- 1Laboratorio de Investigación en Bacteriología Intestinal, Hospital Infantil de México “Federico Gómez”, Mexico City, Mexico
- 2Laboratorio de Inmunidad en Mucosas, Unidad de Biomedicina, Facultad de Estudios Superiores Iztacala, Universidad Nacional Autónoma de México, Tlalnepantla, Mexico
- 3Departamento de Patología, Hospital Infantil de México “Federico Gómez”, Mexico City, Mexico
Curli, a type of fimbriae widely distributed in uropathogenic Escherichia coli (UPEC), are involved in adhesion to human bladder cell surfaces and biofilm development. The role of UPEC curli was evaluated in a murine model of urinary tract infection. The aim of this study was to establish the role of curli in C57BL/6 mice transurethrally infected with curli-producing and non-curli-producing UPEC strains. We confirmed that curli enhanced UPEC colonization in the urinary tract, resulting in damage to both the bladder and kidney. Intranasal immunization with recombinant CsgA protein protected against colonization by curli-producing UPEC in the urinary tract. Quantification of cytokines from urinary tract organs showed increases in interleukin-6 and tumor necrosis factor (TNF) release in the kidneys 48 h postinfection with curli-producing UPEC. By contrast, mice infected with non-curli-producing UPEC showed the highest release of interleukin-6, -10, and -17A and TNF. Curli may obscure other fimbriae and LPS, preventing interactions with Toll-like receptors. When intranasal immunization with recombinant FimH and PapG proteins and subsequent infection with this strain were performed, cytokine quantification showed a decrease in the stimulation and release by the uroepithelium. Thus, curli are amyloid-like fimbriae that enhances colonization in the urinary tract and a possible fitness factor.
Introduction
Curli fimbriae are classified as amyloid fibers and are involved in surface adhesion, cell aggregation, and biofilm formation in Escherichia coli and other Enterobacteriaceae (Kikuchi et al., 2005). Curli facilitate bacterial binding with the extracellular matrix and various serum proteins, such as fibronectin, laminin, plasminogen, and plasminogen activator protein (Barnhart and Chapman, 2006). Subunits of curli and their assembly machinery are encoded on two divergently transcribed operons; the csgBAC operon encodes the major curli subunit CsgA, which is responsible for interaction with the host components (Hufnagel et al., 2015).
Urinary tract infections (UTIs) are one of the most important human infections and are mainly caused by uropathogenic E. coli (UPEC) (Flores-Mireles et al., 2015). In Mexico, UPEC-associated UTIs are the second leading cause of morbidity, with more than four million cases annually (Luna-Pineda et al., 2018a). Furthermore, UPEC clinical isolates have been shown to promote curli expression and biofilm formation at 26°C and in low nutritional conditions (Lim et al., 2014). In addition, curli expression at 37°C has been described in urinary E. coli isolated from patients with bacteremia (Hung et al., 2014). Curli expression was found in 74 and 89% of UPEC isolates from women with cystitis and upper urinary tract symptoms, respectively (Norinder et al., 2012), and these fimbriae were expressed in 56–60% of UPEC isolates from children with cystitis and pyelonephritis (Kudinha et al., 2013). Recently, we described a high frequency (≥95%) of the csgA gene in multidrug-resistant and extensively drug-resistant UPEC clinical strains obtained from pediatric patients with uncomplicated and complicated UTIs (Ochoa et al., 2016; Luna-Pineda et al., 2018b).
Curli-producing UPEC can induce interleukin (IL)-8 release in renal epithelial cells and can interact with antimicrobial peptides LL-37 without affecting bacterial colonization in the kidney (Kai-Larsen et al., 2010). Dimeric and trimeric recombinant proteins generated by our workgroup that included CsgA promoted IL-6 and IL-8 release from the supernatant of bladder HTB-5 cells. The antibodies generated against these recombinant proteins protected against UPEC adherence to HTB-5 cells (Luna-Pineda et al., 2016). The FN075 molecule is an inhibitor of CsgA-formed amyloid fiber and has the ability to attenuate UPEC virulence in a murine model of UTI (Cegelski et al., 2009). The curli fimbriae are associated with biofilm-like structures produced by UPEC and are related to the persistence of this bacterium in the urinary tract (Anderson et al., 2003; Trautner and Darouiche, 2004). Interestingly, curli are associated with adhesion in vitro, which is enhanced by phosphoethanolamine cellulose (Cordeiro et al., 2016; Hollenbeck et al., 2018). Based on this finding, the curli of UPEC could contribute to colonization by attach to cell surface and modifying cytokine release in vivo. This study evaluated the role of curli in a murine model of UTI using curli-producing and non-curli-producing UPEC strains.
Materials and Methods
Mutation and Phenotypic Complementation of Uropathogenic Escherichia coli
The UPEC clinical strain 529U-0712 was evaluated by our workgroup, beginning with 500 isolates from pediatric patients with UTI (Ochoa et al., 2016). The inclusion criteria were (1) amplification of the csgA gene, (2) curli expression, (3) cellulose expression, and (4) antibiotic sensitivity (gentamicin, ampicillin, kanamycin, and chloramphenicol). The csgA gene was inactivated using the Datsenko and Wanner method (Datsenko and Wanner, 2000). Briefly, the inactivation cassette was amplified using the pKD3 vector and specific primers (mCsgA_For 5′-GTTTTACATGAAACTTTTAAAAGTAG CAGCAATTGCAGCAATCGTATTCGTGTAGGCTGGAGCTGCTTC-3′ and mCsgA_Rev 5′-GCGCCCTGTTTCTTTCATACTGATGA TGTATTAGTACTGATGAGCGGTCGCATATGAATATCCTCCTTAG-3′), which contained the gene encoding chloramphenicol resistance. The putative transformative strains were verified by polymerase chain reaction (PCR) using the following specific primers: vCsgA_For 5′-GCCAGTATTTCGCAA GGTGC-3′ and vCsgA_Rev 5′-GGTGTACATATCCCCTTGCTGG-3′. An amplicon of approximately 1,400 base pairs (bp) and the absence of Congo red dye fixation were considered positive indicators of mutation. Mutants were confirmed by transmission electron microscopy (TEM), and phenotype complementation was generated using the pCsgGC vector. The pJcsgGC vector contained the sequences of the csgGFED and csgBAC operons cloned into the plasmid pJET1.2 blunt (Thermo Fisher Scientific, MA, USA) using the following specific primers: CsgG_For 5′-GCGAGCTCGGTTGATATTTGGTTACGC-3′ and CsgC_Rev 5′-CTCTCTTATGCTCGGCAGTTGAGCTC-3′.
Adhesion and Invasion Assays of a Bladder and Renal Cell Lines
The human bladder cell line HTB-5 and human renal cell line HTB-47 acquired from the American Type Culture Collection (ATCC, VA, USA) was grown in Eagle’s minimum essential medium (EMEM) from ATCC. Twenty-four-well plates were prepared with 1 × 105 cells/well in 1 ml of EMEM supplemented with 10% fetal bovine serum (Gibco, Thermo Fisher Scientific, MA, USA) until the cells reached 80% confluence. Previously, the UPEC strain was cultured in conditions to promote curli expression and adjusted to an OD600 of 1. Cells were treated with 2.5% mannose (Sigma-Aldrich Corp, MO, USA) and incubated for 1 h at 37°C under agitation. Cell monolayers were infected with 1 × 107 bacteria (MOI of 100) and incubated for 3 h at 37°C with 5% CO2. The number of colony forming units (CFU) was determined by three methods: (1) after infection, the monolayers were treated with 20 μl of 0.5% Triton X-100 in phosphate buffer solution (PBS) and collected for quantification of total bacteria (not adhered, adhered and intracellular); (2) the infected monolayers were washed three times with 1 ml of PBS, followed by treatment with 1 ml of PBS with 0.1% Triton X-100 and collected for the quantification of adhered and intracellular bacteria; and (3) the infected monolayers were washed three times with 1 ml of PBS, incubated with 1 ml of EMEM with 100 μg/ml of gentamicin for 2 h to remove the adhering bacteria and washed three times with PBS. Finally, the treated monolayers were collected with 1 ml of PBS with 0.1% Triton X-100 for quantification of the intracellular bacteria. Quantification of CFU/mL was performed by the microdilution method described by Hannan and Hunstad (Hannan and Hunstad, 2016). EMEM was used as a negative control in these assays, and the wild-type strain was considered to show 100% adherence-invasion.
Murine Model of Urinary Tract Infection
C57BL/6 female mice at 10–12 weeks were obtained from the “Facultad de Estudios Superiores-Iztacala” of UNAM. The UPEC strain was cultured as described above, and groups of eight mice were inoculated transurethrally with 1 × 108 bacteria in 100 μl of PBS, according to the modified protocol of Hung et al. (2009). Forty-eight hours after infection, the mice were sacrificed to obtain the bladder and the kidneys, which were mechanically homogenized in 1 ml of PBS with 0.05% Triton X-100 (Sigma-Aldrich Corp., Mo, USA). Quantification of CFU/organ was performed as described above. PBS was used as a negative control in this assay, and the wild-type strain was considered to show 100% colonization.
