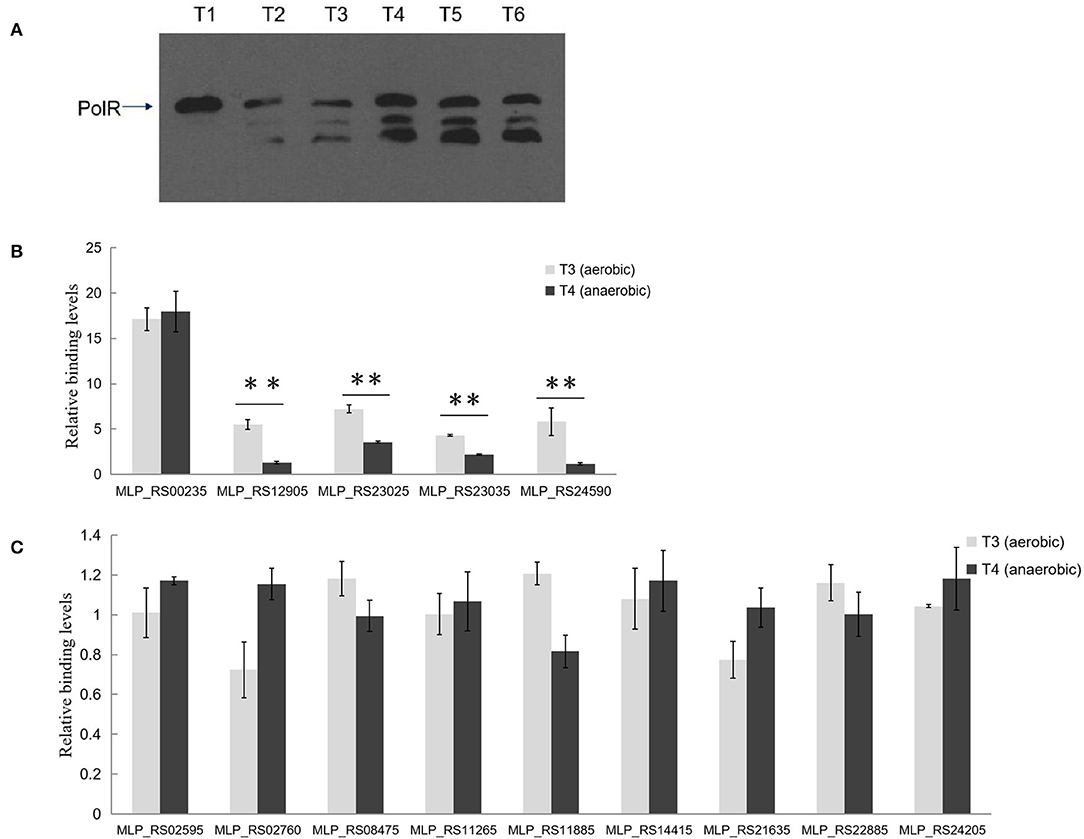
Figure 6. PolR binds to the promoter of genes associated with poly-P metabolism and phosphate transport in vivo. (A) The expression of PolR during growth in the SBR system was detected by Western blotting assay using anti-PolR. (B) The enrichment of the promoters of pit (MLP_RS00235, MLP_RS24590), ppgk (MLP_RS12905), ppk (MLP_RS23025), and pstS (MLP_RS23035) at stage T3 (aerobic condition, light gray) and stage T4 (anaerobic condition, dark gray) was analyzed by ChIP-qPCR assay. (C) The enrichment of the promoters of other Poly-P metabolism related genes. The relative enrichment value of each gene was normalized for the 16S rRNA gene, and the enrichment value of each gene in the input DNA samples was arbitrarily set to one. The values were obtained by three independent experiments and are shown as means ± standard deviations. **, compared with the data at stage T3, P < 0.01.
Discussion
The biosynthesis and degradation pattern of poly-P in PAOs is usually consistent with the hypothesis that the bacteria catabolize poly-P to supply energy for growth under anaerobic conditions (Zhou et al., 2010). However, the global regulatory mechanisms controlling poly-P metabolism in PAOs remained unclear, including for M. phosphovorus. Our findings in this study suggested that the PolS-PolR TCS may be involved in poly-P metabolism and soluble phosphorus transport. PolS has 38% amino acid identity to DevS, the HK that responds to hypoxia in M. tuberculosis (Sousa et al., 2017), suggesting that the PolS-PolR TCS may also respond to the levels of dissolved oxygen.