Histology and Microscopy
Three C57BL/6 mice per group were infected and sacrificed as described above. Bladders and kidneys were obtained and fixed with 10% paraformaldehyde for hematoxylin-eosin staining. Tissue samples were embedded in paraffin, stained with hematoxylin, and sectioned by the “Departamento de Patología” of “Hospital Infantil de México Federico Gómez” (CDMX, México).
The bladder lumen is delimited by a stratified transitional epithelium of three or four cell layers, commonly termed the urothelium, and the epithelial cells are organized into the basement membrane and the lamina propria. The bladder urothelium comprises basal and intermediate epithelial cells underlying a layer of binucleate superficial facet cells (Hung et al., 2009). Cystitis scores were determined as follows: 0 = no significant lesions, 1 = few and occasional PMN cells in the stroma or lumen, as well as occasional perivascular lymphoid infiltration; 2 = presence of PMN cells and moderate edema; 3 = many PMN cells and severe edema. The kidneys basically consist of a capsule formed by connective tissue, a cortex including the renal corpuscles of the nephrons, the medulla above a striated zone (renal pyramid), and a collecting structure (calyx) near the renal pelvis and ureters (Cardiff et al., 2014). Pyelonephritis scores were determined as follows: 0 = no significant lesions; 1 = few and occasional PMN cells in the renal pelvis; 2 = rafts and/or scattered focal aggregates of PMN cells in the renal pelvis, as well as peripelvic inflammation; 3 = presence of numerous and large focal PMN cells in all tissue sections and inflammation in the parenchyma.
Curli-Induced Cytokine Release in the Urinary Tract
Five C57BL/6 mice per group were infected and sacrificed as described above. The bladders were obtained and weighed, sectioned five times, and sonicated in 500 μl of PBS with a pulse of 10 s and 70% amplitude (ultrasonic processor; Cole-Parmer; IL; US). Both kidneys were also obtained and weighed, and they were sectioned 10 times and sonicated in 500 μl of PBS with three pulses of 10 s and 70% amplitude. In both cases, they were supplemented with cOmplete™, Mini Protease Inhibitor Cocktail (Merck KGaA, Darmstadt, Germany). The organ lysates were centrifuged for 10 min at 4,000 rpm, and the supernatants were divided into aliquots in microtubes and stored at −70°C until use. The cytokines included in this study were IL-2, IL-4, IL-6, IL-10, IL-17, IFN-γ, and tumor necrosis factor (TNF). The cytokine release was quantified using a BD Cytometric Bead Array (CBA) Mouse Th1/Th2/Th17 Cytokine Kit (Becton, Dickinson Company, BD Biosciences, CA, USA) and a BD FACSCalibur™ flow cytometer. PBS was used as a control of cytokine basal release in this assay, and the wild-type strain was considered to show 100% cytokine release in a UTI. Data analysis was executed with FCAP Array software version 3.0 of BD Biosciences and FlowJo software version 7.6 (FlowJo, LLC, OR, USA).
Intranasal Immunization Scheme
C57BL/6 female mice at 10–12 weeks were immunized intranasally with CsgA, PapG, and FimH recombinant proteins. The first immunization was carried out with 100 μg of protein in 40 μl (20 μl in each nostril) and two boosts with 50 μg of protein in the same volume (days 7 and 14). The urine samples were recollected (days 18-20) and antibodies identified by Enzyme-Linked ImmunoSorbent Assay. On day 21, the challenge was performed as described above (murine model of UTI), using 108 bacteria in 100 μl of PBS. On day 23, the immunized and infected mice were sacrificed, and the organs were obtained for later use.
Statistical Analysis
Student’s t-test was used to compare differences between means, two-way ANOVA was used to compare means among multiple groups, and the Mann-Whitney U-test was used to compare medians. The analysis and graphics were performed using GraphPad Prism software (version 7). A p value <0.05 was considered statistically significant for all results.
Ethics Statement
The study was reviewed and approved by the Research Committee (Dr. Juan Garduño Espinosa), Ethics Committee (Dr. Luis Jasso Gutierrez), and Biosecurity Committee (Dr. Marcela Salazar Garcia) of “Hospital Infantil de México Federico Gómez” (HIMFG), with permit numbers HIM/2016/099 SSA.1329, HIM/2017/002 SSA.1298, HIM/2017/136 SSA.1456, and HIM 2017-107FF SSA.1421. The UPEC clinical strains were isolated from pediatric patients with UTIs and were obtained from a pre-existing collection (Ochoa et al., 2016). The aforementioned committees provided approval to use the samples from the pre-existing collection, and all samples were anonymized. The physicians from the Infectology Department asked the patients for their permission and consent to use the collected samples employed in this study. Animal assays were performed according to the specifications of the Mexican official standard NOM-62-ZOO-199, which includes technical specifications for the production, care and use of laboratory animals.1 Animal assays included a murine model of UTI, mouse IN immunizations, histology sections, and cytokine quantification from mouse bladders and kidneys.
Data Availability
The datasets generated and/or analyzed during the current study are available from the corresponding author on reasonable request. Additionally, all datasets are available in the repository via the following web link: https://github.com/vlunapineda/Frontiers-in-Cell-and-Infect-Microb.
Results
The UPEC clinical strain 529U-0712 (wild type) was selected based on the presence of the csgA gene, Congo red fixing, antibiotic resistance (ampicillin, chloramphenicol, and kanamycin), and curli expression at 37°C (Supplementary Table S1). This strain was used to generate an isogenic mutant in the csgA gene (csgA::Cm), which was restored through complementation with the plasmid pCsgG-C (complemented strain). The genotypic verification of the mutation was carried out by PCR. Amplicons of 788 bp for the wild-type strain, 1,400 bp for the mutant strain, and 788 bp for the complemented strain were obtained (Supplementary Figure S1). A wild-type strain grown on yeast extract and casamino acid (YESCA) agar supplemented with Congo red showed a high level of fixation of this dye; however, loss of retention was observed in the mutant strain, and fixation restoration was observed in the complemented strain (Supplementary Figure S1). Curli are considered an amyloid-like structure and are characterized as thin fimbria that form bunches similar to curls. The negative-staining micrographs by TEM showed these fine structures in the wild-type and complemented strains, while a loss of these structures was observed in the mutant strain (Supplementary Figure S1).
To confirm the role of curli in adherence, we performed adhesion and invasion assays on human bladder (HTB-5) and renal (HTB-47) cells. The quantification of CFU showed an adhesion mean of 3.5 × 106 CFU/ml for the wild-type strain and a decreased adhesion mean of 7.3 × 105 CFU/ml for the mutant strain to bladder cells. According to the statistical analysis, the decrease in adherence produced a significant change of 79.1% (p < 0.0001), including the complemented strain with p < 0.0001 compared with the mutant strain (Figure 1). The quantitative analysis of adhesion to renal cells showed an adhesion mean of 2.7 × 105 CFU/ml for the wild-type strain and an adhesion mean of 2.1 × 105 CFU/ml for the mutant strain; this difference was not statistically significant, nor was the statistical difference between either and the adhesion mean of the complement strain (Figure 1). Additionally, the testing of bacterial invasion of the bladder HTB-5 and HTB-47 cells using mannose (blocking type 1 fimbriae) and gentamicin treatment (removing attached bacteria) did not show the growth of intracellular bacteria (data not shown).
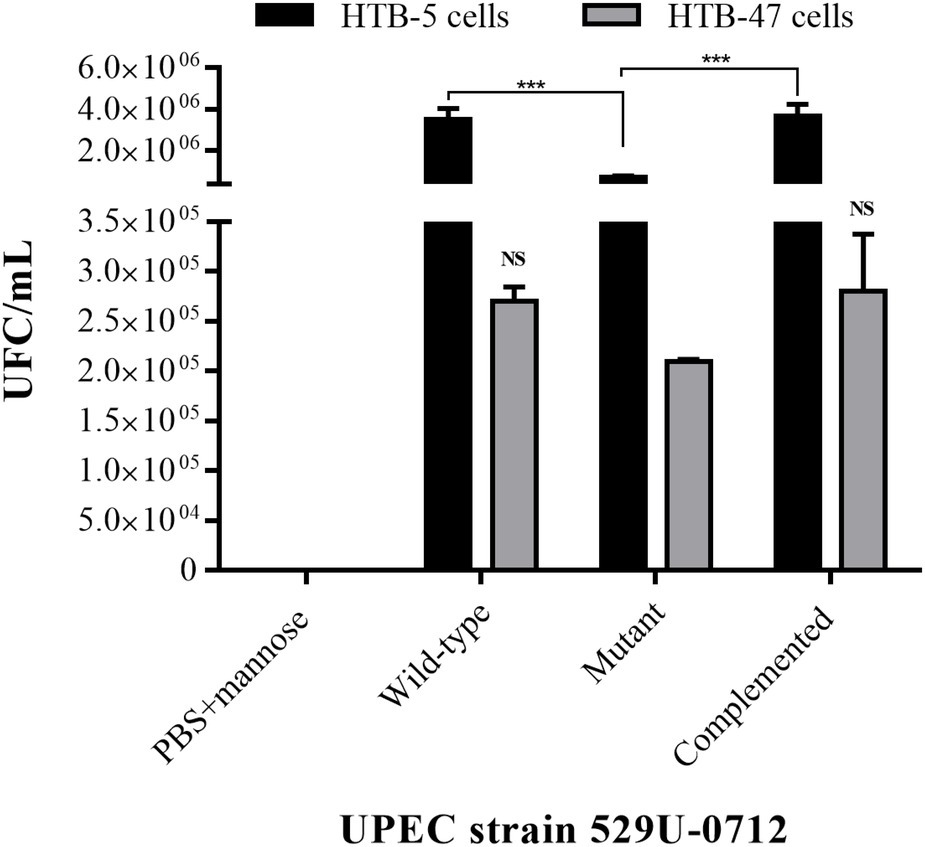
Figure 1. Assays of adherence to bladder HTB-5 cells and renal HTB-47 cells. The wild-type and complemented strains showed similar adhesion profiles, and a significant reduction (p < 0.001) in the mutant strain was observed in bladder cells. In renal cells, no significant differences were identified among all UPEC strains. Quantification of CFU/ml was performed by a plaque microdilution method. The bars represent the mean ± S. E. of three independent experiments. Uninfected cells were used as a control in this experiment. NS = not significant (p > 0.5); *** < 0.001.
Curli-Producing Uropathogenic Escherichia coli Efficiently Colonizes the Mouse Urinary Tract
The role of curli UPEC in the urinary tract was evaluated using a murine model of UTI in C57BL/6 female mice at 10–12 weeks of age. Bacterial quantification in the infected mouse bladder showed a significant decrease (p = 0.0078), with a median of 6.66 × 106 CFU/ml for the mutant strain compared to a median of 4 × 107 CFU/ml for the wild-type strain and a median of 2.33 × 107 CFU/ml for the complemented strain (p = 0.0012; Figure 2). Under the same conditions, the bacterial quantification in infected mouse kidneys showed a significant reduction (p = 0.0078), with a median of 7.33 × 104 CFU/ml for the mutant strain compared to a median of 1.08 × 106 CFU/ml for the wild-type strain and a median of 1.25 × 106 CFU/ml for the complemented strain (p = 0.0054; Figure 3).
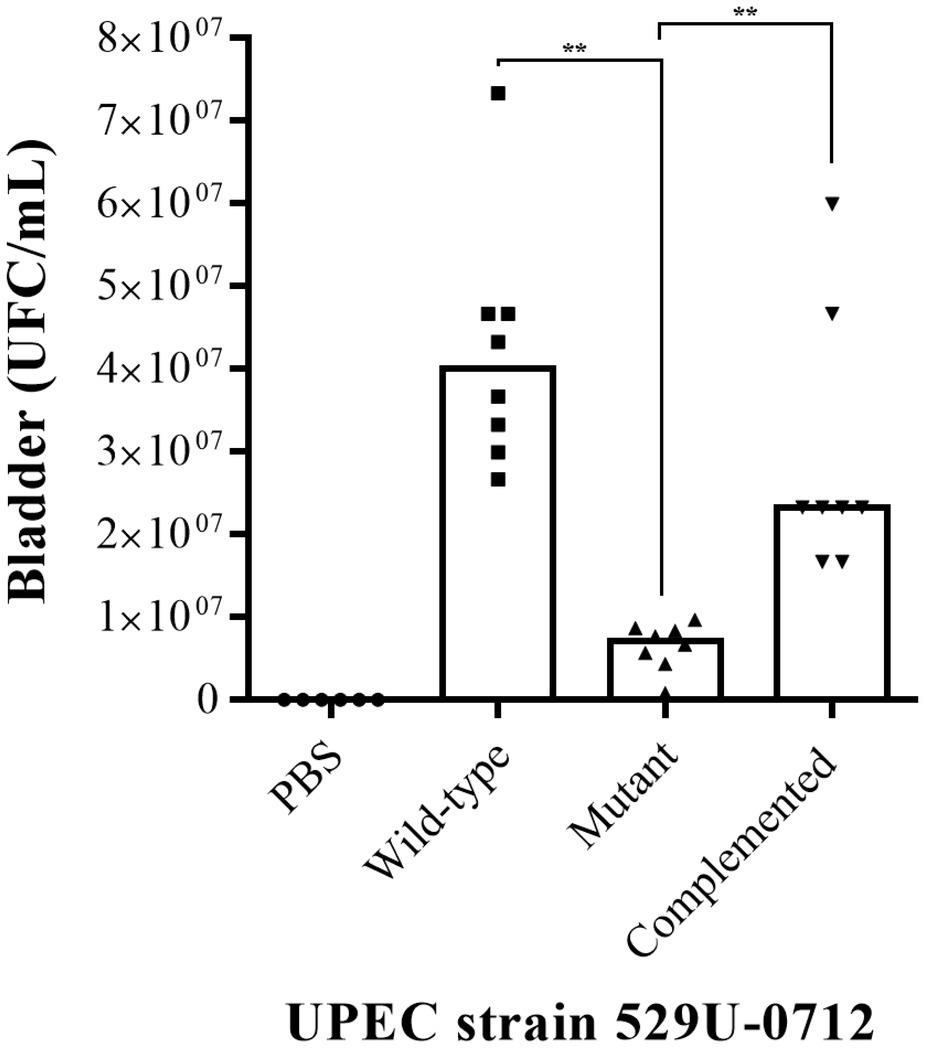
Figure 2. Evaluation of adherence in UPEC strain-infected mouse bladders. The murine model of UTI was generated in female C57BL/6 mice at 10–12 weeks with a transurethral inoculation of 1 × 108 bacteria in 100 μl. Forty-eight hours postinfection, the mice were sacrificed to obtain the bladders, which were homogenized in PBS, and bacteria per organ (CFU/ml) were quantified by a plaque microdilution method. The UPEC clinical strain 529U-0712 was defined as the wild-type strain, the UPEC strain 529U-0712 csgA::Cm was defined as the mutant strain, and complementation with the plasmid pJcsgG-C resulted in the complemented strain. Transurethral inoculation with PBS was used as a control in this experiment. ** = 0.01-0.001.
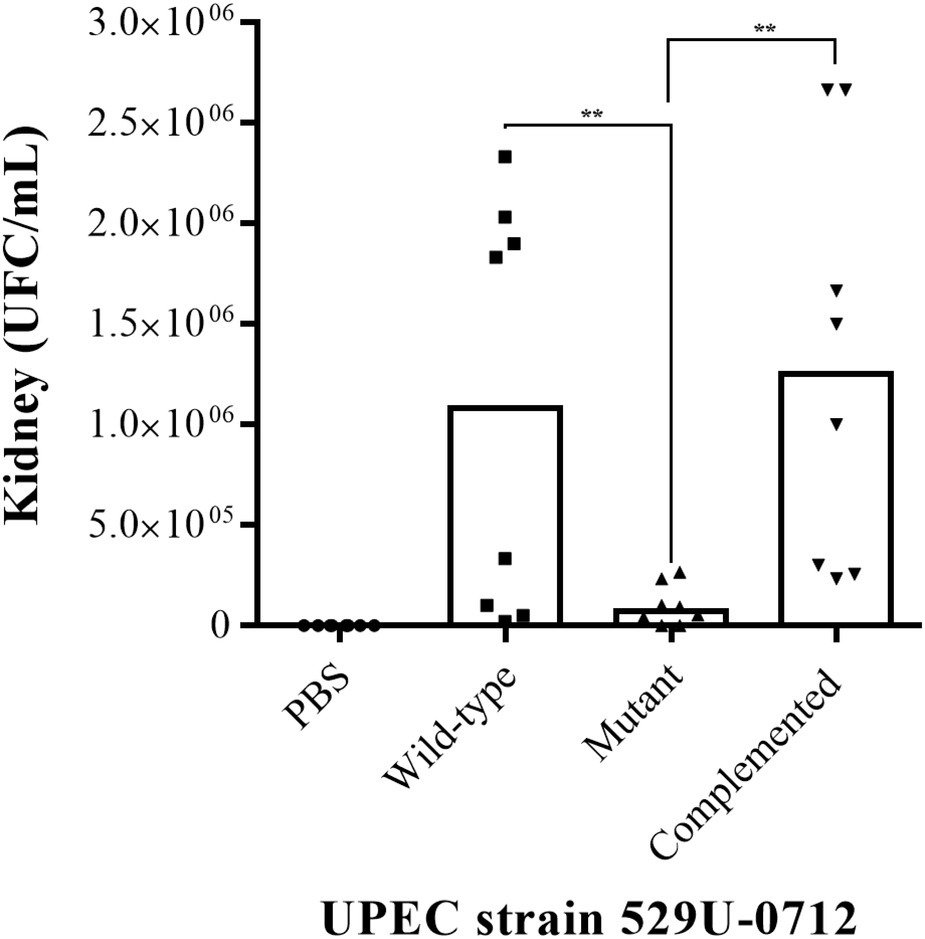
Figure 3. Evaluation of adherence in UPEC strain-infected mouse kidneys. The murine model of UTI was generated in female C57BL/6 mice at 10–12 weeks with a transurethral inoculation of 1 × 108 bacteria in 100 μl. Forty-eight hours postinfection, the mice were sacrificed to obtain the kidneys, which were homogenized in PBS, and bacteria per organ (CFU/ml) were quantified by the plaque microdilution method. The UPEC clinical strain 529U-0712 was defined as the wild-type strain, the UPEC strain 529U-0712 csgA::Cm was defined as the mutant strain, and complementation with the plasmid pJcsgG-C resulted in the complemented strain. Transurethral inoculation with PBS was used as a control in this experiment. ** = 0.01-0.001.