Poly-P is the signature metabolite produced by M. phosphovorus under aerobic conditions, and 11 genes were predicted to be involved in the metabolic processing of poly-P, including three phosphate permeases in the Pit system and a gene cluster for the phosphate transport system PstSCAB. Our study showed notable changes in the transcription levels of most of the genes involved in poly-P metabolism and Pi transport during the aerobic-anaerobic shift, with results similar to those found for strain JN459 (Zhong et al., 2018a). The transcription levels of the four genes ppgk (MLP_RS12905), ppk (MLP_RS23025), pstS (MLP_RS23035) and pit (MLP_RS24590) were down-regulated significantly at T4, which is an anaerobic stage, compared to levels at the aerobic stage T3. Additionally, these four genes were confirmed as targets of PolR by EMSAs and the ChIP-qPCR assay, and the binding affinities of PolR to their promoters in vivo were lower under anaerobic conditions than aerobic conditions. Combined with the transcriptional changes, we deduce that PolR positively regulates the transcription of the four target genes under aerobic conditions and that this control is reduced under anaerobic conditions. In the ChIP-qPCR assay, the high enrichment was detected at the promoter of pit (MLP_RS00235), which encodes the Pit system associated with phosphate transport under high phosphate levels. However, no obvious difference in enrichment was detected for this promoter between aerobic and anaerobic conditions, suggesting that PolR positively regulates the expression of pit (MLP_RS00235) under both aerobic and anaerobic conditions for phosphorus retention in cells (Figure 7).
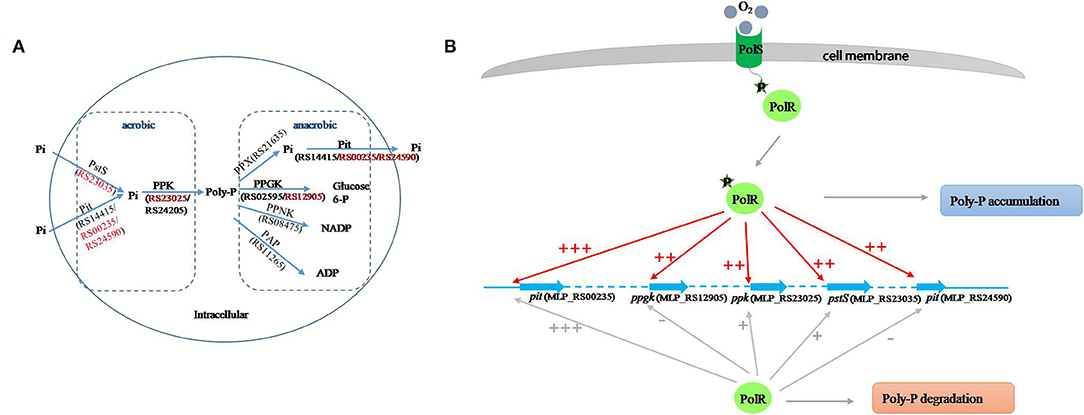
Figure 7. Proposed model of the PolS-PolR regulatory role in poly-P metabolism and Pi transport. (A) The diagram of poly-P metabolic pathway in M. phosphovorus NM-1 (He and McMahon, 2011; Kawakoshi et al., 2012). The locus tags and gene symbols related to the metabolism of Poly-P were annotated. The target genes of PolR were marked in red. (B) The putative mechanism of PolR regulating poly-P metabolism and Pi transport. The regulation of target genes by non-phosphorylated and phosphorylated PolR is indicated by gray lines and red lines, respectively. The relative degree of promoter binding is indicated by the number of “+,”and “–” indicates that PolR does not bind to the promoter.