Histological sections of mouse bladder and kidneys were stained with hematoxylin-eosin and visualized under light microscopy. In the bladder, the adhered wild-type strain was visualized on the surface of the uroepithelium, and invading bacteria were also observed 48 h postinfection (Figure 4). Additionally, the mutant strain was localized in the bladder lumen, with low-adherence and low-invasion bacteria on the uroepithelium (Figure 4). In the kidney, the UPEC wild-type strain was visualized in the renal pelvis, and low bacteria levels were observed in the renal tubules, while the mutant strain was slightly visible in the renal pelvis and barely detectable in the tubules (Figure 4). Curli expression in vivo was determined by Western blotting (WB) using sonicated tissues at 48 h postinfection with collected UPEC strains and anti-CsgA antibodies (Supplementary Figure S2).
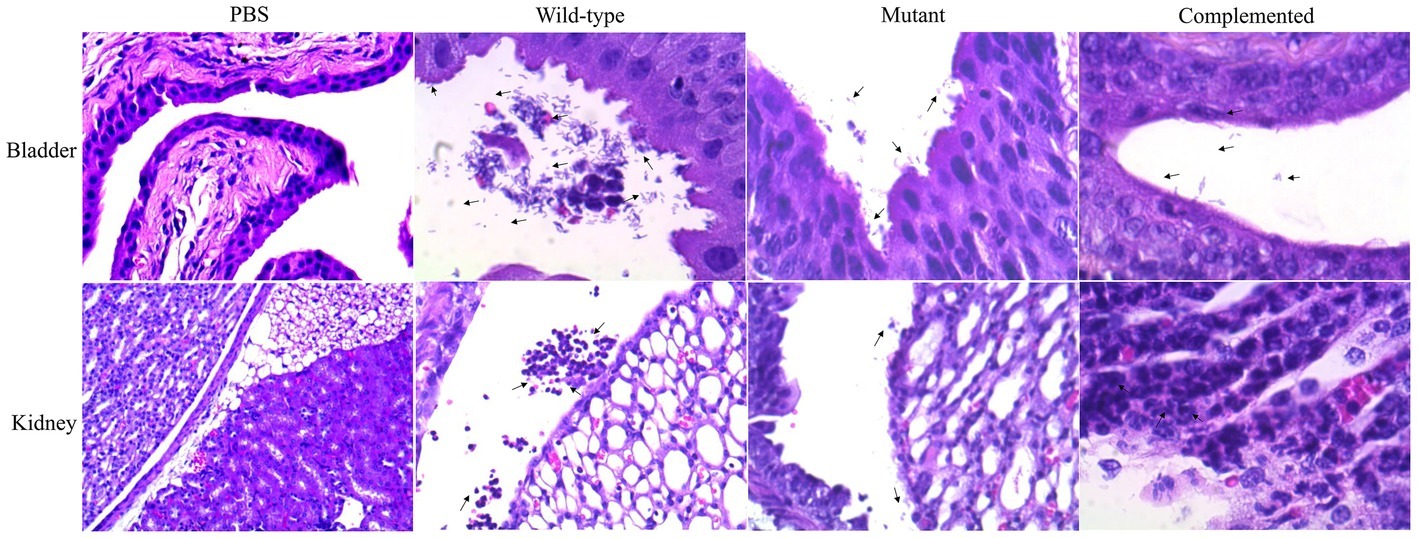
Figure 4. Visualization of UPEC in the bladder and kidney sections. Tissue samples were embedded in paraffin, sectioned, stained with hematoxylin-eosin, and visualized. Black arrows show the presence of bacteria as follows: PBS (control), wild-type strain (UPEC strain 529U-0712), mutant strain (csgA::Cm), and complemented strain (plasmid pJcsgG-C).
The CsgA Structural Protein of Curli Protects Against Colonization of Uropathogenic Escherichia coli in Immunized Mice
Recombinant CsgA (rCsgA) protein was obtained in a previous study (Luna-Pineda et al., 2016). C57BL/6 female mice were immunized intranasally with rCsgA protein and later infected transurethrally with the UPEC strain 529U-0712. Bacterial quantification of UPEC in the total bladder showed a significant reduction (p = 0.0003), with an arithmetical median of 2.7 × 106 CFU/ml for rCsgA protein and 1.9 × 107 CFU/ml for PBS immunization (Figure 5). Nevertheless, the quantification in both kidneys showed arithmetical medians of 5.9 × 105 UFC/ml for PBS immunization and 5.2 × 104 UFC/ml for rCsgA immunization with no significant difference (Figure 5). Interestingly, anti-rCsgA antibodies were identified from the urine following IN immunization with rCsgA to C57BL/6 mice (Supplementary Figure S3). These data showed protection against UPEC and confirmed that curli played a role in bacterial colonization in the urinary tract.
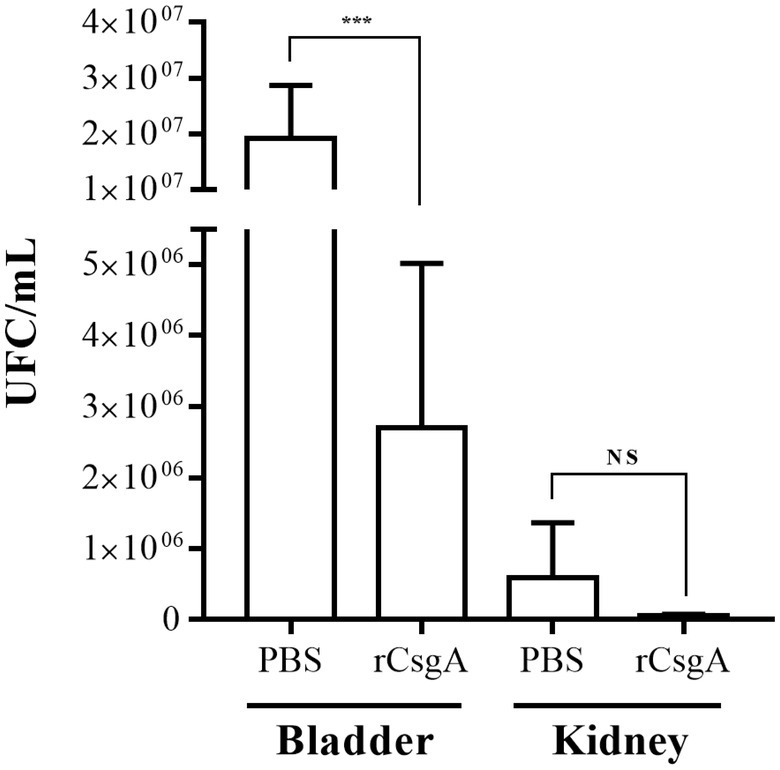
Figure 5. Recombinant CsgA protein immunization generates protection against colonization of UPEC. C57BL/6 female mice at 10–12 weeks were immunized intranasally. The first immunization was carried out with 100 μg of rCsgA protein in 40 μl and two boosts with 50 μg of protein in the same volume. The challenge was performed, and CFU/ml quantification was performed in both infected mouse bladders and kidneys. NS = not significant (p > 0.5); *** < 0.001.
Curli-Producing Uropathogenic Escherichia coli Generate Damage and an Inflammatory Process in the Mouse Urinary Tract
Histological sections of mouse bladder after infection with wild-type UPEC strains visualized by light microscopy showed cystitis with a score of 3. The micrographs showed the presence of polymorphonuclear (PMN) infiltration in the perivascular submucosa and intraepithelial regions and a PMN cumulus in the lumen bladder with strong edema and cellular exfoliation (Figure 6). Cystitis (score = 1) in the mutant group was less severe than that in the wild-type group and showed moderate perivascular PMN infiltration (Figure 6). In the mouse kidney, pyelonephritis with a score of 3 was visualized for the UPEC wild-type strain, and numerous, large focal PMN cells were observed in all tissue sections (intra- and interepithelial sections). Furthermore, PMN cumuli in the pelvis lumen and moderate inflammation of the renal parenchyma were observed (Figure 6). A low pyelonephritis score of 1 was observed in the mutant group, which was characterized by mild inflammation of the renal parenchyma and intraepithelial PMN detection without infiltration into the renal pelvis (Figure 6).
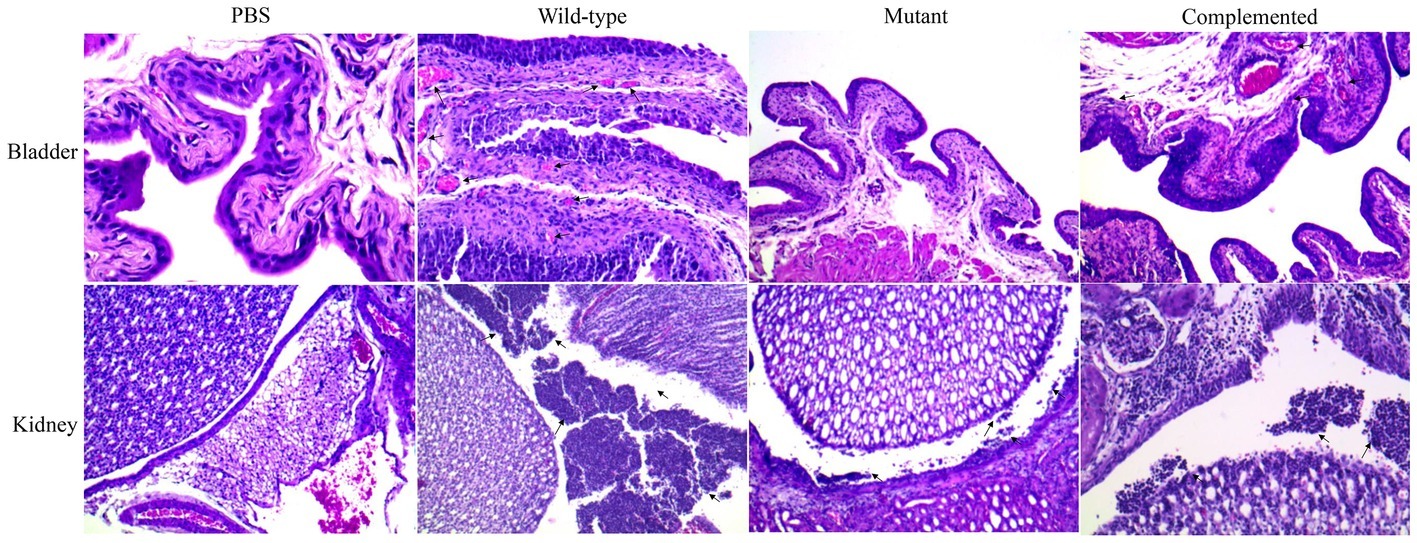
Figure 6. Visualization of damage in the bladder and kidney sections. Tissue samples were paraffin-embedded, sectioned, hematoxylin-eosin-stained, and visualized. Cystitis scores were determined as follows: 0 = no significant lesions; 1 = few and occasional polymorphonuclear (PMN) infiltration in the stroma or lumen, as well as few and occasional perivascular lymphoid microinfiltration; 2 = presence of PMN cells and moderate edema; 3 = many PMN cells and severe edema. Pyelonephritis scores were determined as follows: 0 = no significant lesions; 1 = few and occasional PMN cells in the renal pelvis; 2 = rafts and/or scattered focal aggregates of PMN cells in the renal pelvis, as well as peripelvic inflammation; 3 = presence of numerous and large focal PMN cells in all tissue sections and inflammation extending into the parenchyma.
Curli-Producing Uropathogenic Escherichia coli Modify Cytokine Release in the Mouse Urinary Tract
Cytokine release in the bladder and kidneys of infected mice was quantified to confirm an inflammatory response (Figure 7; Supplementary Figure S4). In wild-type strain-infected mice, IL-6 release in the kidney was significantly increased (p = 0.0001), with a release of 21.4 pg/ml, compared to that of PBS-infected mice; however, a nonsignificant (p = 0.9573) increase was observed in the bladder. In mutant strain-infected mice, an IL-6 release of 227.2 pg/ml was observed in the kidneys, representing a significant increase (p < 0.0001) compared to that in the PBS-infected mice; however, no significant difference in release (p = 0.6618) was observed in the bladder (Figure 7; Supplementary Table S2). We hypothesized that the absence of curli results in the exposure of other structures, such as FimH of type 1 fimbria and PapG of P fimbria. Interestingly, an intranasal (IN) immunization scheme with these recombinant proteins following infection with the mutant strain resulted in a significant decrease (p < 0.0001) in this cytokine (Figure 7; Supplementary Table S2).
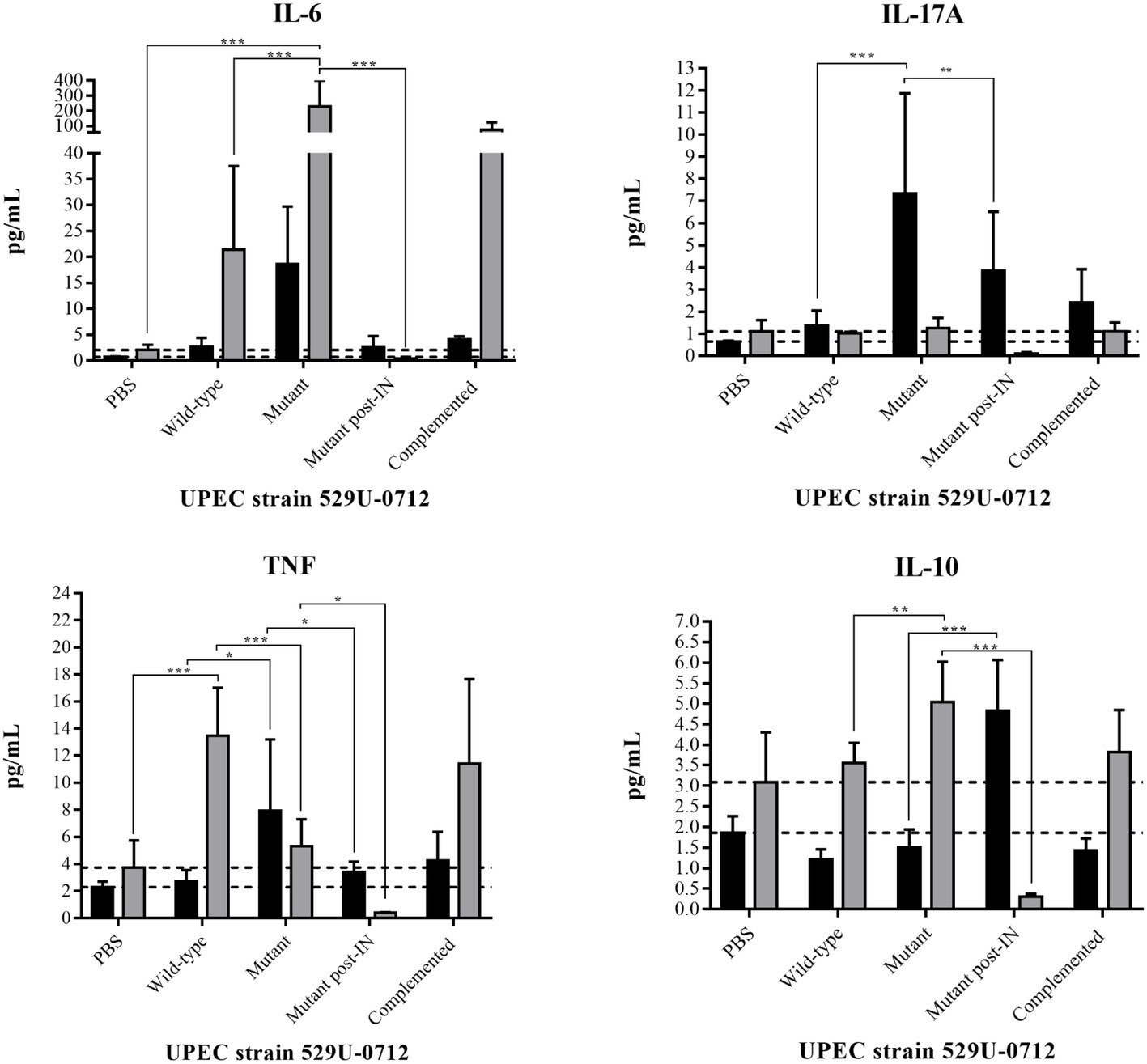
Figure 7. Quantification of cytokine release in the mouse bladder and kidneys. Bladders (black bars) and kidneys (gray bars) were obtained, weighed, sectioned, and sonicated. The lysate organs were centrifuged, and the supernatants were used to quantify the cytokines. The UPEC clinical strain 529U-0712 was defined as the wild-type strain, the UPEC strain 529U-0712 csgA::Cm was defined as the mutant strain, and its complementation with the plasmid pJcsgG-C resulted in the complemented strain. Additionally, IN immunization followed by infection with the mutant strain was included and defined as mutant post-IN. Transurethral inoculation with PBS was used as a control in this experiment. * = 0.05-0.01; ** = 0.01-0.001; *** < 0.001.