In M. tuberculosis, the HK DevS/DosT are activated when the GAF domain senses hypoxia signals or oxygen, resulting in phosphorylation of DevR, which then binds to the promoters of the dormancy regulon and positively regulates the transcription of the associated genes. On the other hand, although GAF domains of DevS and DosT share 75% amino-acid sequence identity, they sense different signals (Kumar et al., 2007; Kaur et al., 2016; Lobao et al., 2019). Moreover, protein All4978 from Cyanobacterium Nostoc sp. PCC7120, containing GAF domain and a DNA binding motif, binds DNA preferentially when exposed to hypoxia (Tang et al., 2015). It is deduced that GAF domain could sense the different signals and work in different ways. In addition, FNR (E. coli K12) and WhiB3 (M. tuberculosis) both sense oxygen/NO by an iron-sulfur cluster [[4Fe−4S]]. While oxygen/NO inhibit the regulatory function of FNR but active that of WhiB3 (Green et al., 2014), suggesting that it has a possibility that regulators with similar sensing domain work in different ways. In this study, the binding of PolR to the ppgk (MLP_RS12905), ppk (MLP_RS23025), pstS (MLP_RS23035), and pit (MLP_RS24590) promoters declined in the aerobic-anaerobic shift, suggesting that hypoxic conditions repressed the phosphorylation of PolS and consequently reduced activation of PolR. We hypothesize that oxygen may be the signal sensed by PolS, although this hypothesis requires further study (Figure 7B).
Under aerobic growth conditions, the anabolism of poly-P is greater than its catabolism in M. phosphovorus, resulting in poly-P accumulation, which is opposite to the pattern of poly-P metabolism in strains cultured under anaerobic conditions. There are three ppk genes encoding PPK in the NM-1 strain, and these genes are responsible for the synthesis of poly-P from ATP (He and McMahon, 2011; Kawakoshi et al., 2012). According to our results with RT-qPCR (Lobao et al., 2019), genes related to the biosynthesis of poly-P, including ppk (MLP_RS23025) and ppk2 (MLP_RS24205), were significantly down-regulated under anaerobic conditions, while the transcription level of ppk2 (MLP_RS02760) showed no obvious changes under aerobic or anaerobic conditions (Figure 4B). PPNK (MLP_RS08475), PAP (MLP_RS11265), PPX (MLP_RS21635), and PPGK (MLP_RS02595, MLP_RS12905) are the enzymes that catalyze poly-P into NADP, ADP, Pi and glucose-6-phosphate, respectively (He and McMahon, 2011; Kawakoshi et al., 2012). Therefore, it is surprising that ppx (MLP_RS21635) and ppgk (MLP_RS12905), which are responsible for poly-P catabolism, were down-regulated under anaerobic conditions, whereas ppnk (MLP_RS08475) and ppgk (MLP_RS02595) were up-regulated (Figure 4B). We speculate that when strains are exposed to aerobic conditions, ppk (MLP_RS23025), and ppk2 (MLP_RS02760, MLP_RS24205) are involved in the synthesis of poly-P while ppx (MLP_RS21635) and ppgk (MLP_RS12905) mainly participate in poly-P degradation. When the growth conditions shift to an anaerobic stage, ppk (MLP_RS23025) and ppk2 (MLP_RS24205) are the major genes involved in poly-P biosynthesis, and ppnk (MLP_RS08475) and ppgk (MLP_RS02595) are the major genes involved in poly-P degradation. Of these genes, the PolS-PolR TCS identified in this study directly regulates ppk (MLP_RS23025) and ppgk (MLP_RS12905) and thereby contributes to regulating poly-P metabolism during the aerobic-anaerobic shift.
Except for poly-P accumulation in aerobic condition, PHA synthesis in anaerobic condition was considered as an important trait of PAOs (Mino, 2000). While in M. phosphovorus, PHA was produced in aerobic condition which is different from that in proteobacterial PAOs (Akar et al., 2006). PHA was likely to be synthesized by β-oxidation pathway and independent to the poly-P accumulation (Kawakoshi et al., 2012). The results of RNA-seq assay indicated that the transcription level of MLP_RS06230 which could be related to the PHA production was significantly down-regulated under anaerobic conditions compared to aerobic conditions in M. phosphovorus JN459 (Kawakoshi et al., 2012; Zhong et al., 2018a). It is deduced that the expression of MLP_RS06230 is also relevant to the dissolved oxygen level. While no conserved DNA binding sequence for PolR or similar sequence was found in the promoter of MLP_RS06230 based on the sequence alignment, thus we speculate that the PHA production is not directly regulated by PolR but other TCS or regulators.