Similarly, IL-17 release in the bladder showed a significant increase of 7.3 pg/ml (p < 0.0001), but no significant difference was observed between PBS- and wild-type strain-infected mice. Under the same conditions and using the mutant strain, a decreased IL-17A release of 3.8 pg/ml in the bladder (p = 0.0032) was observed with previous IN immunization with rFimH and rPapG, although no significant changes were observed in the kidneys (Figure 7; Supplementary Table S2).
TNF release was quantified in the kidneys and showed a value of 13.4 pg/ml in wild-type strain-infected mice with p < 0.0001 compared with PBS-infected mice. A significant decrease (p < 0.0001) in this cytokine was identified in the kidneys of mutant strain-infected mice (5.3 pg/ml) and after previous IN immunization with rFimH and rPapG (0.4 pg/ml). In the bladder, a significant increase (p = 0.0057) in TNF release with a value of 7.9 pg/ml was identified in mutant strain-infected mice, but a significant decrease to 3.4 pg/ml was observed post-IN immunization (Figure 7; Supplementary Table S2).
IL-10, an anti-inflammatory cytokine, was also quantified in infected mice, and similar values were obtained in both the bladder and kidneys of PBS- and wild-type strain-infected mice. However, in the kidneys but not in the bladder of mutant strain-infected mice, a significant increase (p = 0.0037) of 3.5–5.0 pg/ml was identified for this cytokine. Interestingly, in IN-immunized and mutant strain-infected mice, this cytokine showed a significant increase (p < 0.0001) of 4.8 pg/ml in the bladder and a decrease (p < 0.0001) of 0.3 pg/ml in the kidneys (Figure 7; Supplementary Table S2). IL-2, IL-4, and interferon (IFN)-γ cytokine release was similar in both the bladder and kidneys between groups of PBS- and wild-type strain-infected mice; however, a significant decrease (p < 0.0001) was observed in both organs after IN immunization, most likely due to the absence of UPEC colonization in the urinary tract (Supplementary Figure S4, Supplementary Table S2).
Discussion
UPEC colonization in the urinary tract depends on virulence and fitness factors, such as fimbriae, iron uptake, toxins, flagella, autotransporter proteins, and the capsule (Subashchandrabose and Mobley, 2015). Curli, a type of amyloid-like fimbriae, are considered a virulence factor due to their role in adherence to bladder cells and biofilm formation (Kikuchi et al., 2005; Cordeiro et al., 2016; Hollenbeck et al., 2018). In this study, we confirmed an association of curli with adherence to human bladder cells using a mutant in the csgA gene from the clinical strain of UPEC (529U-0712), while the wild-type strain was isolated from the urine of a pediatric patient. These data were consistent with those of other studies performed with HTB-9 (human bladder carcinoma), Vero (African green monkey kidney cells), and HUVEC (human umbilical vein) cell lines. In this study, we included a complemented UPEC strain that confirmed the polar noneffect associated with the selection gene and its promoter (Cordeiro et al., 2016; Hollenbeck et al., 2018). Nevertheless, a role of curli in the colonization of the urinary tract in vivo has poorly been described (Kai-Larsen et al., 2010; Nhu et al., 2018). We established a murine model of UTI by transurethral inoculation in C57BL/6 female mice. The noncurliated UPEC strain (mutant) showed a decrease in colonization in the mouse bladder, which was confirmed by histological sections stained with hematoxylin-eosin. However, rCsgA protein-immunized mice showed protection against bladder colonization by the curliated UPEC strain (wild-type). Additionally, CsgA was shown to exist in a polymeric form in the bladder and a monomeric form in the kidneys of infected mice. Urine in the urinary tract has a variable composition; urine from the bladder is more osmolar than urine from the renal pelvis (Cahill et al., 2003). High osmolarity triggers a two-component regulatory system (EnvZ/OmpR), where phosphorylated OmpR binds to the csgDEFG promoter and actives csgD transcription (Prigent-Combaret et al., 2001). The CsgD protein is the positive regulator that activates csgBA transcription, generating high CsgA protein concentrations (Hammar et al., 1995). A high CsgA concentration generates polymerization, forming CsgA oligomers and fibril-like structures (Wang et al., 2007).
Histological sections (with hematoxylin-eosin staining) of the bladder and kidneys from infected mice showed damage and inflammatory processes. Histological changes after UPEC infection were observed, showing inflammatory processes in the perivascular submucosa with intra- and interepithelial PMN cells as well as the presence of adhered and intracellular bacteria. These results indicated acute cystitis when mice were infected with the wild-type strain; however, decreased in damage and in identified bacteria was observed in the bladder lumen of mice infected with the mutant strain. Acute pyelonephritis was observed in mice infected with the wild-type strain and was characterized by pelvis inflammation, inter- and intratubular PMN infiltration, and the presence of bacteria and abnormal access in the renal parenchymal. By contrast, mutant strain-infected mice showed the least severe damage and lowest levels of bacteria in the kidney. Our data confirmed that curli are a colonization factor, which has been described in bladder cell lines by other groups (Kai-Larsen et al., 2010; Cordeiro et al., 2016; Hollenbeck et al., 2018; Nhu et al., 2018). A role of curli in kidney colonization was also shown in a C57BL/6 mouse model of UTI; however, these fimbriae are not involved in adherence to kidney line cells. Curlicides have been shown to attenuate the virulence of the UPEC strain UTI89, showing a decrease in the colonization and intracellular bacterial community in a murine model of UTI (Cegelski et al., 2009). To confirm this finding, we performed IN immunization with rCsgA in a C57BL/6 mouse model, generating antibodies and protection against UPEC colonization. Previously, we showed that polyclonal antibodies against CsgA inhibited the adherence of the UPEC strain CFT073 to the bladder cell line (Luna-Pineda et al., 2016).
The epithelial cells in the urinary tract are the first line of defense and secrete soluble compounds such as proinflammatory cytokines (Abraham and Miao, 2015). Fimbrial adhesins, such as pathogen-associated molecular patterns (PAMP), are associated with cytokine release and are capable of recognizing pattern recognition receptors, including Toll-like receptor (TLR) 4, in the mucosa of the urinary tract (Sirard et al., 2006). CsgA, a structural protein of curli, interacts with the TLR2-TLR1 dimer to produce IL-6 release in marrow-derived macrophages (Rapsinski et al., 2015). Our previous research identified TLR2 and TLR4 expression in the bladder cell line and showed the release of the cytokines IL-6 and IL-8 by rCsgA protein (Luna-Pineda et al., 2016). Mice infected with curliated UPEC strains (wild-type and complemented strains) also released IL-6 in the kidney, but the highest release of this cytokine was found for the mutant strain.
Synergistically, LPS, type 1 fimbriae and P fimbriae mainly activate the release of IL-6 in vitro and in vivo via TLR4 (Frendeus et al., 2001; Hedlund et al., 2001; Samuelsson et al., 2004; Song et al., 2007). According to our data, we hypothesized that curli might hide the other fimbriae and LPS, preventing interaction with TLR4 and the release of IL-6. In addition, we identified specific IgG antibodies against FimH and PapG proteins in mouse urine following IN immunization using rFimH and rPapG (data not shown). Interestingly, IN immunization with these recombinant proteins followed by infection with the mutant strain resulted in a strong decrease in the release of IL-6 in the kidney, indicating that curli hide these fimbriae, which could be an immune response evasion strategy of UPEC. Intracellular UPEC can generate the release of IL-6 in model mice of UTI, which can be decreased by forskolin action, maintaining the extracellular localization of the bacteria (Bishop et al., 2007). This finding could explain why infection with a mutant strain after previous IN immunization (rFimH and rPapG) decreases the release of this cytokine.