The PhoB-PhoR TCS regulates phosphate transport in E. coli; PhoB is a positive regulator, which is activated by PhoR under low Pi concentrations and which induces the expression of the PstSCAB system and inhibits the Pit system (Lamarche et al., 2008; Lubin et al., 2016). PhoP in Streptomyces, the homologous protein of PhoB, actives the transcription of genes related to scavenging, transport of phosphate under phosphate depletion conditions (Martin et al., 2017). Our study indicated that the binding sites of PolR are highly similar with that of PhoP indentified in Streptomyces which is composed of direct repeat units of 11 nucleotides GtTCAccnnnn (Sola-Landa et al., 2008; Martin et al., 2017). A PhoB-PhoR TCS, encoded by MLP_RS11885 and MLP_RS11890, also exists in M. phosphovorus, suggesting that the PhoB-PhoR TCS could recognize the promoters of target genes of PolR and regulate phosphate transport and phosphate metabolism. It is very likely that PolR regulates the expressions of target genes responsed to the oxygen concentration while PhoP regulates the expressions of target genes responsed to the phosphate concentration. In addition, the MLP_RS22875-MLP_RS22880 TCS of M. phosphovorus is homologous to the SenX3-RegX3 TCS of M. tuberculosis, which regulates the PPK-encoding gene Rv2984 and thereby affects the synthesis of poly-P (Sanyal et al., 2013), suggesting that the expression of ppk may also be regulated by the MLP_RS22875-MLP_RS22880 TCS. We therefore anticipate that poly-P metabolism in strain NM-1 may be co-regulated by PolS-PolR, PhoB-PhoR and SenX3-RegX3.
In conclusion, M. phosphovorus strain NM-1 accumulates poly-P under aerobic conditions and degrades poly-P under anaerobic conditions. In this study, we identified the PolS-PolR TCS (MLP_RS10500 and MLP_RS10490), which shows high similarity with DevS-DevR of M. tuberculosis. We further demonstrated that PolR binds in vitro to the promoters of genes associated with phosphate metabolism, including pit (MLP_RS00235. MLP_RS24590), ppgk (MLP_RS12905), ppk (MLP_RS23025), and pstS (MLP_RS23035), and ChIP-qPCR revealed that PolR also binds to these promoters in vivo under aerobic conditions and suggested that the binding affinity was lower under anaerobic conditions. Transcriptional analyses showed that four of these genes, ppgk (MLP_RS12905), ppk (MLP_RS23025), pstS (MLP_RS23035), and pit (MLP_RS24590), were notably down-regulated when strains were cultivated under anaerobic conditions following aerobic growth. We also identified the consensus sequence GTTCACnnnnnGTTCaC as the conserved binding site of PolR. We speculate that PolS senses an oxygen signal and activates PolR, which directly induces the transcription of the poly-P catabolic gene ppgk (MLP_RS12905); the poly-P synthesis gene ppk (MLP_RS23025); the phosphate transport genes pit (MLP_RS00235, MLP_RS24590), and pstS (MLP_RS23035) to influence the metabolism of poly-P and phosphate transport.
Data Availability
All datasets generated for this study are included in the manuscript/Supplementary Files.
Author Contributions
GC designed the experiment. CZ performed the experiment. PZ prepared the manuscipt. CL and ML analyzed the data. WC, JF, and XQ revised the article.
Funding
This work was supported by the National Natural Science Foundation of China (No. 31500094), the Shandong Natural Science Foundation (No. ZR2015EM018), and the Innovation Project of the Shandong Academy of Medical Sciences.
Conflict of Interest Statement
The authors declare that the research was conducted in the absence of any commercial or financial relationships that could be construed as a potential conflict of interest.
Supplementary Material
The Supplementary Material for this article can be found online at: https://www.frontiersin.org/articles/10.3389/fmicb.2019.02127/full#supplementary-material
Figure S1. Specific binding of PolR to the promoter regions of target genes. The labelled promoters were incubated with 0 μg (lane 1) PolR, 1.0 μg (lane 2) PolR and 3.0 μg (lane 3-5) PolR. 150-fold excess of unlabelled specific probes (lane 4) and nonspecific probes (lane 5) were added in the reactions. Poly(dI-dC) (1.0 μg) was added to all samples as competitor.