IL-17A showed a similar effect in the bladder tissue, but IN immunization resulted in a small decrease in this cytokine. This result suggests that curli could synergistically function with type 1 and P fimbriae in the activation of γδ T cells. These T cells have been identified as the main cells that release IL-17A and have a role in activating the innate immune response in the mouse bladder (Sivick et al., 2010). These data explained why decreased inflammation was observed in histological sections from mouse bladders with little PMN infiltration. Nonetheless, TNF, an inflammatory cytokine, was released in the kidney but not in the bladder when mice were infected with the curliated UPEC strain. Ly6C+ macrophages produce the cytokine TNF in response to infection, which activates resident Ly6C− macrophages to secrete CXCL2 and promote migration of neutrophils (Schiwon et al., 2014). Histological sections showed a high number of PMN cells in both the bladder and kidney. The noncurliated UPEC strain showed a decrease in TNF, while after IN immunization followed by infection with this strain, TNF was absent in the kidney, indicating synergistic activation between curli and other fimbriae (type 1 and P fimbriae). UPEC adheres to NK cells through the type 1 fimbria, activating and releasing TNF as an innate immune response (Gur et al., 2013). In the bladder, a contrary response was identified; when mice were infected with noncurliated UPEC, an increase TNF was detected, but TNF was decreased in post-IN immunization mice. These data also showed that curli might hide the type 1 fimbria, preventing interaction with bladder-resident NK cells and the release of TNF.
IL-10, a master regulator of innate immunity, was identified 2 h postinfection from uroepithelial cells and monocytes and had a protective role in both the bladder and kidney (Duell et al., 2012, 2013). Six hours postinfection, bladder mast cells were shown to release this cytokine, preventing the expression of costimulatory molecules on dendritic cells (Chan et al., 2013). Mice infected with the noncurliated UPEC strain showed the highest release of this cytokine in the kidney, probably due to curli blocking other fimbriae that activate epithelial cells or monocytes 48 h postinfection. Interestingly, IL-10 from the bladder was identified only in mice post-IN immunization that were infected with the noncurliated UPEC strain, confirming that type 1 fimbria was associated with the regulation of this cytokine, as described for the UPEC mutant strain deficient in FimH (Duell et al., 2012). Other cytokines (IL-2, IL-4, and INF-γ) did not show changes in either organ with any UPEC strain.
In conclusion, the curli of UPEC are a fitness factor that enhances colonization in the urinary tract and could be considered a strategy for evasion of the immune system; however, it is necessary to perform other experiments to confirm this hypothesis in the future.
Data Availability
All datasets generated for this study are included in the manuscript and/or the Supplementary Files.
Ethics Statement
The animal study was reviewed and approved by Research, Ethics and Biosecurity Committees of “Hospital Infantil de México Federico Gómez.”
Author Contributions
VL-P and JX-C wrote the main manuscript. VL-P, VC-D, DI-A, and LM-F prepared Figures 1–3, 5. VL-P, SO, AR-L, and PV-M prepared Figures 4, 6. VL-P, AC-C, DI-A, and LM-F prepared Figure 7. VL-P, VC-D, SO, and AC-C prepared the supplementary information. VL-P and JX-C reviewed the manuscript.
Funding
This work was supported by Public Federal Funds grants with the numbers HIM/2016/099 SSA.1329, HIM/2017/002 SSA.1298, HIM/2017/136 SSA.1456, HIM/2017/137 SSA.1432, HIM 2017-107FF SSA.1421, HIM/2018/072 SSA.1527 and HIM/2018/045FF SSA.1503 from the HIMFG. This work was also supported by Consejo Nacional de Ciencia y Tecnología (CONACYT) at Attention to National Problems under account number CONACyT 2015-01-1764.
Conflict of Interest Statement
The authors declare that the research was conducted in the absence of any commercial or financial relationships that could be construed as a potential conflict of interest.
Acknowledgments
We thank Mextli Y. Bermejo-Haro and Marco A. Flores-Oropeza for their technical assistance. VL-P was supported by a postdoctoral fellowship from Dirección General de Asuntos del Personal Académico (DGAPA) at the Universidad Nacional Autónoma de México (UNAM) under the direction of the Dra. Moreno-Fierro in Facultad de Estudios Superiores Iztacala-UNAM, as well as by a scholarship from Fundación Alberto y Dolores Andrade, México City. The micrographs were obtained by Dr. Edgar Oliver López-Villegas in the Centro de Instrumentación de Microscopía of the Escuela Nacional de Ciencias Biologicas of INP.
Supplementary Material
The Supplementary Material for this article can be found online at: https://www.frontiersin.org/articles/10.3389/fmicb.2019.02063/full#supplementary-material
Footnotes
References
Abraham, S. N., and Miao, Y. (2015). The nature of immune responses to urinary tract infections. Nat. Rev. Immunol. 15, 655–663. doi: 10.1038/nri3887
Anderson, G. G., Palermo, J. J., Schilling, J. D., Roth, R., Heuser, J., and Hultgren, S. J. (2003). Intracellular bacterial biofilm-like pods in urinary tract infections. Science 301, 105–107. doi: 10.1126/science.1084550
Barnhart, M. M., and Chapman, M. R. (2006). Curli biogenesis and function. Annu. Rev. Microbiol. 60, 131–147. doi: 10.1146/annurev.micro.60.080805.142106
Bishop, B. L., Duncan, M. J., Song, J., Li, G., Zaas, D., and Abraham, S. N. (2007). Cyclic AMP-regulated exocytosis of Escherichia coli from infected bladder epithelial cells. Nat. Med. 13, 625–630. doi: 10.1038/nm1572
Cahill, D. J., Fry, C. H., and Foxall, P. J. (2003). Variation in urine composition in the human urinary tract: evidence of urothelial function in situ? J. Urol. 169, 871–874. doi: 10.1097/01.ju.0000052404.42651.55
Cardiff, R. D., Miller, C. H., and Munn, R. J. (2014). Manual hematoxylin and eosin staining of mouse tissue sections. Cold Spring Harb. Protoc. 6, 655–658. doi: 10.1101/pdb.prot073411
Cegelski, L., Pinkner, J. S., Hammer, N. D., Cusumano, C. K., Hung, C. S., Chorell, E., et al. (2009). Small-molecule inhibitors target Escherichia coli amyloid biogenesis and biofilm formation. Nat. Chem. Biol. 5, 913–919. doi: 10.1038/nchembio.242
Chan, C. Y., St John, A. L., and Abraham, S. N. (2013). Mast cell interleukin-10 drives localized tolerance in chronic bladder infection. Immunity 38, 349–359. doi: 10.1016/j.immuni.2012.10.019
Cordeiro, M. A., Werle, C. H., Milanez, G. P., and Yano, T. (2016). Curli fimbria: an Escherichia coli adhesin associated with human cystitis. Braz. J. Microbiol. 47, 414–416. doi: 10.1016/j.bjm.2016.01.024
Datsenko, K. A., and Wanner, B. L. (2000). One-step inactivation of chromosomal genes in Escherichia coli K-12 using PCR products. Proc. Natl. Acad. Sci. USA 97, 6640–6645. doi: 10.1073/pnas.120163297
Duell, B. L., Carey, A. J., Dando, S. J., Schembri, M. A., and Ulett, G. C. (2013). Human bladder uroepithelial cells synergize with monocytes to promote IL-10 synthesis and other cytokine responses to uropathogenic Escherichia coli. PLoS One 8:e78013. doi: 10.1371/journal.pone.0078013
Duell, B. L., Carey, A. J., Tan, C. K., Cui, X., Webb, R. I., Totsika, M., et al. (2012). Innate transcriptional networks activated in bladder in response to uropathogenic Escherichia coli drive diverse biological pathways and rapid synthesis of IL-10 for defense against bacterial urinary tract infection. J. Immunol. 188, 781–792. doi: 10.4049/jimmunol.1101231
Flores-Mireles, A. L., Walker, J. N., Caparon, M., and Hultgren, S. J. (2015). Urinary tract infections: epidemiology, mechanisms of infection and treatment options. Nat. Rev. Microbiol. 13, 269–284. doi: 10.1038/nrmicro3432
Frendeus, B., Wachtler, C., Hedlund, M., Fischer, H., Samuelsson, P., Svensson, M., et al. (2001). Escherichia coli P fimbriae utilize the toll-like receptor 4 pathway for cell activation. Mol. Microbiol. 40, 37–51. doi: 10.1046/j.1365-2958.2001.02361.x