Table S1. Primers used in this study.
Table S2. Information of genes related to Poly-P metabolism and phosphorus transport.
References
Akar, A., Akkaya, E. U., Yesiladali, S. K., Celikyilmaz, G., Cokgor, E. U., Tamerler, C., et al. (2006). Accumulation of polyhydroxyalkanoates by Microlunatus phosphovorus under various growth conditions. J. Ind. Microbiol. Biotechnol. 33, 215–220. doi: 10.1007/s10295-004-0201-2
Bailey, T. L., Johnson, J., Grant, C. E., and Noble, W. S. (2015). The MEME Suite. Nucleic Acids Res. 43, W39–W49. doi: 10.1093/nar/gkv416
Coats, E. R., Brinkman, C. K., and Lee, S. (2017). Characterizing and contrasting the microbial ecology of laboratory and full-scale EBPR systems cultured on synthetic and real wastewaters. Water Res. 108, 124–136. doi: 10.1016/j.watres.2016.10.069
Crocetti, G. R., Hugenholtz, P., Bond, P. L., Schuler, A., Keller, J., Jenkins, D., et al. (2000). Identification of polyphosphate-accumulating organisms and design of 16S rRNA-directed probes for their detection and quantitation. Appl. Environ. Microbiol. 66, 1175–1182. doi: 10.1128/AEM.66.3.1175-1182.2000
Fu, J., Qin, R., Zong, G., Liu, C., Kang, N., Zhong, C., et al. (2019). The CagRS two-component system regulates clavulanic acid metabolism via multiple pathways in streptomyces clavuligerus F613-1. Front. Microbiol. 10:244. doi: 10.3389/fmicb.2019.00244
Green, J., Rolfe, M. D., and Smith, L. J. (2014). Transcriptional regulation of bacterial virulence gene expression by molecular oxygen and nitric oxide. Virulence 5, 794–809. doi: 10.4161/viru.27794
He, S., and McMahon, K. D. (2011). Microbiology of ‘Candidatus Accumulibacter' in activated sludge. Microb. Biotechnol. 4, 603–619. doi: 10.1111/j.1751-7915.2011.00248.x
Kaur, K., Kumari, P., Sharma, S., Sehgal, S., and Tyagi, J. S. (2016). DevS/DosS sensor is bifunctional and its phosphatase activity precludes aerobic DevR/DosR regulon expression in Mycobacterium tuberculosis. FEBS J. 283, 2949–2962. doi: 10.1111/febs.13787
Kawakoshi, A., Nakazawa, H., Fukada, J., Sasagawa, M., Katano, Y., Nakamura, S., et al. (2012). Deciphering the genome of polyphosphate accumulating actinobacterium Microlunatus phosphovorus. DNA Res. 19, 383–394. doi: 10.1093/dnares/dss020
Kumar, A., Toledo, J. C., Patel, R. P., Lancaster, J. J., and Steyn, A. J. (2007). Mycobacterium tuberculosis DosS is a redox sensor and DosT is a hypoxia sensor. Proc. Natl. Acad. Sci. U.S.A. 104, 11568–11573. doi: 10.1073/pnas.0705054104
Lamarche, M. G., Wanner, B. L., Crepin, S., and Harel, J. (2008). The phosphate regulon and bacterial virulence: a regulatory network connecting phosphate homeostasis and pathogenesis. FEMS Microbiol. Rev. 32, 461–473. doi: 10.1111/j.1574-6976.2008.00101.x
Lobao, J., Gondim, A., Guimaraes, W. G., Gilles-Gonzalez, M. A., Lopes, L., and Sousa, E. (2019). Oxygen triggers signal transduction in the DevS (DosS) sensor of Mycobacterium tuberculosis by modulating the quaternary structure. FEBS J. 286, 479–494. doi: 10.1111/febs.14734
Lubin, E. A., Henry, J. T., Fiebig, A., Crosson, S., and Laub, M. T. (2016). Identification of the PhoB regulon and role of PhoU in the phosphate starvation response of Caulobacter crescentus. J. Bacteriol. 198, 187–200. doi: 10.1128/JB.00658-15
Madrona, Y., Waddling, C. A., and Ortiz, D. M. P. (2016). Crystal structures of the CO and NOBound DosS GAF-A domain and implications for DosS signaling in Mycobacterium tuberculosis. Arch. Biochem. Biophys. 612, 1–8. doi: 10.1016/j.abb.2016.10.005
Martin, J. F., Rodriguez-Garcia, A., and Liras, P. (2017). The master regulator PhoP coordinates phosphate and nitrogen metabolism, respiration, cell differentiation and antibiotic biosynthesis: comparison in Streptomyces coelicolor and Streptomyces avermitilis. J. Antibiot. 70, 534–541. doi: 10.1038/ja.2017.19
Mino, T. (2000). Microbial selection of polyphosphate-accumulating bacteria in activated sludge wastewater treatment processes for enhanced biological phosphate removal. Biochem. Mosc. 65, 341–348.