Gur, C., Coppenhagen-Glazer, S., Rosenberg, S., Yamin, R., Enk, J., Glasner, A., et al. (2013). Natural killer cell-mediated host defense against uropathogenic E. coli is counteracted by bacterial hemolysinA-dependent killing of NK cells. Cell Host Microbe 14, 664–674. doi: 10.1016/j.chom.2013.11.004
Hammar, M. R., Arnqvist, A., Bian, Z., Olsén, A., and Normark, S. (1995). Expression of two csg operons is required for production of fibronectin- and Congo red-binding curli polymers in Escherichia coli K-12. Mol. Microbiol. 18, 661–670. doi: 10.1111/j.1365-2958.1995.mmi_18040661.x
Hannan, T. J., and Hunstad, D. A. (2016). A murine model for Escherichia coli urinary tract infection. Methods Mol. Biol. 1333, 159–175. doi: 10.1007/978-1-4939-2854-5_14
Hedlund, M., Frendeus, B., Wachtler, C., Hang, L., Fischer, H., and Svanborg, C. (2001). Type 1 fimbriae deliver an LPS- and TLR4-dependent activation signal to CD14-negative cells. Mol. Microbiol. 39, 542–552. doi: 10.1046/j.1365-2958.2001.02205.x
Hollenbeck, E. C., Antonoplis, A., Chai, C., Thongsomboon, W., Fuller, G. G., and Cegelski, L. (2018). Phosphoethanolamine cellulose enhances curli-mediated adhesion of uropathogenic Escherichia coli to bladder epithelial cells. Proc. Natl. Acad. Sci. USA 115, 10106–10111. doi: 10.1073/pnas.1801564115
Hufnagel, D. A., Depas, W. H., and Chapman, M. R. (2015). The biology of the Escherichia coli extracellular matrix. Microbiol. Spectr. 3, 249–273. doi: 10.1128/microbiolspec.MB-0014-2014
Hung, C. S., Dodson, K. W., and Hultgren, S. J. (2009). A murine model of urinary tract infection. Nat. Protoc. 4, 1230–1243. doi: 10.1038/nprot.2009.116
Hung, C., Marschall, J., Burnham, C.-A. D., Byun, A. S., and Henderson, J. P. (2014). The bacterial amyloid curli is associated with urinary source bloodstream infection. PLoS One 9:e86009. doi: 10.1371/journal.pone.0086009
Kai-Larsen, Y., Luthje, P., Chromek, M., Peters, V., Wang, X., Holm, A., et al. (2010). Uropathogenic Escherichia coli modulates immune responses and its curli fimbriae interact with the antimicrobial peptide LL-37. PLoS Pathog. 6:e1001010. doi: 10.1371/journal.ppat.1001010
Kikuchi, T., Mizunoe, Y., Takade, A., Naito, S., and Yoshida, S. (2005). Curli fibers are required for development of biofilm architecture in Escherichia coli K-12 and enhance bacterial adherence to human uroepithelial cells. Microbiol. Immunol. 49, 875–884. doi: 10.1111/j.1348-0421.2005.tb03678.x
Kudinha, T., Johnson, J. R., Andrew, S. D., Kong, F., Anderson, P., and Gilbert, G. L. (2013). Genotypic and phenotypic characterization of Escherichia coli isolates from children with urinary tract infection and from healthy carriers. Pediatr. Infect. Dis. J. 32, 543–548. doi: 10.1097/INF.0b013e31828ba3f1
Lim, J. Y., Pinkner, J. S., and Cegelski, L. (2014). Community behavior and amyloid-associated phenotypes among a panel of uropathogenic E. coli. Biochem. Biophys. Res. Commun. 443, 345–350. doi: 10.1016/j.bbrc.2013.11.026
Luna-Pineda, V. M., Ochoa, S. A., Cruz-Córdova, A., Cázares-Domínguez, V., Reyes-Grajeda, J. P., Flores-Oropeza, M. A., et al. (2018b). Features of urinary Escherichia coli isolated from children with complicated and uncomplicated urinary tract infections in Mexico. PLoS One 13:e0204934. doi: 10.1371/journal.pone.0204934
Luna-Pineda, V. M., Ochoa, S., Cruz-Cordova, A., Cazares-Dominguez, V., Velez-Gonzalez, F., Hernandez-Castro, R., et al. (2018a). Urinary tract infections, immunity, and vaccination. Bol. Med. Hosp. Infant. Mex. 75, 67–78. doi: 10.24875/bmhim.m18000011
Luna-Pineda, V. M., Reyes-Grajeda, J. P., Cruz-Cordova, A., Saldana-Ahuactzi, Z., Ochoa, S. A., Maldonado-Bernal, C., et al. (2016). Dimeric and trimeric fusion proteins generated with fimbrial adhesins of uropathogenic Escherichia coli. Front. Cell. Infect. Microbiol. 6:135. doi: 10.3389/fcimb.2016.00135
Nhu, N. T. K., Phan, M. D., Peters, K. M., Lo, A. W., Forde, B. M., Min Chong, T., et al. (2018). Discovery of new genes involved in Curli production by a Uropathogenic Escherichia coli strain from the highly virulent O45:K1:H7 lineage. MBio 9. pii: e01462–18. doi: 10.1128/mBio.01462-18
Norinder, B. S., Koves, B., Yadav, M., Brauner, A., and Svanborg, C. (2012). Do Escherichia coli strains causing acute cystitis have a distinct virulence repertoire? Microb. Pathog. 52, 10–16. doi: 10.1016/j.micpath.2011.08.005
Ochoa, S. A., Cruz-Córdova, A., Luna-Pineda, V. M., Reyes-Grajeda, J. P., Cázares-Domínguez, V., Escalona, G., et al. (2016). Multidrug- and extensively drug-resistant uropathogenic Escherichia coli clinical strains: phylogenetic groups widely associated with integrons maintain high genetic diversity. Front. Microbiol. 7:2042. doi: 10.3389/fmicb.2016.02042
Prigent-Combaret, C., Brombacher, E., Vidal, O., Ambert, A., Lejeune, P., Landini, P., et al. (2001). Complex regulatory network controls initial adhesion and biofilm formation in Escherichia coli via regulation of the csgD gene. J. Bacteriol. 183, 7213–7223. doi: 10.1128/JB.183.24.7213-7223.2001
Rapsinski, G. J., Wynosky-Dolfi, M. A., Oppong, G. O., Tursi, S. A., Wilson, R. P., Brodsky, I. E., et al. (2015). Toll-like receptor 2 and NLRP3 cooperate to recognize a functional bacterial amyloid, curli. Infect. Immun. 83, 693–701. doi: 10.1128/IAI.02370-14
Samuelsson, P., Hang, L., Wullt, B., Irjala, H., and Svanborg, C. (2004). Toll-like receptor 4 expression and cytokine responses in the human urinary tract mucosa. Infect. Immun. 72, 3179–3186. doi: 10.1128/IAI.72.6.3179-3186.2004
Schiwon, M., Weisheit, C., Franken, L., Gutweiler, S., Dixit, A., Meyer-Schwesinger, C., et al. (2014). Crosstalk between sentinel and helper macrophages permits neutrophil migration into infected uroepithelium. Cell 156, 456–468. doi: 10.1016/j.cell.2014.01.006
Sirard, J. C., Bayardo, M., and Didierlaurent, A. (2006). Pathogen-specific TLR signaling in mucosa: mutual contribution of microbial TLR agonists and virulence factors. Eur. J. Immunol. 36, 260–263. doi: 10.1002/eji.200535777
Sivick, K. E., Schaller, M. A., Smith, S. N., and Mobley, H. L. (2010). The innate immune response to uropathogenic Escherichia coli involves IL-17A in a murine model of urinary tract infection. J. Immunol. 184, 2065–2075. doi: 10.4049/jimmunol.0902386
Song, J., Duncan, M. J., Li, G., Chan, C., Grady, R., Stapleton, A., et al. (2007). A novel TLR4-mediated signaling pathway leading to IL-6 responses in human bladder epithelial cells. PLoS Pathog. 3:e60. doi: 10.1371/journal.ppat.0030060
Subashchandrabose, S., and Mobley, H. L. T. (2015). Virulence and fitness determinants of uropathogenic Escherichia coli. Microbiol. Spectr. 3, 1–20. doi: 10.1128/microbiolspec.UTI-0015-2012
Trautner, B. W., and Darouiche, R. O. (2004). Role of biofilm in catheter-associated urinary tract infection. Am. J. Infect. Control 32, 177–183. doi: 10.1016/j.ajic.2003.08.005
Keywords: uropathogenic Escherichia coli, curli, urinary tract infection, fitness factor, CsgA protein
Citation: Luna-Pineda VM, Moreno-Fierros L, Cázares-Domínguez V, Ilhuicatzi-Alvarado D, Ochoa SA, Cruz-Córdova A, Valencia-Mayoral P, Rodríguez-Leviz A and Xicohtencatl-Cortes J (2019) Curli of Uropathogenic Escherichia coli Enhance Urinary Tract Colonization as a Fitness Factor. Front. Microbiol. 10:2063. doi: 10.3389/fmicb.2019.02063
Edited by:
Sven Hammerschmidt, University of Greifswald, GermanyReviewed by:
Jason John Paxman, La Trobe University, AustraliaTimothy James Wells, University of Queensland, Australia
Copyright © 2019 Luna-Pineda, Moreno-Fierros, Cázares-Domínguez, Ilhuicatzi-Alvarado, Ochoa, Cruz-Córdova, Valencia-Mayoral, Rodríguez-Leviz and Xicohtencatl-Cortes. This is an open-access article distributed under the terms of the Creative Commons Attribution License (CC BY). The use, distribution or reproduction in other forums is permitted, provided the original author(s) and the copyright owner(s) are credited and that the original publication in this journal is cited, in accordance with accepted academic practice. No use, distribution or reproduction is permitted which does not comply with these terms.
*Correspondence: Juan Xicohtencatl-Cortes, juanxico@yahoo.com