Nakamura, K., Hiraishi, A., Yoshimi, Y., Kawaharasaki, M., Masuda, K., and Kamagata, Y. (1995). Microlunatus phosphovorus gen. nov., sp. nov., a new gram-positive polyphosphate-accumulating bacterium isolated from activated sludge. Int. J. Syst. Bacteriol. 45, 17–22. doi: 10.1099/00207713-45-1-17
Novichkov, P. S., Rodionov, D. A., Stavrovskaya, E. D., Novichkova, E. S., Kazakov, A. E., Gelfand, M. S., et al. (2010). RegPredict: an integrated system for regulon inference in prokaryotes by comparative genomics approach. Nucleic Acids Res. 38, W299–W307. doi: 10.1093/nar/gkq531
Sanyal, S., Banerjee, S. K., Banerjee, R., Mukhopadhyay, J., and Kundu, M. (2013). Polyphosphate kinase 1, a central node in the stress response network of Mycobacterium tuberculosis, connects the two-component systems MprAB and SenX3-RegX3 and the extracytoplasmic function sigma factor, sigma E. Microbiol. Soc. 159, 2074–2086. doi: 10.1099/mic.0.068452-0
Sola-Landa, A., Rodriguez-Garcia, A., Apel, A. K., and Martin, J. F. (2008). Target genes and structure of the direct repeats in the DNA-binding sequences of the response regulator PhoP in Streptomyces coelicolor. Nucleic Acids Res. 36, 1358–1368. doi: 10.1093/nar/gkm1150
Sousa, E., Gonzalez, G., and Gilles-Gonzalez, M. A. (2017). Target DNA stabilizes Mycobacterium tuberculosis DevR/DosR phosphorylation by the full-length oxygen sensors DevS/DosS and DosT. FEBS J. 284, 3954–3967. doi: 10.1111/febs.14284
Strokal, M., Yang, H., Zhang, Y., Kroeze, C., Li, L., Luan, S., et al. (2014). Increasing eutrophication in the coastal seas of China from 1970 to 2050. Mar. Pollut. Bull. 85, 123–140. doi: 10.1016/j.marpolbul.2014.06.011
Tanaka, S., Lee, S. O., Hamaoka, K., Kato, J., Takiguchi, N., Nakamura, K., et al. (2003). Strictly polyphosphate-dependent glucokinase in a polyphosphate-accumulating bacterium, Microlunatus phosphovorus. J. Bacteriol. 185, 5654–5656. doi: 10.1128/JB.185.18.5654-5656.2003
Tang, K., Knipp, M., Liu, B. B., Cox, N., Stabel, R., He, Q., et al. (2015). Redox-dependent Ligand Switching in a Sensory Heme-binding GAF Domain of the Cyanobacterium Nostoc sp. PCC7120. J. Biol. Chem. 290, 19067–19080. doi: 10.1074/jbc.M115.654087
Tarayre, C., Nguyen, H. T., Brognaux, A., Delepierre, A., De Clercq, L., Charlier, R., et al. (2016). Characterisation of phosphate accumulating organisms and techniques for polyphosphate detection: a review. Sensors 16:797. doi: 10.3390/s16060797
Tsai, C. S., and Liu, W. T. (2002). Phylogenetic and physiological diversity of tetrad-forming organisms in deteriorated biological phosphorus removal systems. Water Sci. Technol. 46, 179–184. doi: 10.2166/wst.2002.0474
Tu, Y., and Schuler, A. J. (2013). Low acetate concentrations favor polyphosphate-accumulating organisms over glycogen-accumulating organisms in enhanced biological phosphorus removal from wastewater. Environ. Sci. Technol. 47, 3816–3824. doi: 10.1021/es304846s
Wang, Y., Cen, X. F., Zhao, G. P., and Wang, J. (2012). Characterization of a new GlnR binding box in the promoter of amtB in Streptomyces coelicolor inferred a PhoP/GlnR competitive binding mechanism for transcriptional regulation of amtB. J. Bacteriol. 194, 5237–5244. doi: 10.1128/JB.00989-12
Wang, Z., Gao, M., She, Z., Jin, C., Zhao, Y., Yang, S., et al. (2015). Effects of hexavalent chromium on performance and microbial community of an aerobic granular sequencing batch reactor. Environ. Sci. Pollut. Res. Int. 22, 4575–4586. doi: 10.1007/s11356-014-3704-z
Winkler, M., Coats, E. R., and Brinkman, C. K. (2011). Advancing post-anoxic denitrification for biological nutrient removal. Water Res. 45, 6119–6130. doi: 10.1016/j.watres.2011.09.006
Zhang, L., Loaiciga, H. A., Xu, M., Du, C., and Du, Y. (2015). Kinetics and mechanisms of phosphorus adsorption in soils from diverse ecological zones in the source area of a drinking-water reservoir. Int. J. Environ. Res. Public Health 12, 14312–14326. doi: 10.3390/ijerph121114312
Zhang, P., Wu, H., Chen, X. L., Deng, Z., Bai, L., and Pang, X. (2014). Regulation of the biosynthesis of thiopeptide antibiotic cyclothiazomycin by the transcriptional regulator SHJG8833 in Streptomyces hygroscopicus 5008. Microbiology 160, 1379–1392. doi: 10.1099/mic.0.076901-0
Zhong, C., Fu, J., Jiang, T., Zhang, C., and Cao, G. (2018a). Polyphosphate metabolic gene expression analyses reveal mechanisms of phosphorus accumulation and release in Microlunatus phosphovorus strain JN459. Fems. Microbiol. Lett. 365:fny034. doi: 10.1093/femsle/fny034
Zhong, C., Wang, J., Zong, G., Jiang, T., and Cao, G. (2018b). Heterologous expression of Microlunatus phosphovorus regulatory protein Mlp21700 and its binding to promoters of Poly-P metabolic genes in vitro (in Chinese). Microbiology China 45, 780–787. doi: 10.13344/j.microbiol.china.170344
Zhou, Y., Pijuan, M., Oehmen, A., and Yuan, Z. (2010). The source of reducing power in the anaerobic metabolism of polyphosphate accumulating organisms (PAOs) - a mini-review. Water Sci. Technol. 61, 1653–1662. doi: 10.2166/wst.2010.983
Keywords: polyphosphate metabolism, two-component system, gene regulation, Microlunatus phosphovorus, phosphate transport
Citation: Zhong C, Zhang P, Liu C, Liu M, Chen W, Fu J, Qi X and Cao G (2019) The PolS-PolR Two-Component System Regulates Genes Involved in Poly-P Metabolism and Phosphate Transport in Microlunatus phosphovorus. Front. Microbiol. 10:2127. doi: 10.3389/fmicb.2019.02127
Received: 23 May 2019; Accepted: 29 August 2019;
Published: 13 September 2019.
Edited by:
Satoshi Tsuneda, Waseda University, JapanReviewed by:
Antonio Rodríguez-García, Instituto de Biotecnología de León, SpainZ. Petek Cakar, Istanbul Technical University, Turkey
Copyright © 2019 Zhong, Zhang, Liu, Liu, Chen, Fu, Qi and Cao. This is an open-access article distributed under the terms of the Creative Commons Attribution License (CC BY). The use, distribution or reproduction in other forums is permitted, provided the original author(s) and the copyright owner(s) are credited and that the original publication in this journal is cited, in accordance with accepted academic practice. No use, distribution or reproduction is permitted which does not comply with these terms.
*Correspondence: Guangxiang Cao, caozhong0402@163.com
†These authors have contributed equally to this work